- 1Laboratory of Medical Microbiology and Infectious Diseases, School of Biosciences and Veterinary Medicine, University of Camerino, Matelica, Italy
- 2Veterinary Teaching Hospital, School of Biosciences and Veterinary Medicine, University of Camerino, Matelica, Italy
- 3Pathology Laboratory, Department of Veterinary Sciences, University of Messina, Messina, Italy
- 4Pathology Laboratory, School of Biosciences and Veterinary Medicine, University of Camerino, Matelica, Italy
This in vitro study was carried out to evaluate the potential antibacterial properties of canine non-transfusional hemo-components. Therapeutic formulations commonly used for regenerative medicine purposes (platelet-rich plasma, platelet gel, platelet lysate, fibrin glue), considering both leukocyte-rich and leukocyte-poor formulations, but also platelet-poor plasma and activating substances (thrombin, calcium gluconate), were tested to detect elements with potential antimicrobial properties. The antibacterial effect was tested on different bacterial strains (Staphylococcus aureus subspecies aureus, Staphylococcus cohnii subspecies cohnii, Escherichia coli, Pseudomonas aeruginosa, and Klebsiella pneumoniae subspecies pneumoniae) isolated from canine wounds and classified as susceptible, multidrug-, extensively, and pandrug-resistant bacteria toward a known panel of human and veterinary antibiotics. The evaluation was carried out by agar gel diffusion method (Kirby–Bauer) and micro-inhibition in broth using microplates and spectrophotometer reading. The study findings confirmed the hypothesized antibacterial properties of canine non-transfusional hemo-components. A more effective bacteriostatic effect was found against Gram-negative bacteria, drug-resistant too. The presence of leukocytes or platelets does not appear to be essential for the antibacterial effect. Further studies should be conducted to evaluate the exact mechanism of action of the antimicrobial activity. However, non-transfusional hemo-components could be a useful natural aid in controlling bacterial infections in dogs.
Introduction
Antimicrobial resistance (AMR) is a multifaceted topic at the interface of human, animal, and plant health, food hygiene, and environmental sciences, resulting to be a major global public health issue of the 21st century (1, 2).
To counter this increasing phenomenon, both in human and in veterinary medicine, more and more scientists are looking for molecules that can assist or replace the action of antibiotics. The topical use of non-transfusional hemo-components or autologous platelet concentrates (PCs), including platelet-rich plasma (PRP), platelet gel (PG), platelet lysate (PL), and fibrin glue (FG), has gained great popularity during the past 20 years; thus, they have been used, mainly for their regenerative properties, in a variety of human medical fields such as orthopedics (3–7), wound healing (8–12), ophthalmology (13–15), and dentistry (16–20). Despite a recent growing interest, fewer in vivo and in vitro studies in dogs have been published (21–26).
While the potential regenerative effect of PCs has been extensively studied, fewer studies have investigated the possible relationship with bacterial growth. Some authors have empirically supposed that PCs could promote infections, associating them with microbiological culture media and acting as a substrate for the growth of bacteria (27). More recently, on the contrary to this theory, some evidence would attribute to PCs an inhibitory effect on bacterial growth, although the results cannot be considered definitive yet (27–31). The antibacterial properties of PCs are supported by some in vitro studies conducted mostly in humans (28, 30, 32–50), but also in horses (31, 51, 52) and rabbits (53). Systematic reviews of current literature considering in vitro, preclinical, and clinical studies have shown that PCs may have antibacterial properties (54–56).
The antibacterial effect of PCs has also been recently highlighted in in vivo studies on surgical wounds (57), sternotomy wounds (58, 59), osteomyelitis (60–62), spinal infections (63), and bacterial cystitis (64), while another in vivo study showed controversial results (65). Since infection control is a prerequisite for the wound healing process and for avoiding the chronicization event, recently, in a small controlled clinical trial performed in dogs, MRSA-infected skin wounds treated with PRP showed an accelerated healing process with rapid re-epithelialization and granulation tissue formation, reduction of inflammation, and decrease of bacterial loads (66).
Until now, the components of the PCs that control antimicrobial activity have not been fully understood, so the mechanisms of PC interaction with bacteria need further investigation.
In order to detect any elements potentially endowed with antimicrobial properties, we explored the antimicrobial activity of PCs (PRP, PG, PL, FG) against susceptible (S), multidrug-resistant (MDR) extensively drug-resistant (XDR), and pandrug-resistant (PDR) Gram-positive and Gram-negative microorganisms isolated from canine wounds. In this set of in vitro investigations, leukocyte-rich (L-PRP, L-PG) and leukocyte-poor formulations (P-PRP, P-PG), but also platelet-poor plasma (L-PPP, P-PPP) and intermediate elements of the PC production process (thrombin, calcium gluconate), were evaluated.
Materials and Methods
Animals and Blood Collection
The study was performed complying with the Animal Welfare Body of the University of Camerino (protocol number E81AC.10/A) according to the National Legislative Decree n. 26/2014, implementation of EU Directive 2010/63/EU. Blood was collected from five owned dogs at the Veterinary Teaching Hospital, School of Biosciences and Veterinary Medicine, University of Camerino. The owner's informed consent was obtained for participation in the study. All animals were between 1 and 10 years of age, had a minimum body weight of 15 kg, and belonged to different dog breeds (three mixed breed, one Labrador retriever, one English setter). They had no noteworthy medical problems or medical history, except for degenerative joint disease, and did not take any medications. An articular infiltration of autologous PRP was practiced in each dog for the management of degenerative joint disease; an aliquot of whole blood in excess of the amount needed for PRP preparation was used for the in vitro investigations of the present study. Each dog, upon admission, had a complete physical examination, complete blood count, and serum biochemical profile. No animals had platelet disorders or took anticoagulant and antibiotic drugs.
Preparation of Hemo-Components
In each dog, fresh autologous whole blood (50 ml) was collected from the jugular vein using a 60-ml syringe containing anticoagulant citrate dextrose solution A (ACD-A: SALF SpA, Cenate Sotto, Bergamo, Italy) in a ratio of 1:9. Blood collection and preparation procedures were performed as described by Tambella and collaborators (22) in aseptic conditions through a laminar flow cabin (Bicasa, Bernareggio, MB, Italy) and following the Good Laboratory Practices. The hemo-components were obtained from a pool of fresh canine whole blood. Prior to preparation of each hemo-component, an aliquot was used to determine the blood cell count.
Autologous Thrombin
The thrombin-rich solution was obtained from autologous whole blood which was centrifuged (Rotina 46R, Hettich, Milan, Italy) at 650 g for 10 min. The plasma supernatant fraction was mixed with 10% calcium gluconate (B. Braun, Melsungen, Germany) in a ratio of 5:1 and incubated (cooled incubator IL 23R, VWR Incu-Line, Milan, Italy) at 37°C for 30 min. The resulting clot was crushed, and the final supernatant, the thrombin-rich solution, was collected for PRP activation.
Leukocyte-Rich -PPP, -PRP, and -PG
As described by Tambella et al. (22), leukocyte-rich platelet-poor plasma (L-PPP), leukocyte PRP (L-PRP), and leukocyte PG (L-PG) were obtained by a double-spin technique. Whole blood was centrifuged (Rotina 46R, Hettich, Milan, Italy) at 180 g for 20 min. To stratify the concentrated platelet pellet in the bottom layer, and platelet poor plasma (L-PPP) in the supernatant layer, the plasma with the buffy coat layer was centrifuged at 650 g for 15 min. A half amount of L-PPP was picked up and used for the in vitro study. By resuspending the PCs in the residual part of PPP, the L-PRP was obtained. A half amount of L-PRP was used for the in vitro study; to obtain L-PG, the residual L-PRP was transferred in sterile glass Petri dishes and mixed with the thrombin-rich solution and the calcium gluconate in a volumetric ratio of 8:1:0.5. The L-PG production was achieved at room temperature in 5 min.
Leukocyte-Poor (Pure) -PPP, -PRP, and -PG
Pure platelet-poor plasma (P-PPP), pure platelet-rich plasma (P-PRP), and pure platelet gel (P-PG) were obtained by a double-spin technique. In order to reduce the leukocyte concentration as much as possible, the first centrifugation of whole blood was performed at 500 g for 20 min using tubes (VF108SAS Venosafe, Terumo Europe NV, Leuven, Belgium) containing a gel enabling the separation of the different blood components. The plasma fraction was then centrifuged at 2,000 g for 10 min to achieve separation of P-PPP (the supernatant half-fraction) from P-PRP (the residual half-fraction). Similarly, P-PG was obtained by mixing P-PRP with the autologous thrombin-rich solution and calcium gluconate.
FG
Fibrinogen concentrate, the precursor of FG, was obtained following the method proposed by Tarantino et al. (67). This method is characterized by physical separation (centrifugation) and the presence of a special membrane, capable of concentrating fibrinogen. After centrifugation of whole blood (2,000 g for 10 min), the plasma fraction obtained without buffy coat was transferred in special tubes (Amicon Ultra 15, Merck Millipore Ltd, Tullagreen, Carrigtwohill, Co Cork, Ireland) with a 100,000 MWCO (molecular weight cutoff) porosity filter. Then, tubes were centrifuged at 3,600 g for 45 min and the fibrinogen concentrate above the filter was obtained.
PL
PL was produced as reported in previous studies with some modifications (68, 69). Using a two-step centrifugation protocol (180 g for 20 minutes and 1,500 g for 10 minutes), packed PC was produced from whole blood with ACD-A. To remove platelet membranes and other cellular debris and obtain the PL, three repeated cycles of freezing (at −20°C) and thawing (at room temperature) followed by centrifugation at 2,500 g for 20 min were undergone obtaining the PL as supernatant.
Evaluation of Antibacterial Activity
Bacterial Strains and Their Antibiotic Susceptibility Evaluation
To compare the antibacterial effect of each hemo-component, strains isolated from canine wounds were selected from the bacterial collection of the Laboratory of Microbiology and Infectious Diseases, School of Bioscience and Veterinary Medicine, University of Camerino, based on their ability to cause also infections in the surgical setting.
Three different frozen strains of Staphylococcus aureus subspecies aureus, one Staphylococcus cohnii subspecies cohnii, three Klebsiella pneumoniae subspecies pneumoniae, two Pseudomonas aeruginosa, and three Escherichia coli strains were thawed and reconstituted. In addition, Staphylococcus aureus subspecies aureus ATCC® 43300™ strain was used as control. After pre-enrichment in Tryptic Soy Broth (TSB) (Liofilchem, Roseto degli Abruzzi, Italy) and incubation at 37°C for 6 h in aerobic condition, a loop was shown on Columbia Blood Agar (Liofilchem, Italy), Mannitol Salt Agar (Liofilchem, Italy), MacConkey Agar (Liofilchem, Italy), and Pseudomonas Cetrimide Agar (Liofilchem, Italy) and incubated aerobically at 37°C for 24 h. Colonies were identified and confirmed by MALDI-TOF MS (Bruker Daltonics, Hamburg, Germany).
In order to determine their antibiotic susceptibility profile and classify the strains as susceptible, MDR, XDR, and PDR (70), antimicrobial susceptibility testing toward 16 commercial human and veterinary antibiotics, belonging to eight different classes, was performed according to the Clinical and Laboratory Standards Institute and EUCAST guidelines (71, 72).The standard disk diffusion method (Kirby–Bauer test) was used to test amoxicillin and clavulanic acid (AUG 30 μg), penicillin (P 1 UI), oxacillin (OX 1 μg), cefadroxil (CDX 30 μg), cefoxitin (CFX 30 μg), cefquinome (CEQ 30 μg), enrofloxacin (ENR 5 μg), gentamicin (CN 10 μg), sulfamethoxazole and trimethoprim (SXT 25 μg), tetracycline (TE 30 μg), amikacin (AK 30 μg), streptomycin (S 300 μg), metronidazole (M 50 μg), and polymyxin B (PB 300 μg). The E-test method was used for Staphylococcus strains to determine the MICs against vancomycin (VA), and teicoplanin (TEC), as described by the manufacturer (MIC Test Strip, Liofilchem, Italy).
Antibacterial in vitro Evaluation of Hemo-Components
To evaluate the antibacterial effect of different hemo-components and activating agents (PL, FG, thrombin, L-PPP, P-PPP, L-PRP with calcium gluconate, P-PRP with calcium gluconate, L-PRP with thrombin, P-PRP with thrombin, L-PG 35 μl, P-PG 35 μl, L-PG 500 μl, and P-PG 500 μl), the Kirby–Bauer disk diffusion method and the broth inhibition by microtiter method were performed.
Kirby–Bauer Disk Diffusion Method
Following the CLSI (2018) recommendations (71), each microorganism was suspended in sterile saline solution (Thermo Fisher, Milan, Italy) to obtain an optical density equal to 0.5 McFarland (1 × 108 CFU/ml), verified at 550 nm by a spectrophotometer (Jenway, Genova Nano, Bibby Scientific, Staffordshire, UK). A sterile cotton swab was dipped into the inoculum suspension and the excess fluid removed by turning the swab against the inside of the tube to avoid over-inoculation of plates. The inoculum was spread evenly over the entire surface of the Mueller Hinton II agar plate (MH; Liofilchem, Italy) by swabbing in three directions. Each hemo-component was placed by a micropipette (PIPETMAN L P20L 2–20 μl, Gilson, Milan, Italy) on the surface of the media, in contact with the microorganisms to reduce as much as possible the variables that could influence their actions against bacteria. Specifically, four different Petri dishes were used to test hemo-components with and without leukocytes against each microorganism.
The amount of each liquid hemo-component was 8 μl, except for PG, tested at 35 and 500 μk, in the pre-jellification phase. Positive controls were performed inoculating the microbial suspension (McFarland 0.5) on MH (Liofilchem, Italy) alone, whereas the same media inoculated with saline solution or hemo-components, with and without leukocytes only, represented the negative controls. The assay was conducted in duplicate and repeated twice. All plates were incubated at 37°C aerobically and evaluated after 4, 8, and 24 h. For each strain, areas (mm) with bacterial inhibition or areas with few bacterial colonies were compared to the respective positive control.
Broth Inhibition by Microtiter Method
The antibacterial activity was determined using the broth inhibition by microtiter method. Bacterial strains have been grown in Tryptic Soy Broth (Liofilchem, Italy) at 37°C for 6 h, aerobically. The final inoculum concentration was adjusted using a spectrophotometer (Jenway, Genova Nano, Bibby Scientific, Staffordshire, UK) to 1 × 104 CFU/ml (OD540nm), and 20 μl of each bacterial suspension was inoculated into a 96-well microtiter plate (Sero-Wel®, Bibby Sterilin Ltd., UK) containing 10 μl of each hemo-component (PL, FG, L-PRP, P-PRP, L-PRP plus thrombin, P-PRP plus thrombin, L-PRP plus calcium gluconate, P-PRP plus calcium gluconate, L-PPP, P-PPP) and 170 μl of sterile TSB. Quantities of 10, 20, 40, and 180 μl were used for pre-jellification L-PG and P-PG to test the antimicrobial activity toward 20 μl of each bacterial suspension. Each well was tested with the respective negative control represented by the same quantities of the hemo-components only. Positive controls with 20 μl of each bacterial suspension in 170 μl of sterile TSB were considered. As a blank negative control, an amount of 170 μl of sterile TSB was placed in the first and last wells of each plate. In addition, wells containing only each hemo-component were used as negative controls. No 0.01% acetic acid was added to prevent peptide aggregation and release of platelet contents. The microtiter plates were incubated at 37°C aerobically under slow and continuous agitation (300 g/minute) (Asal 715, Milano, Italy) and evaluated after 4, 18, and 24 h of incubation. A visual assessment (48) was conducted after 4 h, while at 18 and 24 h the observations were read using a spectrophotometer (Multiskan Ascent, Thermo Scientific, Waltham, MA, USA), at a wavelength of 540 nm. The test was repeated twice.
Statistical Analysis
All experiments were performed in duplicate. Statistical differences between quantitative variables (mean OD540nm) were evaluated with Student's t-test. To determine the antimicrobial activity, the mean optical density (OD540nm) value recorded for each microorganism and the hemo-component, less the OD540nm value recorded for each hemo-component, was compared to the respective positive controls recorded at the same time. Mean OD values for the different bacteria (susceptible or drug-resistant Gram-positive and Gram-negative strains) and recorded at different times (18–24 h) were analyzed with and without the leukocyte component.
Finally, the mean OD values of bacterial concentrations in the presence of hemo-components with leukocytes were compared with the mean OD values observed in the presence of hemo-components without leukocytes. STATA version 13.0 (STATA Corporation, College Station, TX, USA) was used to conduct the statistical analysis. A p value less than 0.05 was considered statistically significant.
Results
Preparation of Hemo-Components and Whole Blood and Hemo-Component Cell Counts
No technical problems occurred during the preparation of non-transfusional hemo-components. Each hemo-component was easily produced using common laboratory tools and was ready for use in this in vitro study. Cell concentrations were appropriate for the specific characteristics requested for each hemo-component.
In L-PRP and L-PG, platelet concentrations increased by 4.3-fold and leukocyte concentrations increased 2.1-fold compared with whole blood (WB) baseline values.
In L-PPP, platelet count decreased approximately 142-fold compared with L-PRP and leukocytes decreased approximately 45-fold.
As expected, the pure formulations (P-PRP, P-PG, and P-PPP) were leukocyte-depleted. Platelet concentrations in P-PRP and P-PG increased 2.2-fold from WB.
Both platelet and leukocyte concentrations were very low in P-PPP and FG.
There were no detectable blood cells in PL.
The mean number of platelets and white blood cells obtained by blood count analysis from the WB pool and the different hemo-components are reported in Table 1.

Table 1. Leukocyte and platelet concentrations obtained from whole blood (pool of 250 ml from five dogs) and each hemo-component.
Bacterial Strains and Their Antibiotic Susceptibility Evaluation
Among the selected Gram-positive and Gram-negative microorganisms, different susceptibility profiles were observed and classified (Table 2) (70). One strain of S. aureus subspecies aureus and S. cohnii subspecies cohnii, one strain of K. pneumoniae subspecies pneumoniae, and one strain of E. coli resulted to be susceptible to the panel of antibiotics, and one strain of S. aureus subspecies aureus, P. aeruginosa, K. pneumoniae ssp. pneumoniae, and E. coli were MDR, while one strain of S. aureus subspecies aureus, K. pneumoniae ssp. pneumoniae, and E. coli resulted to be extensively drug-resistant. Only one strain of P. aeruginosa was PDR.
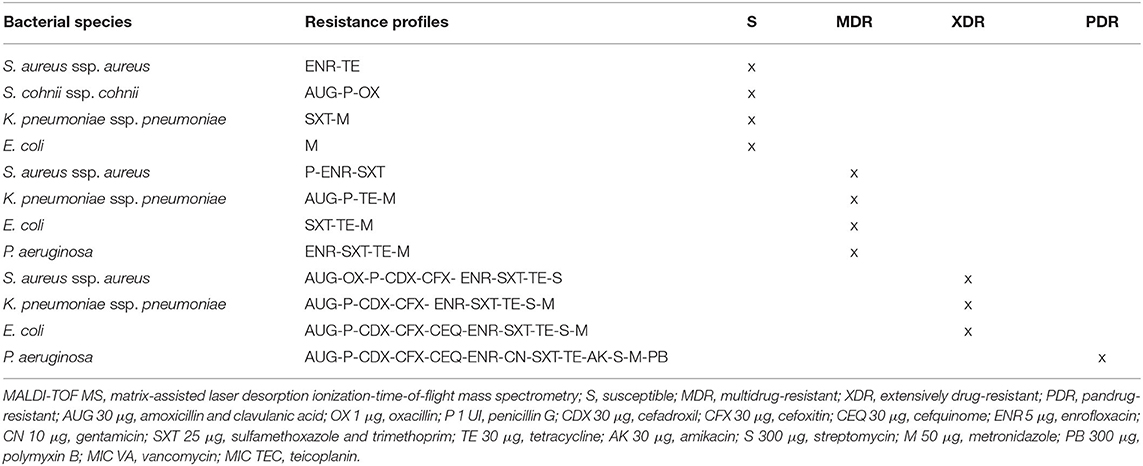
Table 2. Antimicrobial resistance profiles of microorganisms selected and identified by MALDI-TOF MS from canine wounds.
Antibacterial Effect Evaluation of the Hemo-Components
Kirby–Bauer Method
After 4 h of incubation at 37°C under aerobic conditions, no inhibition zones were observed for Gram-positive bacteria. Among Gram-negative microorganisms, some inhibition zones were observed for susceptible K. pneumoniae strains cultured with P-PG and L-PG. In particular, increased mean inhibition zones of 2.5 × 4.5 mm, 7.5 × 10 mm, and 8.5 × 50 mm were recorded for P-PG 35 μl, P-PG 500 μl, and L-PG 500 μl, respectively (Figure 1).
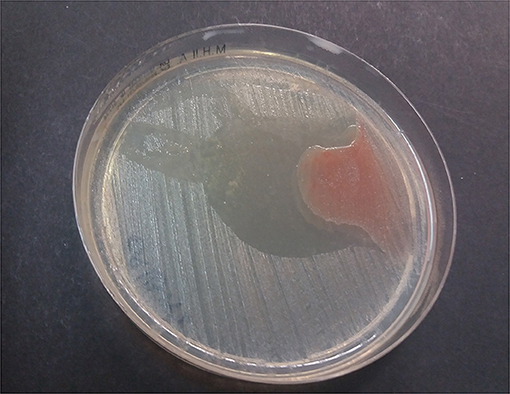
Figure 1. L-PG 500 μl effect against susceptible K. pneumoniae ssp. pneumoniae strain after 4 h at 37°C aerobically.
The MDR K. pneumoniae ssp. pneumoniae strain showed a zone of inhibition to all hemo-components, except for calcium gluconate, and P-PRP plus calcium and thrombin (Table 3, Figures 2, 3). PG showed a greater antibacterial effect, although non-significant differences were recorded between hemo-components both with and without leukocytes.
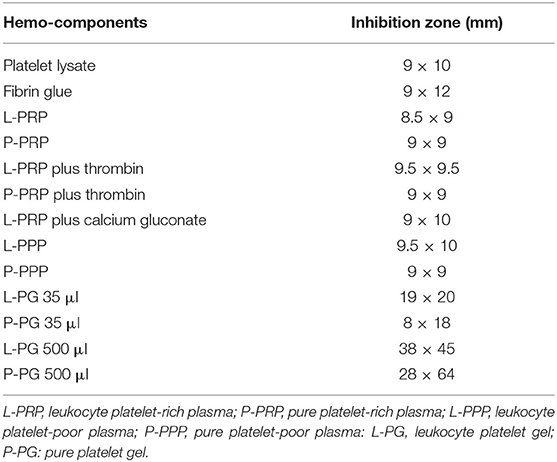
Table 3. Inhibition zones recorded by each hemo-component for multidrug-resistant K. pneumoniae ssp. pneumoniae after 4 h of incubation using the Kirby–Bauer method.
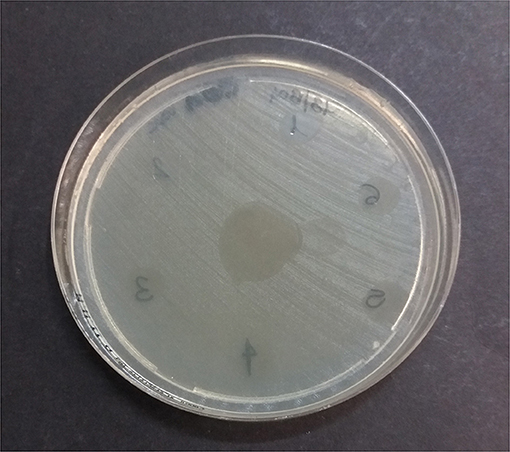
Figure 2. Multidrug-resistant K. pneumoniae ssp. pneumoniae inhibition zones by leukocyte-formulations after 4 h at 37°C aerobically. 1: platelet lysate; 2: thrombin; 3: L-PRP; 4: L-PRP plus thrombin; 5: L-PRP plus calcium gluconate; 6: L-PPP; in the center of the plate: L-PG.
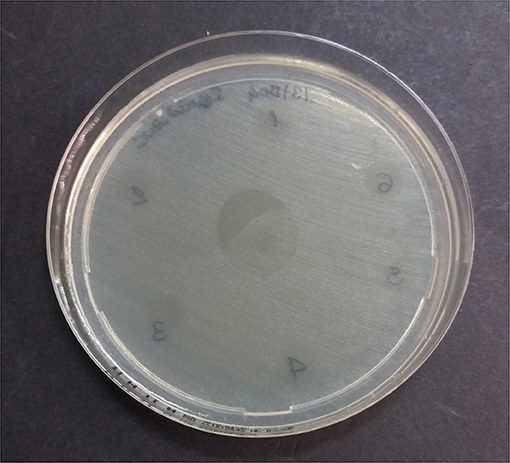
Figure 3. Multidrug-resistant K. pneumoniae ssp. pneumoniae inhibition zones by pure formulations after 4 h at 37°C aerobically. 1: fibrin glue; 2: calcium gluconate; 3: P-PRP; 4: P-PRP plus thrombin; 5: P-PRP plus calcium gluconate; 6: P-PPP; in the center of the plate: P-PG.
For extensively drug-resistant K. pneumoniae ssp. pneumoniae strains, inhibition zones of 2 × 3.5 mm, 4.5 × 37.5 mm, and 5 × 38 mm were observed for P-PG 35 μl, P-PG 500 μl, and L-PG 500 μl, respectively.
The L-PG 500 μl showed an antibacterial effect against the pan drug-resistant P. aeruginosa by recording an inhibition zone of 16.5 × 43 mm. Moreover, all the hemo-components gave areas of bacterial inhibition, except for thrombin and L-PRP with calcium gluconate against the extensively drug-resistant E. coli strain, while P-PPP and P-PG 35 μl, P-PG 500 μl, and L-PG 500 μl inhibited the growth of MDR and susceptible E. coli.
The spectrophotometer reading after 18 h confirmed what was recorded after 4 h of incubation and revealed more defined inhibition zones. In particular, compared to the respective bacterial positive control, significantly reduced areas of bacterial growth (p < 0.05) were observed for the hemo-components against Gram-negative strains (Figure 4, Table 4).
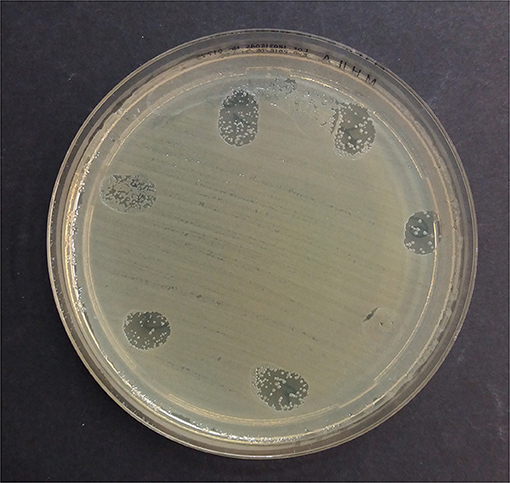
Figure 4. Leukocyte-formulations against the extensively resistant E. coli after 18 h at 37°C aerobically. 1: platelet lysate; 2: thrombin; 3: L-PRP; 4: L-PRP plus thrombin; 5: L-PRP plus calcium gluconate; 6: L-PPP; 7: L-PG.
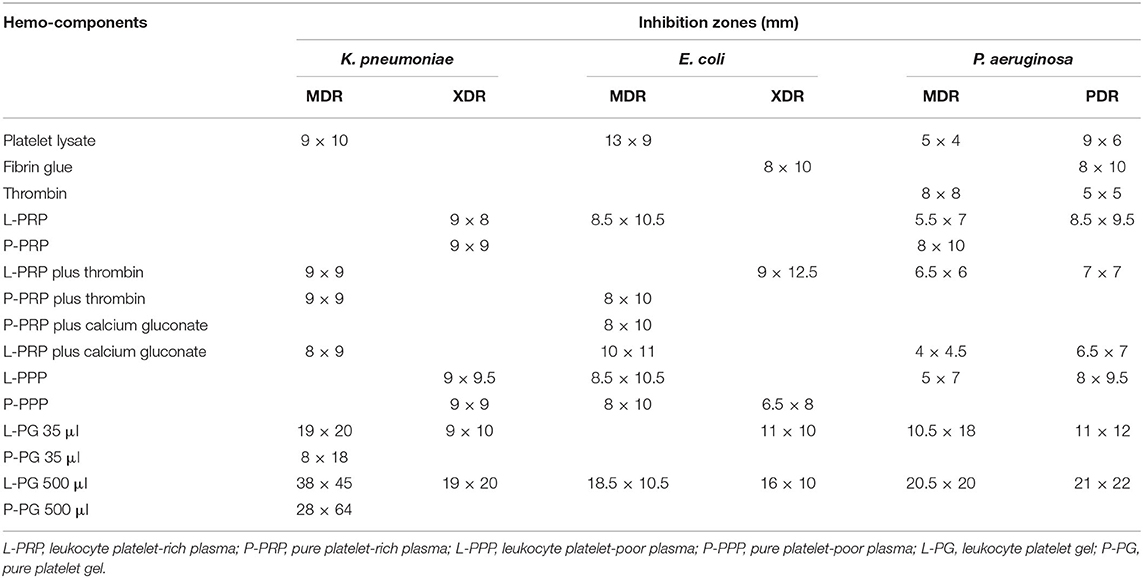
Table 4. Inhibition zones (mm) recorded for Gram-negative bacteria after 18 h of incubation using the Kirby–Bauer method.
After 18 h of incubation, L-PG 35 μl, L-PG 500 μl, and P-PG 500 μl also showed a significant antibacterial effect (p < 0.05) against Gram-positive bacteria. Inhibition zones of 4 × 10 mm, 19 × 26 mm, and 50 × 22 mm were recorded for susceptible S. aureus ssp. aureus in the presence of L-PG 35 μl, L-PG 500 μl, and P-PG 500 μl, respectively. S. cohnii ssp. cohnii susceptible to the panel of antibiotics tested resulted to be inhibited by P-PG 500 μl (4 × 17.5 mm).
At 24 h, the size of each inhibition zones was confirmed.
Antibacterial Evaluation by Broth Inhibition by Microtiter Method
Reductions in bacterial growth (mean OD540nm values) were also recorded with the microtiter method, as early as 4 h for Gram-negative microorganisms. This was confirmed by reading at 18 and 24 h. In relation to Gram affinity, different hemo-components induced a significant decrease in the growth of Gram-negative (0.897 vs. 1.604, t = 3.537 p = 0.001) and resistant bacteria (0.742 vs. 1.908, t = 4.506 p = 0.0005) compared with the corresponding bacterial growth in the absence of the hemo-component. At 18 h, thrombin significantly reduced the MDR P. aeruginosa growth (1.845 vs. 2.258, t = 41.094 p = 0.0001). The presence of leukocytes inhibited the bacterial growth more, but not statistically significant, compared with hemo-components without leukocytes. Significant differences were observed for MDR, XDR K. pneumoniae, E. coli, XDR, and PDR P. aeruginosa (Table 5).
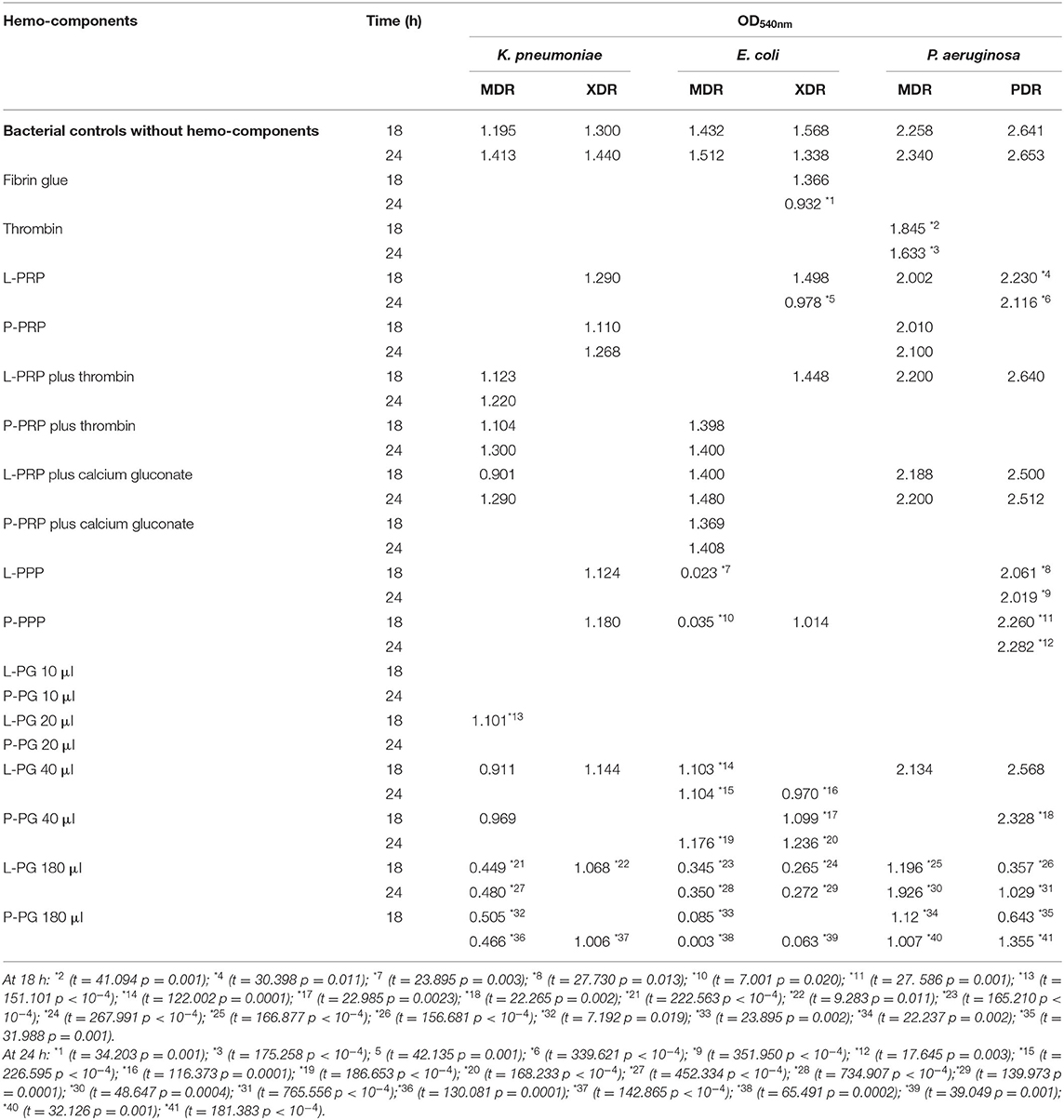
Table 5. OD540nm reductions in Gram-negative bacteria (mean OD540nm bacterium plus hemo-component vs. mean OD540nm bacterial control) recorded after 18 and 24 h of incubation by the broth microdilution method.
No significant bacterial reductions were observed when P-PRP, L-PRP, and P-PRP with thrombin, L-PRP and P-PRP with calcium gluconate, and L-PG 10 μl and P-PG 10 μl were applied for 18 and 24 h.
For Gram-positive bacteria, OD540nm reductions were observed at 18 h for all S. aureus ssp. aureus strains in the presence of FG (1.025 vs. 1.439, t = 114.969 p = 0.0001) and 24 h (1.153 vs. 1.553, t = 222.159 p < 10−4) in comparison to the positive controls.
L-PG 10 μl reduced MDR S. aureus ssp. aureus, both at 18 h (1.15 vs. 1.481, t = 160.803 p < 10−4) and at 24 h (0.665 vs. 0.9765, t = 278.611 p < 10−4). L-PG 20 μl (0.622 vs. 0.955, t = 7.8347 p = 0.0159), L-PG 180 μl (−0.018 vs. 0.955, t = 46.344 p = 0.0005), and P-PG 180 μl (−0.4055 vs. 0.955, t = 11.783 p = 0.007) were able to reduce XDR S. aureus ssp. aureus growth. Similar significant reductions were confirmed for the P-PG 180 μl at 24 h (−0.354 vs. 0.976, t = 1.2 p < 10−4).
For hemo-components such as PL, FG, and thrombin, comparison of the mean ODs recorded at 18 and 24 h for all groups of bacteria showed a non-significant increase, except for FG for resistant bacteria, for which there was a reduction, although not significant.
By the broth microdilution method, non-transfusional hemo-components with and without leukocytes showed similar bacterial properties toward all bacterial strains tested, although a greater but not significant reduction was observed for L-PG 180 μl, at both 18 h (p = 0.252) and 24 h (p = 0.306) (Figures 5–8).
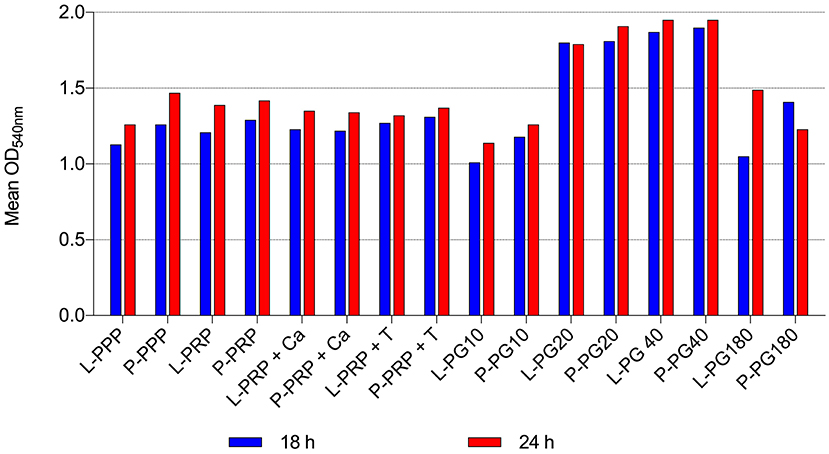
Figure 5. Mean OD540nm values of Gram-positive bacteria observed after 18 and 24 h of incubation in presence of different non-transfusional hemo-components. L-PPP, leukocyte platelet-poor plasma; P-PPP, pure platelet-poor plasma; L-PRP, leukocyte platelet-rich plasma; P-PRP, pure platelet-rich plasma; L-PRP + Ca, leukocyte platelet-rich plasma with calcium gluconate; P-PRP + Ca, pure platelet-rich plasma with calcium gluconate; L-PRP + T, leukocyte platelet-rich plasma with thrombin-rich solution; P-PRP + T, pure platelet-rich plasma with thrombin-rich solution; L-PG10, leukocyte platelet gel 10 μl; P-PG10, pure platelet gel 10 μl; L-PG20, leukocyte platelet gel 20 μl; P-PG20, pure platelet gel 20 μl; L-PG40, leukocyte platelet gel 40 μl; P-PG40, pure platelet gel 40 μl; L-PG180, leukocyte platelet gel 180 μl; P-PG180, pure platelet gel 180 μl.
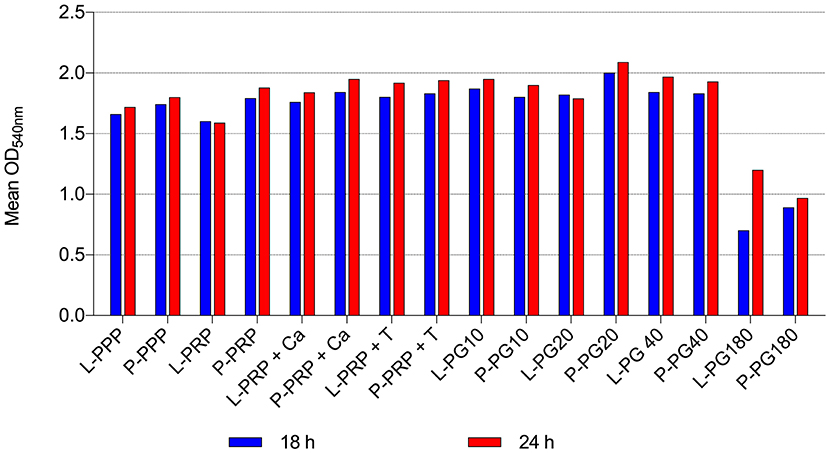
Figure 6. Mean OD540nm values of Gram-negative bacteria observed after 18 and 24 h of incubation in the presence of different non-transfusional hemo-components. L-PPP, leukocyte platelet-poor plasma; P-PPP, pure platelet-poor plasma; L-PRP, leukocyte platelet-rich plasma; P-PRP, pure platelet-rich plasma; L-PRP + Ca, leukocyte platelet-rich plasma with calcium gluconate; P-PRP + Ca, pure platelet-rich plasma with calcium gluconate; L-PRP + T, leukocyte platelet-rich plasma with thrombin-rich solution; P-PRP + T, pure platelet-rich plasma with thrombin-rich solution; L-PG10, leukocyte platelet gel 10 μl; P-PG10, pure platelet gel 10 μl; L-PG20, leukocyte platelet gel 20 μl; P-PG20, pure platelet gel 20 μl; L-PG40, leukocyte platelet gel 40 μl; P-PG40, pure platelet gel 40 μl; L-PG180, leukocyte platelet gel 180 μl; P-PG180, pure platelet gel 180 μl.
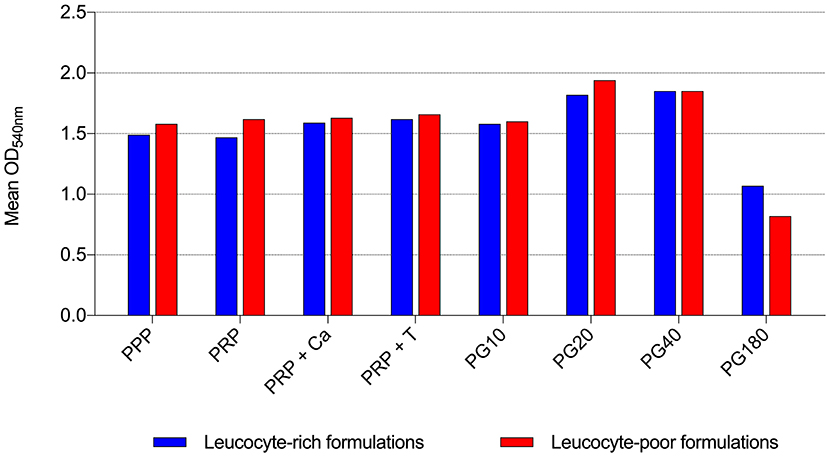
Figure 7. Mean OD540nm values of leukocyte-rich formulations and leukocyte-poor formulations observed after 18 h of incubation in the presence of different non-transfusional hemo-components. PPP, platelet-poor plasma; PRP, platelet-rich plasma; PRP + Ca, platelet-rich plasma with calcium gluconate; PRP + T, platelet-rich plasma with thrombin-rich solution; PG10, platelet gel 10 μl; PG20, platelet gel 20 μl; PG40, platelet gel 40 μl; PG180, platelet gel 180 μl.
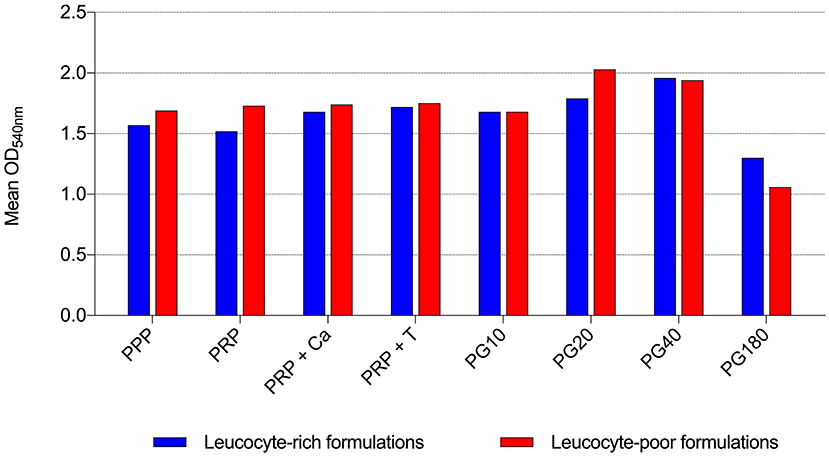
Figure 8. Mean OD540nm values of leukocyte-rich formulations and leukocyte-poor formulations observed after 24 h of incubation in the presence of different non-transfusional hemo-components. PPP, platelet-poor plasma; PRP, platelet-rich plasma; PRP + Ca, platelet-rich plasma with calcium gluconate; PRP + T, platelet-rich plasma with thrombin-rich solution; PG10, platelet gel 10 μl; PG20, platelet gel 20 μl; PG40, platelet gel 40 μl; PG180, platelet gel 180 μl.
Discussion and Conclusions
Several studies have been conducted recently, mainly in human medicine, to demonstrate the antimicrobial effect of non-transfusional hemo-components for topical use.
Few studies have been performed in vitro regarding the potential antimicrobial effect of animal hemo-components (31, 51–53). One study showed antibacterial activity of thrombin-activated platelets in horses (51). Platelet-rich plasma from rabbits had strong in vitro antimicrobial properties against methicillin-susceptible and methicillin-resistant Staphylococcus aureus, Group A Streptococcus, and Neisseria gonorrhoeae (53). Moreover, Lopez evidenced a bacteriostatic effect of equine pure platelet-rich formulations (31). In addition, antibiofilm properties of equine platelet-rich plasma lysate were also highlighted (52).
Some in vivo experimental studies using animal models have also confirmed the potential antimicrobial effect of non-transfusional hemo-components (57, 61–63, 66). Platelet-rich plasma showed antimicrobial properties in rabbit models of osteomyelitis (61, 62) and postoperative spinal implant-associated infections (63). Platelet-rich plasma improved healing of experimentally infected surgical wounds in rats (57) and accelerated healing of canine skin wounds by exerting antibacterial activity, rapid reduction of inflammation, rapid re-epithelialization, and granulation tissue formation (66).
Based on this literature evidence on animals, this study assessed the antimicrobial activity of different hemo-components for non-transfusional use obtained from canine species against bacteria with different characteristics. To the authors' knowledge, this study represents the first steps conducted in vitro to evaluate the potential inhibitory effect of canine non-transfusional hemo-components on bacteria collected from canine wounds and classified according to the sensitivity/resistance spectrum toward a known panel of human and veterinary antibiotics.
Although this study was performed on a pool of WB from a limited number of dogs, the results will potentially contribute to enrich the knowledge on this topic, in order to provide some answers to important questions recently posed by different schools of thought.
The action of the hemo-components has been evaluated against Gram-positive bacteria (S. aureus ssp. aureus, S. cohnii ssp. cohnii) and Gram-negative (P. aeruginosa, E. coli, K. pneumoniae ssp. pneumoniae) isolated from biological samples of canine origin and previously classified as susceptible, resistant, or MDR to a panel of known antibiotics.
The potential antibacterial effect of the hemo-components has been evaluated by both agar gel diffusion (Kirby–Bauer), and broth inhibition using microtiter plates with spectrophotometer readings at a wavelength of 540 nm (OD540nm). PL, FG, and thrombin-rich solution were tested for the first time in veterinary medicine.
WB from dogs with degenerative joint disease who underwent intra-articular therapy with autologous PRP was used in this study; this choice was mainly determined by ethical reasons, but it also allowed us to be more adherent to daily clinical practice. To limit the impact of individual donor variations, as performed in other studies, a pool was created with WB collected from all dogs and all materials tested were obtained from the same pool of dogs (36, 48, 52).
In this study, the cell counts contributed to define the quality of hemo-components preparation procedures, supporting the hypothesis of some authors who relate the platelet concentration to the clinical regenerative effect as it is positively correlated with the concentration of growth factors (73–75). Conversely, an excessively high platelet concentration is considered counterproductive to the healing process, as it has the potential to inhibit the angiogenic process (76). The platelet fold increase from WB and the platelet concentration obtained in the final product could be considered rough measures to define the quality of the production process and of the PRP for clinical use, respectively (77).
Specifically, the platelet concentration in L-PRP was similar to what Mazzucco et al. considered a “reasonable compromise” for determining the quality of the PCs for regenerative medicine therapeutic purposes (77, 78).
Increases in platelet and leukocyte concentrations in PRP compared with baseline concentration in WB were similar to those obtained by Perego et al. (79).
Platelet and leukocyte contents in P-PRP were comparable to those in other studies (42).
Platelet and leukocyte concentrations were much lower (45-fold and 140-fold, respectively) in L-PPP than the L-PRP formulation, similarly to Burnouf who obtained a 50-fold decrease in leukocytes and a 100-fold decrease in platelets (45).
The potential antimicrobial role of leukocytes and platelets was evaluated by comparing the bacterial load (OD) inhibition values for PPP, PRP, PRP with calcium gluconate, PRP with thrombin, and PG in various amounts, in both leukocyte-rich formulations and leukocyte-poor (or pure) formulations. PPP, although rarely used in regenerative medicine for therapeutic purposes, has been tested to define the innate antimicrobial role of plasma or humoral immune response: the result of our study suggests that the antimicrobial bacteriostatic action, especially against Gram-negative bacteria, might be related to plasma components rather than the platelets. In this study, the presence of leukocytes has been shown not to be significant for the antimicrobial activity of hemo-components, although in the presence of leukocytes a reduction in growth was observed for a greater number of microorganisms than in the action of hemo-components without leukocytes. The effect of leukocytes in PCs is the focus of heated scientific debate. Many authors have suggested that the addition of white blood cells could enhance the antibacterial potential (47, 80–82). However, Anitua and collaborators have shown that the addition of leukocytes does not significantly improve the strong antibacterial properties. It is also possible that leukocytes could increase the inflammatory response at the site because they secrete metalloproteinases, pro-inflammatory proteases, and acid hydrolases (42). Other authors agree that the presence of leukocytes does not increase the antibacterial effect of PCs (31–33, 83). In our study, the presence of leukocytes is confirmed to be non-significant for the antimicrobial activity of non-transfusional hemo-components.
By the Kirby–Bauer method, the antibacterial effect of platelet gel (PG), both with and without leukocytes, has been documented as early as 4 h for some bacteria, confirmed at 18 h, especially for slow-growing bacteria, and remained constant until 24 h. Results obtained by the agar gel diffusion method (Kirby–Bauer) were confirmed by broth inhibition using microtiter plates and reading by spectrophotometry. Both L-PG and P-PG showed a reduction in the bacterial load of Gram-negative bacteria, also PDR P. aeruginosa.
Studies using the broth inhibition method did not agree with the observed results. Edelblute et al. showed no antibacterial activity of PG against P. aeruginosa, while significant bactericidal activity was observed against S. aureus and Acinetobacter baumannii (49). Drago et al. showed a lack of action of P-PRP, against P. aeruginosa; on the contrary, growth inhibition was recorded for Gram-positive and yeasts: E. faecalis, Candida albicans, Streptococcus agalactiae, and Streptococcus oralis (30).
Zones of inhibition were observed with L-PG, at the amount of 35 μl in both MDR Gram-negative microorganisms (K. pneumoniae ssp. pneumoniae, P. aeruginosa, and E. coli) and susceptible Gram-positive bacteria. In one study, 30 μl of L-PG induced, after 3 h and up to 48 h, a significant growth inhibition effect for K. pneumoniae, P. aeruginosa, E. coli, and S. aureus (45).
The results of our study are partly consistent with those described by Bielecki et al. who observed growth inhibition of E. coli and methicillin-susceptible and methicillin-resistant S. aureus strains with L-PG 12 μl. The measured inhibition zones were comparable to those of gentamicin and oxacillin, analog of methicillin (28). In contrast to our study, the same authors did not observe a reduction for K. pneumoniae and P. aeruginosa (28).
As the amount of PG tested increased, the inhibitory effect on bacteria also increased, because of the observed reduction in bacterial load. L-PG 500 μl decreased the growth of all microorganisms, except for methicillin-susceptible S. aureus ssp. aureus strain.
The potential antibacterial effect of leukocyte-poor or pure platelet gel (P-PG) was also tested in this study. At the amount of 35 μl, P-PG already showed an area of growth inhibition exclusively for multiresistant K. pneumoniae ssp. pneumoniae, whereas at the amount of 500 μl P-PG showed growth inhibition for more types of bacteria: this effect was observed for all bacterial strains except MDR P. aeruginosa and S. conhii ssp. cohnii.
Inhibitory action was also observed for formulations in the pre-activation phase (L-PRP, PRP-P), although not against Gram-positive bacteria.
In the inhibition zones, characterized by lower growth halos, a bacteriostatic action can be assumed, as for some antibiotic drugs.
A recent systematic review also observed a tendency of PCs to inhibit the growth of microorganisms during the first hours of incubation, whereas they did not seem to be able to completely break down the microbial load, indicating a bacteriostatic rather than a bactericidal activity (56).
On this aspect, in the presence of thrombin-activated PRP, Wu et al. observed a reduction in the number of E. coli, P. aeruginosa, and K. pneumoniae during the first 8 to 12 h, with the greatest reduction observed at 0 to 4 h. The number of bacteria increased again after the 4-h time point because the bacterial killing process was not complete; after this time point, bacterial growth exceeded the killing rate and growth continued until the stationary phase was reached at the 24-h time point (47).
It is interesting to note that PL, FG, and thrombin had an inhibitory action only against Gram-negative bacteria. This finding could open up innovative therapeutic possibilities, potentially exploiting combined therapies in a clinical scenario with infections sustained by these types of bacteria, sometimes rather aggressive and difficult to treat. Specifically, thrombin at the amount of 8 μl was able to create a visible zone of reduced growth for the two P. aeruginosa strains (MDR and PDR). The only strain of S. aureus ssp. aureus (susceptible to the panel of antibiotics) whose bacterial load was significantly decreased by FG was also moderately inhibited by PL and thrombin. Although studies on these hemo-components are very few in the literature, our findings are in contrast to Bielecki and collaborators who observed no inhibitory effect using 12 μl of thrombin against S. aureus, E. coli, K. pneumoniae, P. aeruginosa, and E. faecalis (28).
Other studies have shown that activation of platelets in PRP with thrombin leads to a significant increase in inhibitory activity on bacteria (51). In the same study, addition of thrombin to E. coli was shown not to affect bacterial growth, and addition of thrombin to PPP did not increase the inhibitory effect (51).
Although further studies will need to be done to clarify the mechanism of action, the thrombin-rich solution used in our study may have had antibacterial action by itself, because it is derived from autologous plasma, and it is not thrombin derived from complex chemical extraction.
Some studies have tested the potential link between the antibacterial effect and the platelet activation process with CaCl2, reaching discordant results (45, 48). According to Burnouf et al., activation of coagulation to prepare PG may reduce antimicrobial activity by consuming complement or other inhibitors or by releasing components that support bacterial proliferation (45). Contrariwise, Drago et al. observed that only activated materials were able to inhibit bacterial growth, suggesting that the activation of coagulation is a key step (48).
The antibacterial action against Gram-negative bacteria, observed for L-PPP, P-PPP, L-PRP, and P-PRP and the same with the addition of calcium gluconate and thrombin, is consistent with that documented by Burnouf et al. Using PPP, PRP, and PL, these authors obtained inhibitory action against E. coli, P. aeruginosa, and K. pneumoniae, but not against Gram-positive microorganisms, except for S. aureus (45). The zones of inhibition observed in our study showed a minimum area of 4 × 4.5 mm in contrast to the zones of inhibition of 0.5, 0.5–1, and >1 mm considered by Burnouf et al. (45). Tohidnezhad et al. also observed antibacterial action of PRP with thrombin for E. coli and P. aeruginosa, Enterococcus faecalis, Bacillus megaterium, and Proteus mirabilis (44). Li et al. found that PRP has strong antimicrobial properties in vitro against bacteria such as methicillin-susceptible and methicillin-resistant Staphylococcus aureus, Group A Streptococcus, and Neisseria gonorrhoeae (53).
The bacteriostatic action of the PPP, with and without leukocytes, was shown only for Gram-negative bacteria (E. coli, P. aeruginosa, and K. pneumoniae ssp. pneumoniae) belonging to the MDR and PDR groups. This finding suggests that the antimicrobial bacteriostatic action might be related to plasma components rather than platelets.
The mechanism of the antibacterial effect of PCs is not yet fully understood.
There are several studies suggesting that platelets play a very important role in the innate defense against the induction and progression of endovascular infections. This host defense capacity depends on the ability of platelets to release a group of antimicrobial peptides, known collectively as platelet protein microbicide, at the site of damage or endovascular infection. Indeed, platelets generate oxygen metabolites, including peroxide, hydrogen peroxide, and free radicals; are able to bind and aggregate microorganisms, improving the clearance of pathogens from the bloodstream; have chemotactic action on macrophages; participate in antibody-dependent cellular cytotoxicity functions against pathogens; and, finally, would release a series of potent antimicrobial peptides (84, 85).
In one study, PPP showed no impact on the growth parameters of any of the bacteria tested, whereas PRP showed an antibacterial effect with a strong correlation between platelet concentration and antibacterial activity (38). Mariani et al., instead, showed that both PRP and PPP inhibited bacterial growth for up to 2 h of incubation, but the effect of P-PRP was significantly higher than that of PPP (32).
One study proposed a possible molecular mechanism to explain the antimicrobial effect of PRP at least against E. coli and P. mirabilis through the detection of an antimicrobial peptide in PRP, the human beta-defensin 2 (hBD-2) (44). Another study showed that PCs induced a significant increase in hBD-2 expression in primary keratinocytes cell culture in a concentration- and time-dependent manner (86).
Several antibacterial peptides have also been identified in human platelets, including connective tissue-activating peptide 3 (CTAP-3), platelet factor 4 (PF-4), regulated upon activation–normal T-cell expressed and secreted protein (RANTES), thymosin β-4 (Tβ-4), platelet basic protein (PBP), fibrinopeptide A (FP-A), and fibrinopeptide B (FP-B) (87–89).
Drago et al. also tested P-PPP against Gram-positive bacteria isolated from the oral cavity obtaining similar, although slightly lower, results to P-PRP, whereas platelets alone showed no antibacterial activity (48). The authors concluded that the antimicrobial activity of PCs against E. faecalis, S. agalactiae, S. oralis, and S. aureus is supported by a synergy of plasma components and platelet-derived factors (48).
In our study, in relation to the different groups of microorganisms, significant reductions were observed only for Gram-negative MDR bacteria in the presence of PG 180 μl, both with and without leukocytes. For P-PG 180 μl, the inhibitory action was also documented for Gram-negative PDR bacteria.
The results of this study are certainly very encouraging and stimulate further studies to understand the mechanism of antibacterial effect. A greater knowledge of the mechanism of action could bring considerable advantages for potential practical applications, being able to better outline the indications, the limits, and the conditions of practical use, also for the antibacterial effect in addition to the consolidated regenerative effect of the non-transfusional hemo-components.
To justify the antibacterial effect of hemo-components, future research can be ideally oriented to the investigation of potential plasma factors, ideally starting from the peptides already identified for the human species but considering that there could be differences between the human and canine species, as evidenced by this study regarding the antibacterial effect.
Our study confirmed the hypothesized antibacterial properties of canine non-transfusional hemo-components. The bacteriostatic effect appeared to be higher against Gram-negative bacteria. The presence of neither leukocytes nor platelets seems to be essential for the antibacterial effect. Although the interaction of hemo-components with microbial pathogens needs further investigations because the exact mechanism responsible for the antibacterial activity is not yet fully known, non-transfusional hemo-components have been shown to represent a useful natural substance for infection control, especially at surgical sites in the immediate postoperative period. The emergence of multidrug-, extensively drug-, and pan drug-resistant bacteria poses a significant health and economic threat for animals and humans. Therefore, local application of non-transfusional hemo-components could support the action of molecules with antibiotic activity and represent a suitable alternative to control MDR pathogens.
Data Availability Statement
The raw data supporting the conclusions of this article will be made available by the authors, without undue reservation.
Ethics Statement
The animal study was reviewed and approved by Animal Welfare Body of the University of Camerino. Written informed consent was obtained from the owners for the participation of their animals in this study.
Author Contributions
A-RA and AT contributed to the conception and design of the study. CI organized the database. ES and AT recruited the animals and prepared the hemo-components. A-RA, CI, AC, and ML performed the bacteriological evaluations. A-RA performed the statistical analysis. A-RA, CI, and AT wrote the first draft of the manuscript. AC, CR, GM, GR, LG, and VC wrote sections of the manuscript. All authors contributed to manuscript revision, read, and approved the submitted version.
Funding
This work was supported by a research grant from the University of Camerino, Italy (Fondo di Ateneo per la Ricerca 2014.2015).
Conflict of Interest
The authors declare that the research was conducted in the absence of any commercial or financial relationships that could be construed as a potential conflict of interest.
Publisher's Note
All claims expressed in this article are solely those of the authors and do not necessarily represent those of their affiliated organizations, or those of the publisher, the editors and the reviewers. Any product that may be evaluated in this article, or claim that may be made by its manufacturer, is not guaranteed or endorsed by the publisher.
References
1. World Health Organization (WHO). GLASS Whole-Genome Sequencing for Surveillance of Antimicrobial Resistance: Global Antimicrobial Resistance and Use Surveillance System (GLASS). Geneva: World Health Organization (2020). Available online at: https://www.who.int/health-topics/antimicrobial-resistance.
2. Cloeckaert A, van Duijkeren E, Schwarz S. Antimicrobial Resistance in Animals and the Environment – ARAE 2015. Vet Microbiol. (2016) 194:1–4. doi: 10.1016/j.vetmic.2016.09.001
3. Görmeli G, Görmeli CA, Ataoglu B, Çolak C, Aslantürk O, Ertem K. Multiple PRP injections are more effective than single injections and hyaluronic acid in knees with early osteoarthritis: a randomized, double-blind, placebo-controlled trial. Knee Surg, Sport Traumatol Arthrosc. (2017) 25:958–65. doi: 10.1007/s00167-015-3705-6
4. Samy AM. The role of platelet rich plasma in management of fracture neck femur: new insights. Int Orthop. (2016) 40:1019–24. doi: 10.1007/s00264-015-2844-1
5. Martini LI, Via AG, Fossati C, Randelli F, Randelli P, Cucchi D. Single platelet-rich plasma injection for early stage of Osteoarthritis of the knee. Joints. (2017) 5:2–6. doi: 10.1055/s-0037-1601405
6. Alessio-Mazzola M, Repetto I, Biti B, Trentini R, Formica M, Felli L. Autologous US-guided PRP injection versus us-guided focal extracorporeal shock wave therapy for chronic lateral epicondylitis: a minimum of 2-year follow-up retrospective comparative study. J Orthop Surg. (2018) 26:1–8. doi: 10.1177/2309499017749986
7. Huang Y, Liu X, Xu X, Liu J. Intra-articular injections of platelet-rich plasma, hyaluronic acid or corticosteroids for knee osteoarthritis: a prospective randomized controlled study. Orthopade. (2019) 48:239–47. doi: 10.1007/s00132-018-03659-5
8. Yuksel EP, Sahin G, Aydin F, Senturk N, Turanli AY. Evaluation of effects of platelet-rich plasma on human facial skin. J Cosmet Laser Ther. (2014) 16:206–8. doi: 10.3109/14764172.2014.949274
9. Picard F, Hersant B, Bosc R, Meningaud JP. Should we use platelet-rich plasma as an adjunct therapy to treat “acute wounds,” “burns,” and “laser therapies”: a review and a proposal of a quality criteria checklist for further studies. Wound Repair Regen. (2015) 23:163–70. doi: 10.1111/wrr.12266
10. Martinez-Zapata MJ, Martí-Carvajal AJ, Solà I, Expósito JA, Bolíbar I, Rodríguez L, et al. Autologous platelet-rich plasma for treating chronic wounds. Cochrane Database Syst Rev. (2016) 5:CD006899. doi: 10.1002/14651858.CD006899.pub3
11. Moneib HA, Youssef SS, Aly DG, Rizk MA, Abdelhakeem YI. Autologous platelet-rich plasma versus conventional therapy for the treatment of chronic venous leg ulcers: a comparative study. J Cosmet Dermatol. (2018) 17:495–501. doi: 10.1111/jocd.12401
12. Burgos-Alonso N, Lobato I, Hernández I, Sebastian KS, Rodríguez B, March AG, et al. Autologous platelet-rich plasma in the treatment of venous leg ulcers in primary care: a randomised controlled, pilot study. J Wound Care. (2018) 27:S20–4. doi: 10.12968/jowc.2018.27.Sup6.S20
13. Ronci C, Ferraro AS, Lanti A, Missiroli F, Sinopoli S, Del Proposto G, et al. Platelet-rich plasma as treatment for persistent ocular epithelial defects. Transfus Apher Sci. (2015) 52:300–4. doi: 10.1016/j.transci.2014.12.027
14. Alio JL, Rodriguez AE, De Arriba P, Gisbert S, Abdelghany AA. Treatment with platelet-rich plasma of surgically related dormant corneal ulcers. Eur J Ophthalmol. (2018) 28:515–20. doi: 10.1177/1120672117747042
15. Wróbel-Dudzińska D, Alio J, Rodriguez A, Suchodoła-Ratajewicz E, Kosior-Jarecka E, Rymgayłło-Jankowska B, et al. Clinical efficacy of platelet-rich plasma in the treatment of neurotrophic corneal ulcer. J Ophthalmol. (2018) 2018:3538764. doi: 10.1155/2018/3538764
16. Tabrizi R, Karagah T, Shahidi S, Zare N. Does platelet-rich plasma enhance healing in the idiopathic bone cavity? A single-blind randomized clinical trial. Int J Oral Maxillofac Surg. (2015) 44:1175–80. doi: 10.1016/j.ijom.2015.05.011
17. Del Fabbro M, Bucchi C, Lolato A, Corbella S, Testori T, Taschieri S. Healing of Postextraction Sockets Preserved With Autologous Platelet Concentrates. A systematic review and meta-analysis. J Oral Maxillofac Surg. (2017) 75:1601–15. doi: 10.1016/j.joms.2017.02.009
18. Bhujbal R, Malik NA, Kumar N, Kv S, Parkar MI, Mb J. Comparative evaluation of platelet rich plasma in socket healing and bone regeneration after surgical removal of impacted mandibular third molars. J Dent Res Dent Clin Dent Prospects. (2018) 12:153–8. doi: 10.15171/joddd.2018.024
19. Del Fabbro M, Karanxha L, Panda S, Bucchi C, Nadathur Doraiswamy J, Sankari M, et al. Autologous platelet concentrates for treating periodontal infrabony defects. Cochrane Database Syst Rev. (2018) 11:CD011423. doi: 10.1002/14651858.CD011423.pub2
20. Saleem M, Pisani F, Zahid FM, Georgakopoulos I, Pustina-Krasniqi T, Xhajanka E, et al. Adjunctive platelet-rich plasma (PRP) in infrabony regenerative treatment: a systematic review and RCT's meta-analysis. Stem Cells Int. (2018) 2018:9594235. doi: 10.1155/2018/9594235
21. Fahie MA, Ortolano GA, Guercio V, Schaffer JA, Johnston G, Au J, et al. randomized controlled trial of the efficacy of autologous platelet therapy for the treatment of osteoarthritis in dogs. J Am Vet Med Assoc. (2013) 243:1291–7. doi: 10.2460/javma.243.9.1291
22. Tambella AM, Attili AR, Dini F, Palumbo Piccionello A, Vullo C, Serri E, et al. Autologous platelet gel to treat chronic decubital ulcers: a randomized, blind controlled clinical trial in dogs. Vet Surg. (2014) 43:726–33. doi: 10.1111/j.1532-950X.2014.12148.x
23. Iacopetti I, Patruno M, Melotti L, Martinello T, Bedin S, Badon T, et al. Autologous platelet-rich plasma enhances the healing of large cutaneous wounds in dogs. Front Vet Sci. (2020) 7:575449. doi: 10.3389/fvets.2020.575449
24. Perego R, Spada E, Baggiani L, Martino PA, Proverbio D. Efficacy of a semi automated commercial closed system for autologous leukocyte- and platelet-rich plasma (l-prp) production in dogs: a preliminary study. Animals. (2020) 10:1342. doi: 10.3390/ani10081342
25. Perinelli DR, Bonacucina G, Pucciarelli S, Cespi M, Serri E, Polzonetti V, et al. Rheological properties and growth factors content of Platelet-Rich plasma: relevance in veterinary biomedical treatments. Biomedicines. (2020) 8:429. doi: 10.3390/biomedicines8100429
26. Tambella AM, Bartocetti F, Rossi G, Galosi L, Catone G, Falcone A, et al. Effects of autologous platelet-rich fibrin in post-extraction alveolar sockets: a randomized, controlled split-mouth trial in dogs with spontaneous periodontal disease. Animals. (2020) 10:1343. doi: 10.3390/ani10081343
27. Marx RE. Platelet-rich plasma: evidence to support its use. J Oral Maxillofac Surg. (2004) 62:489–96. doi: 10.1016/j.joms.2003.12.003
28. Bielecki TM, Gazdzik TS, Arendt J, Szczepanski T, Król W, Wielkoszynski T. Antibacterial effect of autologous platelet gel enriched with growth factors and other active substances: an in vitro study. J Bone Jt Surg - Ser B. (2007) 89:417–20. doi: 10.1302/0301-620X.89B3.18491
29. Moojen DJF, Everts PAM, Schure RM, Overdevest EP, Van Zundert A, Knape JTA, et al. Antimicrobial activity of platelet-leukocyte gel against staphylococcus aureus. J Orthop Res. (2008) 26:404–10. doi: 10.1002/jor.20519
30. Drago L, Bortolin M, Vassena C, Taschieri S, Del Fabbro M. Antimicrobial activity of pure platelet-rich plasma against microorganisms isolated from oral cavity. BMC Microbiol. (2013) 13:47. doi: 10.1186/1471-2180-13-47
31. López C, Carmona JU, Giraldo CE, Álvarez ME. Bacteriostatic effect of equine pure platelet-rich plasma and other blood products against methicillin-sensitive Staphylococcus aureus: an in vitro study. Vet Comp Orthop Traumatol. (2014) 27:372–8. doi: 10.3415/VCOT-14-04-0054
32. Mariani E, Filardo G, Canella V, Berlingeri A, Bielli A, Cattini L, et al. Platelet-rich plasma affects bacterial growth in vitro. Cytotherapy. (2014) 16:1294–304. doi: 10.1016/j.jcyt.2014.06.003
33. Mariani E, Canella V, Berlingeri A, Bielli A, Cattini L, Landini MP, et al. Leukocyte presence does not increase microbicidal activity of Platelet-rich Plasma in vitro clinical microbiology and vaccines. BMC Microbiol. (2015) 15:149. doi: 10.1186/s12866-015-0482-9
34. Yang L-C, Hu S-W, Yan M, Yang J-J, Tsou S-H, Lin Y-Y. Antimicrobial activity of platelet-rich plasma and other plasma preparations against periodontal pathogens. J Periodontol. (2015) 86:310–8. doi: 10.1902/jop.2014.140373
35. Badade PS, Mahale SA, Panjwani AA, Vaidya PD, Warang AD. Antimicrobial effect of platelet-rich plasma and platelet-rich fibrin. Indian J Dent Res. (2016) 27:300–4. doi: 10.4103/0970-9290.186231
36. Aggour RL, Gamil L. Antimicrobial effects of platelet-rich plasma against selected oral and periodontal pathogens. Polish J Microbiol. (2017) 66:31–7. doi: 10.5604/17331331.1235227
37. Karde P, Sethi K, Mahale S, Khedkar S, Patil A, Joshi C. Comparative evaluation of platelet count and antimicrobial efficacy of injectable platelet-rich fibrin with other platelet concentrates: an in vitro study. J Indian Soc Periodontol. (2017) 21:97. doi: 10.4103/jisp.jisp_201_17
38. Maghsoudi O, Ranjbar R, Mirjalili SH, Fasihi-Ramandi M. Inhibitory activities of platelet-rich and platelet-poor plasma on the growth of pathogenic bacteria. Iran J Pathol. (2017) 12:79–87. doi: 10.30699/ijp.2017.23386
39. Cieślik-Bielecka A, Bold T, Ziółkowski G, Pierchała M, Królikowska A, Reichert P. Antibacterial activity of leukocyte- and platelet-rich plasma: an in vitro study. Biomed Res Int. (2018) 2018:9471723. doi: 10.1155/2018/9471723
40. Kour P, Pudakalkatti P, Vas A, Das S, Padmanabhan S. Comparative evaluation of antimicrobial efficacy of platelet-rich plasma, platelet-rich fibrin, and injectable platelet-rich fibrin on the standard strains of Porphyromonas gingivalis and Aggregatibacter actinomycetemcomitans. Contemp Clin Dent. (2018) 9:325. doi: 10.4103/ccd.ccd_367_18
41. Çetinkaya RA, Yenilmez E, Petrone P, Yilmaz S, Bektöre B, Simsek B, et al. Platelet-rich plasma as an additional therapeutic option for infected wounds with multi-drug resistant bacteria: in vitro antibacterial activity study. Eur J Trauma Emerg Surg. (2019) 45:555–65. doi: 10.1007/s00068-018-0957-0
42. Anitua E, Alonso R, Girbau C, Aguirre JJ, Muruzabal F, Orive G. Antibacterial effect of plasma rich in growth factors (PRGF®- Endoret®) against Staphylococcus aureus and Staphylococcus epidermidis strains. Clin Exp Dermatol. (2012) 37:652–7. doi: 10.1111/j.1365-2230.2011.04303.x
43. Pham TAV, Tran TTP, Luong NTM. Antimicrobial effect of platelet-rich plasma against porphyromonas gingivalis. Int J Dent. (2019) 2019:7329103. doi: 10.1155/2019/7329103
44. Tohidnezhad M, Varoga D, Wruck CJ, Podschun R, Sachweh BH, Bornemann J, et al. Platelets display potent antimicrobial activity and release human beta-defensin 2. Platelets. (2012) 23:217–23. doi: 10.3109/09537104.2011.610908
45. Burnouf T, Chou ML, Wu YW, Su CY, Lee LW. Antimicrobial activity of platelet (PLT)-poor plasma, PLT-rich plasma, PLT gel, and solvent/detergent-treated PLT lysate biomaterials against wound bacteria. Transfusion. (2013) 53:138–46. doi: 10.1111/j.1537-2995.2012.03668.x
46. Rózalski M, Micota B, Sadowska B, Paszkiewicz M, Wieckowska-Szakiel M, Rózalska B. Antimicrobial/anti-biofilm activity of expired blood platelets and their released products. Postepy Hig Med Dosw. (2013) 67:321–5. doi: 10.5604/17322693.1046009
47. Wu X, Ren J, Yuan Y, Luan J, Yao G, Li J. Antimicrobial properties of single-donor-derived, platelet-leukocyte fibrin for fistula occlusion: an in vitro study. Platelets. (2013) 24:632–6. doi: 10.3109/09537104.2012.761685
48. Drago L, Bortolin M, Vassena C, Romanò CL, Taschieri S, Del Fabbro M. Plasma components and platelet activation are essential for the antimicrobial properties of autologous platelet-rich plasma: an in vitro study. PLoS ONE. (2014) 9:e107813. doi: 10.1371/journal.pone.0107813
49. Edelblute CM, Donate AL, Hargrave BY, Heller LC. Human platelet gel supernatant inactivates opportunistic wound pathogens on skin. Platelets. (2015) 26:13–6. doi: 10.3109/09537104.2013.863859
50. Intravia J, Allen DA, Durant TJS, McCarthy MBR, Russell R, Beitzel K, et al. In vitro evaluation of the anti-bacterial effect of two preparations of platelet rich plasma compared with Cefazolin and whole blood. Muscles Ligaments Tendons J. (2014) 4:79–84. doi: 10.11138/mltj/2014.4.1.079
51. Aktan Í, Dunkel B, Cunningham FM. Equine platelets inhibit E coli growth and can be activated by bacterial lipopolysaccharide and lipoteichoic acid although superoxide anion production does not occur and platelet activation is not associated with enhanced production by neutrophils. Vet Immunol Immunopathol. (2013) 152:209–17. doi: 10.1016/j.vetimm.2012.12.007
52. Gilbertie JM, Schaer TP, Schubert AG, Jacob ME, Menegatti S, Ashton Lavoie R, et al. Platelet-rich plasma lysate displays antibiofilm properties and restores antimicrobial activity against synovial fluid biofilms in vitro. J Orthop Res. (2020) 38:1365–74. doi: 10.1002/jor.24584
53. Li H, Li B. PRP as a new approach to prevent infection: preparation and in vitro antimicrobial properties of PRP. J Vis Exp. (2013) 74:e50351. doi: 10.3791/50351
54. Del Fabbro M, Bortolin M, Taschieri S, Ceci C, Weinstein RL. Antimicrobial properties of platelet-rich preparations. A systematic review of the current pre-clinical evidence. Platelets. (2016) 27:276–85. doi: 10.3109/09537104.2015.1116686
55. D'asta F, Halstead F, Harrison P, Zecchi Orlandini S, Moiemen N, Lord J. The contribution of leucocytes to the antimicrobial activity of platelet-rich plasma preparations: a systematic review. Platelets. (2018) 29:9–20. doi: 10.1080/09537104.2017.1317731
56. Varshney S, Dwivedi A, Pandey V. Antimicrobial effects of various platelet rich concentrates-vibes from in-vitro studies-a systematic review. J Oral Biol Craniofacial Res. (2019) 9:299–305. doi: 10.1016/j.jobcr.2019.06.013
57. Cetinkaya RA, Yilmaz S, Ünlü A, Petrone P, Marini C, Karabulut E, et al. The efficacy of platelet-rich plasma gel in MRSA-related surgical wound infection treatment: an experimental study in an animal model. Eur J Trauma Emerg Surg. (2018) 44:859–67. doi: 10.1007/s00068-017-0852-0
58. Serraino GF, Dominijanni A, Jiritano F, Rossi M, Cuda A, Caroleo S, et al. Platelet-rich plasma inside the sternotomy wound reduces the incidence of sternal wound infections. Int Wound J. (2013) 12:260–4. doi: 10.1111/iwj.12087
59. Hamman BL, Stout LY, Theologes TT, Sass DM, Da Graca B, Filardo G. Relation between topical application of platelet-rich plasma and vancomycin and severe deep sternal wound infections after a first median sternotomy. Am J Cardiol. (2014) 113:1415–9. doi: 10.1016/j.amjcard.2013.12.046
60. Yuan T, Zhang C, Zeng B. Treatment of chronic femoral osteomyelitis with platelet-rich plasma (PRP): a case report. Transfus Apher Sci. (2008) 38:167–73. doi: 10.1016/j.transci.2008.01.006
61. Jia WT, Zhang CQ, Wang JQ, Feng Y, Ai ZS. The prophylactic effects of platelet-leucocyte gel in osteomyelitis: an experimental study in a rabbit model. J Bone Jt Surg - Ser B. (2010) 92:304–10. doi: 10.1302/0301-620X.92B2.22042
62. Li GY, Yin JM, Ding H, Jia WT, Zhang CQ. Efficacy of leukocyte- and platelet-rich plasma gel (L-PRP gel) in treating osteomyelitis in a rabbit model. J Orthop Res. (2013) 31:949–56. doi: 10.1002/jor.22299
63. Li H, Hamza T, Tidwell JE, Clovis N, Li B. Unique antimicrobial effects of platelet-rich plasma and its efficacy as a prophylaxis to prevent implant-associated spinal infection. Adv Healthc Mater. (2013) 2:1277–84. doi: 10.1002/adhm.201200465
64. Mirzaei M, Daneshpajooh A, Farsinezhad A, Jafarian Z, Ebadzadeh MR, Saberi N, et al. The therapeutic effect of intravesical instillation of platelet rich plasma on recurrent bacterial cystitis in women: a randomized clinical trial. Urol J. (2019) 16:609–13. doi: 10.22037/uj.v0i0.5239
65. Sangiovanni TP, Kiebzak GM. Prospective randomized evaluation of intraoperative application of autologous platelet-rich plasma on surgical site infection or delayed wound healing. Foot Ankle Int. (2016) 37:470–7. doi: 10.1177/1071100715623994
66. Farghali HA, AbdElKader NA, AbuBakr HO, Aljuaydi SH, Khattab MS, Elhelw R, et al. Antimicrobial action of autologous platelet-rich plasma on MRSA-infected skin wounds in dogs. Sci Rep. (2019) 9:12722. doi: 10.1038/s41598-019-48657-5
67. Tarantino F, Flores A, Gobbi A, Salomé D. Un nuovo semplice metodo per l'ottenimento della colla di fibrina autologa ad uso chirurgico. La Trasfus del sangue. (2001) 46:37–46.
68. Soffer E, Ouhayoun JP, Dosquet C, Meunier A, Anagnostou F. Effects of platelet lysates on select bone cell functions. Clin Oral Implants Res. (2004) 15:581–8. doi: 10.1111/j.1600-0501.2004.01063.x
69. Eberl C, Speth C, Jacobsen ID, Hermann M, Hagleitner M, Deshmukh H, et al. Candida: Platelet interaction and platelet activity in vitro. J Innate Immun. (2018) 11:52–62. doi: 10.1159/000491030
70. Magiorakos AP, Srinivasan A, Carey RB, Carmeli Y, Falagas ME, Giske CG, et al. Multidrug-resistant, extensively drug-resistant and pandrug-resistant bacteria: An international expert proposal for interim standard definitions for acquired resistance. Clin Microbiol Infect. (2012) 18:268–81. doi: 10.1111/j.1469-0691.2011.03570.x
71. Clinical and Laboratory Standards Institute (CLSI). Performance Standards for Antimicrobial Disk and Dilution Susceptibility Tests for Bacteria Isolated From Animals. In: 4th ed., ed. CLSI supplement VET08. Wayne, PA: Clinical and Laboratory Standards Institute (2018).
72. The European Committee on Antimicrobial Susceptibility Testing (EUCAST). Breakpoint tables for interpretation of MICs and zone diameters. (2018) Version 8.1. Available online at: http://www.eucast.org/
73. Leitner GC, Gruber R, Neumüller J, Wagner A, Kloimstein P, Höcker P, et al. Platelet content and growth factor release in platelet-rich plasma: a comparison of four different systems. Vox Sang. (2006) 91:135–9. doi: 10.1111/j.1423-0410.2006.00815.x
74. Rughetti A, Gallo R, Caloprisco G, Borean A, Necozione S, Dell'Orso L, et al. Platelet gel: assays of three growth factors. Blood Transfus. (2006) 4:92–101.
75. McCarrel T, Fortier L. Temporal growth factor release from platelet-rich plasma, trehalose lyophilized platelets, and bone marrow aspirate and their effect on tendon and ligament gene expression. J Orthop Res. (2009) 27:1033–42. doi: 10.1002/jor.20853
76. Giusti I, Rughetti A, D'Ascenzo S, Millimaggi D, Pavan A. Dell'Orso L, Dolo V. Identification of an optimal concentration of platelet gel for promoting angiogenesis in human endothelial cells. Transfusion. (2009) 49:771–8. doi: 10.1111/j.1537-2995.2008.02033.x
77. Tambella AM, Attili AR, Dupré G, Cantalamessa A, Martin S, Cuteri V, et al. Platelet-rich plasma to treat experimentally-induced skin wounds in animals: a systematic review and meta-analysis. PLoS ONE. (2018) 13:e0191093. doi: 10.1371/journal.pone.0191093
78. Mazzucco L, Balbo V, Guaschino R. “Reasonable compromise” to define the quality standards of platelet concentrate for non-transfusion use (CPunT). Transfus Apher Sci. (2012) 47:207–11. doi: 10.1016/j.transci.2012.06.006
79. Perego R, Proverbio D, Baggiani L, Nora Roggero BDGGSE. In house double centrifugation method for preparation of homologous platelet-rich plasma (Prp) in dogs EC. Vet Sci. (2016) 2:126–32.
80. Bielecki TM, Dohan Ehrenfest D, A Everts P, Wiczkowski A. The role of leukocytes from L-PRP/L-PRF in wound healing and immune defense: new perspectives. Curr Pharm Biotechnol. (2012) 13:1153–62. doi: 10.2174/138920112800624373
81. Cieślik-Bielecka A, Reichert P, Skowroński R, Królikowska A, Bielecki T. A new aspect of in vitro antimicrobial leukocyte- and platelet-rich plasma activity based on flow cytometry assessment. Platelets. (2019) 30:728–36. doi: 10.1080/09537104.2018.1513472
82. Prysak MH, Lutz CG, Zukofsky TA, Katz JM, Everts PA, Lutz GE. Optimizing the safety of intradiscal platelet-rich plasma: an in vitro study with Cutibacterium acnes. Regen Med. (2019) 14:955–67. doi: 10.2217/rme-2019-0098
83. Giusti I, Di Francesco M, D'Ascenzo S, Palumbo P, Rughetti A, Dell'Orso L, et al. Leukocyte depletion does not affect the in vitro healing ability of platelet rich plasma. Exp Ther Med. (2018) 15:4029–38. doi: 10.3892/etm.2018.5887
84. Yeaman MR. The role of platelets in antimicrobial host defense. Clin Infect Dis. (1997) 25:951–68. doi: 10.1086/516120
85. Klinger MHF, Jelkmann W. Role of blood platelets in infection and inflammation. J Interf Cytokine Res. (2002) 22:913–22. doi: 10.1089/10799900260286623
86. Bayer A, Lammel J, Rademacher F, Groß J, Siggelkow M, Lippross S, et al. Platelet-released growth factors induce the antimicrobial peptide human beta-defensin-2 in primary keratinocytes. Exp Dermatol. (2016) 25:460–5. doi: 10.1111/exd.12966
87. Tang YQ, Yeaman MR, Selsted ME. Antimicrobial peptides from human platelets. Infect Immun. (2002) 70:6524–33. doi: 10.1128/IAI.70.12.6524-6533.2002
88. Mussano F, Genova T, Munaron L, Petrillo S, Erovigni F, Carossa S. Cytokine, chemokine, and growth factor profile of platelet-rich plasma. Platelets. (2016) 27:467–71. doi: 10.3109/09537104.2016.1143922
Keywords: platelet-rich plasma, platelet-poor plasma, platelet lysate, platelet concentrates, leucocytes, antibacterial effect, dogs
Citation: Attili A-R, Iacoucci C, Serri E, Cuteri V, Cantalamessa A, Linardi M, Rifici C, Mazzullo G, Rossi G, Galosi L and Tambella AM (2021) Antibacterial Properties of Canine Platelet-Rich Plasma and Other Non-Transfusional Hemo-Components: An in vitro Study. Front. Vet. Sci. 8:746809. doi: 10.3389/fvets.2021.746809
Received: 24 July 2021; Accepted: 26 August 2021;
Published: 04 October 2021.
Edited by:
Roberta Perego, University of Milan, ItalyReviewed by:
Piera Anna Martino, University of Milan, ItalyLivia Camargo Garbin, The University of the West Indies St. Augustine, Trinidad and Tobago
Copyright © 2021 Attili, Iacoucci, Serri, Cuteri, Cantalamessa, Linardi, Rifici, Mazzullo, Rossi, Galosi and Tambella. This is an open-access article distributed under the terms of the Creative Commons Attribution License (CC BY). The use, distribution or reproduction in other forums is permitted, provided the original author(s) and the copyright owner(s) are credited and that the original publication in this journal is cited, in accordance with accepted academic practice. No use, distribution or reproduction is permitted which does not comply with these terms.
*Correspondence: Adolfo Maria Tambella, YWRvbGZvbWFyaWEudGFtYmVsbGEmI3gwMDA0MDt1bmljYW0uaXQ=
†These authors have contributed equally to this work