- 1Department of Neuroimmunology, School of Medicine, Western Sydney University, Campbelltown, NSW, Australia
- 2New South Wales Department of Primary Industries, Elizabeth Macarthur Agricultural Institute, Menangle, NSW, Australia
- 3Department of Veterinary Anatomic Pathology, School of Veterinary Medicine, University of Melbourne, Werribee, VIC, Australia
Chronic intoxication with tryptamine-alkaloid-rich Phalaris species (spp.) pasture plants is known colloquially as Phalaris staggers syndrome, a widely occurring neurological disorder of sheep, cattle, horses, and kangaroos. Of comparative interest, structurally analogous tryptamine-alkaloids cause experimental parkinsonism in primates. This study aimed to investigate the neuropathological changes associated with spontaneous cases of Phalaris staggers in sheep with respect to those encountered in human synucleinopathy. In sheep affected with Phalaris staggers, histological, immunohistochemical, and immunofluorescence analysis revealed significant accumulation of neuromelanin and aggregated α-synuclein in the perikaryon of neurons in the cerebral cortex, thalamus, brainstem, and spinal cord. Neuronal intracytoplasmic Lewy bodies inclusions were not observed in these cases of ovine Phalaris staggers. These important findings established a clear link between synucleinopathy and the neurologic form of Phalaris plant poisoning in sheep, demonstrated in six of six affected sheep. Synucleinopathy is a feature of a number of progressive and fatal neurodegenerative disorders of man and may be a common endpoint of such disorders, which in a variety of ways perturb neuronal function. However, whether primary to the degenerative process or a consequence of it awaits clarification in an appropriate model system.
Introduction
Synucleinopathies are a group of neurodegenerative diseases characterized by the accumulation of α-synuclein and formation inclusions in neurons or oligodendrocytes (1). A recent study by Tayebi and colleagues demonstrated that Phalaris toxicity (PT) led to an accumulation of neuromelanin and aggregated α-synuclein in the central and peripheral nervous system of kangaroos after intoxication with tryptamine-alkaloid-rich Phalaris spp. pasture plants (2). PT is known to affect sheep as well as cattle and horses at pasture; this widely used and studied perennial pasture plant can become toxic after heavy rainfall, which follows a prolonged period of dry weather (3). Sheep with Phalaris staggers, a neurologically progressive and fatal form of PT (4–8), exhibit gait abnormality, hyperexcitability, muscle tremors, incoordination, limb paresis, convulsions, recumbency, collapse/falling, and death (6, 9). It was previously reported that sheep affected with Phalaris staggers show neuropathological lesions that included intense macroscopic green discoloration of the gray matter of several brain regions, including thalamus, brainstem, and ventral horns of the spinal cord and microscopic intraneuronal brown pigmentation (3).
It has long been believed that the serotonin-analogous tryptamine alkaloids (TA) cause Phalaris staggers in sheep (7, 9, 10). TA causes Phalaris staggers due to competitive binding to the serotonergic receptors (10). A historically important landmark study reported by Gallagher et al. (11) demonstrated that sheep developed clinical signs of Phalaris staggers after administration of TA (11), specifically N,N-dimethylated tryptamine alkaloids of Phalaris tuberosa, which induced violent convulsions with spasticity. Similarly, the synthetic MPTP drug, structurally analogous to TA-derivatives (12), caused parkinsonism in humans and monkeys (13–16), an indication that alkaloids might be directly or indirectly implicated in parkinsonism syndromes (17, 18).
In this study, we demonstrate the accumulation of aggregated α-synuclein and neuromelanin in the central nervous system (CNS) of sheep naturally affected by Phalaris staggers. This is the first study to link Phalaris plant poisoning in sheep with α-synucleinopathy classically associated with parkinsonism and some other human neurodegenerative disorders.
Background
Phalaris plants are widely cultivated as pasture grasses because they are robust and grow well on varying soil types. The syndrome of Phalaris staggers is recognized worldwide, and farming communities manage their pastures to minimize losses, but at times cases occur. For the purposes of this investigation, from the Elizabeth Macarthur Agricultural Institute (EMAI), New South Wales (NSW) Department of Primary Industries, we collected archived tissues from six mixed-breed juvenile to 4.5-year-old sheep. Dead or moribund sheep had been submitted for diagnostic postmortem examination between January and November 2017. They were all known to graze on farms across NSW (Table 1) with a history of PT and were exposed to Phalaris spp. After access to Phalaris pastures for some period, all six sheep presented to the referring large animal veterinarian with a variety of neurological signs, which included ataxia, paralysis/paresis, falling, tremor, circling, recumbency, and eventually death (Table 1). After gross postmortem examinations and histopathological assessment at the EMAI, all were diagnosed with chronic Phalaris neurotoxicity based on plant exposure, clinical presentation, and neuropathological changes (Table 1). In all cases, extensive diagnostic investigations ruled out other neurological and non-neurological disorders. The five non-Phalaris-exposed control sheep were cases submitted to EMAI between March and September 2018. Most were classified as adults and some Merino; 2/5 were ewes with pregnancy toxemia, whereas other miscellaneous diagnoses were made. One sudden death case remained undiagnosed, but Phalaris neurotoxicity was ruled out.
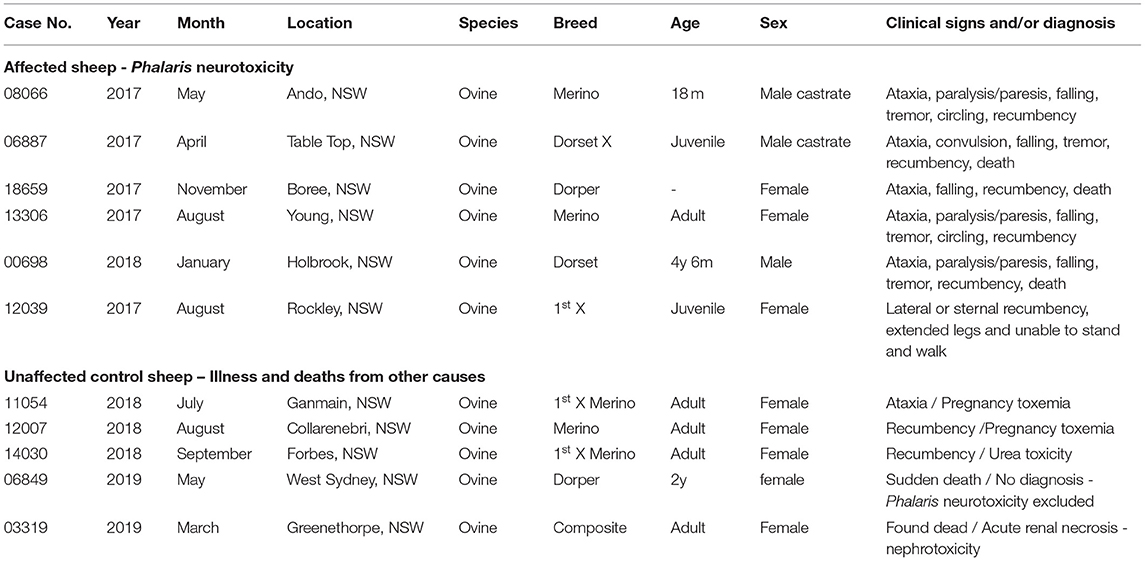
Table 1. Signalment, clinical, and epidemiological details of sheep with Phalaris staggers and controls.
Case Presentation
Animals and Ethics Statement
Six Phalaris-affected sheep and five unaffected controls that succumbed to other disorders (Table 1) were submitted for routine diagnostic postmortem examinations to EMAI having died recently or in extremis and were killed. As such, they are not subject to animal ethics guidelines. All Phalaris-affected and unaffected animals were from various rural areas in the state of NSW, Australia. They represented Merino and other sheep breeds, both males and females, some juvenile and others adult. The oldest known age was 4.5 years (affected).
Clinical Presentation
All Phalaris-affected sheep showed signs of a neurologic disorder; the most prominent signs included incoordination, hyperexcitability, muscle tremors, abnormal gait, thoracic and pelvic limb paresis, convulsions, recumbency, falling, and death. The control sheep died from various conditions, including pregnancy toxemia, urea toxicity, and acute renal necrosis. Phalaris was excluded in the control sheep after clinical and pathological diagnostic investigations.
Histopathology and Immunohistochemistry
For this study, histopathology and immunohistochemistry were performed as described (2). Briefly, the brains of sheep were fixed by immersion in 10% neutral buffered formalin. Four-micrometer sections were stained with hematoxylin and eosin (H&E) or Warthin–Starry stain for neuromelanin or used for immunohistochemistry. After treatment with 80% formic acid for 5 min, sections were subjected to 3% hydrogen peroxide to block endogenous peroxidase activity. This was then followed by staining with 97/8 (19), MJRF1 (Abcam, ab138501) rabbit anti-human α-synuclein polyclonal immunoglobulin G (IgG) (97/8; 1:2,000 dilution), anti-glial fibrillary acidic protein (GFAP) (EMD Millipore, MAB360), or Iba1 macrophage activation marker (Sigma Life Science, SAB2500042). Sections were visualized using the LSAB™ kit (labeled streptavidin–biotin, DAKO) then counterstained with Mayer's hematoxylin. Areas of the brain that were informative were the cerebral cortex, thalamus, brainstem, and spinal cord (Figure 1). Sections stained with H&E showed that, widely, neurons appeared morphologically normal except for the presence of mild to moderate brown multifocal intraneuronal neuromelanin pigments (Figures 1D–F). Neuronal chromatolysis, necrosis, or neuronophagia were absent. These morphological changes were not observed in unaffected sheep (Figures 1A–C). Iba1 staining revealed scarce naïve microglia distribution (data not shown), whereas anti-GFAP antibody displayed strong astrocytic staining (Figure 2). The neuromelanin-like granules were visualized using the WS reaction, which produced an intense dark brown to black discoloration in the cerebral cortex, thalamus, brainstem, and spinal cord neurons of Phalaris-affected sheep (Figures 3C,D). WS-specific neuromelanin granules were confined to the neuronal perikarya and not identified in dendrites or axons; in contrast, neuromelanin granules were not observed in unaffected sheep (Figures 3A,B). To assess whether GFAP increase correlated with neuromelanin intensity staining, we performed image quantification of three sections from each Phalaris-affected (Table 1; Figure 4). Three different areas of the cerebral cortex were analyzed. Signal intensity was visualized by capturing brown (GFAP) and dark (Warthin–Starry) color intensity field images using the Olympus vs. 120 Slide Scanner. Images were analyzed using “Olympus OlyVIA” software. Image processing software, cellSense (Olympus), was used for quantification, and the mean color threshold (brown particles for astroglia and dark for neuromelanin) was calculated in several brain regions, and the result was presented as percentage signal intensity and expressed as mean ± standard error of the mean. Signal intensity of both astroglia and neuromelanin in Phalaris-affected sheep was significantly elevated when compared with that of control unaffected sheep (p < 0.005) (data not shown). Furthermore, neuromelanin signal intensity positivity correlated with GFAP elevation (p < 0.0001; r = 0.5211) (Figure 4), indicating a direct molecular interaction between gliosis and neuromelanosis in Phalaris-affected sheep.
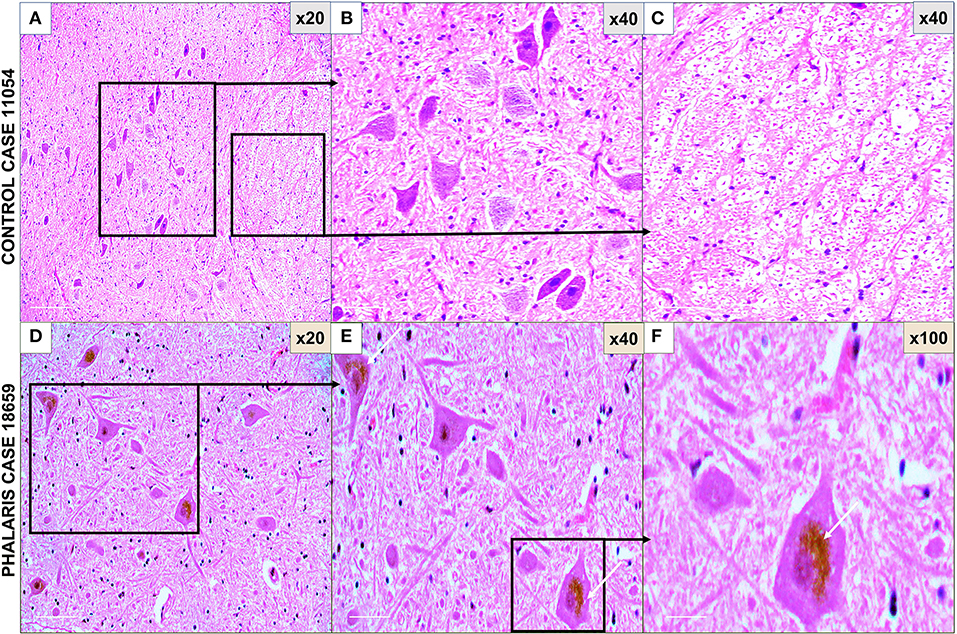
Figure 1. Photomicrographs of microscopic lesions in central nervous system of Phalaris-affected sheep. (A) Normal appearance of brain parenchyma of brainstem of an unaffected sheep (case 11,054). (B,C) Areas a higher magnification of (A). (D) Intense neuromelanin brown pigments in several neurons observed on routine H&E-stained sections of brainstem of a Phalaris-affected sheep (case 18,659). (E,F) Higher magnification of (D) with an inset showing a perinuclear cap of pigment (white arrows). Representative of all affected and unaffected sheep.
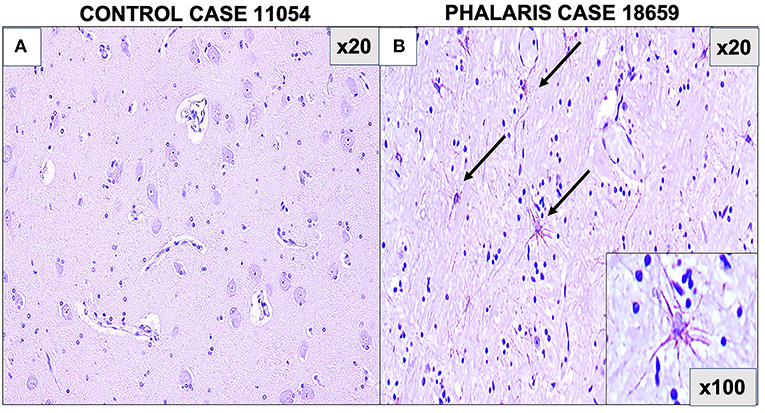
Figure 2. Photomicrographs of GFAP staining in central nervous system of Phalaris-affected sheep. (A) Staining for fibrillary astrocyte in brainstem brain sections of an unaffected sheep (case 11,054). (B) Staining for fibrillary astrocyte (black arrows) in brainstem brain sections of a Phalaris-affected sheep (case 18,659). Representative of all affected and unaffected sheep.
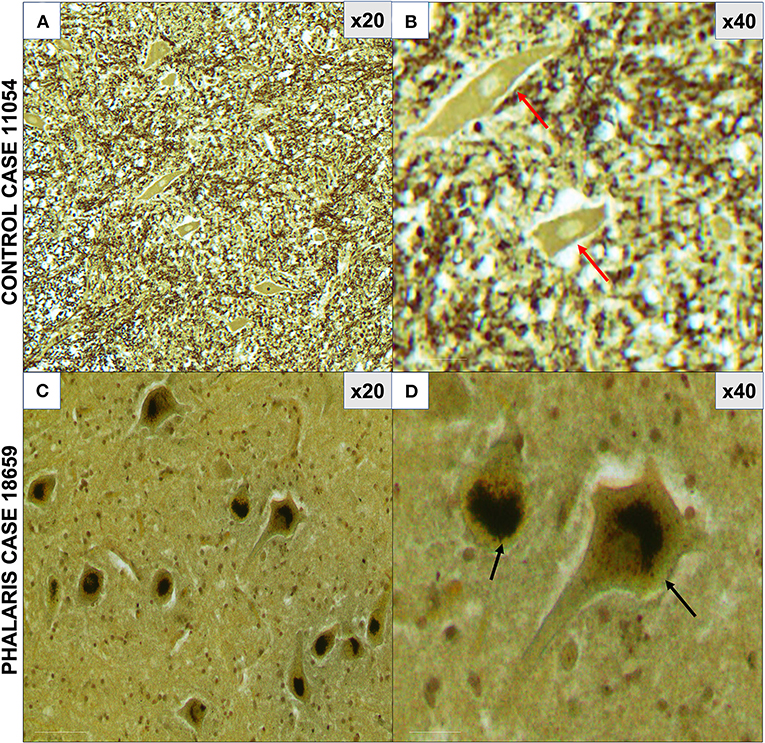
Figure 3. Photomicrographs of neuromelanin in central nervous system of Phalaris-affected sheep. (A) Warthin–Starry reaction did not display presence of neuromelanin in neurons in control unaffected sheep (case 11,054). Note absence of pigments in neurons. (B) Higher magnification of (A). (C) Abundant neuronal intracytoplasmic melanin pigmentation in cerebral cortex (black arrows) revealed by Warthin–Starry reaction stain (case 18,659). (D) Higher magnification of (C) and shows a perinuclear distribution (black arrows). Representative of all affected and unaffected sheep. Red arrow points to a healthy neuron.
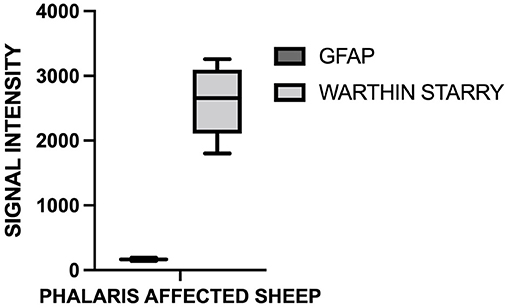
Figure 4. Quantification of GFAP and neuromelanin in Phalaris-affected sheep. GFAP and neuromelanin in cerebral cortex were quantified in six Phalaris-affected sheep. Signal intensity of both GFAP and neuromelanin were correlated (p < 0.0001; r = 0.5211). Data represent mean signal intensity. One-way analysis of variance with Dunnett's post-test was performed using GraphPad Prism version 7.00 for Windows (GraphPad, San Diego, CA, USA) for statistical analysis.
As the presence in neurons of α-synuclein was previously documented in eastern gray kangaroos with Phalaris staggers syndrome (2), we sought confirmation of its presence in this study in affected sheep and anticipated its absence in the control sheep that died from other causes. The 97/8 (Figures 5A,B) and MJFR1 (Figures 5C,D) rabbit anti-human α-synuclein antibodies were used to immunodetect α-synuclein, Lewy bodies, and associated Lewy neurites (20) in sheep affected with Phalaris staggers. Similar to our previous observation reported for eastern gray kangaroos, immunostaining displayed a mix of a fine punctate and conspicuous diffuse perikaryal pattern of aggregated α-synuclein in the cerebral cortex, thalamus, brainstem, and spinal cord of Phalaris-affected sheep, but Lewy bodies and Lewy neurites were not present (Figure 5). Of note, aggregated α-synuclein was not observed in unaffected sheep, which displayed the typical diffuse, normal pattern in synaptic terminals (Figures 5E,F).
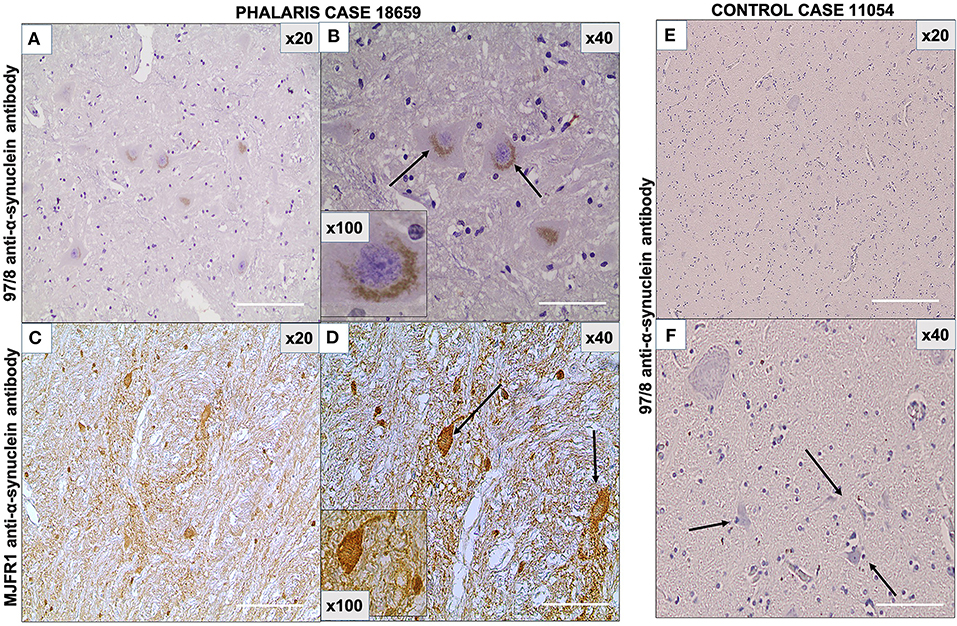
Figure 5. Photomicrographs of immunohistochemical demonstration of α-synuclein in central nervous system of a Phalaris-affected sheep. (A) 97/8 anti-α-synuclein antibody applied to brainstem sections of a Phalaris-affected sheep (case 18,659), which shows densely immunoreactive cytoplasmic aggregates, ranging from ovoid and fusiform to thread-like intensely stained structures. (B) Higher magnification of (A). There is a perinuclear pattern and neurons with seemingly solid deposits (black arrows). (C) MJFR1 anti-α-synuclein antibody of brainstem sections of Phalaris-affected sheep (case 18,659), which shows scattered, strongly immunoreactive neurons and clusters of axons in sweeping, somewhat curved bundles widespread cytosolic distribution of aggregate structures. (D) Higher magnification of (C) showing α-synuclein aggregate structures (black arrows). (E) 97/8 anti-α-synuclein antibody applied to brainstem sections of an unaffected sheep (case 11,054), which does display α-synuclein cytoplasmic aggregates. (F) Higher magnification of (E) showing normal neurons (black arrows). Representative of all affected and unaffected sheep.
Immunofluorescence Staining
Immunofluorescence staining was performed as described (2). Briefly, brain sections were processed for immunohistochemical procedures then stained with GFAP mouse monoclonal antibody IgG (EMD Millipore, MAB360) or 97/8 rabbit polyclonal antibody for 1 h at room temperature. Secondary antibodies diluted in phosphate-buffered saline (anti-mouse IgG fluorescein isothiocyanate-conjugate, Sigma; anti-rabbit IgG Texas-red-conjugate, Sigma) were added for 1 h at room temperature. Fluorescence microscopy was performed with a Leica DM4000B microscope. Images from each source [fluorescein isothiocyanate (450–490 nm) and Texas red (510–560 nm)] were collected by a high-resolution DC500 color camera attached. All images are saved digitally using Leica's IM500 Image Manager Database software from the same field of view. Images were merged using Photoshop 6.0 (Adobe). Confocal laser scanning microscopy was performed with a Zeiss LSM510 confocal system on an inverted Zeiss Axio100M. Z-series and snapshot images were collected. Dual scans were merged using Photoshop 6.0 (Adobe). This particular study showed that α-synuclein aggregates homed to neurons as confirmed by 97/8 staining and co-localized with astrocytes (Figures 6A,B). However, control sheep dis not display presence of a-synuclein aggregates (Figures 6C,D).
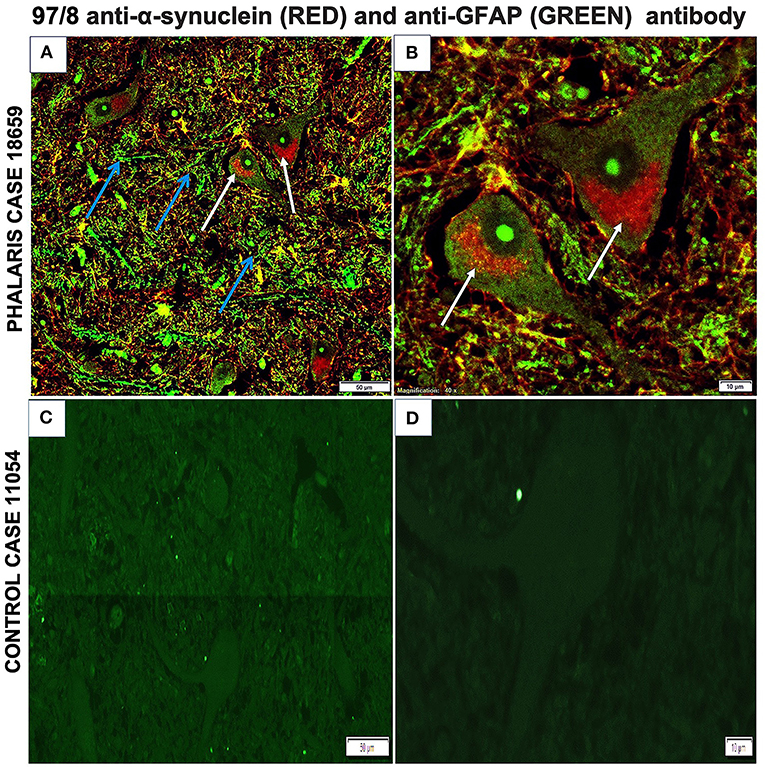
Figure 6. Immunofluorescence co-localization of α-synuclein and GFAP in central nervous system of a Phalaris-affected sheep. (A) Cerebral co-staining with 97/8 polyclonal anti-α-synuclein IgG antibody (white arrow) and anti-GFAP monoclonal IgG antibody (blue arrow) in brainstem sections of Phalaris-affected sheep (case 18,659) show perinuclear α-synuclein deposition. (B) Higher magnification of (A). (C) Cerebral staining with 97/8 polyclonal anti-α-synuclein IgG antibody (red) and anti-GFAP monoclonal IgG antibody (green) of brain sections of control unaffected sheep (case 11,054). Representative of all affected and unaffected sheep. (D) Higher magnification of (C).
Discussion
Phytochemicals are structurally diverse biologically active compounds of plant origin that possess various beneficial and toxic properties toward synucleinopathies (21). Here, we describe the effect of alkaloids from Phalaris on the development of pathology in sheep. We have recently reported cases of synucleino-neuromelanopathy in eastern gray kangaroos affected with PT after ingestion of tryptamine-alkaloid-rich Phalaris spp. In this study, we also describe cases of α-synucleino-neuromelanopathy in sheep associated with PT after grazing on Phalaris pastures. These animals displayed typical neurological deficits previously described for sheep affected with Phalaris staggers (3, 4, 8, 9, 22) and included ataxia, paralysis/paresis, falling, tremor, circling, recumbency, and death. These neurological deficits implicate the caudal brain stem and spinal cord (ataxia, paresis/paralysis, and potentially circling) and possibly further areas of the CNS. Microscopic assessment of H&E-stained brain sections from the sheep confirmed the neuronal accumulation of the neuromelanin brown pigments in these affected animals in the cerebral cortex, thalamus, brainstem, and spinal cord, whereas unaffected animals remained neuromelanin-free. The clinical findings mirror those described previously by other authors for sheep (8, 9, 23–28) and the more recently described lesions in kangaroos (2), which showed conspicuous accumulation of neuromelanin pigments in the neurons of the central and enteric nervous systems, implicating a causative role for the Phalaris plants whose neurotoxicity is well known (6, 8). Moreover, and comparable to our findings in kangaroos (2), the Warthin–Starry reaction is consistent with these neuronal pigments being neuromelanin. In humans, the presence of aggregated neurotoxic species of α-synuclein in the CNS signifies a hallmark of Parkinson's disease (PD) and other related neurodegenerative disorders (29–33). The α-synuclein appearance was previously described as ovoid and fusiform to thread-like aggregates in human PD (29–33). A similar appearance was described in kangaroos affected with PT (2). Although the α-synuclein presentation was morphologically similar in some neurons of sheep affected with PT, the majority presented as fine punctate and conspicuous diffuse perikaryal pattern. This differential expression might suggest an acute clinical phase of the disorder, but whether this is the case needs further confirmation. To rule out whether this difference in the α-synuclein presentation was artifactual and might have been due to α-antibody specificity, staining with the 97/8 rabbit anti-human α-synuclein antibody (19) was confirmed with the widely used and validated MJFR1 rabbit anti-human α-synuclein antibody raised against human recombinant full-length α-synuclein and mapped to amino acids 118–123 (VDPDNE). Of note, a high degree of sequence homology was found between α-synuclein of sheep and α-synuclein of humans (~96%) (34).
The presence of aggregated α-synuclein in neurons of sheep brains was demonstrated in several areas, including the cerebral cortex, thalamus, brainstem, and spinal cord, and near neuromelanin pigments. Both peracute (cardiac) and delayed, chronic (CNS) toxicities are associated with Phalaris consumption. Bourke (3) showed that one neurotoxic alkaloid does not cause cardiac syndrome. The extensive distribution of α-synuclein in different brain regions suggests a potential effect on multiple neuronal populations. It is common for neuropathological studies to reveal more widespread neural injury than indicated by the clinical signs. Gradual poisoning by ingestion might involve progressively wider CNS accumulation, which in time and with longer survival, might mirror neuropathological changes associated with PD (35). However, in a study of α-synuclein accumulation in scrapie-affected sheep and goats, Adjou et al. (36) found widespread granular deposits in the brains, especially the hippocampus and cerebellum. Astrocytosis noted in areas bearing only α-synuclein deposits was believed to suggest a role for synucleinopathy in sheep and goat scrapie. Of interest, in this second neurological disorder of sheep (and goats), α-synuclein deposits in the brain were global, and furthermore, Lewy body formation was not described. All sheep included in these studies and affected with Phalaris staggers showed microglial activation and gliosis.
It was previously reported that the molecular mechanisms underlying the degeneration of axons are distinct from those affecting neuronal bodies. It was confirmed that axonal loss precedes neuronal cell death in PD and other neurodegenerative disorders (37). Moreover, and despite the lack of conclusive evidence related to the molecular mechanisms associated with α-synuclein aggregates accumulation in human idiopathic PD and other parkinsonism syndromes, tryptamine-alkaloids analogous MTPP was shown to cause nigrostriatal toxicity and leads to chronic parkinsonism in humans and monkeys (13–16).
In this report, we described cases of synucleinopathies in sheep, which appear to be as a consequence of ingesting alkaloid-rich Phalaris spp. This study helped characterize the sheep as a higher mammalian model for examining the consequences of progressive CNS synucleinopathy with relevance to parkinsonism. Akin to current methodology to evaluate brain β-amyloid plaques with (18)F-labeled tracers for positron emission tomography, it may be possible in the sheep brain to image α-synuclein accumulation. Most importantly for the veterinary profession, this provides an additional new postmortem diagnostic tool for ovine Phalaris staggers.
Data Availability Statement
The original contributions presented in the study are included in the article/supplementary material, further inquiries can be directed to the corresponding author/s.
Ethics Statement
Ethical review and approval was not required for the animal study because Sheep were submitted for routine diagnostic post-mortem examination to the Department of Primary Industries and as such are not subject to animal ethics guidelines.
Author Contributions
MT: conceived and designed experiments and wrote the manuscript. PP, UH, and RK: performed experiments. MD: designed experiments and revised manuscript. BS: conceived experiments and revised manuscript. All authors contributed to the article and approved the submitted version.
Funding
This study was supported by the University of Western Sydney Start Up funds to MT.
Conflict of Interest
The authors declare that the research was conducted in the absence of any commercial or financial relationships that could be construed as a potential conflict of interest.
Publisher's Note
All claims expressed in this article are solely those of the authors and do not necessarily represent those of their affiliated organizations, or those of the publisher, the editors and the reviewers. Any product that may be evaluated in this article, or claim that may be made by its manufacturer, is not guaranteed or endorsed by the publisher.
Acknowledgments
We wish to thank the staff at the Histology Facility, Elizabeth Macarthur Agricultural Institute, New South Wales Department of Primary Industries, Menangle, New South Wales, Australia.
References
1. Surguchev AA, Surguchov A. Synucleins and gene expression: ramblers in a crowd or cops regulating traffic? Front Mol Neurosci. (2017) 10:224. doi: 10.3389/fnmol.2017.00224
2. Tayebi M, El-Hage CM, Pinczowski P, Whiteley P, David M, Li QX, et al. Summers, Plant poisoning leads to alpha-synucleinopathy and neuromelanopathy in kangaroos. Sci Rep. (2019) 9:16546. doi: 10.1038/s41598-019-53396-8
3. Bacci B, Whiteley PL, Barrow M, Phillips PH, Dalziel J, El-Hage CM. Chronic Phalaris toxicity in eastern grey kangaroos (Macropus giganteus). Aust Vet J. (2014) 92:504–8. doi: 10.1111/avj.12272
4. Bourke CA, Colegate SM, Culvenor RA. Evidence that N-methyltyramine does not cause Phalaris aquatica-related sudden death in ruminants. Aust Vet J. (2006) 84:426–7. doi: 10.1111/j.1751-0813.2006.00075.x
5. McDonald IW. Studies on the etiology of Phalaris staggers in sheep; a preliminary report. Aust Vet J. (1946) 22:91–4. doi: 10.1111/j.1751-0813.1946.tb06454.x
6. Le Souef HD. Poisoning of sheep by Phalaris tuberosa. Aust Vet J. (1948) 24:12. doi: 10.1111/j.1751-0813.1948.tb04587.x
7. Gallagher CH, Koch JH, Hoffman H. Diseases of sheep due to ingestion of Phalaris tuberosa. Aust Vet J. (1966) 42:279–86. doi: 10.1111/j.1751-0813.1966.tb08836.x
8. Bourke CA, Carrigan MJ, Seaman JT, Evers JV. Delayed development of clinical signs in sheep affected by Phalaris aquatica staggers. Aust Vet J. (1987) 64:31–2. doi: 10.1111/j.1751-0813.1987.tb06057.x
9. Bourke CA, Carrigan MJ, Dixon RJ. The pathogenesis of the nervous syndrome of Phalaris aquatica toxicity in sheep. Aust Vet J. (1990) 67:356–8. doi: 10.1111/j.1751-0813.1990.tb07400.x
10. Shen HW, Jiang XL, Winter JC, Yu AM. Psychedelic 5-methoxy-N,N-dimethyltryptamine: metabolism, pharmacokinetics, drug interactions, and pharmacological actions. Curr Drug Metab. (2010) 11:659–66. doi: 10.2174/138920010794233495
11. Gallagher CH, Koch JH, Hoffman H. Electro-myographic studies on sheep injected with the N,N-dimethylated tryptamine alkaloids of Phalaris tuberosa. Int J Neuropharmacol. (1967) 6:223–8. doi: 10.1016/0028-3908(67)90009-3
12. Chiba K, Trevor A, Castagnoli N Jr. Metabolism of the neurotoxic tertiary amine, MPTP, by brain monoamine oxidase. Biochem Biophys Res Commun. (1984) 120:574–8. doi: 10.1016/0006-291X(84)91293-2
13. Burns RS, Chiueh CC, Markey SP, Ebert MH, Jacobowitz DM, Kopin IJ. A primate model of parkinsonism: selective destruction of dopaminergic neurons in the pars compacta of the substantia nigra by N-methyl-4-phenyl-1,2,3,6-tetrahydropyridine. Proc Natl Acad Sci U S A. (1983) 80:4546–50. doi: 10.1073/pnas.80.14.4546
14. Davis GC, Williams AC, Markey SP, Ebert MH, Caine ED, Reichert CM, et al. Chronic parkinsonism secondary to intravenous injection of meperidine analogues. Psychiatry Res. (1979) 1:249–54. doi: 10.1016/0165-1781(79)90006-4
15. Langston JW, Ballard P, Tetrud JW, Irwin I. Chronic parkinsonism in humans due to a product of meperidine-analog synthesis. Science. (1983) 219:979–80. doi: 10.1126/science.6823561
16. Langston JW, Forno LS, Rebert CS, Irwin I. Selective nigral toxicity after systemic administration of 1-methyl-4-phenyl-1,2,5,6-tetrahydropyrine (MPTP) in the squirrel monkey. Brain Res. (1984) 292:390–4. doi: 10.1016/0006-8993(84)90777-7
17. Barbeau A. Etiology of Parkinson's disease: a research strategy. Can J Neurol Sci. (1984) 11:24–8. doi: 10.1017/S0317167100045273
18. Calne DB, Langston JW. Aetiology of Parkinson's disease. Lancet. (1983) 2:1457–9. doi: 10.1016/S0140-6736(83)90802-4
19. Culvenor JG, McLean CA, Cutt S, Campbell BC, Maher F, Jakala P, et al. Non-abeta component of Alzheimer's disease amyloid (NAC) revisited. NAC and alpha-synuclein are not associated with abeta amyloid. Am J Pathol. (1999) 155:1173–81. doi: 10.1016/S0002-9440(10)65220-0
20. Halliday GM, Ophof A, Broe M, Jensen PH, Kettle E, Fedorow H, et al. Alpha-synuclein redistributes to neuromelanin lipid in the substantia nigra early in Parkinson's disease. Brain. (2005) 128:2654–64. doi: 10.1093/brain/awh584
21. Surguchov A, Bernal L, Surguchev AA. Phytochemicals as regulators of genes involved in synucleinopathies. Biomolecule. (2021) 11:624. doi: 10.3390/biom11050624
22. Bourke CA, Colegate SM, Rendell D, Bunker EC, Kuhn RP. Peracute ammonia toxicity: a consideration in the pathogenesis of Phalaris aquatica 'Polioencephalomalacia-like sudden death' poisoning of sheep and cattle. Aust Vet J. (2005) 83:168–71. doi: 10.1111/j.1751-0813.2005.tb11631.x
23. Colegate SM, Anderton N, Edgar J, Bourke CA, Oram RN. Suspected blue canary grass (Phalaris coerulescens) poisoning of horses. Aust Vet J. (1999) 77:537–8. doi: 10.1111/avj.1999.77.8.537
24. Creeper JH, Mitchell AA, Jubb TF, Colegate SM. Pyrrolizidine alkaloid poisoning of horses grazing a native heliotrope (Heliotropium ovalifolium). Aust Vet J. (1999) 77:401–2. doi: 10.1111/j.1751-0813.1999.tb10318.x
25. East NE, Higgins RJ. Canary grass (Phalaris sp) toxicosis in sheep in California. J Am Vet Med Assoc. (1988) 192:667–9.
26. Lean IJ, Anderson M, Kerfoot MG, Marten GC. Tryptamine alkaloid toxicosis in feedlot sheep. J Am Vet Med Assoc. (1989) 195:768–71.
27. Nicholson SS, Olcott BM, Usenik EA, Casey HW, Brown CC, Urbatsch LE, et al. Delayed Phalaris grass toxicosis in sheep and cattle. J Am Vet Med Assoc. (1989) 195:345–6.
28. Bourke CA, Carrigan MJ, Dixon RJ. Upper motor neurone effects in sheep of some beta-carboline alkaloids identified in zygophyllaceous plants. Aust Vet J. (1990) 67:248–51. doi: 10.1111/j.1751-0813.1990.tb07778.x
29. Arima K, Ueda K, Sunohara N, Arakawa K, Hirai S, Nakamura M, et al. NACP/alpha-synuclein immunoreactivity in fibrillary components of neuronal and oligodendroglial cytoplasmic inclusions in the pontine nuclei in multiple system atrophy. Acta Neuropathol. (1998) 96:439–44. doi: 10.1007/s004010050917
30. Galvin JE, Uryu K, Lee VM, Trojanowski JQ. Axon pathology in Parkinson's disease and Lewy body dementia hippocampus contains alpha-, beta-, and gamma-synuclein. Proc Natl Acad Sci U S A. (1999) 96:13450–5. doi: 10.1073/pnas.96.23.13450
31. Irizarry MC, Growdon W, Gomez-Isla T, Newell K, George JM, Clayton DF, et al. Nigral and cortical Lewy bodies and dystrophic nigral neurites in Parkinson's disease and cortical lewy body disease contain alpha-synuclein immunoreactivity. J Neuropathol Exp Neurol. (1998) 57:334–7. doi: 10.1097/00005072-199804000-00005
32. Crowther RA, Daniel SE, Goedert M. Characterisation of isolated alpha-synuclein filaments from substantia nigra of Parkinson's disease brain. Neurosci Lett. (2000) 292:128–30. doi: 10.1016/S0304-3940(00)01440-3
33. Braak H, Sandmann-Keil D, Gai W, Braak E. Extensive axonal lewy neurites in Parkinson's disease: a novel pathological feature revealed by alpha-synuclein immunocytochemistry. Neurosci Lett. (1999) 265:67–9. doi: 10.1016/S0304-3940(99)00208-6
34. Vojdani A, Lerner A, Vojdani E. Cross-reactivity and sequence homology between alpha-synuclein and food products: a step further for Parkinson's disease synucleinopathy. Cells. (2021) 10:1111. doi: 10.3390/cells10051111
35. Halliday GM, Holton JL, Revesz T, Dickson DW. Neuropathology underlying clinical variability in patients with synucleinopathies. Acta Neuropathol. (2011) 122:187–204. doi: 10.1007/s00401-011-0852-9
36. Adjou KT, Allix S, Ouidja MO, Backer S, Couquet C, Cornuejols MJ, et al. Alpha-synuclein accumulates in the brain of scrapie-affected sheep and goats. J Comp Pathol. (2007) 137:78–81. doi: 10.1016/j.jcpa.2007.03.007
Keywords: neuromelanopathy, neurotoxicity, parkinsonism, Phalaris, sheep, α-synuclein, synucleinopathy
Citation: Tayebi M, Pinczowski P, Habiba U, Khan R, David MA and Summers BA (2021) Case Report: Synucleinopathy Associated With Phalaris Neurotoxicity in Sheep. Front. Vet. Sci. 8:736567. doi: 10.3389/fvets.2021.736567
Received: 05 July 2021; Accepted: 09 September 2021;
Published: 14 October 2021.
Edited by:
Bruno Cozzi, University of Padua, ItalyReviewed by:
Andrei Surguchov, University of Kansas Medical Center, United StatesIrina G. Sourgoutcheva, University of Kansas Medical Center, United States
Natalie Landeck, National Institutes of Health (NIH), United States
Copyright © 2021 Tayebi, Pinczowski, Habiba, Khan, David and Summers. This is an open-access article distributed under the terms of the Creative Commons Attribution License (CC BY). The use, distribution or reproduction in other forums is permitted, provided the original author(s) and the copyright owner(s) are credited and that the original publication in this journal is cited, in accordance with accepted academic practice. No use, distribution or reproduction is permitted which does not comply with these terms.
*Correspondence: Mourad Tayebi, bS50YXllYmlAd2VzdGVybnN5ZG5leS5lZHUuYXU=