- Department of Clinical Sciences, North Carolina State University, Raleigh, NC, United States
Postoperative ileus (POI), a decrease in gastrointestinal motility after surgery, is an important problem facing human and veterinary patients. 37.5% of horses that develop POI following small intestinal (SI) resection will not survive to discharge. The two major components of POI pathophysiology are a neurogenic phase which is then propagated by an inflammatory phase. Perioperative care has been implicated, namely the use of opioid therapy, inappropriate fluid therapy and electrolyte imbalances. Current therapy for POI variably includes an early return to feeding to induce physiological motility, reducing the inflammatory response with agents such as non-steroidal anti-inflammatory drugs (NSAIDs), and use of prokinetic therapy such as lidocaine. However, optimal management of POI remains controversial. Further understanding of the roles of the gastrointestinal microbiota, intestinal barrier function, the post-surgical inflammatory response, as well as enteric glial cells, a component of the enteric nervous system, in modulating postoperative gastrointestinal motility and the pathogenesis of POI may provide future targets for prevention and/or therapy of POI.
Introduction
Postoperative ileus (POI), “a delay in the return of normal gastrointestinal motility following surgery” is a disease syndrome that has become increasingly important to manage in both veterinary and human health (1, 2). POI is a complex syndrome that first requires healthcare professionals to agree on correct terminology and definition in order to properly recognize and treat it. Among human healthcare professionals, the American Society for Enhanced Recovery After Surgery (ERAS) has defined a scoring system for what they termed “postoperative gastrointestinal dysfunction” (POGD) (3, 4). The system describes varying degrees of POI with a 0–2 normal score as nausea and vomiting within the first 24–48 h and progresses all the way to scores over 6 that qualify as POGD with painful abdominal distension with tympany, unrelenting nausea when treated with antiemetics, no bowel movements, and large volumes of bilious emesis (3, 4). In the equine field, efforts have been made to define POI as exhibiting over 4 L of gastric reflux with a pH >4.0 upon nasogastric intubation or 2 L/h with repeated intubation, persistent heart rate over 40 beats/min, abdominal discomfort, and evidence of distended SI on ultrasound or rectal palpation (2, 5). Due to variations in past terminology, the ranges of reported incidence rates are wide. Incidence is thought to be between 2 and 60% in human patients, and equine incidence following abdominal surgery is thought to be between 0 and 62% (2, 6–8). Even with a wide range of reported incidence, POI is estimated to cost the US healthcare 1.5 billion dollars each year, and it is well established that increased time within a hospital increases complications (8–13). POI is a critical postoperative complication in the equine field. Horses that develop POI following SI resection are 29.7 times less likely to survive to discharge than similar patients without POI (14). A unique aspect of equine veterinary medicine is that acute abdominal pain, or more commonly referred to as colic, is the most common medical emergency (15). Up to 17.5% of colicking horses require exploratory laparotomy (16–23). With this high incidence of equine patients undergoing gastrointestinal surgery and the significant impact of POI on patient survival rate, it is clear that the equine veterinary field provides a significant opportunity for the study of POI pathophysiology and therapy that will aid human health. In order to properly treat and prevent POI, an attempt must be made to understand its multifactorial pathophysiology. Combining the current knowledge that POI is incited by a rapid neurogenic response and then propagated through an inflammatory response with a better understanding of how breakdown of the intestinal mucosal barrier resulting in translocation of luminal contents contributes to the inflammatory response, and an increasing interest of the role of the microbiota and enteric glia in physiologic function of the gastrointestinal tract, we may hope to develop more successful interventions for our patients.
RISK FACTORS
While POI is a multifactorial disease process, medical professionals have been able to observe a variety of risk factors in both human and equine patients. Both species share a correlation between increased age and increased risk of POI as well as hypovolemia (2, 9–13, 24). In human patients, hypovolemia is noted specifically as blood loss in surgery, while in equine patients it is described as status at admission with tachycardia, increased packed cell volume, and increased serum total protein (2, 9–13, 24). Human risk factors for POI also include male gender, history of airway disease, and perioperative opiate use. Equine patients with SI surgical lesions are known to have an increased risk of POI, while human patients undergoing an ileostomy procedure are also at increased risk (2, 9–13, 24).
Neurogenic Pathophysiology
The development of POI is first incited by a neurogenic response to surgery. Figure 1 demonstrates an outline of how the progression of a surgical procedure leads to dysmotility (24). There is an overall shift in the relative activity of the two major branches of the autonomic nervous system, from normal parasympathetic tone that serves to regulate physiological motility patterns, to an abnormal level of sympathetic tone that tends to reduce motility, potentially leading to functional obstruction (24). Although it would be intuitive to conclude that POI is initiated at the intestinal level, in actuality, POI pathophysiology is initiated with an abdominal incision causing a glutamate release and an adrenergic inhibition of motility (25–28). A study in which a non-selective adrenoceptor antagonist, guanethidine, or an alpha two adrenoceptor antagonist, yohimbine, improved colon motility postoperatively in rats supports this notion (29). The trauma of intestinal manipulation is sensed by the vagus afferent nerves which signal to the hypothalamus (2). The hypothalamus activates a shift toward sympathetic neuronal control, and motility is further inhibited (2). There is also thought to be activation of splanchnic afferent neurons that leads to a non-adrenergic and non-cholinergic stimulation of the vagus nerve and reduction of gastrointestinal motility by vasoactive intestinal peptide and nitric oxide (25, 30). This pathway was demonstrated by adrenergic blockade only partially preventing decreased intestinal motility in rats. The addition of nitrergic blockade to adrenergic blockade successfully prevented POI in this model (25, 31). The impact of vagal and splanchnic activation on reduced gastrointestinal motility from abdominal surgery has also been demonstrated by an observed improvement to motility by cutting both of the nerves in dogs (32). Similarly, gastrointestinal motility following surgery has also been improved in human patients by high epidural local anesthesia (33–36). Most of the neuronal effect is thought to be abated after surgery is completed, however, it is important to consider the effects of a resection and anastomosis procedure on coordination of peristaltic waves (Figure 1) (37, 38). A study performed in mice receiving a SI resection demonstrated an impact on the interstitial cells of Cajal, and ultimately, slow waves and contractions (23). Similar effects have been observed in human distal colon resections (39, 40).
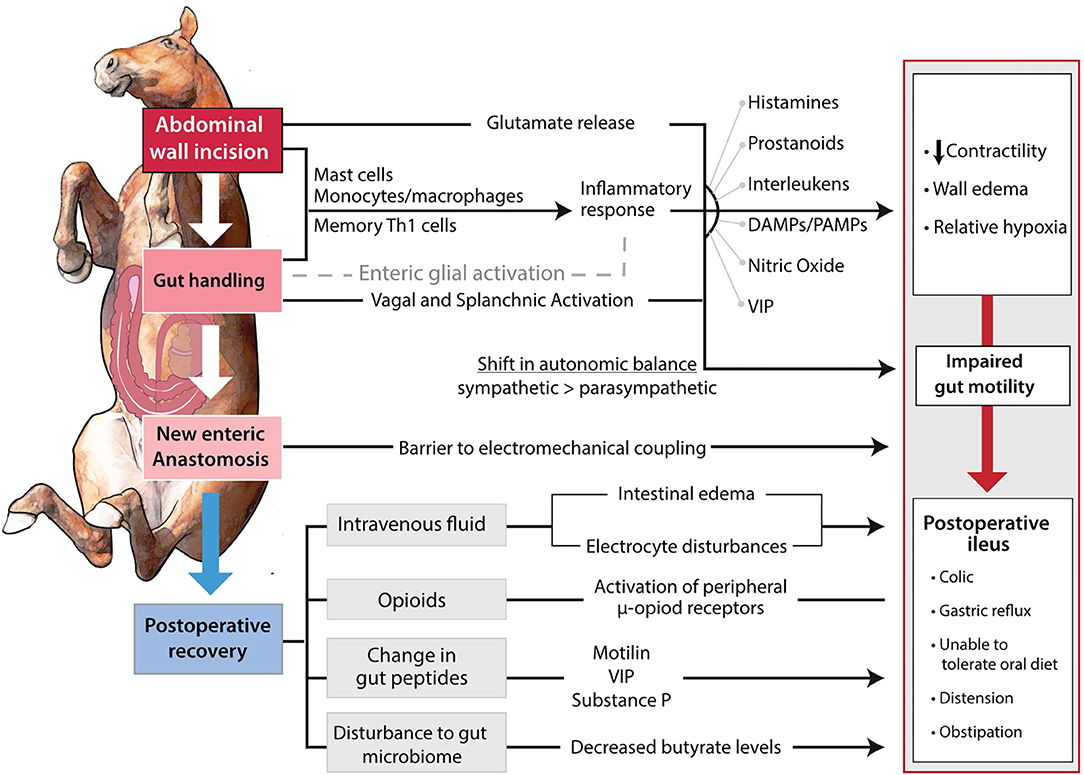
Figure 1. Pathways involved in the development of POI. The surgical incision and manipulation of tissue incite a neurogenic and inflammatory response. The combination of shift in autonomic balance and inflammatory products impair gastrointestinal motility by edema formation and decreased contractility. The impaired gastrointestinal motility is further propagated through the anastomosis site serving as an electromechanical barrier. Postoperatively, improper fluid therapy, opioid use, and potential changes to gastrointestinal peptides, further suppress motility.
Inflammatory Pathophysiology
The decreased motility induced by the initial neurogenic response is further compounded through an inflammatory response in the intestinal muscularis (Figure 1). The peritoneal incision and intestinal manipulation induce the release of proinflammatory molecules in a complex process that requires further definition (41, 42). It has been theorized that tissue handling increases epithelial permeability, which allows pathogen-associated molecular patterns (PAMPs) to travel into the tissue from the intestinal lumen (43). This underexplored mechanism may provide a method of POI prevention to be shown in further detail later. Further, it is hypothesized that the resident muscularis macrophages are stimulated and release prostaglandins and nitric oxide (44, 45). It is important to note that while murine macrophages have demonstrated release of nitric oxide in vitro, this has yet to be show in human and equine macrophages (46, 47). Monocytes, mast cells, and neutrophils enter the muscularis and the leukocytes release inflammatory molecules including inflammatory cytokines, reactive oxygen intermediates, and protease (44, 48–52). Leukocytic chemotaxis has been noted at 3 h postoperatively and is thought to peak at 24 h postoperatively (2). Infiltration of the intestine by inflammatory cells is thought to impair motility by inducing intestinal wall edema via increased capillary permeability and the relaxing effect of nitric oxide and prostaglandin E2 on smooth muscle (53–56). Both the innate and the adaptive immune system are thought to be involved in this inflammatory response. A study by Engel et al. (57) utilizing mice provided a major shift in our understanding of POI by suggesting that the spread of dysmotility throughout the gastrointestinal tract was through the involvement of memory Th1 cells and their activation by local dendritic cells. The impact of inflammation on development of POI has been demonstrated by the administration of anti-inflammatory IL-10 improving motility postoperatively in mice (2, 58). Murine studies have also demonstrated the prevention of POI through inhibiting mast cell and leukocyte chemotaxis (49–52). Interestingly, the prevention of monocyte chemotaxis was not successful in preventing POI and resulted in more neutrophil infiltration into the muscularis externa (59). Other experimental models in mice have shown the improvement of POI in response to mast cell stabilization, macrophage depletion via chlodronate liposomes, or electric vagal stimulation to impair macrophage activation (50, 52, 60, 61). Mast cell stabilization was not successful in improving POI in humans, but laparoscopic surgery has been shown to decrease their activation (51, 62). Additional factors such as the need to exteriorize the intestine, decompress its contents, or perform a resection and anastomosis will increase the inflammation within the intestinal muscularis (63, 64).
An interesting aspect of POI is that while only certain sections of the gastrointestinal tract may be handled during surgery, ileus is commonly panenteric. As previously mentioned in the inflammatory response, activation of memory Th1 cells by IL-12 from dendritic cells at the location of manipulation could contribute to the panenteric decrease in motility by their traveling through the bloodstream (57). It has been speculated that the movement of commensal endotoxins, and PAMPs into the intestinal muscularis due to increased permeability from tissue handling can cause systemic inflammation leading to reduced motility (65). Panenteric decreased motility has also been thought to be attributed to mast cell mediators spread throughout the peritoneum (49, 54, 66). Opioids and electrolyte imbalances would additionally have systemic effects (24).
The pivotal study by Engel et al. (57) hypothesized that intestinal manipulation leads to dendritic cells releasing IL-12 which brings memory Th1 cells to the gastrointestinal tract where they simulate resident macrophages with interferon γ, and the macrophages release nitric oxide. They were able to support the role of dendritic cells and Th1 cells by first showing in a co-culture in vitro model that Th1 cells would release interferon γ and second by conditional knockout of CD11C [+] dendritic cells in mice. They observed that the conditional knockout mice did not develop POI after intestinal manipulation. They also demonstrated that interferon γ knockout mice had improved motility when compared to control, and IL-12 p35 knockout mice had normal motility. These results illustrate the need for further study of the role of intestinal manipulation in POI pathophysiology.
Additional Pathophysiology Components
Neuropeptides, electrolytes, fluid therapy, and opioid use are also thought to contribute to POI development. As previously discussed, the inhibitory neuropeptide vasoactive intestinal peptide is thought to be involved in the non-adrenergic and non-cholinergic stimulation of the vagus nerve (25, 30). Surgery and postoperative fasting impact the levels of vasoactive intestinal peptide, and other stimulatory neuropeptides such as motilin and substance P (24). However, an understanding of neuropeptide levels in patients is required before potential new therapeutic targets may be developed (24). Electrolyte imbalances including hypokalemia, hypocalcemia, and hyponatremia have been observed to be significantly associated with POI and should be corrected in affected patients (9, 24). Anesthetic drugs are known to inhibit gastrointestinal motility, however, their use is unavoidable in many procedures. Opioids functioning at the u-opioid receptor inhibit gut motility through inhibition of acetylcholine release which in turn increases smooth muscle tone (2, 67). Its involvement in human POI is supported by the ability of alvimopan, a μ-opioid receptor antagonist, to increase postoperative recovery (68). This knowledge of opioid involvement appears to go underappreciated in the equine field, where a survey found that “87% of those reporting a POI incidence greater than the median incidence, declared the use of opioids in their treatment regiments” (2, 69, 70). Inappropriate fluid therapy may contribute to POI as a result of intestinal wall edema mentioned previously with inflammation, or electrolyte imbalance (24). Studies in human patients have demonstrated an association between ileus and crystalloid overload and report that for each additional liter of fluid received perioperatively, the overall risk of postoperative complications increases by 32% (71, 72).
Current Therapies
The current therapies for postoperative ileus are broad to address the multifactorial pathology, but unfortunately, are still largely ineffective. For example, NSAID therapy has been utilized to target the role of the inflammatory response in POI development. NSAID therapy has been shown to decrease time to first flatus and stool in human patients undergoing colorectal surgery (73). However, it is imperative to note that the negative impact of nonselective COX inhibition on intestinal healing continues to make it a controversial postoperative therapy (73). In addition, in a study performed on the effects of nonselective COX inhibition and COX-2 selective inhibition on horses that underwent surgery for strangulating SI lesions, 38 and 23% of each group, respectively, still developed POI (74). Prokinetic drugs such as erythromycin, lidocaine, metoclopramide, or neostigmine would seem to be the logical choice in a disease process involving decreased motility, but these drugs have had controversial dosage and efficacy (69, 70, 75). The most common choice of prokinetic is lidocaine (75). A study by Salem et al. (76) evaluated the efficacy of perioperative lidocaine on 318 horses undergoing surgery for SI lesions. Perioperative lidocaine did not have a significant impact on volume or duration of gastric reflux in these horses, an integral part of the clinical definition of equine POI. The lack of universally effective pharmaceutical treatment necessitates additional case management strategies in equine patients including early feeding, supportive fluid therapy, exercise, and avoidance of formation of infection, inflammation, endotoxemia, or adhesions (69). Early feeding encourages gastrointestinal motility and sham feeding in the form of gum chewing, and placing hay within sight but out of reach has been used in human and equine patients, respectively (77, 78). As previously mentioned, mast cell stabilizers in addition to IL-1R, p38 mitogen-activated protein kinase and ICAM-1 blockers, may be potential methods to reduce the inflammatory response (52, 60, 61, 79–81). The Engel et al. (57) group that studied the involvement of memory Th1 cells suggested that future therapies may include IL-12 blockade, or other methods of preventing Th1 cell migration. Vagal nerve stimulation may also be utilized to reduce the inflammatory response and macrophage stimulation, and a laparoscopic method has been developed in pigs (82–84).
Emerging Areas of Interest in Ileus Research
The gut microbiota has been demonstrated to change following surgical procedures, providing justification for study into its role in POI and as a novel potential target for more effective therapy (85, 86). Bifidobacterium and Lactobacillus probiotic genera have been suggested to provide an anti-inflammatory role, and potential for improving the intestinal barrier function (87–89). A 2020 study was performed in guinea pigs using Enterococcus faecaelis, Bacillus mesentericus, and Clostridium butyricum as a probiotic once daily for a week prior to initiation of POI (90). POI was initiated in guinea pigs by laparotomy with cecal manipulation. Overall, the probiotic group had significantly better motility compared to the control group, which was demonstrated by a significantly higher number and weight of feces following the procedure. Both Bifidobacterium and Lactobacillus genera were decreased after the procedure, which the authors felt as indicative of their potential role in POI. The fecal butyrate levels were also measured, as butyrate is involved in colonic transit, contractile responses, and toll like receptor stimulation (91). The fecal butyrate levels were decreased in all groups following the procedure, but the probiotic group had significantly higher levels of butyrate at 5 days post procedure. Further studies in additional animal models would be interesting to assess the potential of preoperative probiotic use in prevention of POI. While many surgeries are emergent, and do not provide a week prior for probiotic administration, recent studies in human patients undergoing gastrointestinal surgeries have shown promise that probiotic therapy initiated at surgery or up to 3 days after surgery are capable of significantly decreasing POI development (92, 93).
Within the enteric nervous system, the enteric glial cells play an increasingly appreciated direct role in regulating the intestinal barrier, and rapid induction of altered barrier function by activated glia could prove to be an important but poorly understood early contributor to POI induction. As previously discussed, it is hypothesized that tissue handling increases epithelial permeability, which allows the translocation of luminal contents, inciting and propriating an inflammatory response (43). Anup et al. (94) demonstrated in a rat model that surgical tissue handling of the SI rapidly increased conductance. Increased conductance was first noted at 30 min post manipulation, and this alteration to barrier function may be proven to occur in even shorter period of time. The inciting cause of this increased barrier function has yet to be determined. A 2020 study by Schneider et al. (95) suggested that purines like adenosine triphosphate released from tissue affected by surgical stress could activate enteric glia, which then play an important role in the inflammatory pathophysiology of POI. Glia are also reactive to mechanical stimulation, and therefore may be activated solely by tissue handling (96). Additional pathways inducing reactive glia include IL-1, S100β, glia endothelin-1, glia endothelin-B, and palmitoylethanolamide receptor (3, 97). Mice treated with anakinra, an IL-1 receptor antagonist, or antibodies against IL-1α or IL-1β had decreased POI development following intestinal manipulation (97). Activated glia produce IL-6, which is known to upregulate claudin 2, a potential method of increasing barrier permeability (97, 98). In addition, activated glia produce inflammatory products such as nitric oxide as well as pathologic levels of s-nitroglutathione, which have been shown to increase barrier permeability (96, 99, 100). If a specific pathway is identified, the prevention of glial activation may be effective in blocking the alteration in barrier function and significantly reduce the inflammatory component of POI development.
Discussion
In conclusion, POI is a multifactorial problem with a serious impact on human and equine health. Additional study of its neurogenic and inflammatory components will be critical in further development of successful methods of treatment and prevention. A better understanding of how elements like the microbiota suppressing inflammation or enteric glial cells driving early changes in barrier permeability could highlight novel targets for more effective interventions in patients at risk for POI.
Author Contributions
All authors listed have made a substantial, direct and intellectual contribution to the work, and approved it for publication.
Conflict of Interest
The authors declare that the research was conducted in the absence of any commercial or financial relationships that could be construed as a potential conflict of interest.
Publisher's Note
All claims expressed in this article are solely those of the authors and do not necessarily represent those of their affiliated organizations, or those of the publisher, the editors and the reviewers. Any product that may be evaluated in this article, or claim that may be made by its manufacturer, is not guaranteed or endorsed by the publisher.
References
2. Lisowski ZM, Pirie RS, Blikslager AT, Lefebvre D, Hume DA, Hudson NPH. An update on equine post-operative ileus: definitions, pathophysiology and management. Equine Vet J. (2018) 50:292–303. doi: 10.1111/evj.12801
3. Mazzotta E, Villalobos-Hernandez EC, Fiorda-Diaz J, Harzman A, Christofi FL. Postoperative ileus and postoperative gastrointestinal tract dysfunction: pathogenic mechanisms and novel treatment strategies beyond colorectal enhanced recovery after surgery protocols. Front Pharmacol. (2020) 11:583422. doi: 10.3389/fphar.2020.583422
4. Hedrick TL, McEvoy MD, Mythen MMG, Bergamaschi R, Gupta R, Holubar SD, et al. American society for enhanced recovery and perioperative quality initiative joint consensus statement on postoperative gastrointestinal dysfunction within an enhanced recovery pathway for elective colorectal surgery. Anesth Analg. (2018) 126:1896–907. doi: 10.1213/ANE.0000000000002742
5. Merritt AM, Blikslager AT. Post operative ileus: to be or not to be? Equine Vet J. (2008) 40:295–6. doi: 10.2746/042516408X302537
6. Vather R, Trivedi S, Bissett I. Defining POI: results of a systematic review and global survey. J Gastrointestinal Surg. (2013) 17:962–72. doi: 10.1007/s11605-013-2148-y
7. Venara A, Neunlist M, Slim K, Barbieux J, Colas PA, Hamy A, et al. POI: pathophysiology, incidence, and prevention. J Vic Surg. (2016) 153:439–46. doi: 10.1016/j.jviscsurg.2016.08.010
8. Goldstein JL, Matuszewski KA, Delaney C, Senagore A, Chiao EF, Shah M, et al. Inpatient economic burden of POI associated with abdominal surgery in the United States. Pharm Ther. (2007) 32:82–90. doi: 10.18553/jmcp.2009.15.6.485
9. Kronberg U, Kiran RP, Soliman MSM, Hammel JP, Galway U, Coffey JC, et al. A characterization of factors determining POI after laparoscopic colectomy enables the generation of a novel predictive score. Ann Surg. (2011) 253:78–81. doi: 10.1097/SLA.0b013e3181fcb83e
10. Millan M, Biondo S, Fraccalvieri D, Frago R, Golda T, Kreisler E. Risk factors for prolonged POI after colorectal cancer surgery. World J Surg. (2012) 36:179–85. doi: 10.1007/s00268-011-1339-5
11. Artinyan A, Nunoo-Mensah JW, Balasubramaniam S, Gauderman J, Essani R, Gonzalez-Ruiz C, et al. Prolonged POI: definition, risk factors, predictors after surgery. World J Surg. (2008) 32:1495–500. doi: 10.1007/s00268-008-9491-2
12. Vather R, Bisset IP. Risk factors for the development of prolonged post-operative ileus following elective colorectal surgery. Int J Colorectal Dis. (2013) 28:1385–91. doi: 10.1007/s00384-013-1704-y
13. Chapuis PH, Bokey L, Keshava A, Rickard MJ, Stewart P, Young CJ, et al. Risk factors for prolonged ileus after resection of colorectal cancer: an observational study of 2400 consecutive patients. Ann Surg. (2013) 257:909–15. doi: 10.1097/SLA.0b013e318268a693
14. Morton AJ, Blikslager AT. Surgical and postoperative factors influencing short-term survival of horses following small intestinal resection: 92 cases (1994-2001). Equine Vet J. (2002) 34:450–4. doi: 10.2746/042516402776117700
15. Curtis L, Burford JH, England GCW, Freeman SL. Risk factors for acute abdominal pain (colic) in the adult horse: a scoping review of risk factors, and a systematic review of the effect of management-related changes. PLoS ONE. (2019) 14:e0219307. doi: 10.1371/journal.pone.0219307
16. Kaneene JB, Miller R, Ross WA, Gallagher K, Marteniuk J, Rook J. Risk factors for colic in the Michigan (USA) equine population. Prev Vet Med. (1997) 30:23–36. doi: 10.1016/S0167-5877(96)01102-6
17. Tinker MK, White NA, Lessard P, Thatcher CD, Pelzer KD, Davis B, et al. Prospective study of equine colic incidence and mortality. Equine Vet J. (1997) 29:448–53. doi: 10.1111/j.2042-3306.1997.tb03157.x
18. Proudman CJ. A two-year, prospective survey of equine colic in general practice. Equine Vet J. (1992) 24:90–93. doi: 10.1111/j.2042-3306.1992.tb02789.x
19. Hudson JM, Cohen ND, Gibbs PG, Thompson JA. Feeding practices associated with colic in horses. J Am Vet Med Ass. (2001) 219:1419–25. doi: 10.2460/javma.2001.219.1419
20. Mair TS. BEVA evidence-based medicine colic survey. In: Abeyasekere LAFJ, editor. Proceedings British Equine Veterinary Association Congress. Newmarket, ON: Barr Equine Veterinary Journal Ltd (2004). p. 58–59.
21. Archer DC, Proudman CJ. Epidemiological clues to preventing colic. Vet J. (2006) 172:29–39. doi: 10.1016/j.tvjl.2005.04.002
22. Curtis L, Burford JH, Thomas JS, Curran ML, Bayes TC, England GC, Freeman SL. Prospective study of the primary evaluation of 1016 horses with clinical signs of abdominal pain by veterinary practitioners, and the differentiation of critical and non-critical cases. Acta Vet Scand. (2015) 57:69. doi: 10.1186/s13028-015-0160-9
23. Freeman DE. Fifty years of colic surgery. Equine Vet J. (2018) 50:423–35. doi: 10.1111/evj.12817
24. Vather R, O'Grady G, Bissett IP, Dinning PG. POI: mechanisms and future directions for research. Clin Exp Pharmacol Physiol. (2014) 41:358–70. doi: 10.1111/1440-1681.12220
25. Boeckxstaens GE, Hirsch DP, Kodde A, Moojen TM, Blackshaw A, Tytgat GN, et al. Activation of an adrenergic and vagally-mediated NANC pathway in surgery-induced fundic relaxation in the rat. Neurogastroenterol Mot. (1999) 11:467–74. doi: 10.1046/j.1365-2982.1999.00172.x
26. Holzer P Lippe IT Amann R. Participation of capsaicin-sensitive afferent neurons in gastric motor inhibition caused by laparotomy and intraperitoneal acid. Neuroscience. (1992) 48:715–22. doi: 10.1016/0306-4522(92)90414-W
27. Kahokeher A, Sammour T, Srinivasa S, Hill AG. Metabolic response to abdominal surgery: the 2-wound model. Surgery. (2011) 149:301–4. doi: 10.1016/j.surg.2010.10.020
28. Julius D, Basbaum AI. Molecular mechanisms of nociception. Nature. (2001) 413:203–10. doi: 10.1038/35093019
29. Fukuda H, Tsuchida D, Koda K, Miyazaki M, Pappas TN, Takahashi T. Inhibition of sympathetic pathways restores POI in the upper and lower gastrointestinal tract. J Gastroenterol Hepatol. (2007) 22:1293–9. doi: 10.1111/j.1440-1746.2007.04915.x
30. Lefebvre RA, Smits GJ, Timmermans JP. Study of NO and VIP as non-adrenergic non-cholinergic neurotransmitters in the pig gastric fundus. Br J Pharmacol. (1995) 116:2017–26. doi: 10.1111/j.1476-5381.1995.tb16406.x
31. DeWinter BY, Boeckxstaens GE, DeMan JG, Moreels TG, Herman AG, Pelckmans PA. Effect of adrenergic and nitrergic blockade on experimental ileus in rats. Br J Pharmacol. (1997) 120:464–8. doi: 10.1038/sj.bjp.0700913
32. Bayliss WM, Starling EH. The movements and innervation of the small intestine. J Physiol. (1899) 24:99–143. doi: 10.1113/jphysiol.1899.sp000752
33. Carli F, Trudel JL, Belliveau P. The effect of intraoperative thoracic epidural anesthesia and postoperative analgesia on bowel function after colorectal surgery: a prospective, randomized trial. Dis Colon Rectum. (2001) 44:1083–9. doi: 10.1007/BF02234626
34. Scheinin B, Asantila R, Orko R. The effect of bupivacaine and morphine on pain and bowel function after colonic surgery. Acta Anaesthesiol Scan. (2008) 31:161–4. doi: 10.1111/j.1399-6576.1987.tb02541.x
35. Ahn H, Bronge A, Johansson K, Ygge H, Lindhagen J. Effect of continuous postoperative epidural analgesia on intestinal motility. Br J Surg. (2005) 75:1176–8. doi: 10.1002/bjs.1800751210
36. Liu SS, Carpenter RL, Mackey DC, Thirlby RC, Rupp SM, Shine TS, et al. Effects of perioperative analgesic technique on rate of recovery after colon surgery. Anesthesiology. (1995) 83:757–65. doi: 10.1097/00000542-199510000-00015
37. Freeman DE. Is there still a place for lidocaine in the (postoperative) management of colics? Vet Clin North Am Equine Pract. (2019) 35:275–88. doi: 10.1016/j.cveq.2019.03.003
38. Farro G, Gomez-Pinilla PJ, Di Giovangiulio M, Stakenborg N, Auteri M, Thijs T, et al. Smooth muscle and neural dysfunction contribute to different phases of murine POI. Neurogastroenterol Motil. (2016) 28:934–47. doi: 10.1111/nmo.12796
39. Narayan P, Singh VK, Agarwal SS, Tandon R, Haq W, Raghubir R, et al. Immunomodulation by opioid peptidomimetic compound. Neuroimmunomodulation. (2001) 9:134–40. doi: 10.1159/000049017
40. Ferraz AA, Wanderley GJ, Santos MA Jr, Mathias CA, Araujo JG Jr, Ferraz EM. Effects of propranolol on human POI. Dig Surg. (2001) 18:305–10. doi: 10.1159/000050157
41. Kalff JC, Türler A, Schwarz NT, Schraut WH, Lee KK, Tweardy DJ, et al. Intra-abdominal activation of a local inflammatory response within the human muscularis externa during laparotomy. Ann Surg. (2003) 237:301. doi: 10.1097/01.SLA.0000055742.79045.7E
42. The FO Bennink RJ Ankum WM Buist MR Busch OR Gouma DJ. Intestinal handling induced mast cell activation and inflammation in human POI. Gut. (2008) 57:33–40. doi: 10.1136/gut.2007.120238
43. Kalff JC, Schraut WH, Simmons RL, Bauer AJ. Surgical manipulation of the gut elicits an intestinal muscularis inflammatory response resulting in postsurgical ileus. Ann Surg. (1998) 228:652–63. doi: 10.1097/00000658-199811000-00004
44. Kalff JC, Carlos TM, Schraut WH, Billiar TR, Simmons RL, Baeur AJ. Surgically induced leukocytic infiltrates within the rat intestinal muscularis mediate POI. Gastroenterology. (1999) 117:378–87. doi: 10.1053/gast.1999.0029900378
45. Schwarz NT, Kalff JC, Türler A, Engel BM, Watkins SC, Billiar TR, et al. Prostanoid production via COX-2 as a causative mechanism of rodent POI. Gastroenterology. (2001) 121:1354–71. doi: 10.1053/gast.2001.29605
46. Kapetanovic R, Fairbairn L, Beraldi D, Sester DP, Archibald AL, Tuggle CK, et al. Pig bone marrow-derived macrophages resemble human macrophages in their response to bacterial lipopolysaccharide. J Immunol. (2012) 188:3382–94. doi: 10.4049/jimmunol.1102649
47. Karagianni AE, Kapetanovic R, McGorum BC, Hume DA, Pirie SR. The equine alveolar macrophage: functional and phenotypic comparisons with peritoneal macrophages. Vet Immunol Immunopathol. (2013) 155:219–28. doi: 10.1016/j.vetimm.2013.07.003
48. Kalff JC, Eskandari MK, Hierholzer C, Schraut WH, Simmons RL, Baeur AJ. Biphasic response to gut manipulation and temporal correlation of cellular infiltrates and muscle dysfunction in rat. Surgery. (1999) 126:498–509. doi: 10.1016/S0039-6060(99)70091-7
49. de Jonge WJ The FO van der Coelen D Bennink RJ Reitsma PH van Deventer SJ. Mast cell degranulation during abdominal surgery initiates POI in mice. Gastroenterology. (2004) 127:535–45. doi: 10.1053/j.gastro.2004.04.017
50. Wehner S, Behrendt FF, Lyutenski BN, Lysson M, Bauer AJ, Hirner A, et al. Inhibition of macrophage function prevents intestinal inflammation and POI in rodents. Gut. (2007) 56:176–85. doi: 10.1136/gut.2005.089615
51. The FO de Jonge WJ Bennink RJ van den Wijngaard RM Boeckxstaens GE. The ICAM-1 antisense oligonucleotide ISIS-3082 prevents the development of POI in mice. Br J Pharmacol. (2005) 146:252–8. doi: 10.1038/sj.bjp.0706303
52. De Jonge WJ Van Den Wijngaard RM The FO Ter Beek ML Bennink RJ Tytgat GNJ. POI is maintained by intestinal immune infiltrates that activate inhibitory neural pathways in mice. Gastroenterol. (2003) 125:1137–47. doi: 10.1016/S0016-5085(03)01197-1
53. Kreiss C, Birder LA, Kiss S, VanBibber MM, Baeur AJ. COX-2 dependent inflammation increases spinal Fos expression during rodent POI. Gut. (2003) 52:527–34. doi: 10.1136/gut.52.4.527
54. Boeckxstaens GE, De Jonge WJ. Neuroimmune mechanisms in POI. Gut. (2009) 58:1300–11. doi: 10.1136/gut.2008.169250
55. Kalff JC, Schraut WH, Billiar TR, Simmons RL, Bauer AJ. Role of inducible nitric oxide synthase in postoperative intestinal smooth muscle dysfunction in rodents. Gastroenterology. (2000) 118:316–27. doi: 10.1016/S0016-5085(00)70214-9
56. Holte K, Sharrock N, Kehle H. Pathophysiology and clinical implications of perioperative fluid excess. Br J Anaesth. (2002) 89:622–32. doi: 10.1093/bja/aef220
57. Engel DR, Koscielny A, Wehner S, Maurer J, Schiwon M, Franken L, et al. T helper type 1 memory cells disseminate POI over the entire intestinal tract. Nat Med. (2010) 16:1407–13. doi: 10.1038/nm.2255
58. Stoffels B, Schmidt J, Nakao A, Nazir A, Chanthaphavong RS, et al. Role of interleukin 10 in murine postoperative ileus. Gut. (2009) 58:648–60. doi: 10.1136/gut.2008.153288
59. Farro G, Stakenborg M, Gomez-Pinilla PJ, Labeeuw E, Goverse G, Giovangiulio MD, et al. CCR2-dependent monocyte-derived macrophages resolve inflammation and restore gut motility in POI. Gut. (2017) 66:2098–109. doi: 10.1136/gutjnl-2016-313144
60. Van Bree SHW, Gomez-Pinilla PJ, van de Bovenkamp FS, Di Giovangiulio M, Farro G, Nemethova A, et al. Inhibition of spleen tyrosine kinase as a treatment of POI. Gut. (2013) 62:1581–90. doi: 10.1136/gutjnl-2012-302615
61. De Jonge WJ, van der Zanden EP. Stimulation of the vagus nerve attenuates macrophage activation by activating the Jak2-STAT3 signaling pathway. Nat Immunol. (2005) 6:844–51. doi: 10.1038/ni1229
62. The FO Buist MR Lei A Bennink RJ Hofland J van den Wijngaard RM. The role of mast cell stabilization in treatment of POI: a pilot study. Am J Gastroenterol. (2009) 104:2257–66. doi: 10.1038/ajg.2009.268
63. Hopster-Iversen CC, Hopster K, Staszyk C, Rohn K, Freeman DE, Rotting, et al. Effects of experimental mechanical manipulations on local inflammation in the jejunum of horses. Am J Vet Res. (2014) 75:385–91. doi: 10.2460/ajvr.75.4.385
64. van Bree SH, Nemethova A, van Bovenkamp FS, Gomez-Pinilla P, Elbers L, DiGiovangiulio M, et al. Novel method for studying POI in mice. Int J Physiol, Pathophysiol Pharmacol. (2012) 4:219–27.
65. Türler A, Schnurr C, Nakao A, Tögel S, Moore BA, Murase N, et al. Endogenous endotoxin participates in causing a panenteric inflammatory ileus after colonic surgery. Ann Surg. (2007) 245:734. doi: 10.1097/01.sla.0000255595.98041.6b
66. Echtenacher B, Männel DN, Hültner L. Critical protective role of mast cells in a model of acute septic peritonitis. Nature. (1996) 381:75–7. doi: 10.1038/381075a0
67. Bauer AJ, Boeckxstaens GE. Mechanisms of POI. Neurogastroenterol Motil. (2004) 16(Suppl. 2):54–60. doi: 10.1111/j.1743-3150.2004.00558.x
68. Vaughan-Shaw P, Fecher I, Harris S, Knight J. A meta-analysis of the effectiveness of the opioid receptor antagonist alvimopan in reducing hospital length of stay and time to GI recovery in patients enrolled in a standardized accelerated recovery program after abdominal surgery. Dis Colon Rectum. (2012) 55:611–20. doi: 10.1097/DCR.0b013e318249fc78
69. Lefebvre D, Hudson NP, Elce YA, Blikslager A, Divers TJ, Handel IG, et al. Clinical features and management of equine post operative ileus (POI):survey of diplomates of the american colleges of veterinary internal medicine (ACVIM),veterinary surgeons (ACVS) and veterinary emergency and critical care (ACVECC). Equine Vet J. (2016) 48:714–9. doi: 10.1111/evj.12520
70. Lefebvre D, Pirie RS, Handel IG, Tremaine WH, Hudson NP. Clinical features and management of equine post operative ileus: Survey of diplomates of the European colleges of equine internal medicine (ECEIM) and veterinary surgeons (ECVS). Equine Vet J. (2016) 48:182–7. doi: 10.1111/evj.12355
71. Gustafsson UO, Hausel J, Thorell A, Ljungqvist O, Soop M, Nygren J. Enhanced recovery after surgery study group. Adherence to the enhanced recovery after surgery protocol and outcomes after colorectal cancer surgery. Arch Surg. (2011) 146:571–7. doi: 10.1001/archsurg.2010.309
72. Brandstrup B, Tonnesen H, Beier-Holgersen R, Hjortso E, Ording H, Lindorff-Larsen K, et al. Effects of intravenous fluid restriction on postoperative complications: comparison of two perioperative fluid regimens: a randomized assessor-blinded multicenter trial. Ann Surg. (2003) 238:641–8. doi: 10.1097/01.sla.0000094387.50865.23
73. Milne TGE, Jaunge R, O'Grady G, Bisset IP. Nonsteroidal anti-inflammatory drugs reduce the time to recovery of gut function after elective colorectal surgery: a systematic review and meta-analysis. Colorectal Dis. (2018) 20:190–8. doi: 10.1111/codi.14268
74. Naylor RJ, Taylor AH, Knowles EJ, Wilford S, Linnenkohl W, Mair TS, et al. Comparison of flunixin meglumine and meloxicam for post operative management of horses with strangulating small intestinal lesions. Equine Vet J. (2014) 46:427–34. doi: 10.1111/evj.12224
75. Van Hoogmoed LM, Nieto JE, Snyder JR, Harmon FA. Survey of prokinetic use in horses with gastrointestinal injury. Vet Surg. (2004) 33:279–85. doi: 10.1111/j.1532-950X.2004.04041.x
76. Salem SE, Proudman CJ, Archer DC. Has intravenous lidocaine improved the outcome in horses following surgical management of small intestinal lesions in a UK hospital population? BMC Vet Res. (2016) 12:157. doi: 10.1186/s12917-016-0784-7
77. Helman C.A. Chewing gum is as effective as food in stimulating cephalic phase gastric secretion. Am J Gastroenterol. (1988) 83:640–2.
78. Freeman DE, Hammock P, Baker GJ, Goetz T, Foreman JH, Schaeffer DJ, et al. Short- and long-term survival and prevalence of POI after small intestinal surgery in the horse. Equine Vet J. (2000) 32(Suppl. 32):42–51. doi: 10.1111/j.2042-3306.2000.tb05333.x
79. Wehner S, Straesser S, Vilz TO, Pantelis D, Sielecki T, de la Cruz VF, et al. Inhibition of p38 mitogen-activated protein kinase pathway as prophylaxis of POI in mice. Gastroenterol. (2009) 136:619–29. doi: 10.1053/j.gastro.2008.10.017
80. Vilz TO, Sommer N, Kahl P, Pantelis D, Kalff JC, Wehner S. Oral CPSI-2364 treatment prevents POI in swine without impairment of anastomotic healing. Cell Physiol Biochem. (2013) 32:1362–73. doi: 10.1159/000356575
81. Borovikova LV, Ivanova S, Zhang M, Yang H, Botchkina GI, Watkins LR, et al. Vagus nerve stimulation attenuates the systemic inflammatory response to endotoxin. Nature. (2000) 405:458–62. doi: 10.1038/35013070
82. Matteoli G, Gomez-Pinilla PJ, Nemethova A, Di Giovangiulio M, Cailotto C, van Bree SH, et al. A distinct vagal anti-inflammatory pathway modulates intestinal muscularis resident macrophages independent of the spleen. Gut. (2014) 63:938–48. doi: 10.1136/gutjnl-2013-304676
83. The FO Boeckxstaens GE Snoek SA Cash JL Bennink R Larosa GJ. Activation of the cholinergic anti-inflammatory pathway ameliorates POI in mice. Gastroenterology. (2007) 133:1219–28. doi: 10.1053/j.gastro.2007.07.022
84. Wolthuis AM, Stakenborg N, D'Hoore A, Boeckxstaens GE. The pig as a preclinical model for laparoscopic vagus nerve stimulation. Int J Colorectal Dis. (2016) 31:211–5. doi: 10.1007/s00384-015-2435-z
85. Shogan BD, Smith DP, Christley S, Gilbert JA, Zaborina O, Alverdy JC. Intestinal anastomotic injury alters spatially defined microbiome composition and function. Microbiome. (2014) 2:35. doi: 10.1186/2049-2618-2-35
86. Sommovilla J, Zhou Y, Sun RC, Choi PM, Diaz-Miron J, Shaikh NN, et al. Small bowel resection induces long-term changes in the enteric microbiota of mice. J Gastrointest Surg. (2015) 19:56–64; discussion 64. doi: 10.1007/s11605-014-2631-0
87. Liu Z, Qin H, Yang Z, Xia Y, Liu W, Yang J, et al. Randomised clinical trial: the effects of perioperative probiotic treatment on barrier function and post-operative infectious complications in colorectal cancer surgery—a double-blind study. Aliment Pharmacol Ther. (2011) 33:50–63. doi: 10.1111/j.1365-2036.2010.04492.x
88. Sugawara G, Nagino M, Nishio H, Ebata T, Takagi K, Ashara T, et al. Perioperative synbiotic treatment to prevent postoperative infectious complications in biliary cancer surgery: a randomized controlled trial. Ann Surg. (2006) 244:706–14. doi: 10.1097/01.sla.0000219039.20924.88
89. Fukuda S, Toh H, Hase K, Oshima K, Nakanishi Y, Yoshimura K, et al. Bifidobacteria can protect from enteropathogenic infection through production of acetate. Nature. (2011) 469:543–7. doi: 10.1038/nature09646
90. Shin SY, Hussain Z, Lee YJ, Park H. An altered composition of fecal microbiota, organic acids, and the effect of probiotics in the guinea pig model of POI. Neurogastroenterol Motil. (2020) 19:e13966. doi: 10.1111/nmo.13966
91. Soret R, Chevalier J, De Coppet P, Poupeau G, Derkinderen P, Segain JP, et al. Short-chain fatty acids regulate the enteric neurons and control gastrointestinal motility in rats. Gastroenterolgoy. (2010) 138:1172–82. doi: 10.1053/j.gastro.2010.01.053
92. Folwarski M, Dobosz M, Małgorzewicz S, Skonieczna-Zydecka K, Kazmierczak-Siedlecka K. Effects of Lactobacillus rhamnosus GG on early postoperative outcome after pylorus-preserving pancreatoduodenectomy: a randomized trial. Eur Rev Med Pharmacol Sci. (2021) 25:397–405. doi: 10.26355/eurrev_202101_24407
93. Bajramagic S, Hodzic E, Mulabdic A, Holjan S, Smajlovic SV, Rovcanin A. Usage of probiotics and its clinical significance at surgically treated patients sufferig from colorectal carcinoma. Med Arch. (2019) 73:316–20. doi: 10.5455/medarh.2019.73.316-320
94. Anup R, Aparna V, Pulimood A, Balasubramanian KA. Surgical stress and the small intestine: role of oxygen free radicals. Surgery. (1999) 125:560–9. doi: 10.1016/S0039-6060(99)70209-6
95. Schneider R, Leven P, Glowka T, Kuzmannov I, Lysson M, Svhneiker B, et al. A novel P2X2-dependent purinergic mechanism of enteric gliosis in intestinal inflammation. EMBO Mol Med. (2020) 13:e12724. doi: 10.15252/emmm.202012724
96. Ochoa-Cortes F, Turco F, Linan-Rico A, Soghomonyan S, Whitaker E, Wehner S, et al. Enteric glial cells: a new frontier in neurogastroenterology and clinical target for inflammatory bowel diseases. Inflamm Bowel Dis. (2016) 22:433–49. doi: 10.1097/MIB.0000000000000667
97. Stoffels B, Hupa KJ, Snoek SA, van Bree S, Stein K, Schwandt T, et al. POI involves interleukin-1 receptor signaling in enteric glia. Gastroenterology. (2014) 146:176–87. doi: 10.1053/j.gastro.2013.09.030
98. Al-Sadi R, Ye D, Boivin M, Guo S, Hashimi M, Ereifej L, et al. Interleukin-6 modulation of intestinal epithealial tight junction permeability is mediated by JNK pathway activation of claudin-2 gene. PLoS ONE. (2014) 9:e85345. doi: 10.1371/journal.pone.0085345
99. Savidge TC, Newman P, Pothoulakis C, Ruhl A, Neunlist M, Bourreille A, et al. Enteric glia regulate intestinal barrier function and inflammation via release of S-nitrosoglutathione. Gastroenterology. (2007) 132:1344–58. doi: 10.1053/j.gastro.2007.01.051
Keywords: intestine, equine, surgery, barrier function, enteric glia and neurons, microbiota
Citation: Hellstrom EA, Ziegler AL and Blikslager AT (2021) Postoperative Ileus: Comparative Pathophysiology and Future Therapies. Front. Vet. Sci. 8:714800. doi: 10.3389/fvets.2021.714800
Received: 25 May 2021; Accepted: 19 August 2021;
Published: 13 September 2021.
Edited by:
Micaela Sgorbini, University of Pisa, ItalyReviewed by:
Brina Lopez, Midwestern University, United StatesShebl E. Salem, Zagazig University, Egypt
Copyright © 2021 Hellstrom, Ziegler and Blikslager. This is an open-access article distributed under the terms of the Creative Commons Attribution License (CC BY). The use, distribution or reproduction in other forums is permitted, provided the original author(s) and the copyright owner(s) are credited and that the original publication in this journal is cited, in accordance with accepted academic practice. No use, distribution or reproduction is permitted which does not comply with these terms.
*Correspondence: Anthony T. Blikslager, anthony_blikslager@ncsu.edu