- PetDx, La Jolla, CA, United States
This proof-of-concept study demonstrates that blood-based liquid biopsy using next generation sequencing of cell-free DNA can non-invasively detect multiple classes of genomic alterations in dogs with cancer, including alterations that originate from spatially separated tumor sites. Eleven dogs with a variety of confirmed cancer diagnoses (including localized and disseminated disease) who were scheduled for surgical resection, and five presumably cancer-free dogs, were enrolled. Blood was collected from each subject, and multiple spatially separated tumor tissue samples were collected during surgery from 9 of the cancer subjects. All samples were analyzed using an advanced prototype of a novel liquid biopsy test designed to non-invasively interrogate multiple classes of genomic alterations for the detection, characterization, and management of cancer in dogs. In five of the nine cancer patients with matched tumor and plasma samples, pre-surgical liquid biopsy testing identified genomic alterations, including single nucleotide variants and copy number variants, that matched alterations independently detected in corresponding tumor tissue samples. Importantly, the pre-surgical liquid biopsy test detected alterations observed in spatially separated tissue samples from the same subject, demonstrating the potential of blood-based testing for comprehensive genomic profiling of heterogeneous tumors. Among the three patients with post-surgical blood samples, genomic alterations remained detectable in one patient with incomplete tumor resection, suggesting utility for non-invasive detection of minimal residual disease following curative-intent treatment. Liquid biopsy allows for non-invasive profiling of cancer-associated genomic alterations with a simple blood draw and has potential to overcome the limitations of tissue-based testing posed by tissue-level genomic heterogeneity.
Introduction
Non-invasive blood-based genomic profiling, often called liquid biopsy, is increasingly employed in human medicine to support cancer diagnosis and management (1, 2). It refers to the measurement of circulating biomarkers present in blood that can be used to study the genomic profiles of underlying conditions (e.g., cancer). The most commonly used form of liquid biopsy is based on analysis of cell-free DNA (cfDNA) in plasma; cfDNA is comprised of DNA fragments released by cells into the circulation through secretion, apoptosis, or necrosis (3), including cfDNA derived from tumor cells (which is known as circulating tumor DNA or ctDNA). It has been demonstrated in studies of human cancer that plasma cfDNA analysis can recapitulate the genomic profile of the underlying tumor tissue (4, 5). Additionally, it offers distinct advantages through its ability to capture the diversity of mutations derived from spatially separated sites within a primary tumor or across metastatic deposits (a fundamental biological feature of cancer, referred to as “tumor heterogeneity”) that may be missed by testing a single tissue sample from a single tumor site (6–8). Liquid biopsy enables genomic profiling of cancer cases that are difficult to biopsy, and its non-invasive nature allows it to be performed safely and repeatedly for disease monitoring. Liquid biopsy has also shown considerable potential as a multi-cancer early detection test that can be used at regular intervals (e.g., annually) for cancer screening in high-risk populations, where detection of the disease at earlier stages can result in improved clinical outcomes (9–12).
A number of studies have demonstrated the presence of ctDNA in canine plasma across multiple cancer types (13–16). The general strategy for detecting tumor-specific cfDNA relies on identifying genomic alterations specific to cancer cells and absent in healthy cells (17). Different cancer types in humans are driven by different classes of genomic alterations (18), a phenomenon that is encountered in canine cancers as well: for instance, single nucleotide variants (e.g., in the KIT gene) are frequently found in mast cell tumors (19) whereas copy number variants (gains and losses) are often encountered in canine sarcomas (20).
Here, we report results generated using an advanced prototype of a novel blood-based liquid biopsy assay (hereinafter “the test”) specifically developed for the non-invasive detection, characterization, and management of cancer in dogs. This is the first report of a next-generation sequencing (NGS) based multi-cancer early detection test designed to simultaneously interrogate multiple classes of cancer-associated genomic alterations, including single nucleotide variants (SNVs), insertions and deletions (indels), translocations, and copy number variants (CNVs), in cfDNA extracted from canine blood samples. This proof-of-concept study aims to demonstrate the potential of liquid biopsy to broadly profile tumor-derived genomic alterations, and to capture the genomic heterogeneity of multiple, spatially separated tumor clones (within the same primary site or across different metastatic sites) that would be missed by traditional single-site tissue biopsy, suggesting broad utility as a diagnostic tool in veterinary oncology.
Methods
Eleven client-owned dogs with a variety of confirmed cancer diagnoses (including localized/regional as well as disseminated/metastatic disease) that were scheduled for surgical resection, and five dogs presumed to be cancer-free (no history of cancer and no clinical signs indicative of cancer at the time of enrollment), were enrolled across multiple care settings. All cancer subjects were enrolled under a dedicated protocol that was independently approved by the institutional animal care and use committee (IACUC) or internal review board at two veterinary centers, and all cancer-free subjects were enrolled under a separate dedicated protocol that was independently approved by the institutional clinical review board at one veterinary center; the protocols were also approved by institutional clinical review boards at the other participating sites, based on each site's requirements. All subjects were client-owned, and written informed consent was obtained from all owners.
From each patient, a baseline blood sample was collected at the time of enrollment. In 9 of the 11 patients with cancer, samples of resected tumor specimens from one or more sites within each primary tumor, and from one or more metastatic sites if available, were collected during the subsequent surgery. In three cancer patients, a post-surgical blood sample was collected 10, 15, and 189 days after surgery, respectively. Clinical and demographic data, plasma volumes, and cfDNA yields for all enrolled subjects are summarized in Table 1.
Blood samples were processed with a double-centrifugation protocol to separate plasma from white blood cells (WBCs) (21–23). CfDNA was extracted from plasma using a proprietary bead-based chemistry protocol optimized to maximize cfDNA yield in canine subjects. DNA from WBC and tumor tissue samples was extracted using QIAamp DNA Mini Blood Kit (Qiagen). Sequencing libraries were generated from DNA extracted from matched tumor (if available), plasma, and WBC samples for each subject. Libraries were prepared by incorporating universal adapters and barcodes into sample DNA via ligation and universal PCR amplification. Amplified libraries were subjected to genome-wide sequencing for CNV analysis. In parallel, the libraries underwent enrichment with a proprietary method targeting selected regions of the canine genome for SNV analysis. All libraries were sequenced using an Illumina NovaSeq 6000.
All sequencing reads were aligned to the CanFam3.1 reference genome (24), and somatic variant calling was performed with a custom bioinformatics pipeline. For subjects who had only a baseline plasma sample available for testing, a proprietary algorithm to detect ctDNA based on fragment length profiles was also applied.
Results
In the nine cancer patients that had tumor tissues analyzed, all nine had somatic alterations detected in tissue, based on aggregate variant calls across all available tissue samples (Supplementary Table 1). In 5 of these subjects, a subset of these variants was also independently detected in the matched pre-operative plasma sample. In two cancer patients without a tumor tissue sample available for analysis, both had somatic alterations detected in their respective pre-operative plasma sample, and both were also classified as cancer-positive by fragment length profiling. Among the seven plasma-positive cancer subjects, two had disseminated/metastatic disease and five had localized/regional disease (one with adjacent lymph node involvement and four without lymph node involvement). All four remaining cancer subjects who had no variants detected in pre-operative plasma had localized disease (two with lymph node involvement and two without lymph node involvement). No SNV or CNV alterations were detected in plasma samples from any of the five presumably cancer-free dogs, and all were also classified as cancer-negative by fragment length profiling. Full subject-level results are summarized in Supplementary Table 1.
The test detected genomic alterations in genes such as TP53, KRAS, EGFR, and PIK3CA that are frequently associated with cancer in both humans and dogs (25, 26). Homologs of some of these alterations are clinically actionable in humans; for example, both the plasma and the tissue of a cholangiocarcinoma patient (PT02) featured an EGFR p.L805R mutation, which is homologous to the human EGFR p.L858R mutation, a common target for EGFR tyrosine kinase inhibitors (27). We also detected mutations in genes that are more specifically associated with canine cancer, such as PTPN11, PDGFRA, and PDGFRB; in particular, the PDGFR protein is a target for the multikinase inhibitor toceranib phosphate (Palladia™) (28, 29), which is commonly used in the treatment of canine cancers.
Genomic profiling of spatially separated tumor tissue samples from the same subject demonstrated genomic heterogeneity across sites within the primary mass as well as across distant sites, in keeping with the polyclonal nature of cancer (17); and these different genomic alterations were collectively captured in plasma. For example, in PT04 (diagnosed with anal sac adenocarcinoma with lymph node metastases), tissue samples were collected from two different locations within the left anal sac primary tumor, and two different locations within the left sub-lumbar lymph node. Four CNVs (on chromosomes 9, 13, 18, 22) are shared across all four samples, while other CNVs are sample-specific: e.g., the CNVs on chromosomes 4 and 27 are specific to the two lymph node samples, while the CNV on chromosome 19 is specific to the two primary tumor samples. The corresponding pre-operative plasma captured most of this heterogeneity, with both shared and private (site-specific) CNV alterations from the various tumor samples reflected in cfDNA (Figure 1).
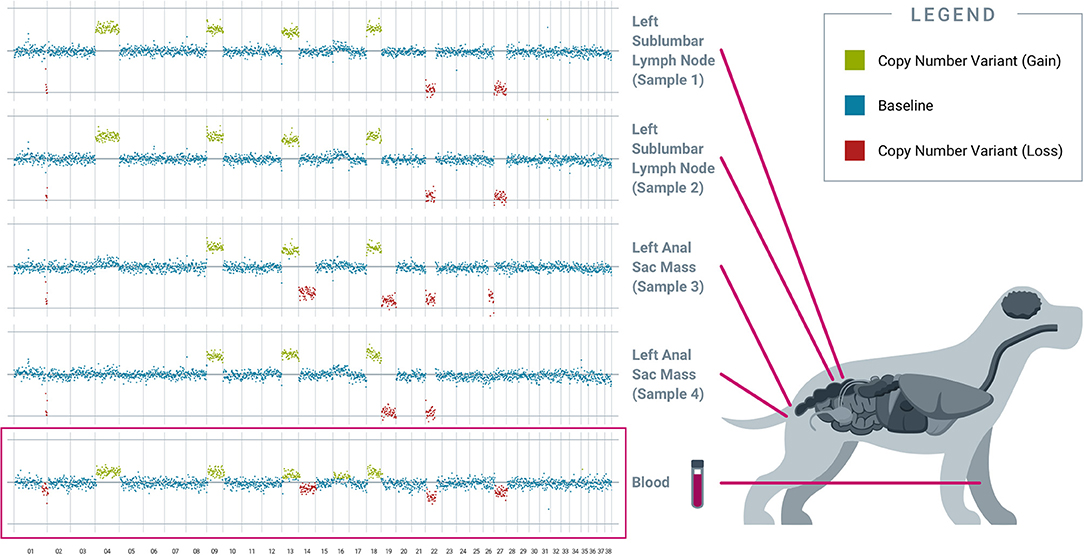
Figure 1. Individual tissue samples reveal distinct somatic copy number alterations, collectively captured in the blood-derived pre-operative plasma. Results from PT04, an 11-year-old female spayed Border Collie diagnosed with apocrine gland anal sac adenocarcinoma of the left anal sac with metastasis to a left sublumbar lymph node, are shown. Four different tumor samples were collected from spatially separated locations at two sites: the left sublumbar lymph node (two samples) and the left anal sac mass (two samples). Copy number variation analysis revealed both inter-site and intra-site tumor heterogeneity. Plasma cfDNA captured shared and private alterations from both tumor sites. All chromosomes shown were interrogated for cancer-associated genomic alterations by the test.
Apart from capturing tumor heterogeneity, the test was also able to identify residual disease in plasma after incomplete surgical resection. PT03 was simultaneously diagnosed with multiple primary cancers, including metastatic pancreatic carcinoma, hemangiosarcoma of the liver and spleen, and hepatocellular carcinoma; at surgery, five tissue samples representing primary and metastatic tumor deposits were obtained for testing. Four somatic alterations, including one SNV in NRAS, one SNV in KRAS, and two distinct SNVs in TP53, were identified across four of the five tissue samples (Figure 2), and multiple CNV alterations were also identified in most of the tissue samples. Three of the four SNVs were also detected in the pre-operative plasma sample, along with the majority of the CNV alterations. Complete histologic resection of the hepatic hemangiosarcoma, splenic hemangiosarcoma, and hepatocellular carcinoma were achieved; however, the metastatic pancreatic carcinoma was incompletely excised. This was consistent with the observation of persistent CNV and SNV alterations in the post-operative plasma sample, confirming the presence of residual disease (Figure 3A). For comparison, curative-intent resection of a cystic renal carcinoma in PT01 was complete, and the post-operative plasma sample showed no evidence of any of the somatic alterations that had been present in the pre-operative plasma or the resected tumor (Figure 3B).
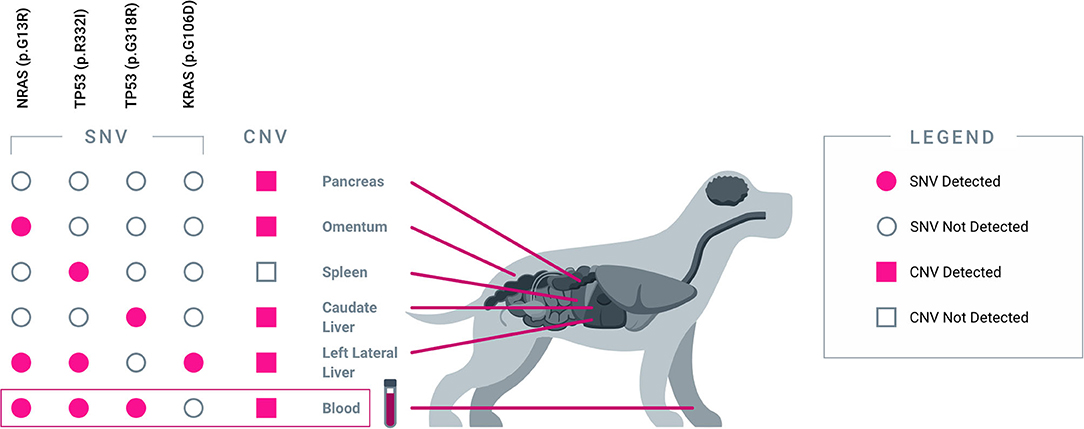
Figure 2. Blood-derived plasma cfDNA captures heterogeneous alterations from five spatially separated sites across multiple organs. Results from PT03, a 14-year-old female spayed mixed breed dog diagnosed with multiple cancers are shown. Five tissue samples were collected from five distinct tumor sites: pancreas (pancreatic carcinoma), omentum (metastatic pancreatic carcinoma), spleen (hemangiosarcoma), caudate liver (metastatic hemangiosarcoma), and left lateral liver (hepatocellular carcinoma). Each tumor sample revealed a unique combination of genomic alterations (SNVs and/or CNVs), some of which were shared across multiple sites. The majority of SNV and CNV alterations were collectively captured in pre-operative plasma cfDNA.
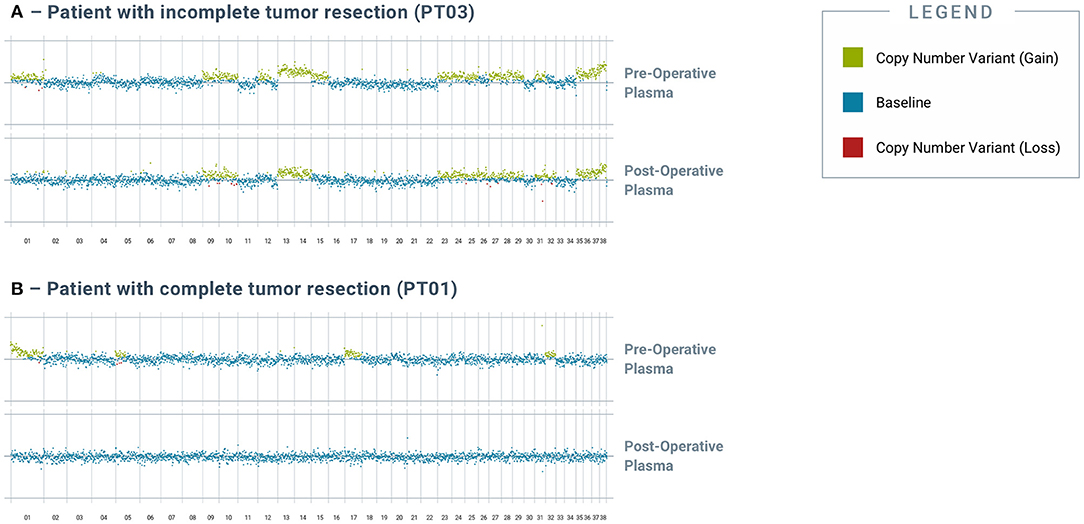
Figure 3. Analysis of post-operative blood-derived plasma cfDNA reveals residual genomic alterations after incomplete resection of tumor. Genomic alterations were detected in post-op blood samples from tumors with incomplete tumor resection but not in those with complete resection. (A) depicts pre- and post-operative plasma CNV traces from PT03, a 14-year-old female spayed mixed breed dog that had incompletely excised metastatic pancreatic carcinoma. (B) depicts pre- and post-operative plasma CNV traces from PT01, a 12-year-old male intact West Highland White Terrier that had completely excised cystic renal carcinoma. All chromosomes shown were interrogated for cancer-associated genomic alterations by the test.
Discussion
This proof-of-concept study is the first to demonstrate the feasibility of using blood-based liquid biopsy to non-invasively detect multiple classes of genomic alterations in dogs with cancer, in both localized and metastatic settings. An advanced prototype of a novel liquid biopsy test, designed to interrogate multiple classes of cancer-associated genomic alterations across the canine genome, revealed alterations in plasma that were shared between different tumor sites as well as alterations that were private to individual tumor sites within a subject; these observations highlight the potential of plasma cfDNA analysis by NGS to capture the genomic heterogeneity of spatially separated tumors within individual canine cancer patients.
Importantly, among the seven patients for whom cancer was detected from blood, three had only one class of genomic alteration (either SNV or CNV) detected, suggesting that profiling multiple classes of genomic alteration improves overall detection of tumor-derived cfDNA compared to relying on just one class. Among patients for whom cancer was not detected in blood, all had localized disease; these results are consistent with the observation in humans that the proportion of patients with detectable tumor-derived cfDNA in plasma is higher in metastatic disease compared to localized disease (30). Larger studies are needed to confirm these observations in dogs.
Furthermore, our results suggest utility for liquid biopsy in detecting minimal residual disease (MRD) following curative-intent surgery. After a complete resection, tumor-specific genomic alterations should no longer be present in plasma cfDNA, whereas such alterations would persist—potentially at lower levels—following an incomplete resection.
The ability of liquid biopsy to detect genomic alterations originating from one or multiple tumor sites within a patient offers several advantages compared to traditional tissue-based testing. First, it is non-invasive and thus poses a lower risk to patients compared to biopsy or surgery. Second, it allows for a more comprehensive characterization of the full genomic landscape of the patient's malignancy, across multiple sites within the primary tumor and across metastatic deposits. This tissue-level heterogeneity, which reflects the polyclonal nature of most clinical cancers, would be otherwise difficult to evaluate in dogs due to the morbidity and expense associated with obtaining multiple tissue samples from the primary as well as metastatic sites. Furthermore, obtaining multiple tissue samples is generally not performed in dogs with gross metastatic disease, as aggressive therapy is not typically pursued in these individuals, and the invasiveness of collecting samples from metastatic lesions would cause an unacceptable level of morbidity in a patient with advanced cancer (31). A blood test to characterize and monitor malignant disease, regardless of its location(s) within the body, is less burdensome and facilitates serial testing for longitudinal monitoring of the disease.
Liquid biopsy testing can support multiple applications across the continuum of canine cancer care, including: screening (for early detection) in high-risk populations, aid in diagnosis, detection of MRD, selection of targeted therapies, and monitoring for cancer recurrence or for treatment response by serial testing; and promises to bring the power of precision oncology to veterinary practice through a simple blood draw that does not require changes to the clinical routine (17). Finally, a liquid biopsy test covering cancer-associated regions of the genome that have high homology between dogs and humans can enable identification of somatic alterations in dogs that have clinically actionable human homologs. Such insights gained from liquid biopsy based genomic profiling could be used to speed the adoption of targeted human cancer therapeutics for the treatment of canine cancer.
Widespread access to multi-cancer early detection testing would be highly beneficial for canine patients, given that cancer is by far the leading cause of death in dogs (32). The current results demonstrate the value of interrogating multiple classes of genomic alterations simultaneously in order to improve sensitivity for detection of cancer. Furthermore, to our knowledge these results are the first to suggest that fragment length profiling may provide utility for cancer detection in dogs. It is important to note that an advanced prototype version of the test was employed in the current study, and further validation in larger cohorts of cancer-diagnosed and cancer-free subjects will be required to fully characterize the analytical performance as well as the clinical sensitivity and specificity of the test.
Conclusion
Blood-based liquid biopsy testing—first used for non-invasive prenatal testing (33) and more recently for cancer detection and management—is becoming increasingly common in human medicine. Historically, successful advances in human medicine have been quickly adopted for veterinary use after demonstration of utility in companion animals. This study represents the first-ever application of liquid biopsy to simultaneously profile multiple classes of genomic alterations using next-generation sequencing for the non-invasive detection and characterization of cancer in dogs, an important first step toward demonstrating such utility. Additionally, we demonstrate that canine cancers, like human cancers, exhibit significant intra-patient genomic heterogeneity at the tissue level. The sampling challenges imposed by this heterogeneity can be significantly mitigated by a non-invasive liquid biopsy testing approach, which provides a comprehensive, systemic view of the genomic landscape of malignant lesions throughout the body, and which can be easily incorporated into the current clinical routine. Finally, we demonstrate the potential utility of the test for detecting MRD by showing that residual somatic genomic alterations in plasma post-surgery correlate with incomplete surgical resection. Taken together, the findings of this study demonstrate that non-invasive liquid biopsy testing has the potential to support multiple use cases across the continuum of cancer care, for the benefit of canine patients.
Data Availability Statement
Restrictions apply to the datasets: The datasets presented in this article are not readily available because they contain proprietary information. Requests to access the datasets should be directed to c2NpZW5jZSYjeDAwMDQwO3BldGR4LmNvbQ==.
Ethics Statement
All cancer-diagnosed subjects were enrolled under a dedicated protocol, which was independently reviewed and approved by the institutional animal care and use committees (IACUCs) at the University of Guelph Ontario Veterinary College and the University of Minnesota Veterinary Medical Center, as well as by institutional clinical review boards at additional sites based on each study site's requirements; all cancer-free subjects were enrolled under a separate dedicated protocol, which was independently approved by the Veterinary Centers for America (VCA) Clinical Studies Institutional Review Board, as well as by institutional clinical review boards at additional sites based on each study site's requirements. Written informed consent was obtained from the owners for the participation of their animals in this study.
Author Contributions
KK, JC, DG, and AF contributed to the conception and design of the study. LM, RM-P, LH, and AF managed the logistics of patient recruitment and sample collections. KK, PN, GH, DF, JT, IC, and DT generated the data and performed data analysis. KK and DT wrote the first draft of the manuscript. All authors contributed to manuscript revisions and approved the submitted version.
Funding
This study received funding from PetDx. The funder had the following involvement with the study: study design, data collection and analysis, decision to publish, and preparation of the manuscript.
Conflict of Interest
All authors are employed by or affiliated with PetDx; and receive compensation from PetDx and/or hold vested or unvested equity in PetDx.
Acknowledgments
The authors thank Jason Loftis for assistance with developing the illustrations in this manuscript, Sara Pracht, Sofya Gefter, Daisy Peji, Alma D. Galicia Ortiz, Natasha Shoemaker, Mary Tefend, and Drs. Amber Wolf-Ringwall, Terry Clark, Erin Portillo, K. Scott Griffin, Chelsea Tripp and the doctors and staff at Bridge Animal Referral Centre, and the team at the Mona Campbell Centre for Animal Cancer, OVC, and the Institute for Comparative Cancer Investigation, University of Guelph for sample collections.
Supplementary Material
The Supplementary Material for this article can be found online at: https://www.frontiersin.org/articles/10.3389/fvets.2021.704835/full#supplementary-material
References
1. Husain H, Velculescu VE. Cancer DNA in the circulation: the liquid biopsy. JAMA. (2017) 318:1272–4. doi: 10.1001/jama.2017.12131
2. Ignatiadis M, Sledge GW, Jeffrey SS. Liquid biopsy enters the clinic — implementation issues and future challenges. Nat Rev Clin Oncol. (2021) 18:297–312. doi: 10.1038/s41571-020-00457-x
3. Diaz LA, Bardelli A. Liquid biopsies: genotyping circulating tumor DNA. J Clin Oncol. (2014) 32:579–86. doi: 10.1200/JCO.2012.45.2011
4. Jahangiri L, Hurst T. Assessing the concordance of genomic alterations between circulating-free DNA and tumour tissue in cancer patients. Cancers. (2019) 11:1938. doi: 10.3390/cancers11121938
5. Chae YK, Davis AA, Carneiro BA, Chandra S, Mohindra N, Kalyan A, et al. Concordance between genomic alterations assessed by next-generation sequencing in tumor tissue or circulating cell-free DNA. Oncotarget. (2016) 7:65364–73. doi: 10.18632/oncotarget.11692
6. Gerlinger M, Rowan AJ, Horswell S, Larkin J, Endesfelder D, Gronroos E, et al. Intratumor heterogeneity and branched evolution revealed by multiregion sequencing. N Engl J Med. (2012) 366:883–92. doi: 10.1056/NEJMoa1113205
7. Russano M, Napolitano A, Ribelli G, Iuliani M, Simonetti S, Citarella F, et al. Liquid biopsy and tumor heterogeneity in metastatic solid tumors: the potentiality of blood samples. J Exp Clin Canc Res. (2020) 39:95. doi: 10.1186/s13046-020-01601-2
8. Dagogo-Jack I, Shaw AT. Tumour heterogeneity and resistance to cancer therapies. Nat Rev Clin Oncol. (2018) 15:81–94. doi: 10.1038/nrclinonc.2017.166
9. Liu MC, Oxnard GR, Klein EA, Swanton C, Seiden MV, Consortium C, et al. Sensitive and specific multi-cancer detection and localization using methylation signatures in cell-free DNA. Ann Oncol. (2020) 31:745–59. doi: 10.1016/j.annonc.2020.04.013
10. Cohen JD, Li L, Wang Y, Thoburn C, Afsari B, Danilova L, et al. Detection and localization of surgically resectable cancers with a multi-analyte blood test. Science. (2018) 359:926-930. doi: 10.1126/science.aar3247
11. Hubbell E, Clarke CA, Aravanis AM, Berg CD. Modeled reductions in late-stage cancer with a multi-cancer early detection test. Cancer Epidemiol Prev Biomarkers. (2021) 30:460–8. doi: 10.1158/1055-9965.EPI-20-1134
12. Ofman J, Raza A. Taking early cancer detection to the next level. Sci Am. (2020) 18:297–312. Available online at: https://www.scientificamerican.com/article/taking-early-cancer-detection-to-the-next-level/
13. Prouteau A, Denis JA, Fornel PD, Cadieu E, Derrien T, Kergal C, et al. Circulating tumor DNA is detectable in canine histiocytic sarcoma, oral malignant melanoma, and multicentric lymphoma. Sci Rep. (2021) 11:877. doi: 10.1038/s41598-020-80332-y
14. Tagawa M, Shimbo G, Inokuma H, Miyahara K. Quantification of plasma cell-free DNA levels in dogs with various tumors. J Vet Diag Invest. (2019) 31:836-43. doi: 10.1177/1040638719880245
15. Beck J, Hennecke S, Bornemann-Kolatzki K, Urnovitz HB, Neumann S, Ströbel P, et al. Genome aberrations in canine mammary carcinomas and their detection in cell-free plasma DNA. PLoS ONE. (2013) 8:e75485. doi: 10.1371/journal.pone.0075485
16. Lorch G, Sivaprakasam K, Zismann V, Perdigones N, Contente-Cuomo T, Nazareno A, et al. Identification of recurrent activating HER2 mutations in primary canine pulmonary adenocarcinoma. Clin Cancer Res. (2019) 25:5866–77. doi: 10.1158/1078-0432.CCR-19-1145
17. Chibuk J, Flory A, Kruglyak KM, Leibman N, Nahama A, Dharajiya N, et al. Horizons in veterinary precision oncology: fundamentals of cancer genomics and applications of liquid biopsy for the detection, characterization, and management of cancer in dogs. Frontiers Vet Sci. (2021) 8:664718. doi: 10.3389/fvets.2021.664718
18. Ciriello G, Miller ML, Aksoy BA, Senbabaoglu Y, Schultz N, Sander C. Emerging landscape of oncogenic signatures across human cancers. Nat Genet. (2013) 45:1127–33. doi: 10.1038/ng.2762
19. Webster JD, Yuzbasiyan-Gurkan V, Kaneene JB, Miller R, Resau JH, Kiupel M. The role of c-KIT in tumorigenesis: evaluation in canine cutaneous mast cell tumors. Neoplasia. (2006) 8:104–11. doi: 10.1593/neo.05622
20. Gardner HL, Sivaprakasam K, Briones N, Zismann V, Perdigones N, Drenner K, et al. Canine osteosarcoma genome sequencing identifies recurrent mutations in DMD and the histone methyltransferase gene SETD2. Commun Biol. (2019) 2:266. doi: 10.1038/s42003-019-0487-2
21. Kumar M, Choudhury Y, Ghosh SK, Mondal R. Application and optimization of minimally invasive cell-free DNA techniques in oncogenomics. Tumor Biol. (2018) 40:1010428318760342. doi: 10.1177/1010428318760342
22. Pérez-Barrios C, Nieto-Alcolado I, Torrente M, Jiménez-Sánchez C, Calvo V, Gutierrez-Sanz L, et al. Comparison of methods for circulating cell-free DNA isolation using blood from cancer patients: impact on biomarker testing. Transl Lung Cancer Res. (2016) 5:665–72. doi: 10.21037/tlcr.2016.12.03
23. Sorber L, Zwaenepoel K, Jacobs J, Winne KD, Goethals S, Reclusa P, et al. Circulating cell-free DNA and RNA analysis as liquid biopsy: optimal centrifugation protocol. Cancers. (2019) 11:458. doi: 10.3390/cancers11040458
24. Lindblad-Toh K, Wade CM, Mikkelsen TS, Karlsson EK, Jaffe DB, Kamal M, et al. Genome sequence, comparative analysis and haplotype structure of the domestic dog. Nature. (2005) 438:803–19. doi: 10.1038/nature04338
25. Vogelstein B, Papadopoulos N, Velculescu VE, Zhou S, Diaz LA, Kinzler KW. Cancer genome landscapes. Science. (2013) 339:1546–58. doi: 10.1126/science.1235122
26. Alsaihati BA, Ho K-L, Watson J, Feng Y, Wang T, Zhao S. Canine tumor mutation rate is positively correlated with TP53 mutation across cancer types and breeds. Biorxiv [Preprint]. (2020). doi: 10.1101/2020.07.15.205286
27. Lynch TJ, Bell DW, Sordella R, Gurubhagavatula S, Okimoto RA, Brannigan BW, et al. Activating mutations in the epidermal growth factor receptor underlying responsiveness of non–small-cell lung cancer to Gefitinib. N Engl J Med. (2004) 350:2129–39. doi: 10.1056/NEJMoa040938
28. Berger EP, Johannes CM, Jergens AE, Allenspach K, Powers BE, Du Y, et al. Retrospective evaluation of toceranib phosphate (Palladia®) use in the treatment of gastrointestinal stromal tumors of dogs. J Vet Intern Med. (2018) 32:2045–53. doi: 10.1111/jvim.15335
29. Yamazaki H, Miura N, Lai Y-C, Takahashi M, Goto-Koshino Y, Yasuyuki M, et al. Effects of toceranib phosphate (palladia) monotherapy on multidrug resistant lymphoma in dogs. J Vet Med Sci. (2017) 79:16–0457. doi: 10.1292/jvms.16-0457
30. Bettegowda C, Sausen M, Leary RJ, Kinde I, Wang Y, Agrawal N, et al. Detection of circulating tumor DNA in early- and late-stage human malignancies. Sci Transl Med. (2014) 6:224ra24. doi: 10.1126/scitranslmed.3007094
31. Withers SS, York D, Choi JW, Woolard KD, Laufer-Amorim R, Sparger EE, et al. Metastatic immune infiltrates correlate with those of the primary tumour in canine osteosarcoma. Vet Comp Oncol. (2019) 17:242–52. doi: 10.1111/vco.12459
32. Fleming JM, Creevy KE, Promislow DEL. Mortality in North American dogs from 1984 to 2004: an investigation into age-, size-, and breed-related causes of death. J Vet Intern Med. (2011) 25:187–98. doi: 10.1111/j.1939-1676.2011.0695.x
Keywords: cell-free DNA, dog, cancer, tumor, genomic, liquid biopsy, circulating tumor DNA, mutation
Citation: Kruglyak KM, Chibuk J, McLennan L, Nakashe P, Hernandez GE, Motalli-Pepio R, Fath DM, Tynan JA, Holtvoigt LE, Chorny I, Grosu DS, Tsui DWY and Flory A (2021) Blood-Based Liquid Biopsy for Comprehensive Cancer Genomic Profiling Using Next-Generation Sequencing: An Emerging Paradigm for Non-invasive Cancer Detection and Management in Dogs. Front. Vet. Sci. 8:704835. doi: 10.3389/fvets.2021.704835
Received: 04 May 2021; Accepted: 28 May 2021;
Published: 08 July 2021.
Edited by:
Carlos Eduardo Fonseca-Alves, Paulista University, BrazilReviewed by:
Antonio Fernando Leis Filho, São Paulo State University, BrazilJong Hyuk Kim, University of Minnesota Twin Cities, United States
Copyright © 2021 Kruglyak, Chibuk, McLennan, Nakashe, Hernandez, Motalli-Pepio, Fath, Tynan, Holtvoigt, Chorny, Grosu, Tsui and Flory. This is an open-access article distributed under the terms of the Creative Commons Attribution License (CC BY). The use, distribution or reproduction in other forums is permitted, provided the original author(s) and the copyright owner(s) are credited and that the original publication in this journal is cited, in accordance with accepted academic practice. No use, distribution or reproduction is permitted which does not comply with these terms.
*Correspondence: Dana W.Y. Tsui, ZHRzdWkmI3gwMDA0MDtwZXRkeC5jb20=; Andi Flory, YWZsb3J5JiN4MDAwNDA7cGV0ZHguY29t
†These authors contributed equally to this work and share first authorship
‡These authors contributed equally to this work and share senior authorship