- 1Department of Animal Biosciences, University of Guelph, Guelph, ON, Canada
- 2Department of Animal Science, Universidade Federal do Rio Grande do Sul, Porto Alegre, Brazil
- 3Department of Human Health and Nutritional Sciences, University of Guelph, Guelph, ON, Canada
Although emerging data suggests a greater influence of gluconeogenic precursors, endurance sled dogs have long appeared to rely heavily on fatty acid oxidation for sustained energy production. However, much of the research investigating lipid utilization during exercise in sled dogs has been carried out with dogs subjected to extended bouts of endurance exercise. Less is known about changes in fatty acid composition in endurance training sled dogs subjected to short bouts of exercise, and fewer data define how fatty acid composition may change in distinct lipid fractions. As such, the study objective was to assess whether short bouts of submaximal exercise would affect fatty acid profiles of serum lipid fractions in endurance training sled dogs. Fifteen privately-owned Siberian huskies were used (8 females: 4 intact, 4 spayed; 7 males: 2 intact, 5 neutered), with an average age of 4.6 ± 2.5 years and body weight of 24.8 ± 4.2 kg. Throughout the diet acclimation and remainder of the study, all dogs were fed a dry extruded diet that met or exceeded all AAFCO nutrient recommendations. Dogs were weighed weekly and fed to maintain baseline body weight. A 12-week exercise regimen was designed to incorporate weekly increases in running distance, but weather played a role in setting the daily distance. On weeks 2, 5, and 11, an exercise challenge was implemented whereby dogs would run 4 km at 15 km/h in teams of 4. Pre- and post-exercise blood samples were taken, and gas chromatography was used to evaluate fatty acid profiles of all identified serum lipid fractions (cholesterol ester, diacylglycerol, free fatty acid, phospholipids, triglyceride). Data were analyzed using PROC MIXED of SAS, with dog as a random effect and week and sampling time point as fixed effects. Composition of oleic (18:1n9), linoleic (18:2n6), and alpha-linolenic (18:3n3) acids in the free fatty acid fraction decreased by ~9, 10, and 60%, respectively, following exercise (P ≤ 0.05). The results presented herein suggest that aside from a degree of depletion of these 18-carbon unsaturated fatty acids, short bouts of submaximal exercise do not induce considerable changes to sled dog fatty acid profiles.
Introduction
Largely due to the insulative properties of their coats decreasing thermoregulatory energy expenditure at low environmental temperatures, the maintenance energy requirements of Siberian huskies are similar to or even lower than many other breeds (1, 2). However, as level of exercise and energy expenditure are positively associated (3), the requirement for dietary energy will increase as exercise increases in duration and intensity (3). Due to the extended duration of the exercise performed, combined with the fact that sled dogs are typically housed, trained and raced outdoors during months where the ambient temperature is below the thermoneutral zone of these breeds (4, 5), racing sled dogs can require upwards of 1,000 kcal/kg of metabolic body weight per day (6). As such, adequate provision of dietary energy is a critical aspect of nutritional programs developed for sporting dogs undergoing endurance-style training and competition, especially at sub-thermoneutral temperatures.
Depending on the duration and intensity of physical exertion, exercising dogs will utilize several sources of energy to support the demands of working muscle (1). While contributions from the phosphagen system, anaerobic and/or aerobic glycolysis support the immediate energy demands of working muscle, lipid oxidation has been recognized as a principal source of metabolic fuel for endurance-style athletes (7, 8). Endurance racing sled dogs have long appeared to rely heavily on the oxidation of fatty acids (FA) for sustained energy production, and in fact, the contribution of lipid oxidation to total energy production of dogs is twice that of humans, both at rest and during exercise (9, 10). This is due, in part, to the greater affinity for albumin to bind free fatty acids (FFA) in dogs relative to less aerobic species such as humans or goats (11–13), since transport of exogenous FA into working muscle relies on albumin binding (14). Dogs also have a greater mitochondrial volume and capillary red cell volume than goats, which likely enhances the oxygen-transporting capacity of the exercising dog. Furthermore, in addition to possessing greater intracellular stores of both glycogen and lipids, lipid droplets appear to be more tightly associated with mitochondria in the dog than the goat (13). Moreover, while there are a variety of hormones responsible for the regulation of substrate metabolism during a bout of exercise, the catecholamines norepinephrine and epinephrine play a predominant role in initiating the FA oxidation cascade in the adipocyte (14). Serum concentrations of both norepinephrine and epinephrine increased above resting levels in dogs subjected to as little as 5 min of moderate intensity exercise, with the exercise-induced response of epinephrine appearing to be greater in dogs than in humans (15). This may also lend some indication as to greater capacity for dogs to mobilize and oxidize fats compared to less aerobic species like humans, at least during exercise.
Feeding sled dogs high fat diets can increase resting levels of serum triglycerides (TAG) and FFA to be used as oxidative fuel sources (16), which can “spare” the use of muscle glycogen, therefore delaying the onset of fatigue related with muscle glycogen depletion (17, 18). As such, sled dogs have traditionally been fed high fat diets; however, more recent research suggests that sled dogs may in fact rely on carbohydrate oxidation during extended submaximal exercise, sustained by gluconeogenic precursors (e.g., amino acids, glycerol, lactate), even when fed low-to-moderate carbohydrate diets (19, 20). Although sled dogs are experiencing FFA depletion when subjected to extreme distance (e.g., 1,500+ km) submaximal exercise (20, 21), based on serum metabolomic data from Alaskan huskies, it appears as though prolonged aerobic exercise may create a beta-oxidation bottle neck, as accumulation of carnitine-bound FA (binding required for mitochondrial translocation) was evident, particularly midway (~775 km) through a multi-day ultra-endurance race (20).
Much of the research that has helped shape our understanding of lipid utilization during exercise in sled dogs (16, 18–23) has been done under conditions of long-distance races or training bouts of extended duration; however, less is known regarding changes in FA profiles in endurance training sled dogs subjected to short bouts of exercise. Moreover, as incremental conditioning regimens, which are comprised of exercise duration and/or intensity increasing in a stepwise manner, have long been implemented for endurance training athletes (24–28), improving our understanding of lipid metabolism and utilization during short and extended bouts of submaximal exercise is imperative. Finally, although more labor intensive than determining total serum lipid, the assessment of FA in circulating lipid fractions is valuable as these pools will all differ in FA composition, and as such may reflect tissue level changes in response to metabolic perturbations (29). Examining the effects of interventions, such as exercise, on total serum lipids may mask changes occurring in any single lipid fraction, potentially leading to inaccurate conclusions (30). As such, the objective of this current study was to investigate whether short bouts of submaximal exercise (4 km at 15 km/h) would affect FA profiles of serum lipid fractions in endurance training sled dogs. We hypothesized that exercise bouts of this duration and intensity would not induce changes in FA composition of any serum lipid fraction.
Materials and Methods
Animals and Housing
The present experiment was approved by the University of Guelph's Animal Care Committee (animal use protocol #4008). Fifteen client-owned domestic Siberian huskies (8 females: 4 intact, 4 spayed; 7 males: 2 intact, 5 neutered), with an average age of 4.6 ± 2.5 years and body weight (BW) of 24.8 ± 4.2 kg, were used in the study. Sixteen dogs were enrolled in the study for exercise purposes (so as to balance the gangline); however, due to an aversion to the restraint and collection procedure, no blood samples were collected from one dog (spayed female) throughout the trial period. Dogs were housed and trained at an off-site facility (Rajenn Siberian Huskies, Ayr, ON) that had been visited and approved by the University of Guelph's Animal Care Services. During the study, dogs were pair or group-housed in free-run, outdoor kennels that ranged in size from 3.5 to 80 square meters and contained between 2 and 10 dogs each. Two dogs were removed from the trial (one neutered male on week 7, one neutered male on week 9) due to exercise-related injuries; all data collected from them up until their respective points of removal are included.
Diets and Study Design
Throughout the diet acclimation period (4 weeks) (16, 31, 32) the remainder of the study period (10 weeks), all dogs were fed a dry extruded diet (Champion Petfoods LT., Morinville, AB) that met or exceeded all NRC (3) and AAFCO (33) nutrient recommendations (Table 1) and were fed once daily at 17:00 h. Diet intake levels at the beginning of the acclimation period were determined from historical feeding records; BW was recorded for each dog at the beginning of the acclimation period and measured each week subsequently. Food allotments were adjusted to maintain the dogs' initial BW. For detailed information regarding diet intake and BW throughout the study period, refer to Templeman et al. (27). At feeding, all dogs were tethered and fed individually to allow for monitoring/measurement of diet consumption. Any orts were weighed and recorded daily. Throughout the entire trial period, all dogs were allowed ad libitum access to fresh water.
Exercise Regimen and Exercise Challenges
A 12-week exercise regimen (2 weeks during diet acclimation, 10 weeks thereafter) was proposed whereby exercise duration would increase incrementally. However, decisions regarding the distance ran each day and number of stops (e.g., for water) were made with consideration of the ambient temperature and humidity. Inclement weather also caused the removal of the exercise challenge proposed to take place on week 8. Training consisted of dogs running on a standard 16-dog gangline with the gangline attached to an all-terrain vehicle with one rider who controlled the machine in its lowest gear. A pace of 15 km/h was averaged throughout the training period. Running pace and distance traveled was measured using a digital speedometer and odometer on the all-terrain vehicle. On weeks 2, 5, and 11, one off-day (no training) was replaced by an exercise challenge whereby dogs would run a consistent distance at a consistent pace as a team of 4 dogs. Four-dog teams were predetermined based on each dogs' position in the 16-dog gangline. All challenge groups for all weeks (2, 5, and 11) ran for 4 km at 15 km/h, with exercise challenges commencing at 07:00 h. In brief, dogs ran ~80 km total in total during the first 2 weeks, and ~1,250 km total over the next 10 weeks. For additional details regarding the exercise regimen (e.g., anticipated and actual daily/weekly run distances) or exercise challenges (e.g., determination of challenge distance), refer to Templeman et al. (27).
Blood Sample Collection and Analysis
On exercise challenge days (weeks 2, 5, and 11), 5 ml pre-challenge blood samples were taken via cephalic venipuncture with a serum Vacutainer® system (Becton, Dickinson and Company, Franklin Lakes, NJ, USA) following an overnight fast. Pre-exercise samples were taken 15 min prior to performing the exercise challenge (06:45 h). Once the challenge commenced (~16 min in duration), dogs were immediately watered and then had a post-exercise blood sample taken (~07:20 h) using the same procedure as stated above. All blood samples were centrifuged at 2,000 × g for 20 min at 4°C using a Beckman J6-MI centrifuge (Beckman Coulter, Indianapolis, IN), then serum aliquots were collected, frozen and stored at −80°C prior to analysis. Serum samples were analyzed for FA content and FA fractions using gas chromatography as described below.
Lipid extraction was done using the Folch method (34) Serum samples were thawed on ice then 100 μl of serum was added to 900 μl 0.1 M KCL (Sigma-Aldrich, Oakville, ON). A freshly prepared 2:1 CHCl3: MeOH (Sigma-Aldrich, Oakville, ON) mixture was added and the samples were vortexed, flushed with nitrogen, and kept overnight at 4°C. Samples were centrifuged at 357 × g for 10 min at 21°C using a Beckman J6-MI centrifuge (Beckman Coulter, Indianapolis, IN) and the chloroform layer was collected, dried down under nitrogen, and reconstituted with 100 μl of chloroform.
All samples were analyzed by lipid-class thin-layer chromatography (TLC). In brief, G-plates (EMD Chemicals Inc., Billerica, MS) were activated in an oven for 1 h at 100°C prior to being spotted by samples. Once spotted with the reconstituted samples, the G-plates were then placed in a TLC tank with freshly made TLC solvent containing 80 ml petroleum ether, 20 ml ethyl ether, and 1 ml acetic (Sigma-Aldrich, Oakville, ON). Following this, the plates were then lightly sprayed with 0.1% (w/v) 8-Anilino-1-naphthalenesulfonic acid ammonium (Sigma-Aldrich, Oakville, ON) before being visualized under UV light. Lipid class bands corresponding to cholesterol ester (CE), diacylglycerol (DAG), FFA, phospholipids (PL), and TAG were confirmed using authentic standards and collected for further analysis.
Methylation was performed by adding 2 ml of hexane and 2 ml of 14% BF3-MeOH (Sigma-Aldrich, Oakville, ON) to the samples and incubating them at 100°C for 60 min. Following methylation, 2 ml of double distilled H2O was added to the samples and the solution was immediately vortexed for 30 s to halt methylation. Samples were centrifuged at 357 × g for 10 min at 21°C using a Beckman J6-MI centrifuge (Beckman Coulter, Indianapolis, IN), the hexane layer was collected and dried down under nitrogen before reconstitution in 50 μl of hexane. Fatty acid methyl esters were quantified on an Agilent 6890 gas chromatograph (Agilent Technologies, Mississauga, ON) equipped with flame ionization detection and separated on an DB-FFAP fused-silica capillary column (15 m, 0.1 μm film thickness, 0.1 mm i.d.; Agilent Technologies, Mississauga, ON). Samples were injected in 200:1 split mode. The injector and detector ports were set at 250°C. Fatty acid methyl esters were eluted using a temperature program set initially at 150°C and held for 15 s, increased at 35°C/min and held at 170°C for 3 min, increased at 9°C/min to 225°C, and finally increased 80°C/min to 245°C and held for 2.2 min. The run time per sample is 12 min. The carrier gas was hydrogen, set to a 30 ml/min constant flow rate. Peaks were identified by retention times of FA methyl ester standards (Nu-Chek-Prep, Elysian, MN) using EZchrom Elite v. 3.2.1 software (Agilent Tech., Mississauga, ON). Fatty acid composition was calculated as percent (%) of total peak area.
Statistical Analysis
Statistical analyses were performed with Statistical Analysis System (SAS) (v. 9.4; SAS Institute Inc., Cary, NC). All data were analyzed using PROC MIXED, where dog was treated as a random effect and week and sampling time point (pre- or post-exercise) were treated as fixed effects. Week was also treated as a repeated measure. When the fixed effects were significant, means were separated using the Tukey HSD. A Shapiro-Wilk test was used to assess normality and data were not transformed (P > 0.05). Statistical significance was declared at a P ≤ 0.05. Results are reported as least square means (LSM) ± standard error (SE) of the mean.
Results
For the isolated CE fraction, no differences were identified between the pre- and post-exercise % composition for any FA for any week (Supplementary Table 1) or when pooled across weeks (P > 0.05, Table 2). With regard to differences observed with time, % composition of myristic (14:0; SFA), myristoleic (14:1; MUFA), palmitic (16:0; SFA), palmitoleic (16:1n9; MUFA), vaccenic (18:1n11; MUFA), linoleic (18:2n6; PUFA), and arachidonic (20:4n6; PUFA) acids all differed with week (P ≤ 0.05, Table 2). For each lipid fraction, only FA with mean % compositions of >5 will be discussed further; however, data for all FA identified for each fraction are described in Table 2 (CE), Table 3 (DAG), Table 4 (FFA), Table 5 (PL), and Table 6 (TAG). Percent composition of palmitic (16:0; SFA) acid was greater at week 11 than at weeks 2 and 5 (P ≤ 0.05, Table 2), but did not differ between weeks 2 and 5 (P > 0.05, Table 2). Linoleic (18:2n6; PUFA) acid % composition was greater at weeks 2 and 5 compared to week 11 (P ≤ 0.05, Table 2), but did not differ between weeks 2 and 5 (P > 0.05, Table 2). Arachidonic acid (20:4n6; PUFA) % composition was greater at week 2 than at weeks 5 and 11 and were greater at week 5 than week 11 (P ≤ 0.05, Table 2).
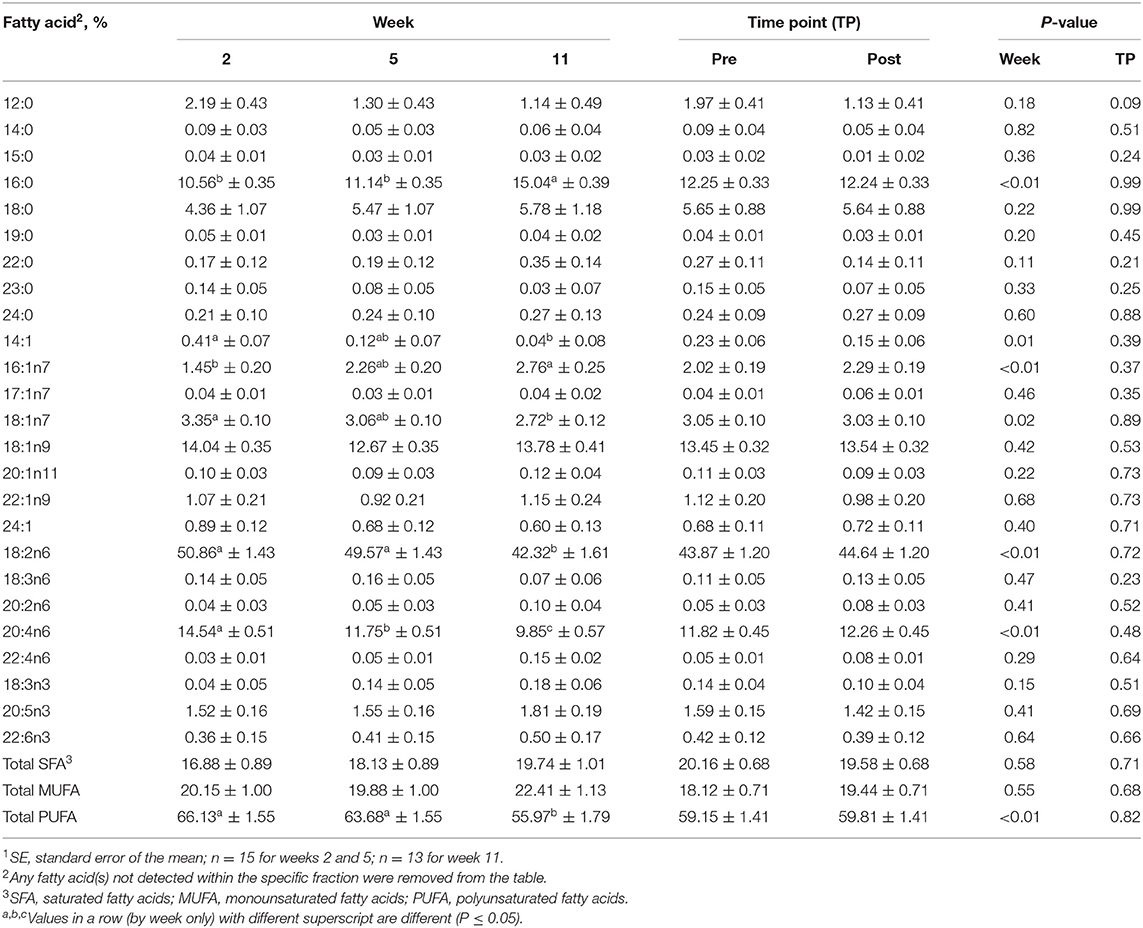
Table 2. Mean fatty acid composition (±SE1) and percent contribution of saturated fatty acids (SFA), monounsaturated fatty acids (MUFA), and polyunsaturated fatty acids (PUFA) for the cholesterol ester (CE) fraction identified in serum of all dogs at weeks 2, 5, and 11 (pooled across pre- and post-exercise sampling time points) and at the pre- and post-exercise sampling time points (pooled across weeks 2, 5, and 11).
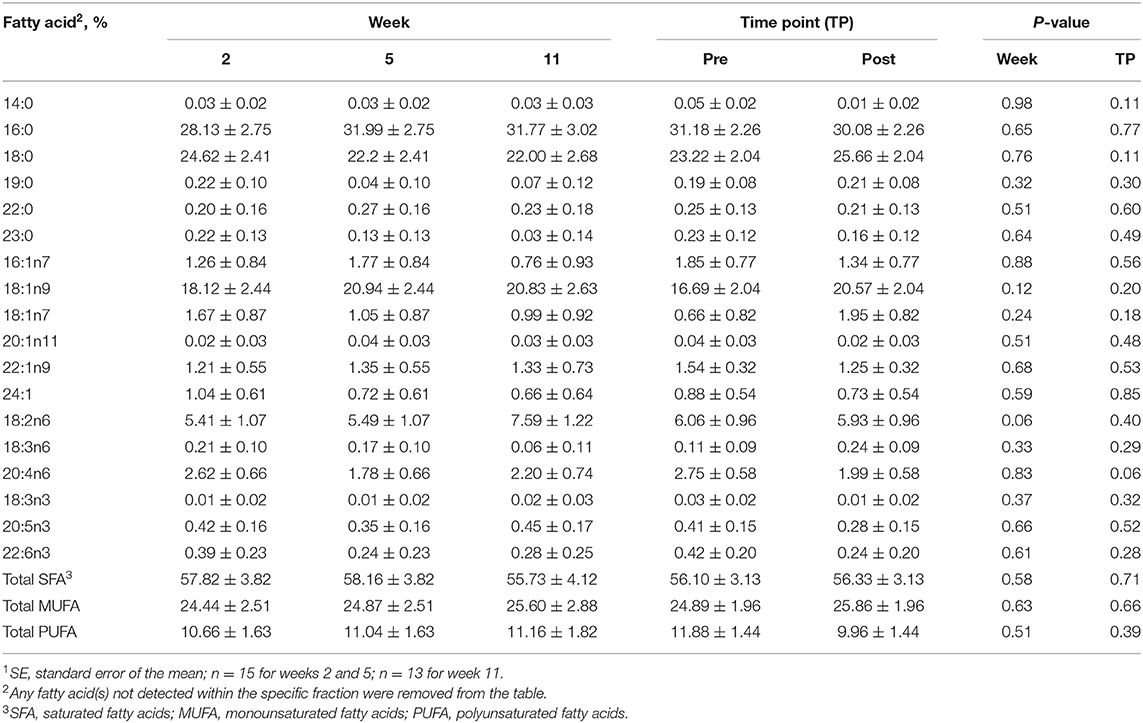
Table 3. Mean fatty acid composition (±SE1) and percent contribution of saturated fatty acids (SFA), monounsaturated fatty acids (MUFA), and polyunsaturated fatty acids (PUFA) for the diacylglycerol (DAG) fraction identified in serum of all dogs at weeks 2, 5, and 11 (pooled across pre- and post-exercise sampling time points) and at the pre- and post-exercise sampling time points (pooled across weeks 2, 5, and 11).
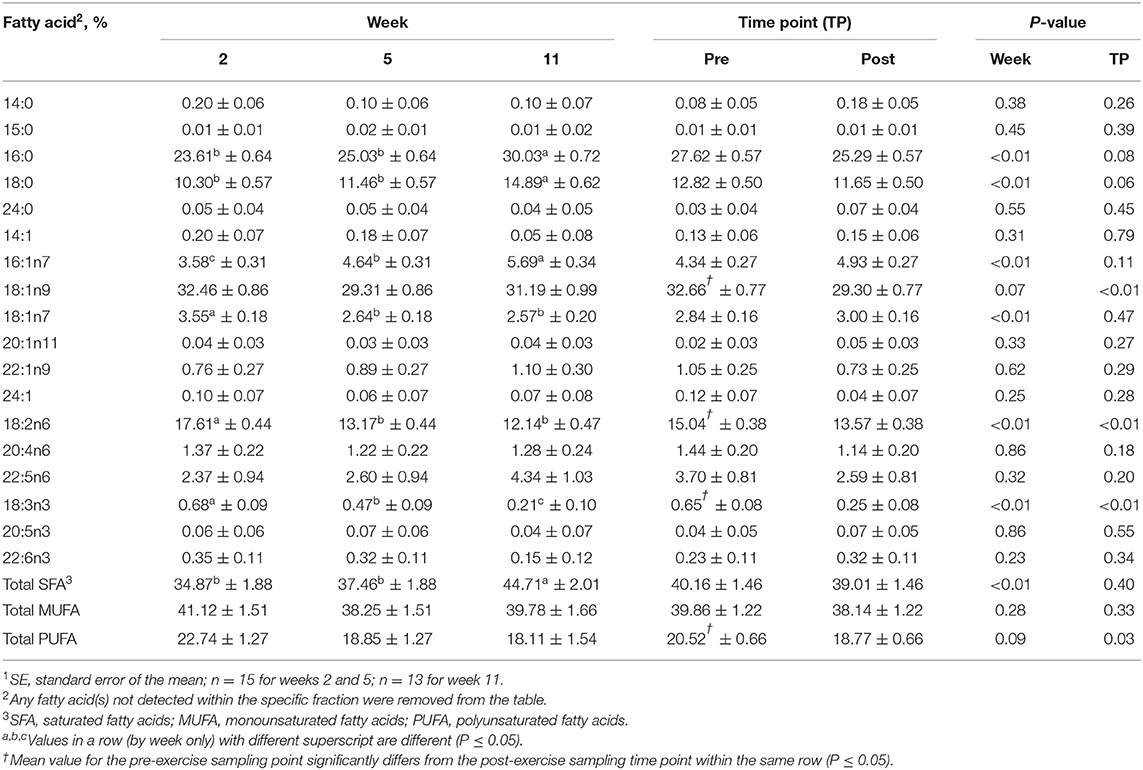
Table 4. Mean fatty acid composition (±SE1) and percent contribution of saturated fatty acids (SFA), monounsaturated fatty acids (MUFA), and polyunsaturated fatty acids (PUFA) for the free fatty acid (FFA) fraction identified in serum of all dogs at weeks 2, 5, and 11 (pooled across pre- and post-exercise sampling time points) and at the pre- and post-exercise sampling time points (pooled across weeks 2, 5, and 11).
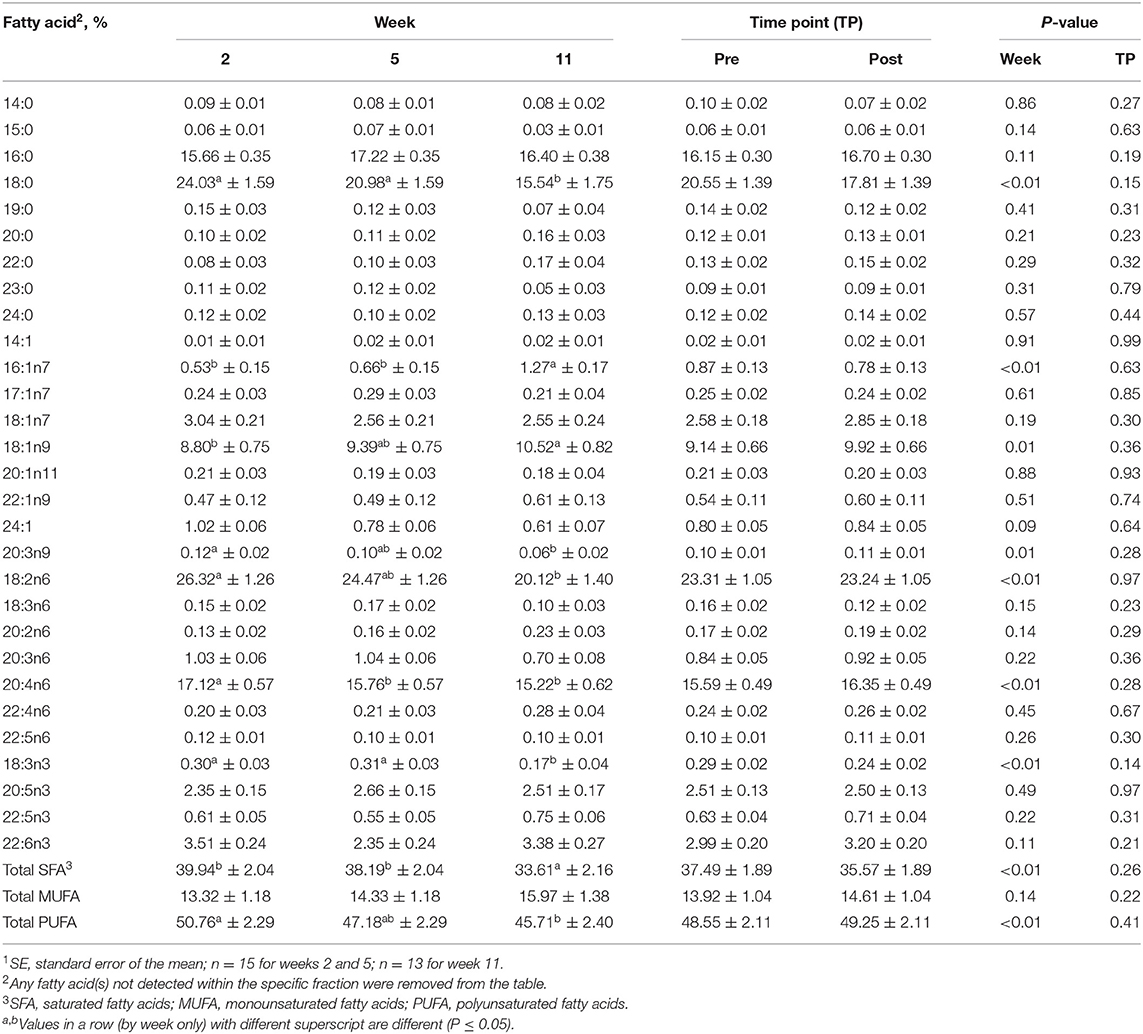
Table 5. Mean fatty acid composition (±SE1) and percent contribution of saturated fatty acids (SFA), monounsaturated fatty acids (MUFA), and polyunsaturated fatty acids (PUFA) for the phospholipid (PL) fraction identified in serum of all dogs at weeks 2, 5, and 11 (pooled across pre- and post-exercise sampling time points) and at the pre- and post-exercise sampling time points (pooled across weeks 2, 5, and 11).
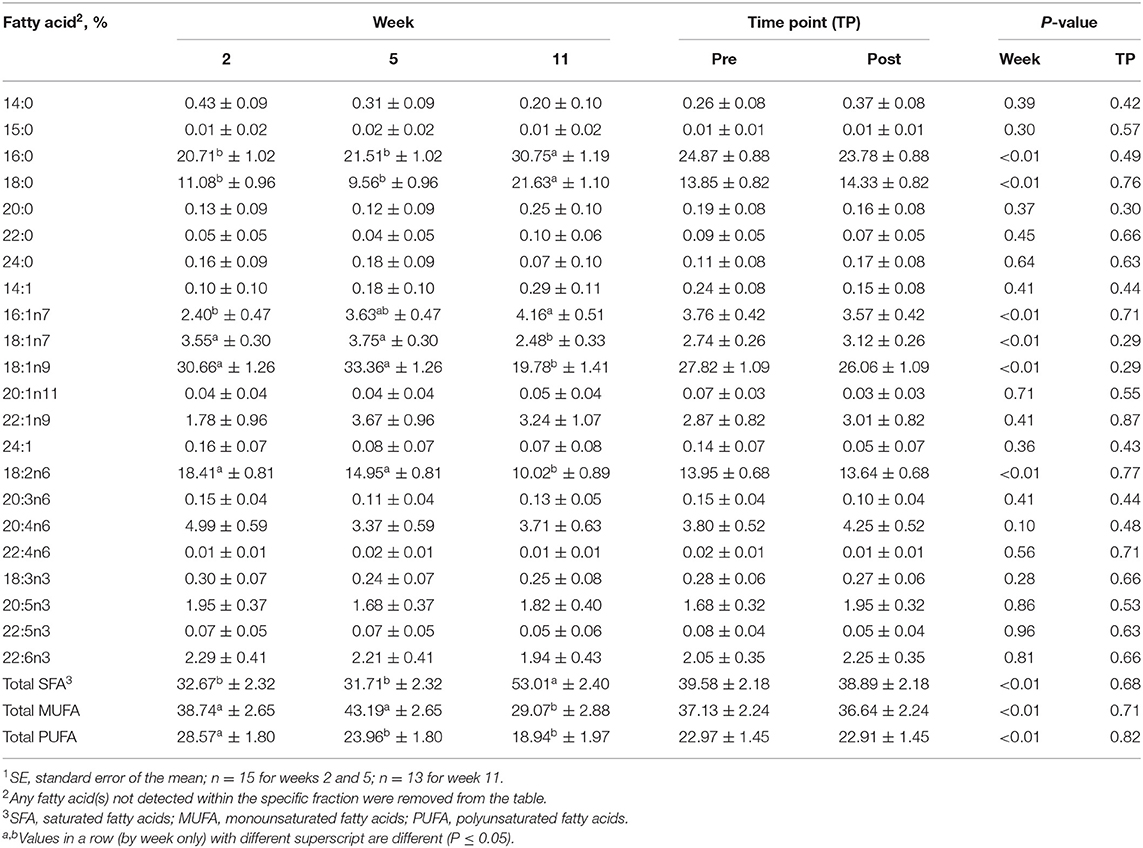
Table 6. Mean fatty acid composition (±SE1) and percent contribution of saturated fatty acids (SFA), monounsaturated fatty acids (MUFA), and polyunsaturated fatty acids (PUFA) for the triacylglycerol (TAG) fraction identified in serum of all dogs at weeks 2, 5, and 11 (pooled across pre- and post-exercise sampling time points) and at the pre- and post-exercise sampling time points (pooled across weeks 2, 5, and 11).
For the DAG fraction, no differences were identified between the pre- and post-exercise % compositions for any FA for any week (Supplementary Table 2) or when pooled across weeks (P > 0.05, Table 3). As well, no differences were observed with week for any FA (P > 0.05, Table 3).
For the FFA fraction, no differences were identified between the pre- and post-exercise % composition for any FA for any week (Supplementary Table 3); however, when these data were pooled, the pre-exercise % compositions of oleic (18:1n9; MUFA), linoleic (18:2n6; PUFA), and alpha-linolenic (18:3n3; PUFA) acids were greater than post-exercise (P ≤ 0.05, Table 4). For differences observed over time, % compositions of palmitic (16:0; SFA), palmitoleic (16:1n9; MUFA), stearic (18:0; SFA), vaccenic (18:1n11; MUFA), linoleic (18:2n6; PUFA), and alpha-linolenic (18:3n3; PUFA) acids all differed with week (P ≤ 0.05, Table 4). Percent compositions of palmitic (16:0; SFA) and stearic (18:0; SFA) acids were greater at week 11 than at weeks 2 and 5 (P ≤ 0.05, Table 4), but did not differ between weeks 2 and 5 (P > 0.05, Table 4). Percent composition of palmitoleic (16:1n9; MUFA) acid was greater at week 11 than at weeks 5 and 2 and was greater at week 5 than week 2 (P ≤ 0.05, Table 4). Linoleic (18:2n6; PUFA) acid % composition was greater at week 2 than at weeks 5 and 11 (P ≤ 0.05, Table 4), but did not differ between weeks 5 and 11 (P > 0.05, Table 4).
For the PL fraction, no differences were observed between pre- and post-exercise % composition for any FA for any week (Supplementary Table 4) or when pooled across weeks (P > 0.05, Table 5). With regard to differences observed with time, % compositions of palmitoleic (16:1n9; MUFA) and oleic (18:1n9; MUFA) increased with time. The % compositions of stearic (18:0; SFA), linoleic (18:2n6; PUFA), alpha-linolenic (18:3n3; PUFA), mead (20:3n9; PUFA), and arachidonic (20:4n6; PUFA) acids all decreased with week (P ≤ 0.05, Table 5). Percent composition of stearic (18:0; SFA) acid was greater at weeks 2 and 5 compared to week 11 (P ≤ 0.05, Table 5), but did not differ between weeks 2 and 5 (P > 0.05, Table 5). Oleic (18:1n9; MUFA) acid % composition was greater on week 11 than on week 2 (P ≤ 0.05, Table 5), but on week 5 did not differ from weeks 2 or 11 (P > 0.05, Table 5). Percent composition of linoleic acid (18:2n6; PUFA) was greater on week 2 compared to week 11 (P ≤ 0.05, Table 5), but on week 5 did not differ from weeks 2 or 11 (P > 0.05, Table 5). Arachidonic acid (20:4n6; PUFA) % composition as greater on week 2 than on weeks 5 and 11 (P ≤ 0.05, Table 5), but did not differ between weeks 5 and 11 (P > 0.05, Table 5).
For the TAG fraction, no differences were observed between pre- and post-exercise % compositions for any FA for any week (Supplementary Table 5) or when pooled across weeks (P > 0.05, Table 6). For differences observed with week, % compositions of palmitic (16:0; SFA), palmitoleic (16:1n9), stearic (18:0; SFA), oleic (18:1n9; MUFA), vaccenic (18:1n11; MUFA), and linoleic (18:2n6; PUFA) acids all differed with week (P ≤ 0.05, Table 6). Palmitic (16:0; SFA) and stearic (18:0; SFA) acid % compositions were greater at week 11 compared to weeks 2 and 5 (P ≤ 0.05, Table 6), but did not differ between weeks 2 and 5 (P > 0.05, Table 6). Oleic (18:1n9; MUFA) and linoleic (18:2n6; PUFA) acid % compositions were greater for weeks 2 and 5 than for week 11 (P ≤ 0.05, Table 6), but did not differ between weeks 2 and 5 (P > 0.05, Table 6).
Discussion
Fatty acid profiles of serum lipid fractions of endurance training sled dogs were examined before and after short bouts of submaximal exercise during a multi-week endurance training regimen. The objective was to investigate whether these short exercise bouts affect sled dog FA profiles, as shifts in serum FA had been observed in sled dogs subjected to extended durations of submaximal exercise (16, 20, 21).
The results presented herein suggest that bouts of submaximal exercise of 15 min or less (4 km at 15 km/h) induce few changes to sled dog FA profiles. Composition of oleic (18:1n9; MUFA), linoleic (18:2n6; PUFA), and alpha-linolenic (18:3n3; PUFA) acids in the FFA fraction decreased by ~9, 10, and 60%, respectively, following exercise, suggesting a degree of depletion and/or tissue uptake of these 18-carbon unsaturated FA in response to these short runs. Gold et al. (35) subjected mongrel dogs to 20–25 min of submaximal exercise and reported reductions in serum concentrations of 18:1n9 and 18:2n6 FFA (~6 and 2%, respectively), but concentrations of 18:3n3 were not reported. These changes are less substantial than those observed in the current study, but the dogs used by Gold et al. (35) were running at approximately half the pace (6 km/h) than those in the current study. Unfortunately, little information was presented regarding the conditioning of the dogs prior to the exercise challenge, or the diets being fed (35). McClelland et al. (12) set out to investigate how or if the serum FFA responses to exercise would differ between two species of varying aerobic capacity: dogs and goats. The comparable aspect of this study was confounded by differences in dietary FA composition; however, comparison aside, when dogs were subjected to exercise demanding 40% of their maximal rate of oxygen consumption (VO2max), oleic acid (18:1n9) concentrations decreased by ~10% in serum by ~20–30 min of exercise. This decrease was short lived, though, as serum concentrations were greater than pre-exercise levels by ~100 min after exercise and staying elevated for up to 190 min post-exercise (12). Concentrations of linoleic acid (18:2n6) were unchanged after ~20–30 min of exercise, but the serum concentrations decreased by ~12% after 30 min and remained lower than pre-exercise levels during post-exercise recovery (12). Unfortunately, concentrations of 18:3n3 were also not reported and the breed of dog used was not disclosed by (12). As a note, the dogs in the current study were running at a pace of 15 km/h, which would be equivalent in relative intensity to ~50% of VO2max pace for moderately trained sled dogs (29.5 km/h) (36). Ultimately, these data suggest that changes to Siberian husky FA profiles induced in response to short bouts of submaximal exercise are similar in specific FFA and in magnitude of change to that of other breeds that would be used less prominently for endurance sled dog racing, but may be used for different types of endurance-style activity, such as hunting, search and rescue or scent detection (37, 38).
Although short bouts of exercise only minimally affected FA profiles, changes were observed as dogs progressed through training in the present study. Noteworthy outcomes included decreasing serum % composition of linoleic (18:2n6) and arachidonic (20:4n6) acids. Linoleic acid, an essential FA for all mammals including dogs (39), is a metabolic precursor of arachidonic acid, and while the diet contained moderate levels of linoleic acid, the arachidonic acid content was low (<0.5%). As such, the reduction in linoleic acid over time may be evidence of its use to synthesize arachidonic acid by way of delta 6 desaturase, elongase, and delta 5 desaturase (40). However, with % composition of arachidonic acid also decreasing over time and as the dogs trained and specifically in the CE and PL fractions, the demand for downstream metabolites of arachidonic acid, such as eicosanoids, appeared to have been greater than the supply from linoleic acid and/or the diet. When released from membrane PL, arachidonic acid can be converted to an array of different eicosanoids, such as prostaglandins, many of which are involved in the promotion of inflammatory responses, including exercise-induced inflammatory responses (41). Considering that the dogs in the present study were exercising 4 days a week for 12 weeks, it is likely that they were experiencing some degree of acute and/or chronic inflammation. These data may indicate that as exercise conditioning continues, that endurance training dogs may benefit from supplemental omega 6 FA; however, the changes observed in FA profiles over time in this study may alternatively suggest that a diet acclimation period of >4 weeks [identified with Alaskan huskies by (16); mixed breed dogs by (31), and cats by (32)] may be necessary to capture diet-induced changes to lipid profiles for adult Siberian huskies. Angle et al. (42) investigated the effects of exercise and diet (high fat vs. low fat vs. high PUFA) on olfactory capability in certified scent detection dogs and fed each diet for 8 weeks so as to allow for “metabolic adjustments to the diet” prior to olfaction testing. While differences in olfactory acuity were observed, with dogs on high PUFA diet being 1.4 times as likely to find a target than those on the high fat diet, there was never any support or evidence provided as to why this 8-week adaptation period was necessary (42). However, if a dietary adaptation of 8 weeks is necessary for exercising or performance dogs when investigating outcomes related to lipid metabolism, perhaps this may lend some support as to why changes over time, particularly for the essential PUFA linoleic acid (18:2n6) were more evident at 11 weeks rather than at 5 weeks.
A limitation of this study that should be acknowledged is that since these dogs experienced changes in body composition (increase in lean mass, decrease in fat mass, but no change in overall BW; 27) throughout the study period, it is not possible to differentiate between the effect of diet and the effect of exercise-induced changes to endogenous fat stores. Unfortunately, there is little information available on the diet (Redpaw PowerEdge 32k, Redpaw Inc., Franklin, WI) the dogs were fed prior to this study. All that is known is the minimal amount of crude fat (20%) from the guaranteed analysis, but the authors did not have access to data on the true (analyzed) fat content and/or FA composition of the diet. As such, no inferences can be drawn regarding how or if the FA composition of this diet contributed to changes observed over time in the present study. An additional limitation was the lack of a control group of dogs that remained sedentary throughout the study period. Having the ability to compare FA profiles between sedentary and actively-training dogs with all other management conditions being maintained would have provided insight into how or if the changes in FA profiles throughout the study period were a result of diet, exercise, environment, or a combination of these factors. However, when working with a cohort of client owned sled dogs preparing for a racing season, this was simply not feasible.
There is value in assessing FA composition of individual lipid fractions as these pools differ considerably in FA composition and each play distinct physiological roles (30). In fact, diversity of lipid fractions can be used to indicate the development or attenuation of various metabolic conditions, such as diabetes and obesity, in human and canine subjects alike (43–46); although, these outcomes were not necessarily within scope for this project as all dogs participating were healthy. With regard to assessing FA composition in healthy, exercising dogs, serum FFA has been examined most commonly (12, 21, 35), as it is known to be the most labile fraction during exercise. While the data presented herein will help to partially fill that void, future research evaluating changes in FA composition within lipid fractions in response to extended bouts of submaximal exercise is warranted. Furthermore, it would be of interest to assess these changes in FA composition within distinct lipid fractions of other tissues, such as adipose or muscle, alongside of serum, as these data may bridge our understanding of lipid mobilization and muscle metabolism in the exercising dog. Finally, the authors acknowledge that by only determining and reporting FA composition of individual lipid fractions as % of total peak area, that changes in FA composition should be interpreted with caution. While these data are valuable considering the dearth of available literature in this area, particularly with endurance trained sled dogs, it should be recognized that when expressing the values as % composition, each analyzed FA has the potential to influence the relative % of any/all other FA, as opposed to expressing the values as concentrations, where changes in FA are independent of each other (30, 47). As well, it should be recognized that an assessment of FA composition in serum simply provides an indication as to what is occurring in blood at that moment of time, essentially representing the balance between FA release into circulation and/or uptake into tissue. These samples do not represent kinetic or dynamic measurements of FA mobilization, and as such, it is important to keep this limitation in mind when interpreting changes (or lack thereof) in FA composition.
Conclusions
The results from this study indicate that aside from reductions in % composition of oleic, linoleic, and alpha linolenic acids observed in the FFA lipid fraction, short bouts of submaximal exercise do not induce considerable changes to sled dog FA profiles. Moreover, depletion of these select 18-carbon unsaturated FA are comparable to changes observed in less endurance-specialized breeds subjected to exercise of similar intensity and duration. Changes in FA profiles in all lipid fractions aside from DAG were observed throughout the duration of the study; however, due to a lack of a sedentary control group of dogs, it is not possible to determine if these outcomes were a result of diet (e.g., insufficient dietary adaptation) or exercise training (e.g., reductions in endogenous lipid stores). While the data presented herein helps to improve our understanding of lipid utilization in sled dogs, future research investigating changes in circulating FA as well as gluconeogenic precursors (e.g., amino acids, lactate, glycerol) in both exercising and sedentary sled dogs is warranted to elucidate the extent to which these endurance-trained dogs rely on lipid oxidation during exercise.
Data Availability Statement
The raw data supporting the conclusions of this article will be made available by the authors, without undue reservation.
Ethics Statement
The animal study was reviewed and approved by University of Guelph's Animal Care Committee (animal use protocol #4008). Written informed consent was obtained from the owners for the participation of their animals in this study.
Author Contributions
JRT and AKS designed research. JRT conducted research. JRT, LT, DWLM, and AKS analyzed the data and wrote the manuscript. AKS had primary responsibility for the final content. All authors have read and approved the final manuscript.
Conflict of Interest
The authors declare that the research was funded by Champion Petfoods LT. (Morinville, AB) and Mitacs (Toronto, ON), which could be construed as a potential conflict of interest; however, the authors did not receive any personal financial incentives.
Publisher's Note
All claims expressed in this article are solely those of the authors and do not necessarily represent those of their affiliated organizations, or those of the publisher, the editors and the reviewers. Any product that may be evaluated in this article, or claim that may be made by its manufacturer, is not guaranteed or endorsed by the publisher.
Supplementary Material
The Supplementary Material for this article can be found online at: https://www.frontiersin.org/articles/10.3389/fvets.2021.704770/full#supplementary-material
References
1. Grandjean D, Paragon BM. Nutrition of racing and working dogs. Part 1: energy metabolism of dogs. Compend Cont Educ. (1992) 14:1608–15.
2. Mussa P, Prola L. Dog nutrient requirements: new knowledge. Vet Res Commun. (2005) 29:35–8. doi: 10.1007/s11259-005-0008-2
3. National Research Council. Nutrient Requirements of Dogs and Cats. 2nd rev. ed. Washington, DC: National Academies Press (2006).
4. Scholander PF, Hock R, Walters V, Irving L. Adaptation to cold in arctic and tropical mammals and birds in relation to body temperature, insulation, and basal metabolic rate. Biol Bull. (1950) 99:259–71. doi: 10.2307/1538742
5. Gerth N, Redman P, Speakman J, Jackson S, Starck JM. Energy metabolism of Inuit sled dogs. J Comp Physiol. (2010) 180:577–89. doi: 10.1007/s00360-009-0432-7
6. Hinchcliff KW, Reinhart GA, Burr JR, Schreier CJ, Swenson RA. Metabolizable energy intake and sustained energy expenditure in Alaskan sled dogs during heavy exertion in the cold. Am J Vet Res. (1997) 58:1457–62.
7. Gollnick PD. Exercise, adrenergic blockage, and free fatty acid mobilization. Am J Physiol. (1967) 213:734–8. doi: 10.1152/ajplegacy.1967.213.3.734
8. Romijn JA, Coyle EF, Sidossis LS, Gastaldelli A, Horowitz JF, Endert E, et al. Regulation of endogenous fat and carbohydrate metabolism in relation to exercise intensity and duration. Am J Physiol Endocrinol Metab. (1993) 265:E380–91. doi: 10.1152/ajpendo.1993.265.3.E380
9. Meyer JH, Doty JE. GI transit and absorption of solid food: multiple effects of guar. Am J Clin Nutr. (1988) 48:267–73. doi: 10.1093/ajcn/48.2.267
10. Hill RC. The nutritional requirements of exercising dogs. J Nutr. (1998) 128:2686S–90S. doi: 10.1093/jn/128.12.2686S
11. McClelland G, Zwingelstein G, Taylor C, Weber J. Increased capacity for circulatory fatty acid transport in a highly aerobic mammal. Am J Physiol. (1994) 266:R1280–6. doi: 10.1152/ajpregu.1994.266.4.R1280
12. McClelland G, Zwingelstein G, Taylor CR, Weber JM. Effect of exercise on the plasma nonesterified fatty acid composition of dogs and goats: species with different aerobic capacities and diets. Lipids. (1995) 30:147–53. doi: 10.1007/BF02538268
13. Weibel EW, Taylor CR, Weber J, Vock R, Roberts TJ, Hoppeler H. Design of the oxygen and substrate pathways VII. Different structural limits for oxygen supply to muscle mitochondria. J Exp Biol. (1996) 199:1699–709. doi: 10.1242/jeb.199.8.1699
14. Van der Vusse GJ, Glatz JF, Van Nieuwenhoven FA, Reneman RS, Bassingthwaighte JB. Transport of long-chain fatty acids across the muscular endothelium. Adv Exp Med Biol. (1998) 441:181–91. doi: 10.1007/978-1-4899-1928-1_17
15. Péronnet F, Nadeau RA, de Champlain J, Magrassi P, Chatrand C. Exercise plasma catecholamines in dogs: role of adrenals and cardiac nerve endings. Am J Physiol. (1981) 241:243–7. doi: 10.1152/ajpheart.1981.241.2.H243
16. Reynolds AJ, Fuhrer L, Dunlap HL, Finke MD, Kallfelz FA. Lipid metabolite responses to diet and training in sled dogs. J Nutr. (1994) 124:2754–9. doi: 10.1093/jn/124.suppl_12.2754S
17. Green HJ. How important is endogenous muscle glycogen to fatigue in prolonged exercise? Can. J Physiol Pharmacol. (1991) 69:290–7. doi: 10.1139/y91-045
18. Reynolds AJ, Hinchcliff KW, Reinhart GA. The effect of diet on performance. In: Proceedings of Performance Dog Nutrition Symposium. Dayton, OH: The Iams Company (1995).
19. Miller BF, Drake JC, Peelor FF, Biela LM, Geor R, Hinchcliff K, et al. Participation in a 1000- mile race increases the oxidation of carbohydrate in Alaskan sled dogs. J Appl Physiol. (2015) 118:1502–9. doi: 10.1152/japplphysiol.00588.2014
20. Gamble LJ, Boesch JM, Frye CM, Hansen JW, Locasale X, Liu MS, et al. Serum metabolomics of Alaskan sled dogs during endurance racing. Comp Exerc Physiol. (2018) 14:149–59. doi: 10.3920/CEP180010
21. Ermon V, Yazwinski M, Milizio JG, Wakshlag JJ. Serum chemistry and electrolyte alterations in sled dogs before and after a 1600 km race: dietary sodium and hyponatraemia. J Nutr Sci. (2014) 3:e26. doi: 10.1017/jns.2014.39
22. Hammel EP, Kronfeld DS, Ganjam VK, Dunlap HL. Metabolic responses to exhaustive exercise in racing sled dogs fed diets containing medium, low, or zero carbohydrate. Am J Clin Nutr. (1977) 30:409–18. doi: 10.1093/ajcn/30.3.409
23. McKenzie E, Holbrook T, Williamson K, Royer C, Valberg S, Hinchcliff K, et al. Recovery of muscle glycogen concentrations in sled dogs during prolonged exercise. Med Sci Sports Exerc. (2005) 37:1307–12. doi: 10.1249/01.mss.0000175086.41080.01
24. Denis C, Dormois D, Castells J, Bonnefoy R, Padilla S, Geyssant A, et al. Comparision of incremental and steady state tests of endurance training. Eur J Appl Physiol. (1988) 57:474–81. doi: 10.1007/BF00417996
25. Oshima Y, Tanaka S, Miyamoto T, Wadazumi T, Kurihara N, Fujimoto S. Effects of endurance training above the anaerobic threshold on isocapnic buffering phase during incremental exercise in middle-distance runners. Jpn J Phys Fit Sports Med. (1998) 47:43–51. doi: 10.7600/jspfsm1949.47.43
26. Alves JC, Santos A, Jorge P, Lafuente MP. Changes in physiological, haematological and biochemical parameters in police working dogs during a treadmill incremental exercise test. Comp Exerc Physiol. (2020) 16:387–349. doi: 10.3920/CEP200016
27. Templeman JR, Thornton E, Cargo-Froom C, Squires EJ, Swanson K S, Shoveller AK. Effects of incremental exercise and dietary tryptophan supplementation on the amino acid metabolism, serotonin status, stool quality, fecal metabolites, and body composition of mid-distance trained sled dogs. J Anim Sci. (2020) 98:skaa128. doi: 10.1093/jas/skaa128
28. Robinson E, Thornton E, Templeman JR, Croney CC, Niel L, Shoveller AK. Changes in behaviour and voluntary physical activity exhibited by sled dogs throughout incremental exercise conditioning and intermittent rest days. Animals. (2021) 11:118. doi: 10.3390/ani11010118
29. Furtado JD, Begari J, Campos H. Comparison of the utility of total plasma fatty acids versus those in cholesteryl ester, phospholipid, and triglyceride as biomarkers of fatty acid intake. Nutrients. (2019) 11:2081. doi: 10.3390/nu11092081
30. Nikolaidis MG, Mougios V. Effects of exercise on fatty-acid composition of blood and tissue lipids. Sports Med. (2004) 34:1051–76. doi: 10.2165/00007256-200434150-00004
31. Bauer JE, Dunbar BL, Bigley KE. Dietary flaxseed in dogs results in differential transport and metabolism of (n-3) polyunsaturated fatty acids. J Nutr. (1998) 128:2641S–4S. doi: 10.1093/jn/128.12.2641S
32. Trevizan L, Kessler AD, Brenna JT, Lawrence P, Waldron MK, Bauer JE. Maintenance of arachidonic acid and evidence of Δ5 desaturation in cats fed γ-linolenic and linoleic acid enriched diets. Lipids. (2011) 47:413–23. doi: 10.1007/s11745-011-3651-0
33. Association of American Feed Control Officials. AAFCO Manual. West Lafayette, IN: AAFCO Incorporated (2016).
34. Folch J, Lees M, Sloan Stanley GH. A simple method for the isolation and purification of total lipides from animal tissue. J Biol Chem. (1957) 226:497–509. doi: 10.1016/S0021-9258(18)64849-5
35. Gold M, Miller HI, Issekutz B Jr, Spitzer JJ. Effect of exercise and lactic acid infusion on individual free fatty acids of plasma. Am J Physiol. (1963) 205:902–4. doi: 10.1152/ajplegacy.1963.205.5.902
36. Banse HE, Sides RH, Ruby BC, Warwick MB. Effects of endurance training on VO2max and submaximal blood lactate concentrations of untrained sled dogs. Equine Comp Exerc Physiol. (2007) 4:89–94. doi: 10.1017/S1478061507811455
37. Sherman BL, Gruen ME, Case BC, Foster ML, Fish RE, Lazarowski L, et al. A test for the evaluation of emotional reactivity in Labrador retrievers used for explosives detection. J Vet Behav. (2015) 10:94–102. doi: 10.1016/j.jveb.2014.12.007
38. Hare E, Kelsey KM, Neidermeyer GM, Otto CM. Long-term behavioral resilience in search-and-rescue dogs responding to the September 11, 2001 terrorist attacks. Appl Anim Behav Sci. (2021) 234:105173. doi: 10.1016/j.applanim.2020.105173
39. Malcicka M, Visser B, Ellers J. An evolutionary perspective on linoleic acid synthesis in animals. Evol Biol. (2018) 45:15–26. doi: 10.1007/s11692-017-9436-5
40. Tallima H, Ridi R. Arachidonic acid: physiological roles and potential health benefits - a review. J Adv Res. (2017) 24:33–41. doi: 10.1016/j.jare.2017.11.004
41. Trappe TA, Liu SZ. Effects of prostaglandins and COX-inhibiting drugs on skeletal muscle adaptations to exercise. J Appl Physiol. (1985) 115:909–19. doi: 10.1152/japplphysiol.00061.2013
42. Angle CT, Wakshlag JJ, Gillette RL, Steury T, Haney P, Barrett J, et al. The effects of exercise and diet on olfactory capability in detection dogs. J Nutr Sci. (2014) 3:e44. doi: 10.1017/jns.2014.35
43. Chikamune T, Katamoto H, Ohashi F, Shimada Y. Serum lipid and lipoprotein concentrations in obese dogs. J Vet Med Sci. (1995) 57:595–8. doi: 10.1292/jvms.57.595
44. Perassolo MS, Almeida JC, Prá RL, Mello VD, Maia AL, Moulin CC, et al. Fatty acid composition of serum lipid fractions in type 2 diabetic patients with microalbuminuria. Diabetes Care. (2003) 26:613–8. doi: 10.2337/diacare.26.3.613
45. Jericó MM, De Camargo Chiquito F, Kajihara K, Moreira MA, Gonzales R, Machado FL, et al. Chromatographic analysis of lipid fractions in healthy dogs and dogs with obesity or hyperadrenocorticism. J Vet Diagn Invest. (2009) 21:203–7. doi: 10.1177/104063870902100204
46. Quehenberger O, Dennis EA. The human plasma lipidome. N Engl J Med. (2011) 365:1812–23. doi: 10.1056/NEJMra1104901
Keywords: endurance exercise, lipid metabolism, Siberian huskies, lipid fraction, lipid mobilization
Citation: Templeman JR, Trevizan L, Ma DWL and Shoveller AK (2021) Fatty Acid Profiles of Serum Lipid Fractions Change Minimally in Sled Dogs Before and After Short Bouts of Exercise. Front. Vet. Sci. 8:704770. doi: 10.3389/fvets.2021.704770
Received: 05 May 2021; Accepted: 03 August 2021;
Published: 23 August 2021.
Edited by:
Gregory C. Henderson, Purdue University, United StatesReviewed by:
Grant B. McClelland, McMaster University, CanadaPatrick Davitt, University of the Sciences, United States
Copyright © 2021 Templeman, Trevizan, Ma and Shoveller. This is an open-access article distributed under the terms of the Creative Commons Attribution License (CC BY). The use, distribution or reproduction in other forums is permitted, provided the original author(s) and the copyright owner(s) are credited and that the original publication in this journal is cited, in accordance with accepted academic practice. No use, distribution or reproduction is permitted which does not comply with these terms.
*Correspondence: Anna K. Shoveller, YXNob3ZlbGwmI3gwMDA0MDt1b2d1ZWxwaC5jYQ==