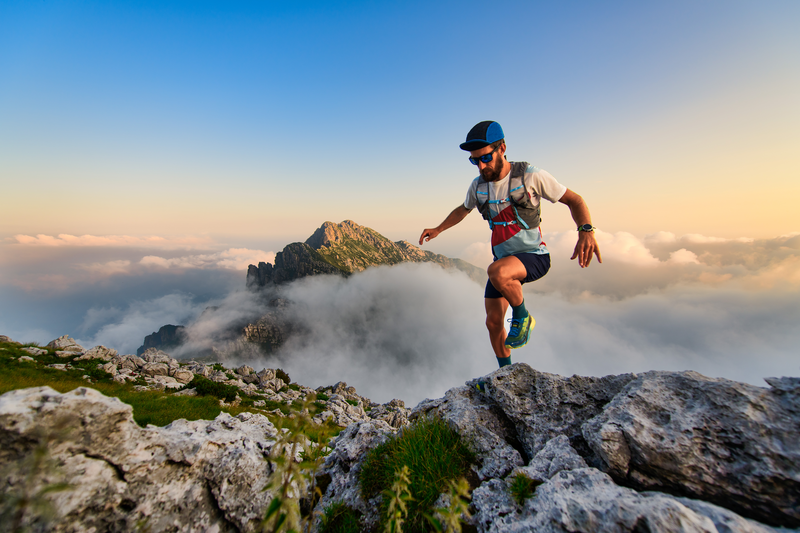
95% of researchers rate our articles as excellent or good
Learn more about the work of our research integrity team to safeguard the quality of each article we publish.
Find out more
ORIGINAL RESEARCH article
Front. Vet. Sci. , 06 August 2021
Sec. Veterinary Infectious Diseases
Volume 8 - 2021 | https://doi.org/10.3389/fvets.2021.693506
This article is part of the Research Topic Antibiotic Resistance and its Continuity in the Environmental Niche View all 19 articles
The emergence of multidrug-resistant bacteria in companion animals is an increasing concern in view of the concept of One Health. The antimicrobials linezolid (LZD) and tigecycline (TGC) are effective against multidrug-resistant bacteria isolated from humans; however, thus far, no previous study has evaluated the efficacy of these drugs against bacteria isolated from companion animals. This study aimed to evaluate the efficacy of LZD and TGC against bacteria that were isolated from companion dogs and showed resistance to all classes of antimicrobial agents. Clinical samples (auditory channel, eye, skin, and urine) were collected from dogs that visited the Veterinary Medical Teaching Hospital of Konkuk University (Seoul, South Korea) from October 2017 to September 2020. In total, 392 bacterial isolates were obtained, of which 85 were resistant to all classes of antimicrobial agents tested and were, therefore, considered potentially pan-drug resistant (PDR). The susceptibility of isolates to LZD and TGC was determined by the disk diffusion method and interpreted using the Clinical Laboratory Standards Institute guidelines. In total, 95.6% (43/45) and 97.8% (44/45) of gram-positive isolates were susceptible to LZD and TGC, respectively, whereas 82.5% (33/40) of gram-negative isolates were sensitive to TGC. In conclusion, both agents showed favorable efficacy, with the susceptibility rates for all potential PDR bacteria, except Pseudomonas spp., ranging from 72.7 to 100%. Thus, these drugs may serve as excellent antimicrobial options for veterinary medicine in the future.
The emergence of multidrug-resistant (MDR) bacteria, which are resistant to three or more categories of antimicrobials, in companion animals is highly concerning. Particularly, resistance to antimicrobials is growing among bacteria such as Staphylococcus aureus, Staphylococcus pseudintermedius, and Escherichia coli (1), which cause infections in dogs. The transmission of such bacteria can be either direct or indirect among dogs, owners, and veterinary staff. Indeed, practicing veterinarians are far more likely to experience nasal colonization with S. aureus than the general population (2). Notably, under the One Health concept, companion animals have been documented to be reservoirs of some high-risk MDR clones of Enterobacteriaceae (3), which are likely to be acquired from their human owners. Overuse of antimicrobials in veterinary clinics may amplify antimicrobial resistance and result in a subsequent spread of resistant microorganisms to animal owners. Therefore, the appropriate use of antimicrobials to prevent pain, illness, or death should be adopted for infection management in animals to improve the public health of both humans and animals.
Previous studies that analyzed the temporal trends of antimicrobial resistance in small collections of bacterial isolates from infected companion animals have provided evidence for a significant increase in antimicrobial resistance, particularly to agents frequently used in clinical settings, such as cephalosporins, ampicillins, and quinolones (4).
Linezolid (LZD) is a member of the oxazolidinone class of synthetic antibacterial agents that inhibit bacterial protein synthesis through a unique mechanism. In contrast to other inhibitors of protein synthesis, oxazolidinones act early in translation by preventing the formation of a functional initiation complex (5). Discovered in 1987 at E.I. DuPont de Nemours and Co., Inc., DuP-721 was the first well-characterized oxazolidinone (5) that exhibited strong activity against MDR gram-positive pathogens (6). In fact, it is currently used for the treatment of clinical methicillin-susceptible and methicillin-resistant S. aureus infections and for managing infections caused by vancomycin-resistant enterococci (7) in humans.
Tigecycline (TGC) is a glycylcycline antibiotic that is effective against a variety of gram-positive and gram-negative bacteria (8). TGC is currently one of the most potent antimicrobial agents for treating infections caused by MDR bacteria in humans (8). This drug has demonstrated in vitro activity against important resistant organisms, including methicillin-resistant S. aureus, penicillin-resistant Streptococcus pneumoniae, and vancomycin-resistant enterococcal species, in addition to extended-spectrum beta-lactamase-producing E. coli and Klebsiella pneumoniae (9). A previous study has shown that more than 90% of Enterobacteriaceae isolates are susceptible to this drug (10).
Nevertheless, LZD and TGC have not been used as first- or second-line treatment options in companion animals owing to concerns that the overuse and abuse of antimicrobials in animals would limit treatment options for human bacterial infections, in view of the One Health concept. Moreover, no previous study has evaluated the efficacies of LZD and TGC against bacteria originating from companion animals.
The present study aimed to evaluate the efficacies of LZD and TGC against potential pan-drug-resistant (PDR) bacteria (i.e., resistant to all classes of antimicrobial agents) isolated from dogs. Antimicrobial stewardship and related policies are beyond the scope of the current work.
A total of 359 clinical samples were collected from different lesions in dogs that visited the Veterinary Medical Teaching Hospital of Konkuk University (Seoul, South Korea) from October 2017 to September 2020. The samples were immediately placed into a transport medium (ESwab, Copan, Brescia, Italy). The sampling sites included the auditory channels, eyes/conjunctiva, gastrointestinal tract, skin/mucosa, blood, and urogenital tract (Table 1). Auditory channel, eye, and skin samples were routinely collected using sterile cotton swabs, and urine samples were collected by cystocentesis. In addition, we collected at least 2 g of feces, which were cubed to ~ to inch on a single side using a fecal loop. Peripheral blood was collected by venipuncture of the jugular vein to identify systemic infections. Furthermore, cerebrospinal fluid was obtained at the junction between lumbar vertebrae 5 and 6 using a conventional lumbar tapping method, and samples from the peritoneal walls were collected with sterile cotton swabs. All samples were immediately transported to the NosVet Laboratory (Gyeonggi-do, South Korea) and analyzed within 3–4 h.
The animal study and the protocol was reviewed and approved by the Institutional Animal Care and Use Committee (KU20218). Written informed consent was obtained from the owners for the participation of their animals in this study.
In total, 392 isolates were obtained from the dogs by directly inoculating blood agar plates with the clinical samples using cotton swabs, followed by incubation of the agar plates at 37°C for up to 24 h. Morphologically identical colonies were picked and sub-cultured onto blood agar plates, and species were identified using a matrix-assisted laser desorption/ionization mass spectrometer (ASTA, Gyeonggi-do, South Korea). Bacterial stock solutions were stored at −20°C.
Commercial antimicrobial disk diffusion tests were performed by NosVet, Inc., according to the Clinical Laboratory Standards Institute (CLSI) guidelines (VET08). Susceptibility to 21 antibiotics from 10 classes, namely, amikacin (AK), amoxicillin/clavulanic acid (AMC), ampicillin (AMP), azithromycin (AZM), cefixime (CFM), cefotaxime (CTX), cefpodoxime (CPD), ceftazidime (CAZ), cephalexin (CL), cephazolin (KZ), ciprofloxacin (CIP), clindamycin (DA), doxycycline (DO), enrofloxacin (ENR), erythromycin (E), gentamicin (CN), lincomycin (MY), ofloxacin (OFX), spiramycin (SP), sulfamethoxazole/trimethoprim (SXT), and tetracycline (TE), was determined using a Vitek® AST-P601 card (bioMérieux, Marcy l'Étoile, France).
Two additional antibiotics, namely, LZD and TGC (Thermo Fisher Scientific, Waltham, MA, USA), which are not included in the CLSI VET08 guidelines, were tested against potential PDR strains. Susceptibility to LZD and TGC was determined by the disk diffusion method and interpreted based on the CLSI guidelines. The European Committee on Antimicrobial Susceptibility Testing (EUCAST) guidelines were used when information was missing in the CLSI guidelines. Briefly, bacteria were inoculated from stock solutions onto Mueller–Hinton agar plates and incubated at 37°C for 24 h. Colonies were suspended in normal saline, and the turbidity was adjusted to a 0.5 McFarland standard equivalent (~108 colony-forming units per milliliter). Sterile cotton swabs were dipped into inoculation broth and subsequently streaked over Mueller–Hinton agar plates. Antibiotic disks of 30 μg of LZD and 15 μg of TGC were then placed on these plates, followed by incubation of the plates at 37°C for 24 h. Diameters of the inhibition zones were used to categorize bacteria as susceptible, intermediate resistant, and resistant according to the CLSI and EUCAST guidelines.
Descriptive statistics were used for the analyses of signalment, clinical data, and laboratory findings. All statistical analyses were performed using Microsoft Excel (Microsoft Corp., Redmond, WA, USA). An exact chi-square test was used to compare the efficacies of LZD, TGC, and the 21 other antibiotics against potential PDR bacteria from dogs. Differences with P-values of <0.05 were considered statistically significant.
Based on the antibiotic sensitivity evaluation conducted by NosVet, Inc., 211 bacterial isolates were classified as extensively drug resistant (XDR), as previously described (11). Of these 211 isolates, 57 were resistant to eight classes and 69 were resistant to nine classes, while 85 were resistant to all ten classes of antimicrobial agents tested and were thus considered potential PDR bacteria (11). Table 2 presents the taxonomic distribution of the 85 isolates.
According to the tests conducted by NosVet, Inc., 163 (65%) of 249 gram-positive strains showed sensitivity to AK, 131 (52.6%) were sensitive to AMC, 90 (36.1%) were sensitive to AMP, 110 (44.2%) were sensitive to AZM, 5 (2.0%) were sensitive to CFM, 89 (35.7%) were sensitive to CTX, 88 (35.3%) were sensitive to CPD, 11 (4.4%) were sensitive to CAZ, 97 (39.0%) were sensitive to CL, 114 (45.8%) were sensitive to KZ, 20 (8.0%) were sensitive to CIP, 93 (37.3%) were sensitive to DA, 124 (49.8%) were sensitive to DO, 133 (53.4%) were sensitive to ENR, 99 (39.8%) were sensitive to E, 88 (35.3%) were sensitive to CN, 8 (3.2%) were sensitive to MY, 125 (50.2%) were sensitive to OFX, 87 (34.9%) were sensitive to SP, 84 (33.7%) were sensitive to SXT, and 85 (34.1%) were sensitive to TE. Among these strains, 215 (86.3%) were MDR, 125 (50.2%) were XDR, and 45 (18.0%) were potential PDR bacteria (Supplementary File 1).
Among the potential PDR gram-positive bacteria, 95.6% (43/45) and 97.8% (44/45) of isolates were susceptible to LZD (Figure 1) and TGC (Figure 2), respectively. Superiority of LZD and TGC over the other antibiotics was statistically analyzed, and the result is presented in Tables 3, 4. Overall, the potential PDR bacteria were significantly more susceptible to these two agents than to the other 21 agents (P < 0.05). The average diameter of the zone of inhibition for LZD was 31 mm, which fell within the susceptibility zone diameter of gram-positive bacteria for LZD. The average diameter of the zone of inhibition was 24.5 mm for TGC, which exceeded the zone of resistance size.
Figure 1. Efficacy of linezolid compared to 21 antibiotics for potential pan-drug-resistant (PDR) bacteria from dogs. Circles indicate the susceptibility of gram-positive potential PDR isolates for each antibiotic. Squares indicate the average susceptibility of gram-positive potential PDR isolates.
Figure 2. Efficacy of tigecycline compared to 21 antibiotics for potential pan-drug-resistant (PDR) bacteria from dogs. Circles indicate the susceptibility of potential PDR isolates for each antibiotic. Squares indicate the average susceptibility of potential PDR isolates.
Table 3. Superiority of linezolid efficacy over 21 different antibiotics to eradicate potential pan-drug-resistant bacteria from dogs.
Table 4. Superiority of tigecycline efficacy over 21 different antibiotics to eradicate potential pan-drug-resistant bacteria from dogs.
One hundred sixty Staphylococcus spp. isolates showed sensitivity to AK (93.7%), followed by AMC (53.1%) and DA (52.4%). Of these isolates, 30 (18.7%) were potential PDR strains, and all of them were susceptible to LZD and TGC, with an average diameter of the zone of inhibition of 31.7 mm.
Forty-two Enterococcus spp. isolates showed sensitivity to AMP (17.5%), followed by AMC (16.8%). Of these isolates, 11 (26.2%) were potential PDR strains. The sensitivity of potential PDR Enterococcus faecium and Enterococcus faecalis isolates to LZD was 83.3% (5/6) and 80.0% (4/5), respectively, and the sensitivity to TGC was 83.3% (5/6) and 100% (5/5), respectively.
One Rothia nasimurium, one Streptococcus canis, and two potential PDR Corynebacterium auriscanis isolates were susceptible to LZD and TGC.
According to the tests conducted by NosVet, Inc., 105 (73.4%) of the 143 gram-negative strains showed sensitivity to AK, 68 (47.6%) were sensitive to AMC, 33 (23.1%) were sensitive to AMP, 37 (25.9%) were sensitive to AZM, 56 (39.2%) were sensitive to CFM, 61 (42.7%) were sensitive to CTX, 59 (41.3%) were sensitive to CPD, 83 (58.0%) were sensitive to CAZ, 55 (38.5%) were sensitive to CL, 49 (34.3%) were sensitive to KZ, 17 (11.9%) showed sensitivity to CIP, 4 (2.8%) were sensitive to DA, 58 (40.6%) were sensitive to DO, 64 (44.8%) were sensitive to ENR, 11 (7.7%) were sensitive to E, 79 (55.2%) were sensitive to CN, 1 (0.7%) showed sensitivity to MY, 71 (49.7%) were sensitive to OFX, 1 (0.7%) showed sensitivity to SP, 53 (37.1%) were sensitive to SXT, and 50 (35.0%) were sensitive to TE. Among these strains, 130 (90.9%) were MDR, 87 (60.8%) were XDR, and 40 (27.9%) were potential PDR bacteria (Supplementary File 1).
Of note, 82.5% (33/40) of the potential PDR gram-negative isolates were sensitive to TGC. The average inhibition zone diameter was 18.5 mm for positive isolates, which slightly exceeded the resistance cutoff. Among Enterobacteriaceae, 100% (12/12) of the E. coli strains and 72.7% (8/11) of the K. pneumoniae strains, as well as single Citrobacter freundii, Enterobacter aerogenes, and Enterobacter cloacae complex isolates, were susceptible to TGC. The overall sensitivity to TGC was 88.5% (23/26).
Fifty-two E. coli isolates showed sensitivities of 27.3% to AK and 21.0% to CN. Of these, 12 (23.0%) were potential PDR strains, as recommended by the CLSI VET08 guidelines. The average diameter of the zone of inhibition for TGC was 18.6 mm, which fell within the susceptibility zone (≥18 mm).
Nineteen isolates of K. pneumoniae showed <10% sensitivity to the 21 antibiotics, and 11 (57.8%) were potential PDR strains, which was very high compared to that in other species. Furthermore, K. pneumoniae showed the highest resistance rate (27.3%; 3/11) to TGC among the bacteria tested in this study. The inhibition zone diameters of the resistant isolates were in the range of 13–17 mm, which was within the resistance zone (≥18 mm).
Among the 31 P. mirabilis isolates, 10 were classified as potential PDR strains, and only one of these (10%; 1/10) was classified as being resistant to TGC.
P. aeruginosa isolates were less sensitive to the 21 antibiotics tested than those of other species. Only three of the 13 isolates were classified as potential PDR strains, and all three isolates (100%) were resistant to TGC, with an inhibition zone diameter of 0 mm.
One (100%) isolate of each of the following species was susceptible to TGC: C. freundii, E. aerogenes, E. cloacae complex, and an unclassified species of Pasteurellaceae.
To the best of our knowledge, no studies reported the susceptibility of S. aureus, isolated from animals, to LZD and TGC; however, some reports indicated that all human S. aureus isolates show susceptibility to these drugs (12, 13), consistent with our isolates from dog samples.
S. pseudintermedius, the most common opportunistic pathogen in dogs (14), exhibits resistance to commonly used antimicrobials (15). It is the most common pathogen causing recurrent skin infections in dogs because of allergies, endocrine diseases, or other immunocompromising factors including old age and cancer (15). The possibility of S. pseudintermedius transmission from animals to humans was evaluated in four clinical human cases, among which two dog owners and their dogs carried identical S. pseudintermedius strains (16). Although some strains can acquire methicillin resistance and cause severe refractory infections, even potential PDR strains are susceptible to LZD and TGC.
R. nasimurium and S. pseudintermedius can exhibit increased pathogenicity through synergistic effects (17); if these bacteria are potential PDR, then the associated fatality rate can significantly increase.
E. faecalis is the most frequently encountered enterococcal species in the anus and tonsils of dogs, followed by E. faecium (18). These two species are considered the third and fourth most prevalent nosocomial human pathogens worldwide, respectively (18), necessitating their control in both humans and animals. Although there is scarce evidence for susceptibility testing of Enterococcus spp. in animal samples, the sensitivity of E. faecalis and E. faecium isolates from human samples to LZD was 94.3 and 93.5%, respectively (12). In another study, the sensitivity of Enterococcus spp. isolates to TGC was 100% (201/201) (19), consistent with our results.
Oxazolidinones, including LZD, are excluded from gram-negative bacteria-related infection treatment because they enhance pump activity against LZD and expel the antibiotic from the cytoplasm, resulting in lower LZD accumulation levels in E. coli, C. freundii, and E. aerogenes than in S. aureus and E. faecium (20).
E. coli is frequently encountered and causes severe infections in both humans and dogs (21). The possible transmission of virulent and/or resistant E. coli strains between animals and humans through numerous pathways is highly concerning (21). E. coli represents a main reservoir of resistance genes probably responsible for treatment failure in both human and veterinary medicine (22). Indeed, an increasing number of resistance genes have been identified in E. coli during the past decades, mostly acquired through horizontal gene transfer (23). In the enterobacterial gene pool, E. coli acts as a donor and recipient of resistance genes from other bacteria (24). Hence, the broad-spectrum resistance of this species is quite likely, considering that most recommended antimicrobials do not effectively inhibit its growth. Therefore, TGC may broaden antimicrobial treatment choices for refractory E. coli infections.
K. pneumoniae is an important nosocomial agent that spreads easily (25) and causes community-onset infections in companion animals and humans. It is the second most common Enterobacteriaceae species causing urinary tract infections in dogs; strains are frequently MDR, posing important therapeutic limitations (26, 27). In previous studies, 84.6% (55/60) and 87.6% (340/388) of human K. pneumoniae isolates showed TGC susceptibility (19, 28). Our results showed a lower susceptibility TGC rate (73.7%), indicating the stronger resistance of isolates of animal origins to TGC. The resistance gene appears to have originated from the chromosome of a Pseudomonas species and may have been transferred to plasmids by adjacent site-specific integrases. Although the gene appears to be rare in human clinical isolates, the transferability of the gene cluster and its broad-spectrum substrate make further dissemination of this mobile TGC resistance determinant possible (29). The rapid development of TGC resistance necessitates further expansion of other treatment options.
Human Proteus spp. and P. aeruginosa isolates exhibit strong resistance to TGC. Nevertheless, we evaluated their susceptibility to TGC because strains of the same species may have different antimicrobial susceptibility profiles depending on their host of origin (30). P. mirabilis is the epitome of an opportunistic nosocomial pathogen in humans and animals (31), causing urinary tract infections (32) and chronic otitis externa (33) in companion animals. Moreover, P. mirabilis has low susceptibility to TGC (34). Moreover, a novel TGC resistance gene, tet, has recently been identified in Proteus species isolated from animals (35). Fortunately, isolates from the current study, collected over 3 years, showed sensitivity to TGC, suggesting that TGC-resistant P. mirabilis has not yet been disseminated in Korea.
P. aeruginosa is a clinically important opportunistic pathogen causing serious acute and chronic infections (36). It is ubiquitous in the environment and can persist in water and soil despite minimal nutrients, tolerating a broad spectrum of humidity and temperature conditions (37). P. aeruginosa is one of the pathogens that most frequently acquire or develop multidrug resistance (37). The exceptional array of intrinsic and acquired drug resistance mechanisms employed by P. aeruginosa renders the antibiotic-based treatment of these infections difficult. One important resistance mechanism is mediated by the resistance–nodulation–cell division family of efflux pumps (38). In one study, all (15/15) human P. aeruginosa isolates were resistant to TGC (28). Similarly, our results showed that TGC is not suitable for treating P. aeruginosa infections.
C. auriscanis was first discovered in a dog with ear infection (39) and typically acts as an opportunist in mixed infections associated with bacterial otitis externa, which can be resolved by treating and controlling other causative agents, but it may have pathological significance when occurring alone (40). C. auriscanis is often resistant to beta-lactam antibiotics; therefore, other antimicrobials may be necessary if skin lesions are not resolved after antimicrobial therapy (41).
S. canis is considered part of the healthy microbiota of the skin and mucosa of dogs but may be responsible for opportunistic infections. In dogs, S. canis is isolated from skin infections, urogenital and respiratory tract infections, otitis externa, septicemia, necrotizing fasciitis, and streptococcal toxic shock syndrome (42). Only one strain was tested in this study and found to be susceptible to LZD and TGC, indicating they are possible treatment options for S. canis infections.
E. aerogenes and E. cloacae complexes are members of the intestinal microbiota and are commonly MDR. Fortunately, both isolates were susceptible to TGC in this study, indicating the potential application of TGC for treating urinary tract infections, accounting for 38% (8/21) of animal cases, and wound infections, accounting for 19% (4/21) (43). C. freundii is intrinsically resistant to AMP, AMP/sulbactam, and cephalosporins and causes sepsis in dogs (44). A single isolate of this species was tested in this study and was susceptible to TGC; therefore, this antibiotic may be considered for treating C. freundii infections.
This study has several limitations. First, for certain bacteria (e.g., C. auriscanis, Pasteurellaceae, P. mirabilis, P. aeruginosa, and R. nasimurium), there are no susceptibility or resistance criteria. Second, many countries prohibit LZD and TGC use in animal settings to preserve treatment options for human infections. However, these agents may be employed in the future under well-organized antimicrobial stewardship frameworks and policies, and our results provide foundational knowledge for using LZD and TGC in veterinary medicine.
In conclusion, we evaluated the efficacies of TGC and LZD against potential PDR bacteria that are frequently isolated from companion dogs, and the results showed resistance of the organisms to all other antimicrobial classes recommended by the veterinary CLSI guidelines. Both agents showed favorable efficacy, with susceptibility rates of all potential PDR bacteria, except P. aeruginosa, ranging from 72.7 to 100%. Thus, TGC and LZD may serve as promising antimicrobial options for veterinary medicine in the future. For application in patients, in vivo pharmacokinetic and pharmacological studies are needed. To avoid exacerbating bacterial antibiotic resistance, legal regulations for TGC and LZD are needed to prevent their misuse.
The original contributions presented in the study are included in the article/Supplementary Material, further inquiries can be directed to the corresponding author/s.
The animal study was reviewed and approved by Institutional Animal Care and Use Committee (KU20218). Written informed consent was obtained from the owners for the participation of their animals in this study.
D-HK and J-HK conceptualized and designed the study, analyzed the data, drafted and edited the manuscript, and approved the final submission. All authors contributed to the article and approved the submitted version.
This work was supported by Korea Institute of Planning and Evaluation for Technology in Food, Agriculture and Forestry (IPET) through (Development of technology for immunomodulatory ability of useful exosomes derived from stem cells for companion animal) Project, funded by Ministry of Agriculture, Food and Rural Affairs (MAFRA)(321013-01).
The authors declare that the research was conducted in the absence of any commercial or financial relationships that could be construed as a potential conflict of interest.
All claims expressed in this article are solely those of the authors and do not necessarily represent those of their affiliated organizations, or those of the publisher, the editors and the reviewers. Any product that may be evaluated in this article, or claim that may be made by its manufacturer, is not guaranteed or endorsed by the publisher.
The Supplementary Material for this article can be found online at: https://www.frontiersin.org/articles/10.3389/fvets.2021.693506/full#supplementary-material
1. Lloyd DH. Reservoirs of antimicrobial resistance in pet animals. Clin Infect Dis. (2007) 45:S148–52. doi: 10.1086/519254
2. Jordan D, Simon J, Fury S, Moss S, Giffard P, Maiwald M, et al. Carriage of methicillin-resistant Staphylococcus aureus by veterinarians in Australia. Aust Vet J. (2011) 89:152–9. doi: 10.1111/j.1751-0813.2011.00710.x
3. Abraham S, Wong HS, Turnidge J, Johnson JR, Trott DJ. Carbapenemase-producing bacteria in companion animals: a public health concern on the horizon. J Antimicrob Chemother. (2014) 69:1155–7. doi: 10.1093/jac/dkt518
4. Normand EH, Gibson NR, Reid SW, Carmichael S, Taylor DJ. Antimicrobial-resistance trends in bacterial isolates from companion-animal community practice in the UK. Prev Vet Med. (2000) 46:267–78. doi: 10.1016/S0167-5877(00)00149-5
5. Shinabarger D. Mechanism of action of the oxazolidinone antibacterial agents. Expert Opin Investig Drugs. (1999) 8:1195–202. doi: 10.1517/13543784.8.8.1195
6. Slatter JG, Adams LA, Bush EC, Chiba K, Daley-Yates PT, Feenstra KL, et al. Pharmacokinetics, toxicokinetics, distribution, metabolism and excretion of linezolid in mouse, rat and dog. Xenobiotica. (2002) 32:907–24. doi: 10.1080/00498250210158249
7. Seixas R, Monteiro V, Carneiro C, Vilela CL, Oliveira M. First report of a linezolid-resistant MRSA (methicillin resistant Staphylococcus aureus) isolated from a dog with a severe bilateral otitis in Portugal. Rev Vet. (2011) 22:81–4. doi: 10.30972/vet.2221826
8. Sato T, Harada K, Usui M, Tsuyuki Y, Shiraishi T, Tamura Y, et al. Tigecycline susceptibility of Klebsiella pneumoniae complex and Escherichia coli isolates from companion animals: the prevalence of tigecycline-nonsusceptible K.pneumoniae complex, including internationally expanding human pathogenic lineages. Microb Drug Resist. (2018) 24:860–7. doi: 10.1089/mdr.2017.0184
9. Meagher AK, Passarell JA, Cirincione BB, Van Wart SA, Liolios K, Babinchak T, et al. Exposure–response analyses of tigecycline efficacy in patients with complicated skin and skin-structure infections. Antimicrob Agents Chemother. (2007) 51:1939–45. doi: 10.1128/AAC.01084-06
10. Hoban DJ, Reinert RR, Bouchillon SK, Dowzicky MJ. Global in vitro activity of tigecycline and comparator agents: Tigecycline evaluation and surveillance trial 2004–2013. Ann Clin Microbiol Antimicrob. (2015) 14:27. doi: 10.1186/s12941-015-0085-1
11. Falagas ME, Karageorgopoulos DE. Pandrug resistance (PDR), extensive drug resistance (XDR), and multidrug resistance (MDR) among gram-negative bacilli: need for international harmonization in terminology. Clin Infect Dis. (2008) 46:1121–2. doi: 10.1086/528867
12. Jones RN, Ballow CH, Biedenbach DJ. Multi-laboratory assessment of the linezolid spectrum of activity using the Kirby-Bauer disk diffusion method: report of the Zyvox® Antimicrobial Potency Study (ZAPS) in the United States. Diagn Microbiol Infect Dis. (2001) 40:59–66. doi: 10.1016/S0732-8893(01)00235-8
13. Sorlózano A, Gutiérrez J, Salmerón A, Luna JD, Martínez-Checa F, Román J, et al. Activity of tigecycline against clinical isolates of Staphylococcus aureus and extended-spectrum β-lactamase-producing Escherichia coli in Granada, Spain. Int J Antimicrob Agents. (2006) 28:532–6. doi: 10.1016/j.ijantimicag.2006.07.010
14. Bannoehr J, Guardabassi L. Staphylococcus pseudintermedius in the dog: taxonomy, diagnostics, ecology, epidemiology and pathogenicity. Vet Dermatol. (2012) 23:253–66.e51. doi: 10.1111/j.1365-3164.2012.01046.x
15. Pinchbeck LR, Cole LK, Hillier A, Kowalski JJ, Rajala-Schultz PJ, Bannerman TL, et al. Genotypic relatedness of staphylococcal strains isolated from pustules and carriage sites in dogs with superficial bacterial folliculitis. Am J Vet Res. (2006) 67:1337–46. doi: 10.2460/ajvr.67.8.1337
16. Lozano C, Rezusta A, Ferrer I, Pérez-Laguna V, Zarazaga M, Ruiz-Ripa L, et al. Staphylococcus pseudintermedius human infection cases in Spain: dog-to-human transmission. Vector Borne Zoonotic Dis. (2017) 17:268–70. doi: 10.1089/vbz.2016.2048
17. Bemis DA, Bryant MJ, Reed PP, Brahmbhatt RA, Kania SA. Synergistic hemolysis between β-lysin-producing Staphylococcus species and Rothia nasimurium in primary cultures of clinical specimens obtained from dogs. J Vet Diagn Invest. (2014) 26:437–41. doi: 10.1177/1040638714532098
18. European Centre for Disease Prevention and Control (ECDC). Annual epidemiological report on communicable diseases in Europe. Euro Surveill. (2011) 16:20012. doi: 10.2900/14803
19. Fernández Canigia L, Kaufman S, Lanata L, Vay C, Giovanakis M, Bantar C, et al. Multicenter study to assess the in vitro activity of tigecycline by disk diffusion test against clinical isolates from Argentina. Chemotherapy. (2009) 55:20–7. doi: 10.1159/000167788
20. Schumacher A, Trittler R, Bohnert JA, Kümmerer K, Pagès JM, Kern WV. Intracellular accumulation of linezolid in Escherichia coli, Citrobacter freundii and Enterobacter aerogenes: role of enhanced efflux pump activity and inactivation. J Antimicrob Chemother. (2007) 59:1261–4. doi: 10.1093/jac/dkl380
21. Kaper JB, Nataro JP, Mobley HLT. Pathogenic Escherichia coli. Nat Rev Microbiol. (2004) 2:123–40. doi: 10.1038/nrmicro818
22. Johnson JR, Kaster N, Kuskowski MA, Ling GV. Identification of urovirulence traits in Escherichia coli by comparison of urinary and rectal E. coli isolates from dogs with urinary tract infection. J Clin Microbiol. (2003) 41:337–45. doi: 10.1128/JCM.41.1.337-345.2003
23. Piras C, Soggiu A, Greco V, Martino PA, Del Chierico F, Putignani L, et al. Mechanisms of antibiotic resistance to enrofloxacin in uropathogenic Escherichia coli in dog. J Proteomics. (2015) 127:365–76. doi: 10.1016/j.jprot.2015.05.040
24. Chen JW, Huang HH, Chang SM, Scaria J, Chiu YL, Chen CM, et al. Antibiotic-resistant Escherichia coli and sequence type 131 in fecal colonization in dogs in Taiwan. Microorganisms. (2020) 8:1439. doi: 10.3390/microorganisms8091439
25. Martin RM, Cao J, Brisse S, Passet V, Wu W, Zhao L, et al. Molecular epidemiology of colonizing and infecting isolates of Klebsiella pneumoniae. mSphere. (2016) 1:e00261–16. doi: 10.1128/mSphere.00261-16
26. Marques C, Belas A, Franco A, Aboim C, Gama LT, Pomba C. Increase in antimicrobial resistance and emergence of major international high-risk clonal lineages in dogs and cats with urinary tract infection: 16 year retrospective study. J Antimicrob Chemother. (2018) 73:377–84. doi: 10.1093/jac/dkx401
27. Marques C, Belas A, Aboim C, Cavaco-Silva P, Trigueiro G, Gama LT, et al. Evidence of sharing of Klebsiella pneumoniae strains between healthy companion animals and cohabiting humans. J Clin Microbiol. (2019) 57:e01537–18. doi: 10.1128/JCM.01537-18
28. Samonis G, Maraki S, Karageorgopoulos DE, Vouloumanou EK, Falagas ME. Synergy of fosfomycin with carbapenems, colistin, netilmicin, and tigecycline against multidrug-resistant Klebsiella pneumoniae, Escherichia coli, and Pseudomonas aeruginosa clinical isolates. Eur J Clin Microbiol Infect Dis. (2012) 31:695–701. doi: 10.1007/s10096-011-1360-5
29. Lv L, Wan M, Wang C, Gao X, Yang Q, Partridge SR, et al. Emergence of a plasmid-encoded resistance-nodulation-division efflux pump conferring resistance to multiple drugs, including tigecycline, in Klebsiella pneumoniae. mBio. (2020) 11:1–15. doi: 10.1128/mBio.02930-19
30. European Food and Safety Authority (EFSA). Guidance on the assessment of bacterial susceptibility to antimicrobials of human and veterinary importance. EFSA J. (2012) 10:2740. doi: 10.2903/j.esfa.2012.2740
31. Harada K, Niina A, Shimizu T, Mukai Y, Kuwajima K, Miyamoto T, et al. Phenotypic and molecular characterization of antimicrobial resistance in Proteus mirabilis isolates from dogs. J Med Microbiol. (2014) 63:1561–7. doi: 10.1099/jmm.0.081539-0
32. Bubenik LJ, Hosgood GL, Waldron DR, Snow LA. Frequency of urinary tract infection in catheterized dogs and comparison of bacterial culture and susceptibility testing results for catheterized and noncatheterized dogs with urinary tract infections. J Am Vet Med Assoc. (2007) 231:893–9. doi: 10.2460/javma.231.6.893
33. Zamankhan Malayeri H, Jamshidi S, Zahraei Salehi T. Identification and antimicrobial susceptibility patterns of bacteria causing otitis externa in dogs. Vet Res Commun. (2010) 34:435–44. doi: 10.1007/s11259-010-9417-y
34. Visalli MA, Murphy E, Projan SJ, Bradford PA. AcrAB multidrug efflux pump is associated with reduced levels of susceptibility to tigecycline (GAR-936) in Proteus mirabilis. Antimicrob Agents Chemother. (2003) 47:665–9. doi: 10.1128/AAC.47.2.665-669.2003
35. Liu D, Zhai W, Song H, Fu Y, Schwarz S, He T, et al. Identification of the novel tigecycline resistance gene tet(x6) and its variants in Myroides, Acinetobacter and Proteus of food animal origin. J Antimicrob Chemother. (2020) 75:1428–31. doi: 10.1093/jac/dkaa037
36. López-de-la-Cruz J, Pérez-Aranda M, Alcudia A, Begines B, Caraballo T, Pajuelo E, et al. Dynamics and numerical simulations to predict empirical antibiotic treatment of multi-resistant Pseudomonas aeruginosa infection. Commun Nonlinear Sci Numer Simul. (2020) 91:105418. doi: 10.1016/j.cnsns.2020.105418
37. Paz-Zarza VM, Mangwani-Mordani S, Martínez-Maldonado A, Álvarez-Hernández D, Solano-Gálvez SG, Vázquez-López R. Pseudomonas aeruginosa: pathogenicity and antimicrobial resistance in urinary tract infection. Rev Chilena Infectol. (2019) 36:180–9. doi: 10.4067/S0716-10182019000200180
38. Dean CR, Visalli MA, Projan SJ, Sum PE, Bradford PA. Efflux-mediated resistance to tigecycline (GAR-936) in Pseudomonas aeruginosa PAO1. Antimicrob Agents Chemother. (2003) 47:972–8. doi: 10.1128/AAC.47.3.972-978.2003
39. Collins MD, Hoyles L, Lawson PA, Falsen E, Robson RL, Foster G. Phenotypic and phylogenetic characterization of a new Corynebacterium species from dogs: description of Corynebacterium auriscanis sp. nov. J Clin Microbiol. (1999) 37:3443–7. doi: 10.1128/JCM.37.11.3443-3447.1999
40. Henneveld K, Rosychuk RAW, Olea-Popelka FJ, Hyatt DR, Zabel S. Corynebacterium spp. in dogs and cats with otitis externa and/or media: a retrospective study. J Am Anim Hosp Assoc. (2012) 48:320–6. doi: 10.5326/JAAHA-MS-5791
41. Boynosky NA, Stokking LB. Retrospective evaluation of canine dermatitis secondary to Corynebacterium spp. J Am Anim Hosp Assoc. (2015) 51:372–9. doi: 10.5326/JAAHA-MS-6243
42. Weese JS. Investigation of Enterobacter cloacae infections at a small animal veterinary teaching hospital. Vet Microbiol. (2008) 130:426–8. doi: 10.1016/j.vetmic.2008.02.009
43. Wanger A, Chavez V, Huang RSP, Wahed A, Actor JK, Dasgupta A. Microbiology and Molecular Diagnosis in Pathology: A Comprehensive Review for Board Preparation, Certification and Clinical Practice, Microbiology and Molecular Diagnosis in Pathology. Amsterdam: Elsevier (2017). 96 p.
Keywords: linezolid, tigecycline, antimicrobial resistance, pan-drug-resistant, companion animals
Citation: Kim D-H and Kim J-H (2021) Efficacy of Tigecycline and Linezolid Against Pan-Drug-Resistant Bacteria Isolated From Companion Dogs in South Korea. Front. Vet. Sci. 8:693506. doi: 10.3389/fvets.2021.693506
Received: 11 April 2021; Accepted: 12 July 2021;
Published: 06 August 2021.
Edited by:
Marina Spinu, University of Agricultural Sciences and Veterinary Medicine of Cluj-Napoca, RomaniaReviewed by:
Mahdi Askari Badouei, Ferdowsi University of Mashhad, IranCopyright © 2021 Kim and Kim. This is an open-access article distributed under the terms of the Creative Commons Attribution License (CC BY). The use, distribution or reproduction in other forums is permitted, provided the original author(s) and the copyright owner(s) are credited and that the original publication in this journal is cited, in accordance with accepted academic practice. No use, distribution or reproduction is permitted which does not comply with these terms.
*Correspondence: Jung-Hyun Kim, anVuZ2h5dW5Aa29ua3VrLmFjLmty
Disclaimer: All claims expressed in this article are solely those of the authors and do not necessarily represent those of their affiliated organizations, or those of the publisher, the editors and the reviewers. Any product that may be evaluated in this article or claim that may be made by its manufacturer is not guaranteed or endorsed by the publisher.
Research integrity at Frontiers
Learn more about the work of our research integrity team to safeguard the quality of each article we publish.