- 1Faculty of Veterinary Medicine, University of Teramo, Teramo, Italy
- 2Faculty of Bioscience and Agro-Food and Environmental Technology, University of Teramo, Teramo, Italy
- 3Department of Veterinary Medical Sciences, Alma Mater Studiorum University of Bologna, Bologna, Italy
The aim of this study was to evaluate the effects of basal dietary supplementation with linseed and linseed plus vitamin E in Marchigiana young bulls on the instrumental color, oxidative stability, and fatty acid composition of beef steaks with and without treatment with a blend of essential oils (oregano and rosemary) after storage times of 0, 3, 6, and 9 days. The fatty acid composition was evaluated in meat after 0 and 9 days of storage. No variation in the fatty acid composition of each type of meat was observed after storage. The use of dietary linseed and vitamin E, compared with linseed alone, significantly improved the oxidative stability, lightness (L*), and redness (a*) of the meat. A higher degree of oxidation was observed in meat samples from animals in the group fed linseed (L) followed by the control group (C) and the group fed linseed and vitamin E (LE). Moreover, the essential oils treatment (O) exerts a significant effect on beef oxidative stability of the group LE more than groups C and L during storage. The fatty acid composition of the meat was also affected by the diet: levels of healthful fatty acids (PUFA, n-3 PUFA, and CLA) were higher in meat from the groups that received linseed than the control group. Our results suggest that dietary vitamin E and treatment with essential oils are effective approaches to preserving the stability of beef cattle receiving a diet enriched in unsaturated fatty acids for up to 9 days of storage.
Introduction
In Italy, the first meat that received the Protected Geographical Indication from the European Community was the “white beef of the Central Apennines” (1), which includes fresh meat from the three cattle breeds in central Italy: Chianina, Marchigiana, and Romagnola, which are, together with Piemontese, the main specialized breeds for meat production in Italy, and the quality of their products is widely recognized all over the world (2). In particular, Marchigiana husbandry techniques involve pasture in the summer and a stalling period during winter. In the finishing period, the animals are usually kept in stalls, and the fattening system is based on concentrates rich in corn and soybean meal, which improve the meat's fatty acid profile by conferring a high proportion of linoleic acid, thus increasing the n-6-to-n-3 ratio (3, 4).
The use of linseed to fatten bullocks has been demonstrated to be effective in increasing the percentage of n-3 fatty acids in the intramuscular fat (5). Recent years have seen considerable interest in the dietary supplementation of ruminants with polyunsaturated fatty acids (PUFAs), because of the positive effects of these molecules on human health, such as decreasing low-density lipoprotein and cholesterol, and conferring anti-inflammatory, anti-atherogenic, and anti-carcinogenic effects (6).
Several studies have reported favorable effects of dietary linseed supplementation on different ruminant products, such as milk, cheese, and meat (7–9). However, the natural enrichment of meat with PUFAs could potentially enhance meat's susceptibility to lipid oxidation and rancid taste (10), thereby altering meat sensorial properties and shelf life (11).
To overcome these adverse effects, a useful approach to improve meat oxidative stability and shelf life could involve endogenous or exogenous antioxidants. Consumers prefer antioxidants from natural rather than synthetic sources (12), because of the potential toxicity of the latter. Essential oils (EOs) are natural compounds extracted from officinal plants with antimicrobial and antioxidant properties; therefore, EOs have attracted interest as additives in the food industry (13). Experimental studies have shown that the antimicrobial potential is greater when a mixture of EOs, rather than single EO, is used (14, 15).
EOs from oregano (Origanum vulgare L.) and rosemary (Rosmarinus officinalis L.) have potential as natural food antioxidants (14, 16). Oregano and rosemary EOs are recognized as safe, and the final products meet consumer sensory acceptability requirements (17).
The aim of this study was to evaluate the effect of linseed and vitamin E dietary supplementation in Marchigiana beef cattle on the instrumental color, oxidative stability, and fatty acid composition of steaks with and without treatment with a blend of EOs from oregano and rosemary, after different storage times.
Materials and Methods
Animals and Diets
The Marchigiana young bulls were managed during the experiment according to the European Union (18), concerning the protection of animals kept for farming purposes, and were slaughtered according to the The Council of the European Union (19) of September 24, 2009, on the protection of animals at the time of killing.
The experiment was conducted at a commercial farm ~15 km northeast of Teramo, Abruzzo, Southern Italy. A total of 36 Marchigiana young bulls (440.7 ± 1.8 kg weight and 12 months of age) were allotted to three experimental groups according to body weight, such that the mean weight of each group was comparable.
Animals were stratified by weight and divided into three homogeneous groups that received the following diets during the finishing period of ~45 days: group C (control diet), group L (linseed), and group LE (linseed + vitamin E). Samples of total mixed ration (TMR) were collected every week and analyzed according to the standard methods of AOAC (20) for dry matter (DM), crude protein (CP), ether extract (EE), and ash. Neutral detergent fiber (NDF), acid detergent fiber (NDF), and acid detergent lignin (ADL) were determined as described by Van Soest et al. (21). All feed samples were also analyzed for FA composition as described by Lock et al. (22). The ingredients and chemical compositions of the diets are shown in Table 1. The dry matter intake was monitored by group and was calculated daily as the difference between the amount of feed offered and that refused. All animals were individually weighed every week to determine the average daily gain (ADG).
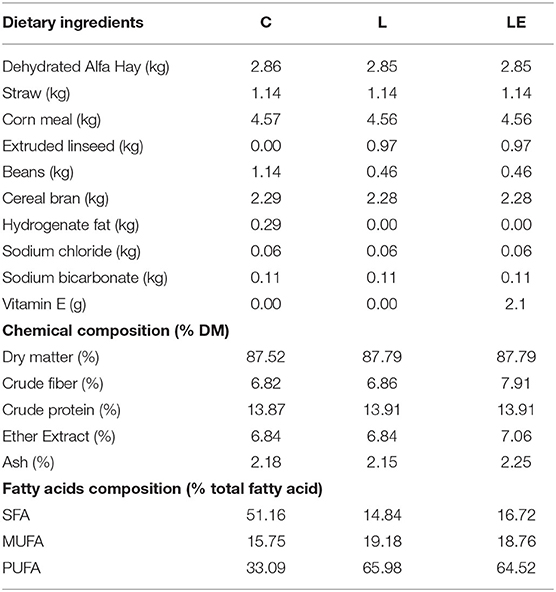
Table 1. Ingredients of the three experimental diets: C (control), L (linseed), and LE (linseed + vitamin E).
Carcass Traits
At the end of the finishing period, the young bulls were transported to an abattoir 30 min away. At the abattoir, the animals were weighed and slaughtered according to EU Regulations with a captive-bolt pistol and dressed according to commercial practice. The hot carcass weight (HCW) was recorded. The carcasses were graded for conformation and fatness by an expert evaluator according to the SEUROP classification (23). The SEUROP system defines six classes in order to classify carcasses according to their muscle conformation. The S class is “superior”; the E class is “excellent”; the U class is “very good”; the R class is “good”; the O class is “fair”; and the P class is “poor.” M. longissimus dorsi pH was measured in situ at 45 min post mortem (pH1) before entry into the cooling room, at the right side between the 5th and the 6th lumbar vertebrae (24), with a penetrating electrode adapted to a portable pHmeter (Crison pHmeter 507 and a 52–32 spear electrode, Crison Instruments, Spain). The carcasses were then cooled at 4°C for 24 h, and the final pH (pH24) was determined in situ with the same instruments on the same muscle. At 24 h, the carcasses were weighed (cold carcass weight). The dressing percentage was calculated as the ratio of the cold carcass weight to the final live weight. After 48 h in the cooling room, the carcasses were transferred to the laboratory for dissection under refrigerated conditions.
Preparation of Steak Tray Samples
Eight steaks 25 mm thick from the left longissimus dorsi muscle between the last rib and the 6th lumbar vertebra of each carcass were aseptically cut and placed on a 17.5 × 25.5 × 4.0 cm polystyrene tray. Four steaks were treated by dipping into a blend of rosemary and oregano (1:1) EOs [0.5 ml rosemary + 0.5 ml oregano + 999 ml saline phosphate buffer (PBS) per kg of meat] for 60 s, and the rest were dipped in PBS solution as a control. PBS is isotonic and non-toxic. All samples (n = 288) underwent modified atmosphere packaging (66% O2; 25% CO2; 9% N2) and were then stored at +4°C for 9 days and sampled for the subsequent analysis.
Whole trays were placed in a dark chamber at 4°C and then removed from the chamber after 0, 3, 6, and 9 days for the following analyses: pH, instrumental color (lightness, L*; redness, a*; and yellowness, b*) and thiobarbituric acid reactive substances (TBARS). Ferric reducing antioxidant power (FRAP), vitamin E content and fatty acid profiles were determined at days 0 and 9 of storage.
Meat Quality: pH and Color Measurements
The pH of the meat samples was measured with a penetrating electrode adapted to a portable pH meter (Crison pHmeter 507 and a 52–32 spear electrode, Crison Instruments, Spain). Steak samples from each treatment during storage (0, 3, 6, and 9 days) were subjected to pH recording and were allowed to bloom in direct contact with air for 1 h before colorimetric measurements. At the same time point, meat color parameters (lightness, L*; redness, a*; and yellowness, b*) were measured on steak samples according to the CIELab system with a Minolta Chroma Meter CR-300 (Minolta Camera Co., Osaka, Japan) with a D65 illuminant and an 8-mm aperture. Color measurements were performed on steak surfaces and are reported as the means of three measurements.
Thiobarbituric Acid Reactive Substance Value
The lipid oxidation of meat samples was evaluated by TBARS measurement with the method of Inserra et al. (25) at 0, 3, 6, and 9 days of storage. Three replicates were run for each sample. Briefly, aliquots of 2.5 g of meat samples were homogenized with 12.5 ml of distilled water in a water/ice bath. Then, 12.5 ml of trichloroacetic acid (10% w/v) was added to precipitate proteins, and the samples were vortexed. The homogenates were filtered (Whatman No. 1), and 4 ml of filtrate was added to 1 ml of 0.06 M aqueous thiobarbituric acid in screw cap tubes. The tubes were kept in a water bath at 80°C for 90 min, and the absorbance of each sample was read at 532 nm with a UV spectrophotometer. TBARS values were calculated from a standard curve with different concentrations of TEP (1,1,3,3-tetraethoxypropane) and are expressed as milligrams of malonaldehyde (MDA)/kg of meat.
Ferric Reducing Antioxidant Power Method
The spectrophotometric FRAP method was used for antioxidant capacity determination according to Szydłowska-Czerniak et al. (26). The FRAP reagent−2.5 ml of a 10 mmol/L TPTZ solution in 40 mmol/L HCl, 2.5 ml of 10 mmol/L FeCl3, and 25 ml of 0.1 mol/L acetate buffer (pH 3.6)—was prepared freshly and incubated at 40°C for 15 min. Then, 0.1–0.3 ml of methanolic extracts of meat samples and 2 ml of FRAP reagent were transferred into a 10-ml volumetric flask, and the volume was adjusted up to 10 ml with redistilled water. The blue solutions obtained were kept at room temperature for 10 min and centrifuged at 10,000 rpm for 10 min. The absorbance was measured at 593 nm against a reagent blank (2 ml of FRAP reagent adjusted to 10 ml with redistilled water). The results are expressed as μmol Trolox equivalent/g dw. Analyses were performed in triplicate on each extract.
Vitamin E Analysis
Muscle vitamin E (α-tocopherol) concentrations were measured according to Franco et al. (27): 0.8 g of meat was minced and dissolved in 10 ml of methanol; then, internal standard (60 μg DL-α-tocopheryl acetate 98%) and 2 mg of BHT (butylhydroxytoluene) were added to avoid oxidation. The tube was vortexed for 30 s and then centrifuged at 4,000 g for 10 min at 20°C. Two milliliters of supernatant was removed and filtered through a 0.20-μm filter 13 mm in diameter. Aliquots of 10 μl were injected with an Alliance 2695 high-performance liquid chromatograph (Waters, Milan, Italy). A C18 (150 mm × 4.6 mm i.d., Atlantis®) column with a 5-μm particle size (Waters, Milan, Italy) was used. The mobile phase was HPLC-grade methanol acidified with 0.1% trifloracetic acid, at a flow rate of 1.4 ml/min. A 2,475 fluorescence detector (Waters, Milan, Italy) with 295 nm and 325 nm excitation and emission wavelengths, respectively, was used. Integration of peak areas of vitamin E was performed in Empower 2™ advanced software (Waters, Milan, Italy). The concentration of α-tocopherol was expressed as μg/g of fresh muscle. Standard solutions of α-tocopherol, 0.08–4.0 μg/ml, were prepared to obtain a calibration curve.
Fatty Acid Profile
Briefly, intramuscular lipids were extracted according to the protocol of Folch et al. (28). After cold methylation of FAs with the technique of Frega and Lerker (29), the FA profile was determined at day 0 and 9 days of storage from extracts by gas chromatography with a Chrompack CP-SIL 88 capillary column (100 m length, 0.2 m film thickness, 0.25 mm inner diameter), from 160°C (1 min) to 175°C (4°C/min) for a total of 28 min, and then from 175 to 215°C (55°C/min) for 35 min with the injector at 250°C and the ionized flame detector at 260°C. Before statistical analysis, the data on FA composition were processed to calculate the following FA classes and indices: MUFAs (FAs with a single double bond), PUFAs (FAs with more than one double bond); SFAs (FAs without double bonds); n-3 (Σ n-3: sum of C18:3n-3, C20:5n-3, C22:5n-3 and C22:6n-3); and n-6 (Σn-6: sum of C18:2n-6t9,t12, C18:2n-6, CLAt10,c12, C18:3n-6, C20:3n-6, C20:4n-6 and C22:4n-6). The I-Harris index (30) was calculated as the sum of EPA and DHA, and the peroxidability index (PI) was calculated according to the following equation (31): (% dienoic acid × 1) + (% trienoic acid × 2) + (% tetraenoic acid × 3) + (% pentaenoic acid × 4) + (% hexaenoic acid × 5). FA quantities were expressed as mg FA/100 g sample.
Statistical Analysis
Data were analyzed with GLM procedure of the SPPS software package for Windows (SPSS ver. 18; SPSS Inc., Chicago, USA), including the fixed effects of dietary treatment, use of essential oils and storage time and interactions between main effects. Results for interactions are not reported because they were not significant. Duncan's test was used to assess significant differences between means.
P < 0.05 was considered the threshold for significant differences. Results are presented as treatment mean and standard error of the mean.
Results
Animal Performance and Carcass Traits
The mean initial body weights were 441.9, 438.8, and 440.1 kg, and the mean final body weights were 516.0, 515.3, and 520.2 kg for C, L, and LE, respectively (Table 2). Dietary treatments did not affect the final live weights. The ADG of the animals was 1.66 kg/day for the animals in group C and 1.71 kg/day and 1.77 kg/day for the animals in group L and LE, respectively. Feed intake (kg/day) was similar among dietary groups (8.9, 9.1, and 9.3 kg/day for C, L, and LE, respectively).
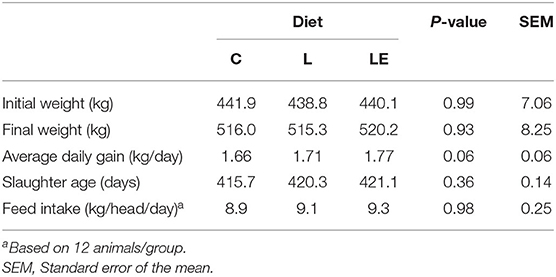
Table 2. Effect of the experimental diets C (Control), L (Linseed), and LE (linseed + vitamin E) on Marchigiana beef in vivo performance.
Carcass traits are presented in Table 3. The carcass weight, dressing percentage and carcass classification (conformation and fat cover) did not show differences among experimental groups. According to conformation, all carcasses were assigned to class U (very good). The dietary treatment did not influence pH1 and pH24. Nevertheless, the animals that received supplementation with linseed had a similar dressing percentage to that in the animals in group C.
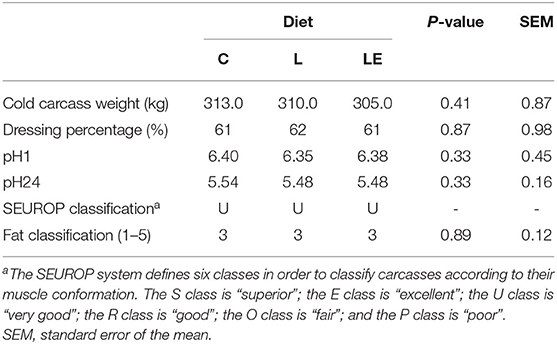
Table 3. Effect of the experimental diets C (Control), L (Linseed), and LE (linseed + vitamin E) on Marchigiana beef carcass traits.
Meat Quality Traits: pH and Color Parameters
The storage time and EO treatment significantly affected meat pH (Figure 1). The mean pH values were significantly lower in meat treated with EOs (O) after 6 and 9 days of storage (5.62 and 5.69, respectively) than in the samples without oils (WO) (5.70 and 5.76, respectively).
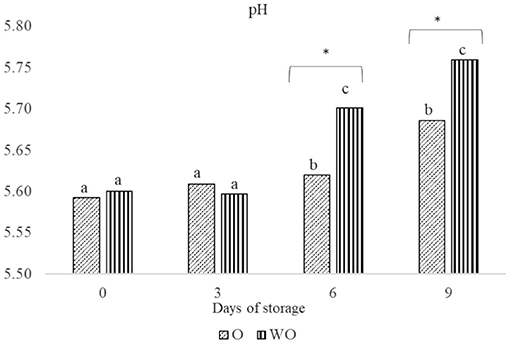
Figure 1. Effect of treatment with (O) and without (WO) essential oils on Marchigiana beef meat pH during storage time (0, 3, 6, and 9 days). Different letters on the top of the bars denote significant differences (P < 0.05) between experimental groups on days of storage. Asterisk denotes significant differences in the same group between the meat with (O) and without (WO) essential oils (P < 0.05).
Table 4 presents the meat color parameters (L*, a*, and b*) of meat from three experimental groups with or without EO treatment. The L values decreased in all experimental groups, with storage time, and between the O and WO samples. In particular, in group C, the significant differences between the O and WO meat were evident from 3 days of storage, while in group L, it was evident at time 0. In contrast, in the LE group, we observed a significantly higher value of L* in O meat than WO meat only at 9 days of storage. We observed the same trend for a*. Indeed, the a* values decreased with storage time in all dietary treatments, and the values were significantly higher at time 0 and lower after 9 days of storage (P < 0.05). The meat treated with EO showed a higher value of a* than the samples without EO at 6 (P < 0.05) and 9 days (P < 0.05) of storage in the C and L groups. In contrast, in the LE group, the redness value differed in O vs. WO meat samples only after 9 days of storage (P < 0.05). The b* values showed significant differences among storage times. No differences were observed between the O and WO samples.
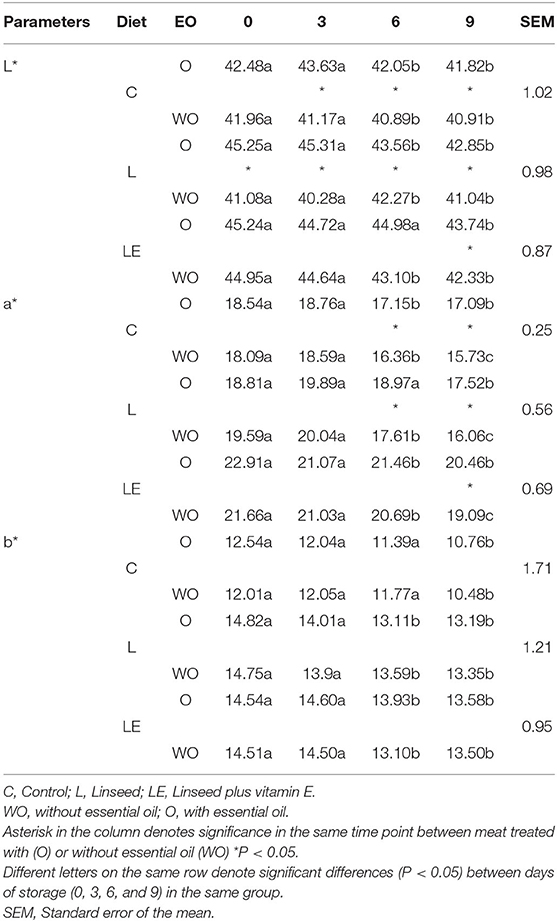
Table 4. Effects of time (0, 3, 6, and 9 days of storage), diets (C, L, and LE), and essential oils (O and WO) on Marchigiana beef meat color parameters (L*, a*, and b*).
Figures 2A,B show the differences among the C, L, and LE groups at the same storage time. The lightness values were higher in the L and LE groups treated with O at 0 and 3 days of storage, than in the group C that showed the lowest value for each of these time points (P < 0.05). The lightness of C and L groups WO was lower for all the time points compared to group LE (P < 0.05). At 6 days of storage, we observed a significantly higher (P < 0.05) value of L* in the LE group treated with EO than that in the C group, whereas L had an intermediate value. At 9 days, the L* values were lower in C and L (P < 0.05) than in LE. The L* values of WO meat samples were higher in the LE group than the L and C groups (P < 0.05) at all storage times.
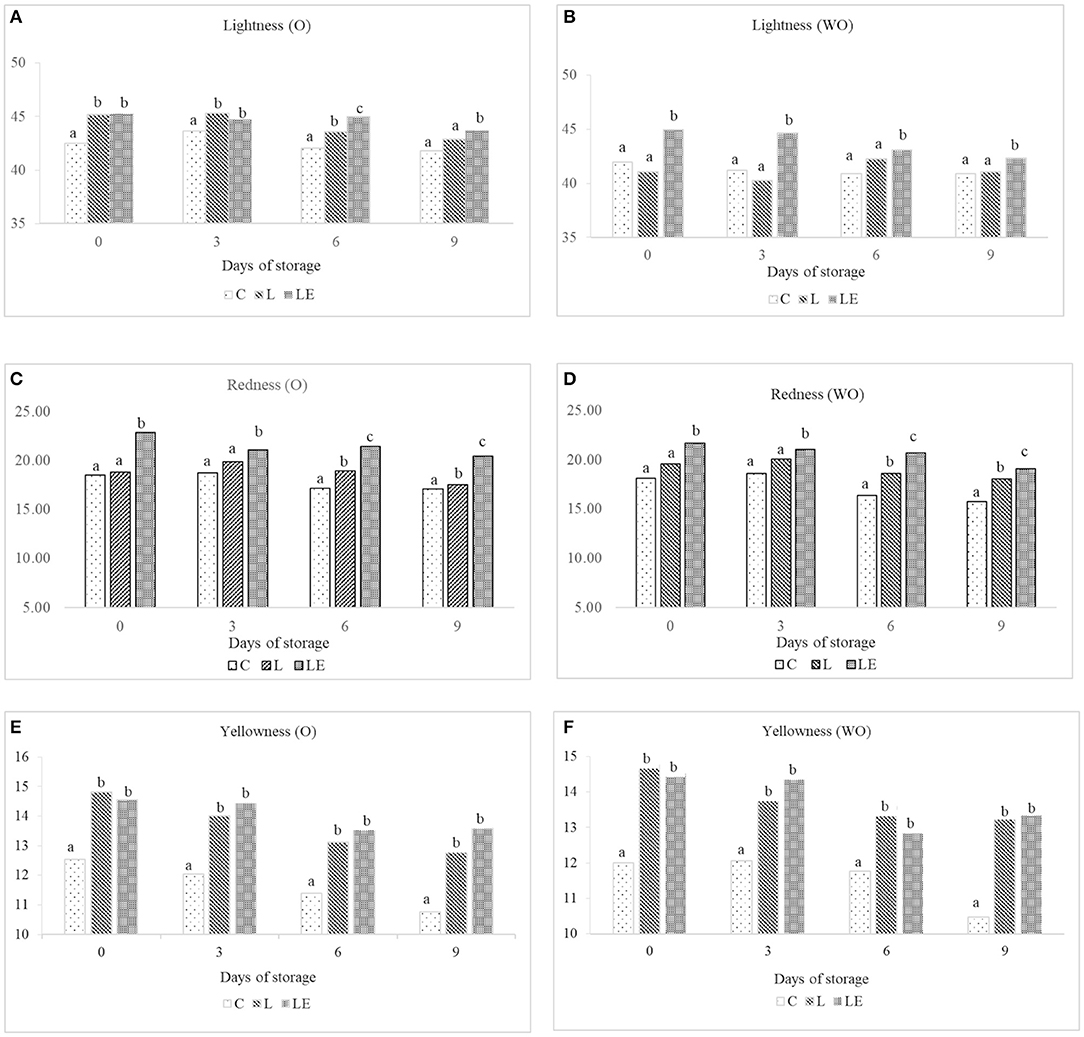
Figure 2. Effect of the experimental diets (C, L, and LE) on Lightness, L* (A,B) redness, a* (C,D), and yellowness, b* (E,F) of Marchigiana beef meat treated with (O) and without essential oils (WO) during the storage time. C, Control; L, Linseed; LE, Linseed and vitamin E. Different letters on the top of the bars denote significant differences (P < 0.05) between groups during days of storage.
The a* (Figure 2C) differed significantly (P < 0.05) from days 0 to 9 between the experimental groups treated with EO. In particular, at time 0, a higher value of a* was recorded for LE than C and L (P < 0.05). The same trend was observed on day 3. At 6 and 9 days of storage, a* (P < 0.05) was significantly higher in LE than L, whereas the lowest value was recorded in C. We observed the same trend in WO meat samples (Figure 2D). The b* values showed significant differences between dietary treatments at day 0 and during storage in L and LE compared with C (Figure 2E). The same trend was found in WO meat samples (Figure 2F).
Meat Oxidative Stability
In O meat samples, no significant differences were found between dietary treatments for TBARS on days 0 and 3 of storage (Figure 3). On days 6 and 9 of storage, TBARS were significantly lower (P < 0.05) in the LE group (0.36 mg MDA/kg and 0.38 mg MDA/kg, respectively) than in the C and L groups (0.51 and 0.62 mg MDA/kg, respectively, on day 6; 0.72 and 0.80 mg MDA/kg, respectively, on day 9). In contrast, in WO meat samples, the TBARS values were significantly higher in C and L than LE from day 3. On days 6 and 9, the TBARS values were higher in L than C and LE (P < 0.05).
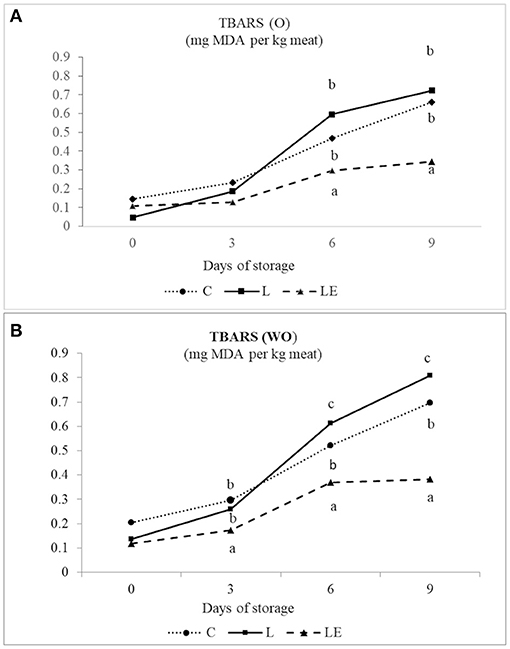
Figure 3. Effect of experimental diets (C, L, and LE) on TBARS concentration in Marchigiana beef meat treated with (O) (A) and without essential oils (WO) (B) during the storage time.
The FRAP values showed no differences between the dietary groups and for O and WO samples on day 0 of storage (data not shown). In contrast, on day 9, LE O samples had higher values than O and WO samples in the C and L groups (P < 0.05) (Figure 4). In all groups, the FRAP values were significantly different between the O and WO samples (P < 0.05).
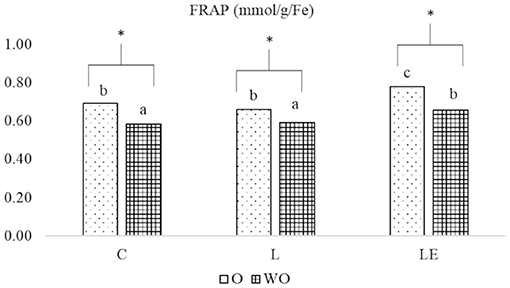
Figure 4. Effect of experimental diets (C, L, and LE) on FRAP concentration in Marchigiana beef meat treated with (O) and without essential oils (WO) at 9 days of storage. Different letters on the top of the bars denote significant differences (P < 0.05) between different dietary treatments (C, L, and LE). Asterisk denotes significant differences in the same group between the meat with (O) and without (WO) essential oils.
The dietary content of vitamin E was significantly higher in the LE group (P < 0.01) than in the C and L groups at day 0 (data not shown) and at day 9 of storage (Figure 5). The use of EOs resulted in significantly higher vitamin E content in the C and L groups.
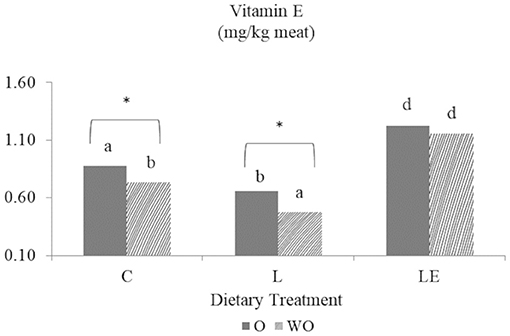
Figure 5. Effect of experimental diets (C, L, and LE) on Vitamin E concentration on Marchigiana beef meat treated with (O) and without essential oils (WO) at 9 days of storage. Different letters on top of bars denote significant differences (P < 0.05) between different dietary treatments (C, L, and LE). Asterisk denotes significant differences in the same group between the meat with (O) and without essential oils (WO).
Fatty Acid Profile
The FA composition of intramuscular fat is shown in Table 5. Dietary linseed supplementation significantly increased the content of PUFA (245.93 and 249.25 mg/100 g of sample, respectively, in L and LE, as compared with 201.94 mg/100 g of sample C), while SFA had an opposite trend, showing the higher value for group C (865.49 mg/100 g of sample) compared to L (753.87 mg/100 g of sample) and LE (742.18 mg/100 g of sample). L and LE also showed a higher content of n-3 PUFA than C, although these FAs were higher in LE (63.77 mg/100 g of sample) than L (47.41 mg/100 g of sample) (P < 0.05). The total n-6 PUFA was affected by the inclusion of dietary linseed, which were lower (P < 0.05) in L and LE than in C. The meat from L and LE, compared with C, had higher percentages (P < 0.05) of EPA and DHA (I-Harris index) (9.03 and 8.90 mg/100 g sample in L and LE, respectively, and 7.74 mg/100 g of sample in C). In contrast, the CLA value was higher in the LE group (14.47 mg/100 g of meat) and lower in the C group (5.02 mg/100 g of sample (P < 0.05), whereas the L group had an intermediate value (10.56 mg/100 g sample) between those of LE and C. In contrast, the PI was higher in LE than C, whereas L showed an intermediate value between those of C and LE (P < 0.05). On day 9, a significantly higher content of SFA was observed in WO meat, while PUFA, n-3, and n-6 were found higher in meat treated with O than WO meat samples.
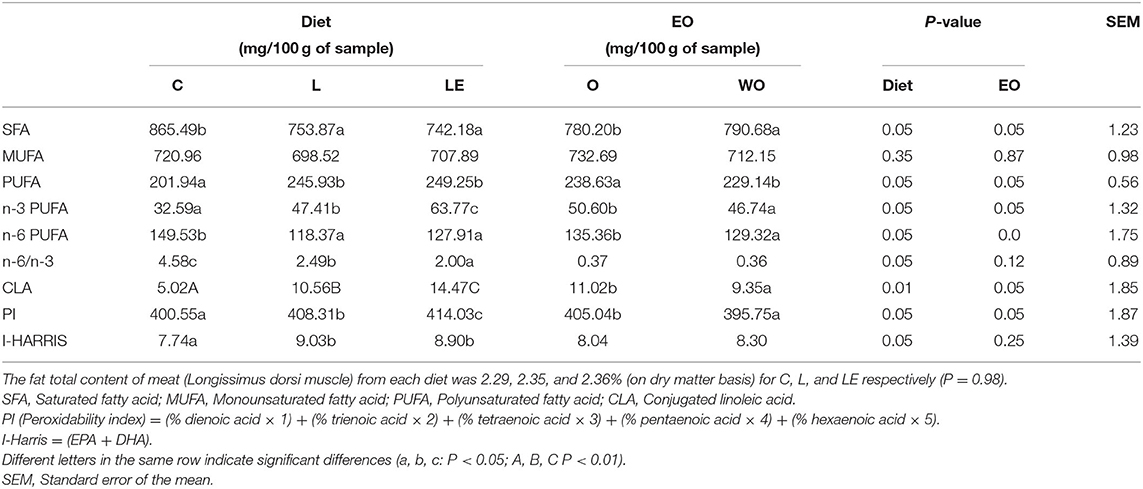
Table 5. Effects of experimental diets (C, L, and LE) and treatment (EO) with (O) and without essential oils (WO) on health indices and pro-oxidant fatty acids on Marchigiana beef meat at 9 days of storage.
Discussion
Animal Performance and Carcass Traits
The experimental diets did not significantly affect growth performance, in agreement with findings from other studies performed on finishing steers fed vegetable oilseeds (32–34). The lack of effect of linseed supplementation on animal performance may be ascribed to the similar energetic and protein content and to the similar dry matter intake. Generally, growth performance parameters depend primarily on breed, slaughter weight, age, and sex; because the animals were all of the same breed, age, and sex, it is plausible that there were no differences in growth performance (8). Similar results have been found by Kim et al. (35) in a study on the use of whole flaxseed in Hanwoo steers.
The ADG in the present study was slightly higher than the value observed by Mattii et al. (32) in Marchigiana beef (final weights ~755.0 kg); this effect may be ascribed to the different weights at slaughter.
Carcass traits did not show differences between treatment groups; in the present study, a lower dressing percentage was found than that reported by Pezzi et al. (36), probably because of the different ages and weights at slaughter. The dressing percentage increases with slaughter weight (8).
Carcass pH24 values showed no differences among the three experimental groups, ranging between 5.48 and 5.54; these values were within the normal range, as reported by Rousset and Renerre (37).
Meat Quality Traits: pH and Color Parameters
The pH of meat packaged in a modified atmosphere in O and WO samples after storage under refrigerated temperature, is given in Figure 1. The significant increase in pH in all groups during storage may be attributed to microbial metabolism of secondary substrates after the depletion of glucose. According to Gill (38), after exhaustion of stored glucose, bacteria use amino acids released during protein breakdown, thus leading to the liberation of ammonia and amines, and consequently increasing the pH. Although the pH increased during storage, we observed significant differences between the O and WO samples. The O meat samples had a lower pH at days 6 and 9 of storage, probably because of the protective effect of EOs against substrate decomposition, as described by Ribeiro et al. (13).
Beef color parameters during the storage period indicated that color was influenced by the type of diet, the storage time, and the use of EOs.
As shown in Table 4, the L* value decreased (P < 0.05) gradually in all experimental groups during storage and slightly differed depending on the presence or absence of EO treatment. In the group L, the differences between O and WO were evident from time 0, while from day 3 of storage, this difference was also evidenced in groups C and L, whereas the difference in the LE group was evident at 9 days of storage between the O and WO samples. Several studies (39–41) have reported higher L* values for steak after supplementation of feedlot cattle with vitamin E and flaxseed rather than flaxseed alone. Our results suggest that the use of dietary vitamin E and the use of EO treatment of meat may protect the color stability of the meat more than the use of EOs alone. Our findings are also consistent with those of Arnold et al. (42), who confirmed that supplementation with α-tocopheryl acetate enhances color stability in beef, because α-tocopherol is closely associated with phospholipids in cell membranes (43). The L* values varied significantly among the three dietary treatments, but with increasing storage time, the L* decreased for all dietary treatments (Figures 2A,B). Other authors have reported that fat modification of meat from animals fed diets rich in oils is associated with high L* of the finished product (44, 45).
Therefore, the presence of vitamin E in meat enriched with PUFAs increases stability against oxidation and makes the meat more desirable (46).
The a* decreased with increasing storage time for all dietary treatments from day 0 to 9, and the lowest value was observed at day 9 of storage (Table 4). The discoloration of red meat cuts is generally associated with oxidation of the iron atom within the heme group in red oxymyoglobin to brownish metamyoglobin, and it depends on the presence of reducing systems and on lipid oxidation (11). Moreover, at 9 days of storage, the a* value of LE was significantly higher in group O compared to WO. According to Zhang et al. (47), this result is probably due to the phenolic compounds of EOs, which can regenerate endogenous tocopherol in the phospholipid bilayers of lipoproteins. Moreover, the stabilizing effect of natural antioxidants on color has been described in other studies with various antioxidants from ingredients such as avocado (48) and rosemary and lemon balm (49).
In contrast, Juárez et al. (50) have reported that a dietary regimen with flaxseed and vitamin E did not preserve the color parameters in patties during storage. In the ground beef, in contrast to the steak used in the present research, cellular integrity is likely to be disrupted, owing to greater exposure of the tissues to oxygen and simultaneous dilution of antioxidant concentrations (51). The b* was influenced by the storage time and the different dietary treatments (Table 4). The b* value was higher in L and LE than C, at all storage times (Figures 2E,F). This finding agrees with results from Gomez et al. (45), who have found that the color parameters of meat, particularly yellowness, are closely associated with the color properties of the raw materials used in the diet formulation. In all groups on days 6 and 9, the b* values had decreased significantly; in contrast, some authors (27, 52) have found that an increase in b* reflects degradation of beef meat quality and is notably positively correlated with the sensory degradation of color, an increase in microbial count, fat oxidation, and other negative effects.
Meat Oxidative Stability
To predict the oxidative stability of lipids in meat samples, we used TBARS tests. Values above 2 mg/kg of meat are considered critical, because they indicate a level of lipid oxidation products that can produce a rancid smell and taste that can be detected by consumers (53). Figures 3A,B show that the TBARS values were significantly influenced by the diet, storage time, and use of EOs. The results showed a higher degree of oxidation in meat samples from animals in the L group, followed by the C group and LE group. These results were evident for both O and WO samples. The use of EO treatment in meat samples in group L and LE resulted in lower lipid oxidation at day 6 than that in WO samples. The lipid oxidation increased in groups C and L after 3 days of storage. These results differ from those reported by Rojas and Brewer (54), in which beef patties treated with rosemary EO showed significantly higher TBARS content than controls on day 4 of storage. The presence of salt probably accelerated lipid oxidation. Moreover, minced meat develops high levels of oxidation, because fatty acids are exposed to pro-oxidants, such as iron released from muscle cells, and to the effects of greater bacterial degradation (55).
These results suggest that the use of EOs in beef steak may be a favorable strategy to limit the oxidation process in meat, owing to their high concentrations of phenolic compounds (56), carnosic acid, carnosol, rosmarinic acid, caffeic acid, and flavonoids that are responsible for antioxidant activity (57, 58).
Our results are in accordance with those of Juárez et al. (50), who have shown a decrease in TBARS concentrations after 3 days of storage in beef from animals fed flaxseeds and vitamin E, and a significant increase in these substances in meat obtained from animals supplemented exclusively with flax seeds.
The literature also highlights the combined effects of using rosemary and oregano EOs. Rosemary extract is highly antioxidant and therefore is widely used in the food industry (13). Several authors have reported the efficacy of rosemary in decreasing lipid oxidation in various foods (59, 60). Oregano extract is effective in inhibiting peroxidation through the initial neutralization of free radicals, subsequent blocking of peroxidation and finally chain-breaking activity (61).
Our FRAP results confirmed the findings of Berdahl and McKeague (58) and Rodríguez-Rojo et al. (60), who have reported a high ability of EOs to prevent oxidation. Moreover, the antioxidant capacity of meat treated with EOs remained high during storage, as compared with that of samples not treated with EO, thus indicating that the active antioxidant compounds of rosemary and oregano are able to maintain antioxidant activity over time.
Vitamin E content, as expected, was higher in LE and lower in L, whereas C showed an intermediate value. Because α-tocopherols rapidly neutralize oxidation (62), we presumed that the higher and easily oxidizable substrate (PUFA) content in L required a greater expenditure of vitamin E. Moreover, Dal Bosco et al. (63) demonstrated that meat from rabbits fed linseed required a higher expenditure of vitamin E, probably due to the ability of the vitamin E to rapidly counteract the oxidation as described by Burton and Traber (62). Vitamin E 2.1 g/head/day added to the diet of Marchigiana beef cattle at ~518.0 kg BW is adequate to obtain greater oxidative stability in the meat and consequently a longer shelf life of the product.
Fatty Acids Profile
Results obtained at day 0 were compared with results obtained at 9 days to estimate the fatty acid profile evolution. Indeed, no variation in fatty acid profiles was observed after storage in all experimental groups (data not shown). These findings are consistent with those of Douny et al. (64), who have reported no differences in FA profiles in pork meat after 6 weeks of +4°C storage. The results of our study showed that the LE and L groups had similar concentrations of PUFAs, but the n-3 values were lower in L than LE meat samples. These results are probably due to the effect of vitamin E on the rumen microbiota. As suggested by Juárez et al. (46), vitamin E can shift in rumen PUFA biohydrogenation toward production of trans-11 C18:1 rather than trans-10 C18 through the action of Butirivibrio fibrisolvens, which is responsible for the hydrogenation of linoleic acid and linolenic acid to vaccenic acid (65). In contrast, Schäfers et al. (66) have reported that the use of the same quantity of vitamin E in the diet is unable to change the outflow of fatty acids from the rumen in Holstein dairy cows, as compared with dairy cows receiving a diet without vitamin E. Our results clearly showed that higher levels of PUFAs with higher concentrations of α-tocopherol in beef caused an increase in n-3 PUFA and CLA. Another possible explanation for the lower levels of n-3 and CLAs in the meat in group L may be that the oxidation of these FAs matched more in group L than LE. We hypothesize that vitamin E protected this FA in the LE group.
The beef n-6/n-3 FA ratio was higher in C than L and LE, thus demonstrating how dietary linseed decreases this ratio to the recommended value, lower than the threshold of 4.0, in beef meat (67). This result occurred for two reasons: the higher content of n-3 FA originating from extruded linseed integration and the decrease in n-6 concentration, in line with previous works (68, 69). The same results were found by Mordenti et al. (70) in fresh meat obtained from crossbred heifers fed with linseed.
EPA (20:5 n-3) and DHA (22:6 n-3) fatty acids are very important PUFAs recognized for their beneficial effects on the cardiovascular system and on the brain and visual system during fetal development and throughout life (71). The lower concentrations of SFAs, higher I-Harris index values (sum of EPA and DHA), and higher n-3PUFAs in groups L and LE than group C are important results in terms of the benefits to human health. However, the content of n-3 PUFA and CLAs was higher in LE than in L. These discrepancies probably occurred in response to the addition of vitamin E with linseed in the LE group different to the group that received only linseed. Indeed, vitamin E may counteract the shift in the biohydrogenation pathways by supporting the increase of the same fatty acid like CLA and same PUFA with a mechanism action that is not well-known (72). The peroxidation index was higher in LE than in L, despite the same concentration of linseed being used in the diet. This result was not surprising, given the higher value of long-chain fatty acids with double bonds in LE than L and C. The role of vitamin E was probably fundamental in preserving the concentrations of long-chain fatty acids over time to a greater extent than those in C and L. This result is consistent with findings reported by Jerónimo et al. (73), in which a higher PI in meat of lambs supplemented with oil was characterized by a higher content of PUFA. Indeed, in our study, the PI was calculated for meat after 9 days of storage and not on fresh meat. For this reason, the higher value of this index in LE can be considered favorable from a nutritional point of view.
The diet also affected CLA concentrations in beef meat in the three experimental groups, resulting in higher values in groups L and LE than C. These findings are consistent with results reported in the literature: diets rich in linoleic and linolenic acids are well-known to increase the levels of C18:1 t11 acid in meat as a product of rumen biohydrogenation, owing to stearoyl CoA desaturase, which converts vaccenic acids to the corresponding CLA 9c,11t isomer, the main CLA isomer, during the bio-hydrogenation process (74).
The role of vitamin E on the FAs composition of the beef meat was really fundamental. As explained before, vitamin E, on the one hand, protects beef meat PUFAs against peroxidation and, on the other hand, is able to affect the biohydrogenation pathways of dietary PUFAs in the rumen and consequently indirectly improving the CLA composition of meat products (75). However, other authors have not found any evidence of an effect on the fatty acid composition of beef meat by using a high dosage of vitamin E (50).
Conclusion
Dietary supplementation with linseed significantly influenced the concentrations of PUFAs. Moreover, feeding a diet with linseed and vitamin E significantly affected the content of n-3 and CLA in meat. Our data also showed that EOs appear to protect unsaturated fatty acids against lipid oxidation for up to 9 days of storage. The use of vitamin E at 2.1 g/head/day in the diets of Marchigiana young bulls effectively decreased meat lipid oxidation for up to 9 days of storage in a modified atmosphere. The oxidative stability of the meat was affected by the use of dietary vitamin E and meat treatment with a blend of rosemary and oregano EOs, which enhanced the positive natural antioxidant effects during storage.
Data Availability Statement
The raw data supporting the conclusions of this article will be made available by the authors, without undue reservation.
Ethics Statement
Ethical review and approval was not required for the animal study because, this study was conducted within the normal breeding activities of the farm and the animals were slaughtered at the end of their productive cycle as indicated by the Protected Geographical Indication of White Beef of the Central Apennines. Written informed consent was obtained from the owners for the participation of their animals in this study.
Author Contributions
ACM and MM performed the laboratory work. DC, LMEM, and LL performed data analysis. IF and MG wrote the manuscript. GV and AF assisted with editing the manuscript. All authors have read and agreed to the final version of the manuscript.
Funding
This present study had been carried out in the framework of the Rural Development Programme 2007–2013, Abruzzo Region, Measure 1.2.4. Cooperation for the development of new products, technologies in the agriculture and food sector and in the forestry sector and of the Project Demetra (Dipartimenti di Eccellenza 2018–2022, CUP_C46C18000530001), funded by the Italian Ministry for Education, University and Research.
Conflict of Interest
The authors declare that the research was conducted in the absence of any commercial or financial relationships that could be construed as a potential conflict of interest.
Acknowledgments
The authors also thank the Azienda Agricola San Lorenzo Sardinara (Teramo) for farm support.
References
1. The Commission of the European Communities. Commission regulation (EC) No. 134/98 of 20 January 1998 supplementing the Annex to Regulation (EC) No. 1107/96 on the registration of geographical indications designations of origin under the procedure laid down in Article 17 of Council Regulation (EEC) No. 2081/92. Off J Eur Commun L. (1998) 15:6–7.
2. Lasagna E, Ceccobelli S, Di Lorenzo, P, Albera A, Filippini F, Sarti FM, et al. Comparison of four italian beef cattle breeds by means of functional genes. Ital J Anim Sci. (2015) 14:86–9. doi: 10.4081/ijas.2015.3465
3. Insausti K, Beriain MJ, Alzueta MJ, Carr TR, Purroy A. Lipid composition of the intramuscular fat of beef from spanish cattle breeds stored under modified atmosphere. Meat Sci. (2004) 66:639–46. doi: 10.1016/S0309-1740(03)00182-7
4. Blanco M, Casasús I, Ripoll G, Panea B, Albertí P, Joy M. Lucerne grazing compared with concentrate-feeding slightly modifies carcase and meat quality of young bulls. Meat Sci. (2010) 84:545–52. doi: 10.1016/j.meatsci.2009.10.010
5. Scollan ND, Dhanoa MS, Choi NJ, Maeng WJ, Enser M, Wood JD. Biohydrogenation and digestion of long chain fatty acids in steers fed on different sources of lipid. J Agric Sci. (2001) 136:345–55. doi: 10.1017/S0021859601008796
6. Wang Y, Jacome-Sosa MM, Proctor SD. The role of ruminant trans fat as a potential nutraceutical in the prevention of cardiovascular disease. Food Res Int. (2012) 46:460–8. doi: 10.1016/j.foodres.2011.08.019
7. Caroprese M, Marzano A, Marino R, Gliatta G, Muscio A, Sevi A. Flaxseed supplementation improves fatty acid profile of cow milk. J Dairy Sci. (2010) 93:2580–8. doi: 10.3168/jds.2008-2003
8. Albertí P, Gómez I, Mendizabal JA, Ripoll G, Barahona M. Sarriés, et al. Effect of whole linseed and rumen-protected conjugated linoleic acid enriched diets on feedlot performance, carcass characteristics, and adipose tissue development in young Holstein bulls. Meat Sci. (2013) 94:208–14. doi: 10.1016/j.meatsci.2013.01.015
9. Fusaro I, Giammarco M, Odintsov Vaintrub M, Chincarini M, Manetta AC, Mammi LME, et al. Effects of three different diets on the fatty acid profile and sensory properties of fresh pecorino cheese “Primo sale.” Asian-Australas J Anim Sci. (2020) 33:1991–8. doi: 10.5713/ajas.19.0452
10. Ponnampalam EN, Sinclair AJ, Hosking BJ, Egan AR. Effects of dietary lipid type on muscle fatty acid composition, carcass leanness, and meat toughness in lambs. J Anim Sci. (2002) 80:628–36. doi: 10.2527/2002.803628x
11. Faustman C, Sun Q, Mancini R, Suman SP. Myoglobin and lipid oxidation interactions: mechanistic bases and control. Meat Sci. (2010) 86:86–94. doi: 10.1016/j.meatsci.2010.04.025
12. Moure A, Cruz JM, Franco D, Dominguez JM, Sineiro J, Dominguez H, et al. Natural antioxidants from residual sources. Food Chem. (2001) 72:145–71. doi: 10.1016/S0308-8146(00)00223-5
13. Ribeiro JS, Santos MJMC, Silva LKR, Pereira LCL, Santos IA, da Silva Lannes SC, et al. Natural antioxidants used in meat products: a brief review. Meat Sci. (2019) 148:181–8. doi: 10.1016/j.meatsci.2018.10.016
14. Burt S. Essential oils: their antibacterial properties and potential applications in foods–a review. Int J Food Microbiol. (2004) 94:223–53. doi: 10.1016/j.ijfoodmicro.2004.03.022
15. Paparella A, Taccogna L, Aguzzi I, Chaves-López C, Serio A, Marsilio F, et al. Flow cytometric assessment of the antimicrobial activity of essential oils against listeria monocytogenes. Food Control. (2008) 19:1174–82. doi: 10.1016/j.foodcont.2008.01.002
16. Horbańczuk OK, Kurek MA, Atanasov AG, Brnčić M, Rimac Brnčić S. The effect of natural antioxidants on quality and shelf life of beef and beef products. Food Technol. Biotechnol. (2019) 57:439–47. doi: 10.17113/ftb.57.04.19.6267
17. Manhani MR, Nicoletti MA, Barretto ACDS, De Jesus GR, Camila Munhoz C, De Abreu GR, et al. Antioxidant action of rosemary and oregano extract in pre-cooked meat hamburger. Food Nutr Sci. (2018) 09:806–17. doi: 10.4236/fns.2018.97060
18. European Union. Council directive (EC) No. 98/58/EC of 20 July 1998 concerning the protection of animals kept for farming purposes. Off J Eur Commun L. (1998) 221:23–27.
19. The Council of the European Union. Council regulation (EC) no. 1099/2009 of 24 September 2009 on the protection of animals at the time of killing. Off J Eur Union L. (2009) 303:1–30.
20. AOAC. Official Methods of Analysis. 16th Edn. Arlington, VA: Association of Official Analytical Chemists (2002).
21. Van Soest PJ, Robertson JB, Lewis BA. Methods for dietary fiber, neutral detergent fiber, and non-starch polysaccharides in relation to animal nutrition. J Dairy Sci. (1991) 74:3583–97. doi: 10.3168/jds.S0022-0302(91)78551-2
22. Lock AL, Teles BM, Perfield JW, Bauman DE, Sinclair LA. A conjugated linoleic acid supplement containing trans-10, cis-12 reduces milk fat synthesis in lactating sheep. J Dairy Sci. (2006) 89:1525–32. doi: 10.3168/jds.S0022-0302(06)72220-2
23. European Union. Commission delegated regulation (EU) 2017/1182 of 20 April 2017 supplementing regulation (EU) No 1308/2013 of the European parliament of the council as regards the Union scales for the classification of beef, pig sheep carcasses as regards the reporting of market prices of certain categories of carcasses live animals. Off J Eur Union L. (2017) 171:74–99.
24. Scientific Association of Animal Production. Methods for the Assessment of Quality Characteristics of Meat. Perguia, Italy: Università degli Studi di Perugia (1996).
25. Inserra L, Priolo A, Biondi L, Lanza M, Bognanno M, Gravador R, et al. Dietary citrus pulp reduces lipid oxidation in lamb meat. Meat Sci. (2014) 96:1489–93. doi: 10.1016/j.meatsci.2013.12.014
26. Szydłowska-Czerniak A, Tułodziecka A, Szłyk E. Determination of antioxidant capacity of unprocessed and processed food products by spectrophotometric methods. Food Anal. Methods. (2012) 5:807–13. doi: 10.1007/s12161-011-9314-1
27. Franco D, González L, Bispo E, Latorre A, Moreno T, Sineiro J, et al. Effects of calf diet, antioxidants, packaging type and storage time on beef steak storage. Meat Sci. (2012) 90:871–80. doi: 10.1016/j.meatsci.2011.10.008
28. Folch J, Lees M, Sloane Stanley GH. A simple method for the isolation and purification of total lipides from animal tissues. J Biol Chem. (1957) 226:497–509. doi: 10.1016/S0021-9258(18)64849-5
29. Frega N, Lerker G. Rapid methods for the quality control of food oils. Riv Ital Sostanze Gr. (1984) 61:385–91.
30. Harris WS, Assaad B, Poston WC. Tissue omega-6/omega-3 fatty acid ratio and risk for coronary artery disease. Am J Cardiol. (2006) 98:19i−26i. doi: 10.1016/j.amjcard.2005.12.023
31. Witting LA. Lipid peroxidation in vivo. J Am Oil Chem Soc. (1965) 42:908–13. doi: 10.1007/BF02632443
32. Mattii S, Priori S, Trombetta MF. Influence of sunflower cake supplementation on marchigiana carcass and meat quality. Ital J Anim Sci. (2009) 8:513–5. doi: 10.4081/ijas.2009.s2.513
33. Corazzin M, Bovolenta S, Sepulcri A, Piasentier E. Effect of whole linseed addition on meat production and quality of italian simmental and holstein young bulls. Meat Sci. (2012) 90:99–105. doi: 10.1016/j.meatsci.2011.06.008
34. Conte G, Serra A, Casarosa L, Ciucci F, Cappucci A, Bulleri E, et al. Effect of linseed supplementation on total longissimus muscle lipid composition and shelf-life of beef from young maremmana bulls. Front Vet Sci. (2019) 5:326. doi: 10.3389/fvets.2018.00326
35. Kim C, Kim J, Oh Y, Park E, Ahn G, Lee G, et al. Effects of flaxseed diets on performance, carcass characteristics and fatty acid composition of hanwoo steers. Asian-Australas J Anim Sci. (2009) 22:1151–159. doi: 10.5713/ajas.2009.90096
36. Pezzi P, Martino G, Simone N, Fusaro I, Giammarco M, Lambertini L. Feeding dehydrated alfalfa increases polyunsaturated fatty acids concentration in marchigiana beef muscle. Ital J Anim Sci. (2005) 4:269–71. doi: 10.4081/ijas.2005.2s.269
37. Rousset S, Renerre M. Effect of CO2 or vacuum packaging on normal and high pH meat shelf-life. Int J Food Sci Technol. (1991) 26:641–52. doi: 10.1111/j.1365-2621.1991.tb02009.x
38. Gill CO. Meat spoilage and evaluation of the potential storage life of fresh meat. J Food Prot. (1983) 46:444–52. doi: 10.4315/0362-028X-46.5.444
39. Houben JH, van Dijk A. Effects of dietary vitamin e supplementation and packaging on the colour stability of sliced pasteurized beef ham. Meat Sci. (2001) 58:403–7. doi: 10.1016/S0309-1740(01)00042-0
40. Realini CE, Duckett SK, Brito GW, Dalla Rizza M, De Mattos D. Effect of pasture vs. concentrate feeding with or without antioxidants on carcass characteristics, fatty acid composition, and quality of Uruguayan beef. Meat Sci. (2004) 66:567–77. doi: 10.1016/S0309-1740(03)00160-8
41. LaBrune HJ, Reinhardt CD, Dikeman ME, Drouillard JS. Effects of grain processing and dietary lipid source on performance, carcass characteristics, plasma fatty acids, and sensory properties of steaks from finishing cattle. J Anim Sci. (2008) 86:167–72. doi: 10.2527/jas.2007-0011
42. Arnold RN, Arp SC, Scheller KK, Williams SN, Schaefer DM. Tissue equilibration and subcellular distribution of vitamin e relative to myoglobin and lipid oxidation in displayed beef. J Anim Sci. (1993) 71:105–18. doi: 10.2527/1993.711105x
43. Kagan VE, Quinn PJ. The interaction of alpha-tocopherol and homologues with shorter hydrocarbon chains with phospholipid bilayer dispersions. A fluorescence probe study. Eur J Biochem. (1988) 171:661–7. doi: 10.1111/j.1432-1033.1988.tb13837.x
44. Martinez B, Miranda J, Vazquez B, Fente C, Franco C, Rodriguez J, et al. Development of a hamburger patty with healthier lipid formulation and study of its nutritional, sensory, stability properties. Food Bioprocess Technol. (2012) 5:200–8. doi: 10.1007/s11947-009-0268-x
45. Gomez I, Sarries MV, Ibanez FC, Beriain MJ. Quality characteristics of a low-fat beef patty enriched by polyunsaturated fatty acids and vitamin D3. J Food Sci. (2018) 83:454–63. doi: 10.1111/1750-3841.14043
46. Juárez M, Dugan MER, Aalhus JL, Aldai N, Basarab JA, Baron VS, et al. Dietary vitamin e inhibits the trans 10-18:1 shift in beef backfat. Can J Anim Sci. (2010) 90:9–12. doi: 10.4141/CJAS09088
47. Zhang W, Xiao S, Samaraweera H, Lee EJ, Ahn DU. Improving functional value of meat products. Meat Sci. (2010) 86:15–31. doi: 10.1016/j.meatsci.2010.04.018
48. Rodríguez-Carpena JG, Morcuende D, Estévez M. Avocado by-products as inhibitors of color deterioration and lipid and protein oxidation in raw porcine patties subjected to chilled storage. Meat Sci. (2011) 89:166–73. doi: 10.1016/j.meatsci.2011.04.013
49. Lara MS, Gutierrez JI, Timón M, Andrés AI. Evaluation of two natural extracts (Rosmarinus officinalis L. and Melissa officinalis L.) as antioxidants in cooked pork patties packed in MAP. Meat Sci. (2011) 88:481–8. doi: 10.1016/j.meatsci.2011.01.030
50. Juárez M, Dugan MER, Aldai N, Basarab JA, Baron VS, McAllister TA, et al. Beef quality attributes as affected by increasing the intramuscular levels of vitamin e and omega-3 fatty acids. Meat Sci. (2012) 90:764–9. doi: 10.1016/j.meatsci.2011.11.010
51. Hultin HO. Potential lipid oxidation problems in fatty fish processing. In: Davis N, editor. Fatty Fish Utilization: Upgrading from Feed to Food. Raleigh, NC: University of North Carolina Sea Grant College Program (1988). p. 185–223.
52. Insausti K, Beriain MJ, Lizaso G, Carr TR, Purroy A. Multivariate study of different beef quality traits from local spanish cattle breeds. Animal. (2008) 2:447–58. doi: 10.1017/S1751731107001498
53. Campo MM, Nute GR, Hughes SI, Enser M, Wood JD, Richardson RI. Flavour perception of oxidation in beef. Meat Sci. (2006) 72:303–11. doi: 10.1016/j.meatsci.2005.07.015
54. Rojas MC, Brewer MS. Effect of natural antioxidants on oxidative stability of cooked, refrigerated beef and pork. J. Food Sci. (2007) 72:S282–8. doi: 10.1111/j.1750-3841.2007.00335.x
55. Riley PA, Enser M, Nute GR, Wood JD. Effects of dietary linseed on nutritional value and other quality aspects of pig muscle and adipose tissue. Anim Sci. (2000) 71:483–500. doi: 10.1017/S1357729800055454
56. Karre L, Lopez K, Getty KJK. Natural antioxidants in meat and poultry products. Meat Sci. (2013) 94:220–7. doi: 10.1016/j.meatsci.2013.01.007
57. Thorsen MA, Hildebrandt KS. Quantitative determination of phenolic diterpenes in rosemary extracts: aspects of accurate quantification. J Chromatogr A. (2003) 995:119–25. doi: 10.1016/S0021-9673(03)00487-4
58. Berdahl DR, McKeague J. Rosemary sage extracts as antioxidants for food preservation. In: Shahidi F, editor. Handbook of Antioxidants for Food Preservation. Cambridge, UK: Woodhead publishing. (2015). p. 177–217. doi: 10.1016/B978-1-78242-089-7.00008-7
59. Sánchez-Escalante A, Djenane D, Torrescano G, Beltrán JA, Roncalés P. The effects of ascorbic acid, taurine, carnosine and rosemary powder on colour and lipid stability of beef patties packaged in modified atmosphere. Meat Sci. (2001) 58:421–9. doi: 10.1016/S0309-1740(01)00045-6
60. Rodríguez-Rojo S, Varona S, Núñez M, Cocero MJ. Characterization of rosemary essential oil for biodegradable emulsions. Ind. Crops Prod. (2012) 37:137–40. doi: 10.1016/j.indcrop.2011.11.026
61. Cervato G, Carabelli M, Gervasio S, Cittera A, Cazzola R, Cestaro B. Antioxidant properties of oregano (Origanum vulgare) leaf extracts. J Food Biochem. (2000) 24:453–65. doi: 10.1111/j.1745-4514.2000.tb00715.x
62. Burton GW, Traber MG. Vitamin e: antioxidant activity, biokinetics, and bioavailability. Annu Rev Nutr. (1990) 10:357–82. doi: 10.1146/annurev.nu.10.070190.002041
63. Dal Bosco A, Mattioli S, Cullere M, Szendro Z, Gerencsér Z, Matics Z, et al. Effect of diet and packaging system on the oxidative status and polyunsaturated fatty acid content of rabbit meat during retail display. Meat Sci. (2018) 143:46–51. doi: 10.1016/j.meatsci.2018.04.004
64. Douny C, Khoury RE, Delmelle J, Brose F, Degand G, Moula N, et al. Effect of storage and cooking on the fatty acid profile of omega-3 enriched eggs and pork meat marketed in Belgium Food Sci Nutr. (2015) 3:140–52. doi: 10.1002/fsn3.197
65. Klieve AV, Hennessy D, Ouwerkerk D, Forster RJ, Mackie RI, Attwood GT. Establishing populations of Megasphaera elsdenii YE 34 and Butyrivibrio fibrisolvens YE 44 in the rumen of cattle fed high grain diets. J Appl Microbiol. (2003) 95:621–30. doi: 10.1046/j.1365-2672.2003.02024.x
66. Schäfers S, Meyer U, von Soosten D, Krey B, Hüther L, Tröscher A, et al. Influence of vitamin e on organic matter fermentation, ruminal protein and fatty acid metabolism, protozoa concentrations and transfer of fatty acids. J Anim Physiol Anim Nutr. (2018) 102:1111–9. doi: 10.1111/jpn.12929
67. Department of Health. Report on Health and Social Subjects No. 46. Nutritional Aspects of Cardiovascular Disease. London: HMSO (1994).
68. Scollan ND, Dannenberger D, Nuernberg K, Richardson I, MacKintosh S, Hocquette J-F, et al. Enhancing the nutritional and health value of beef lipids and their relationship with meat quality. Meat Sci. (2014) 97:384–94. doi: 10.1016/j.meatsci.2014.02.015
69. Vossen E, Claeys E, Raes K, van Mullem D, De Smet S. Supra-nutritional levels of α-tocopherol maintain the oxidative stability of n-3 long-chain fatty acid enriched subcutaneous fat and frozen loin, but not of dry fermented sausage. J Sci Food Agric. (2016) 96:4523–30. doi: 10.1002/jsfa.7668
70. Mordenti AL, Brogna N, Merendi F, Formigoni A, Sardi L, Cardenia V, et al. Effect of dietary inclusion of different lipid supplements on quality and oxidative susceptibility of beef meat. Ital J Anim Sci. (2018) 18:105–10. doi: 10.1080/1828051X.2018.1485517
71. Vannice G, Rasmussen H. Position of the academy of nutrition and dietetics: dietary fatty acids for healthy adults. J Acad Nutr Diet. (2014) 114:136–53. doi: 10.1016/j.jand.2013.11.001
72. Pottier J, Focant M, Debier C, De Buysser G, Goffe C, Mignolet E, et al. Effect of dietary vitamin e on rumen biohydrogenation pathways and milk fat depression in dairy cows fed high-fat diets. J Dairy Sci. (2006) 89:685–92. doi: 10.3168/jds.S0022-0302(06)72131-2
73. Jerónimo E, Soldado D, Sengo S, Francisco A, Fernandes F, Portugal APV, et al. Increasing the α-tocopherol content and lipid oxidative stability of meat through dietary Cistus ladanifer L. in lamb fed increasing levels of polyunsaturated fatty acid rich vegetable oils. Meat Sci. (2020) 164:108092. doi: 10.1016/j.meatsci.2020.108092
74. Griinari JM, Corl BA, Lacy SH, Chouinard PY, Nurmela KVV, Bauman DE. Conjugated linoleic acid is synthesized endogenously in lactating dairy cows by Δ9-desaturase. J Nutr. (2000) 130:2285–91. doi: 10.1093/jn/130.9.2285
Keywords: Marchigiana, muscle color, vitamin E, essential oils, meat, TBARS
Citation: Fusaro I, Cavallini D, Giammarco M, Manetta AC, Martuscelli M, Mammi LME, Lanzoni L, Formigoni A and Vignola G (2021) Oxidative Status of Marchigiana Beef Enriched in n-3 Fatty Acids and Vitamin E, Treated With a Blend of Oregano and Rosemary Essential Oils. Front. Vet. Sci. 8:662079. doi: 10.3389/fvets.2021.662079
Received: 31 January 2021; Accepted: 22 April 2021;
Published: 08 June 2021.
Edited by:
Claudio Forte, University of Turin, ItalyReviewed by:
Nikola Puvača, University Business Academy in Novi Sad, SerbiaEmiliano Lasagna, University of Perugia, Italy
Copyright © 2021 Fusaro, Cavallini, Giammarco, Manetta, Martuscelli, Mammi, Lanzoni, Formigoni and Vignola. This is an open-access article distributed under the terms of the Creative Commons Attribution License (CC BY). The use, distribution or reproduction in other forums is permitted, provided the original author(s) and the copyright owner(s) are credited and that the original publication in this journal is cited, in accordance with accepted academic practice. No use, distribution or reproduction is permitted which does not comply with these terms.
*Correspondence: Melania Giammarco, mgiammarco@unite.it