- 1Department of Animal Reproduction and Artificial Insemination, Veterinary Research Division, National Research Center, Cairo, Egypt
- 2Department of Veterinary Clinical Sciences, College of Veterinary Medicine, Iowa State University, Ames, IA, United States
- 3Department of Pharmacology, Faculty of Veterinary Medicine, Cairo University, Cairo, Egypt
- 4Department of Biomedical Sciences, College of Veterinary Medicine, Iowa State University, Ames, IA, United States
In equine parturition, the role of progestins along with the nuclear progesterone receptor (nPR) signaling pathway in the placenta is not completely clarified. The progestins play an integral role in maintaining myometrial quiescence during the late stage of pregnancy via acting on nPR isoforms (PRA and PRB; PRB is more active than PRA). The current study aimed to determine the PRA and PRB expressions in the term equine placenta at the gene and protein levels. Six term equine placentas were used in this study. Reverse transcription polymerase chain reaction (RT-PCR) was used to quantify the mRNA expression for PRA and PRB. The protein expression was detected using the Western Blot technique. The results revealed that the mRNA and protein expressions for PRA were significantly higher (P < 0.0001) in the term equine placental tissue compared to the mRNA and protein expressions of PRB. These results demonstrated that nPRs are detectable in the term placenta of mares and PRA is the dominant isoform expressed. The present findings raised the possibility that the PRA plays an important role in the parturition process and expulsion of the placenta in mares.
Introduction
The placenta is a crucial organ for a successful pregnancy establishment. It provides oxygen and nutrients to the conceptus (1, 2), as well as acts as an endocrine organ that provides steroid hormones including estradiol (E2) and progesterone (P4) (3, 4). P4 is the core component of pregnancy maintenance in mammals. The P4 performs its action non-genomically through binding to membrane receptors and genomically via binding to nuclear receptors (nPRs) (5–7). There are two isoforms of nPRs; PRA and PRB which are encoded by the same gene, but regulated by different promoters (8–10). PRA is the less active or inactive form of P4 receptors and shorter in amino acid sequence than PRB, the active form of the receptors (11–13).
In humans and other primates, P4 is crucial for maintaining the pregnancy (14, 15). However, the gestational physiology of mares is intricate. In mares, pregnancy is maintained even with undetectable plasma P4 concentrations (<0.5 ng/mL) starting in mid-pregnancy. Recently, it was reported that other pregnanes, mainly 5 α-dihydroprogesterone (5α-DHP) and 3α-DHP, maintain the pregnancy until term (16–18). The potency of DHP is equivalent to P4 on equine PR (18). Moreover, the parturition process in equine is accompanied by a drop of the DHP levels (progestin withdrawal) a few days before foaling (19). Very recent studies suggested that the infectious placentitis in equine is accompanied by progestin withdrawal, a decrease of the PR mRNA expression and a small degree of decline of the PRB protein in the myometrium which parallels with an increase of the inflammatory cytokines triggering the myometrial activation (20, 21).
The myometrium is the key maternal tissue for parturition (22–25). There is an interesting question as to whether the equine placenta is involved in the feto-maternal signaling pathway during parturition. For decades, it was well-known that P4 exerts its effect on the uterus and indirectly regulates placental function, whereas the existence of nPRs in the placenta is not completely understood (26–29). However, studies in humans suggested that the placenta is one of the target tissues for the P4 effect (3, 30, 31). The changes in the expression of nPR isoforms in the human placenta and its role in the feto-maternal signaling pathway for initiation of parturition have been revealed (32, 33). However, little is known about the changes of nPR isoforms in the equine placenta during parturition. To our best knowledge, this is the first study that determined both nPRs; mRNA and protein expressions of PRA and PRB in the equine placenta at the normal term labor.
We hypothesized that both nPR isoforms (PRA and PRB) exist in the term equine placenta. Thus, the present study aimed to determine the PRA and PRB expressions in the equine term placenta at the gene and protein levels.
Materials and Methods
Placental Tissue Processing
Placentas from Quarter Horse mares were collected immediately after foaling (n = 6) in the Large Animal Hospital, College of Veterinary Medicine, Iowa State University. This study was approved by the Institutional Animal Care and Use Committee of Iowa State University. The age of the mares was 7–10 years. The foaling processes were without complications and the gestation lengths were ranged between 332 and 358 days. The placentas were weighed and examined for completeness and abnormalities shortly after foaling. All placentas were normal. The tissue samples were taken from the chorioallantoic region. Immediately, the samples were dissected to remove the connective tissue, washed with chilled phosphate-buffered saline (PBS), immersed in liquid nitrogen and stored at −80°C for further studies.
RNA Isolation and cDNA Synthesis
Total RNA was isolated using the Absolutely RNA Miniprep Kit RNA (Agilent Technologies, Santa Clara, CA, USA) according to the manufacturer's instructions. The concentration and purity of the isolated RNA were determined by the Nanodrop 2000 spectrophotometer (Thermo Scientific, St Peters, MO, USA) by measuring the wavelengths at 260 and 280 nm, respectively. Total RNA (500 ng) was reverse-transcribed with random primers using the high capacity RNA to cDNA Kit (Applied Biosystems, Fermont, CA, USA) following the manufacturer's instructions.
Quantitative RT-PCR
The PCR reaction was performed using the Quant Studio™ 3 System (Applied Biosystems, Fermont, CA, USA). All samples were performed in triplicates using Power ™ SYBR Green Master Mix (Applied Biosystems, Cat# 43-676-59) along with specific primers (Table 1) designed using the Invitrogen primer design tool according to published sequences in the NCBI database and synthesized at Iowa State University's DNA Facility. Two sets of primers were designed for nuclear progesterone receptors. One is attached to the PR common sequence and detects both PRA and PRB. The other one is specific for the N-terminus of the PRB and detects only PRB. Double distilled water without cDNA template was used as a negative control in the PCR reaction. The amount of mRNA encoding PRA was calculated by subtracting the relative abundance of PRB mRNA from that of total PR mRNA. PCR efficiency was estimated using LinRegPCR (version 11.0). The relative mRNA abundance for the gene of interest was expressed as arbitrary units compared to the β-actin housekeeping gene (ACTB) mRNA using the ΔΔCT method as described (34).
Protein Isolation and Quantification
Frozen placental samples (~100 mg) were dissected and homogenized in the radioimmunoprecipitation assay buffer (RIPA,150 mM NaCl, 1%Triton X-100, 0.5% sodium deoxycholate, 0.1% SDS, 50 mM Tris, pH 8.0) with Halt™protease inhibitor cocktail (Thermo Scientific Corporation, St Peters, MO, USA) using the Tissumizer (Tekmar, Cincinnati, OH, USA). The protein concentration of each lysate was determined using the BCA assay (Pierce, Thermo scientific corporation, Cleveland, OH, USA).
Western Blot Analysis
The extracted proteins of 40 μg were diluted in 2x gel loading buffer (40% glycerol, 1 M Tris-HCl, pH 6.8, 2.5% β-mercaptoethanol, 8% sodium, dodecyl sulfate (SDS), and 0.01% bromophenol blue). The mixture was heated for 5–10 min at 100°C for complete denaturation. The denatured proteins were separated by polyacrylamide gel electrophoresis with SDS (10% SDS–PAGE) using Mini-PROTEAN System® (Bio-Rad, Hercules, CA, USA). T47D whole cell lysate (Santa Cruz Biotechnology, Santa Cruz, CA, USA, Cat# SC-2293) was used as the positive control for PRA and PRB in Western Blot analysis. The separated proteins were transferred to nitrocellulose membranes (Pierce, Thermo scientific corporation, Cleveland, OH, USA) using the Criterion™ System (Bio-Rad, Hercules, CA, USA). Subsequently, the membranes were blocked with 5% dry skimmed milk in Tris-buffered saline and Tween 20 (TBST) buffer (100 mM Tris–HCl, 0.9% NaCl, and 0.05% Tween 20) for 1 h at room temperature. The membranes were incubated overnight at 4°C with hPRa6 monoclonal antibody which recognizes both PRA and PRB (Invitrogen, Thermo Scientific Corporation, Cat# MA5-12653) and anti β-actin antibody (Santa Cruz Biotechnology, Cat# Sc-81178) as a loading control at 1:200 dilution. Membranes were washed 5 times for 5 min each with TBST buffer and incubated with the secondary antibody (Alex fluor®680 goat anti-mouse antibody) at 1:10,000 dilution (Invitrogen, Thermo Scientific Corporation, Cat# A-21058) for 1 h at room temperature. Then, it was washed again before being applied to the imaging system. The LI-COR Odyssey imaging system was used to scan the membrane and imaging to detect the immunofluorescent bands. Band intensity was measured by Image J analysis software.
Data Analysis
The data are presented as the mean ± SEM and were statistically analyzed using Student's t-test after checking for normality. The P < 0.05 was treated as statistically significant. The qPCR data were normalized using β-actin as a housekeeping gene and analyzed using the ΔΔCT method to obtain the relative abundance for mRNA encoding PRA and PRB (34). The amount of mRNA encoding PRA was calculated by subtracting the relative abundance of PRB mRNA from that of total PR mRNA. The relative abundance of PRA and PRB was obtained by dividing their values by β-actin values. The band intensity of the PR proteins and calibrator was identified using Image J software Graph Pad Prism 5.0 software package (GraphPad Software, Inc., San Diego, CA, USA) was used for performing all the calculations (35).
Results
PRA and PRB mRNA in Equine Placenta
The mRNA encoding PRA and PRB have been confirmed according to the amplicon size on the representative agarose gel of RT-PCR products of PRAB and PRB; product size: PRAB 327 bp, PRB 196 bp and β-actin 230 bp (Figure 1).
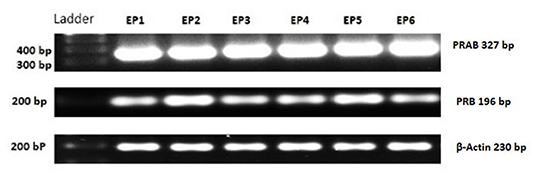
Figure 1. PCR products for PRAB, PRB, and housekeeping gene (β-Actin) of equine placental tissue samples (EP) 1–6. Bands appear at the expected size (PRAB 327 bp, PRB 196 bp and β-Actin 230 bp).
The expression of PRA mRNA was significantly higher (P < 0.0001) than the PRB mRNA expression (Figure 2).
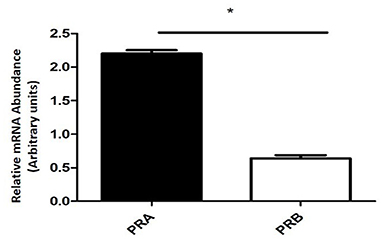
Figure 2. Relative mRNA abundance encoding PRA and PRB determined by qPCR in equine placental tissue samples (mean ± SEM, n = 6; *P < 0.0001).
PRA and PRB Protein Expression in Equine Placenta
The Western blot of samples from equine placental tissue showed that the band intensity of PRA was markedly higher than that of PRB (Figure 3). The PRA and PRB bands were confirmed by the molecular weight and T74D whole cell lysate as a positive control.
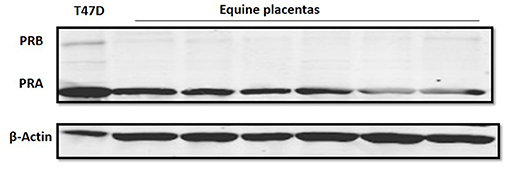
Figure 3. Progesterone receptor protein expression in equine placental tissue at term (n = 6). Immunoblot data show PRA and PRB, as well as β-actin as a loading control band. The T47D lysate was used as a positive control for PRA and PRB bands.
In the equine placental tissue, the protein expression of PRA was significantly higher (P < 0.0001) compared to that of PRB (Figure 4).
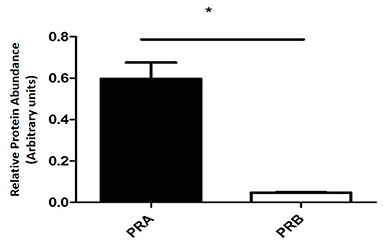
Figure 4. PRA and PRB protein relative abundance of equine placental tissue samples at term (mean ± SEM, n = 6; normalized to β-actin; *P < 0.0001).
Discussion
To our best knowledge, this is the first study that determined both nPR isoforms; PRA and PRB transcripts and protein expression in the equine placenta at the normal term labor. In the present study, we demonstrated that the placenta expressed both nPR isoforms. The PRA was the dominant isoform expressed in the equine placenta, while PRB was hardly expressed or absent. This extremely low expression of PRB in equine placenta could be related to the initiation of parturition. These findings suggested that the placenta might be involved in the initiation of the parturition signaling. Moreover, the foaling process could be associated with the predominance of PRA, the inactive form of progesterone receptors over PRB expression, the active form of the receptors (progestin functional withdrawal), leading to stimulation of the inflammatory cascade and uterine contraction genes within the myometrial tissue.
Progesterone (P4) is the principal steroid hormone for pregnancy maintenance in most species. The protective action of P4 on pregnancy is mediated through nPRs in the target tissues. There are two isoforms of nPRs; both PRA and PRB are from the same gene, but transcribed by different promoters. PRA inactivates or suppresses, while PRB actively mediates the P4 actions (32). In the present study, both RT-PCR and Western Blot data revealed that the mRNA and protein expressions of PRA were profoundly higher than those of PRB in equine placenta at the normal term labor. These results are consistent with the findings of previous studies in human placenta (32, 33, 36). These investigators detected both nPR isoforms in the human placenta and found an increase in the expression of PRA compared to PRB expression following parturition. These results support the crucial role of the PRA in functional progesterone withdrawal and initiation of parturition. In pregnant women, PRA plays an important role in the parturition process and expulsion of the placenta via its direct and indirect actions on the inflammatory cascade and myometrial activation (37). PRA suppresses the anti-inflammatory actions of P4 mediated by PRB. Furthermore, PRA directly upregulates the pro-inflammatory gene expression (37). On the other hand, the findings of the present study were not consistent with the earlier findings in the human placenta that PRs did not exist in the term placenta (38, 39). This inconsistency may be due to the difference in the sensitivity of the methods and techniques used. These investigators used the nuclear exchange assay, which has difficulties in the detection of PR in the placenta due to high endogenous P4 concentration (30). Moreover, our findings are parallel to those observed in human myometrium at parturition. They found strong evidence for the superiority of PRA over PRB expression (14, 37). However, little is known about the nPR isoform changes in the equine placenta during parturition. An earlier study used immunohistochemistry (IHC) for the detection of the nPRs in different stages of equine pregnancy between 110 and 309 days (40). These researchers did not detect nPRs in the placental tissues at the late pregnancy stage. However, they did not use specific antibodies for different nPR isoforms. Furthermore, RT-PCR and Western Blot, the techniques we used in the present study, are more sensitive and specific for the detection of PRs compared to IHC (41).
In the present study, we found a marked increase in the PRA mRNA and protein expression compared to PRB in the equine placenta following foaling. These results followed those of recent studies in equine myometrium. They revealed that the experimentally induced placentitis in late pregnant mares is associated with the decrease of the PRB expression compared to PRA expression in the myometrium (20, 21). This drop in the nPRs expression is followed by an increase in the inflammatory cytokines and contraction-associated proteins, initiating a strong contraction of the myometrium and expulsion of the fetus (42). These findings emphasize the important role of PRA in initiating the labor. The dominance of PRA over PRB expression could be involved in the fetomaternal signaling of the initiation of parturition.
The findings of this study suggested that the equine placenta is involved in the progestins-nPRs signaling at labor. These findings supported the evidence from previous observations in humans that the placenta is one of the target tissues for the progestin effect (3, 30, 31). Our novel hypothetical scenario in foaling is that the parturition signal begins from the fetus, affecting the nPRs levels toward the PRA dominance within the placenta. That signal might be extended and activate the myometrium. The gestational and parturient physiology between human and equine are similar (19). This would support our hypothesis that the foaling is associated with the predominance of PRA over PRB expression (progestin functional withdrawal) which inhibits the progestins' protective function during pregnancy.
In conclusion, the present study showed that PRA, the less active or inactive form of nPRs, dominated over PRB, the active form of the receptors, in the mare placenta at term. The present findings raise the possibility that the PRA plays an important role in the parturition process in the mares including the expulsion of the placenta. Moreover, the equine parturition might be associated with a local functional progestin withdrawal in the placental tissues initiating the parturition process. These findings will help us understand the mechanisms by which progestins target the nPR isoforms. Moreover, they could help us understand the changes occurring in the term and preterm labor.
Data Availability Statement
The original contributions presented in the study are included in the article/supplementary material, further inquiries can be directed to the corresponding author/s.
Ethics Statement
The animal study was reviewed and approved by Institutional Animal Care and Use Committee of Iowa State University.
Author's Note
The present study was performed at Iowa State University where AN was a visiting scholar.
Author Contributions
WH, AN, and SS designed the study. SS collected and prepared the tissue samples. AN performed the mRNA and protein studies. AA performed the statistical analysis. AN and AH prepared and edited the manuscript. WH edited the final version of the manuscript. All authors read and approved the final version of the manuscript.
Funding
This work was funded by the Egyptian Ministry of Higher Education. The funder had no role in the study design, data collection, and analysis, decision to publish, or preparation of the manuscript.
Conflict of Interest
The authors declare that the research was conducted in the absence of any commercial or financial relationships that could be construed as a potential conflict of interest.
References
1. Davis KA, Klohonatz KM, Bouma GJ, Bruemmer JE. androgen receptor in the term equine placenta. J Equine Vet Sci. (2017) 54:8–11. doi: 10.1016/j.jevs.2016.09.012
2. Arai KY, Tanaka Y, Taniyama H, Tsunoda N, Nambo Y, Nagamine N, et al. Expression of inhibins, activins, insulin-like growth factor-I and steroidogenic enzymes in the equine placenta. Domest Anim Endocrinol. (2006) 31:19–34. doi: 10.1016/j.domaniend.2005.09.005
3. Zachariades E, Mparmpakas D, Pang Y, Rand-Weaver M, Thomas P, Karteris E. Changes in placental progesterone receptors in term and preterm labour. Placenta. (2012) 33:367–72. doi: 10.1016/j.placenta.2012.01.002
4. Fowden AL, Forhead AJ, Sferruzzi-Perri AN, Burton GJ, Vaughan OR. Review: endocrine regulation of placental phenotype. Placenta. (2015) 36:S50–9. doi: 10.1016/j.placenta.2014.11.018
5. Grazul-Bilska AT, Thammasiri J, Kraisoon A, Reyaz A, Bass CS, Kaminski SL, et al. Expression of progesterone receptor protein in the ovine uterus during the estrous cycle: effects of nutrition, arginine and FSH. Theriogenology. (2018) 108:7–15. doi: 10.1016/j.theriogenology.2017.11.008
6. Kowalik MK, Rekawiecki R, Kotwica J. The putative roles of nuclear and membrane-bound progesterone receptors in the female reproductive tract. Reprod Biol. (2013) 13:279–89. doi: 10.1016/j.repbio.2013.09.001
7. Mulac-Jericevic B, Conneely OM. Reproductive tissue selective actions of progesterone receptos. Reproduction. (2004) 128:139–46. doi: 10.1530/rep.1.00189
8. Viville B, Charnock-Jones DS, Sharkey AM, Wetzka B, Smith SK. Distribution of the A and B forms of the progesterone receptor messenger ribonucleic acid and protein in uterine leiomyomata and adjacent myometrium. Hum Reprod. (1997) 12:815–22. doi: 10.1093/humrep/12.4.815
9. Steinhauser CB, Bazer FW, Burghardt RC, Johnson GA. Expression of progesterone receptor in the porcine uterus and placenta throughout gestation: correlation with expression of uteroferrin and osteopontin. Domest Anim Endocrinol. (2017) 58:19–29. doi: 10.1016/j.domaniend.2016.07.002
10. Jacobsen BM, Horwitz KB. Progesterone receptors, their isoforms and progesterone regulated transcription. Mol Cell Endocrinol. (2012) 357:18–29. doi: 10.1016/j.mce.2011.09.016
11. Pieber D, Allport VC, Bennett PR. Progesterone receptor isoform A inhibits isoform B-mediated transactivation in human amnion. Eur J Pharmacol. (2001) 427:7–11. doi: 10.1016/S0014-2999(01)01189-X
12. Lee II, Maniar K, Lydon JP, Kim JJ. Akt regulates progesterone receptor B-dependent transcription and angiogenesis in endometrial cancer cells. Oncogene. (2016) 35:5191–201. doi: 10.1038/onc.2016.56
13. Kowalik MK, Rekawiecki R, Kotwica J. Expression of membrane progestin receptors (mPRs) in the bovine corpus luteum during the estrous cycle and first trimester of pregnancy. Domest Anim Endocrinol. (2018) 63:69–76. doi: 10.1016/j.domaniend.2017.12.004
14. Mesiano S, Chan EC, Fitter JT, Kwek K, Yeo G, Smith R. Progesterone withdrawal and estrogen activation in human parturition are coordinated by progesterone receptor A expression in the myometrium. J Clin Endocrinol Metab. (2002) 87:2924–30. doi: 10.1210/jcem.87.6.8609
15. Henson MC. Pregnancy maintenance and the regulation of placental progesterone biosynthesis in the baboon. Hum Reprod Update. (1998) 4:389–405. doi: 10.1093/humupd/4.4.389
16. Scholtz EL, Krishnan S, Ball BA, Corbin CJ, Moeller BC, Stanley SD, et al. Pregnancy without progesterone in horses defines a second endogenous biopotent progesterone receptor. PNAS. (2014) 111:3365–70. doi: 10.1073/pnas.1318163111
17. Legacki EL, Corbin CJ, Ball BA, Wynn M, Loux S, Stanley SD, et al. Progestin withdrawal at parturition in the mare. Reproduction. (2016) 152:323–31. doi: 10.1530/REP-16-0227
18. Legacki EL, Corbin CJ, Ball BA, Scoggin KE, Stanley SD, Conley AJ. Steroidogenic enzyme activities in the pre- and post-parturient equine placenta. Reproduction. (2018) 155:51–9. doi: 10.1530/REP-17-0472
19. Conley AJ. Review of the reproductive endocrinology of the pregnant and parturient mare. Theriogenology. (2016) 86:355–65. doi: 10.1016/j.theriogenology.2016.04.049
20. El-Sheikh Ali H, Legacki EL, Loux SC, Esteller-Vico A, Dini P, Scoggin KE, et al. Equine placentitis is associated with a downregulation in myometrial progestin Signaling. Biol Reprod. (2019) 101:162–76. doi: 10.1016/j.jevs.2018.05.115
21. Ali HE, Ball B. Cervix and myometrium : role in preterm and normal term birth in mare. Clin Theriogenol. (2020) 12:340–5.
22. Palliser HK, Zakar T, Symonds IM, Hirst JJ. Progesterone receptor isoform expression in the guinea pig myometrium from normal and growth restricted pregnancies. Reprod Sci. (2010) 17:776–82. doi: 10.1177/1933719110371517
23. Patel B, Elguero S, Thakore S, Dahoud W, Bedaiwy M, Mesiano S. Role of nuclear progesterone receptor isoforms in uterine pathophysiology. Hum Reprod Update. (2015) 21:155–73. doi: 10.1093/humupd/dmu056
24. Soloff MS, Jeng YJ, Izban MG, Sinha M, Luxon BA, Stamnes SJ, et al. Effects of progesterone treatment on expression of genes involved in uterine quiescence. Reprod Sci. (2011) 18:781–97. doi: 10.1177/1933719111398150
25. Welsh TN, Hirst JJ, Palliser H, Zakar T. Progesterone receptor expression declines in the guinea pig uterus during functional progesterone withdrawal and in response to prostaglandins. PLoS ONE. (2014) 9:1–10. doi: 10.1371/journal.pone.0105253
26. Rivera J, Cano A. Oestrogen and progesterone receptors in human term placenta. Measurement by binding assays and immunological methods. Placenta. (1989) 10:579–88. doi: 10.1016/0143-4004(89)90049-0
27. Padayachi T, Pegoraro RJ, Rom L, Joubert SM. Enzyme immunoassay of oestrogen and progesterone receptors in uterine and intrauterine tissue during human pregnancy and labour. J Steroid Biochem Mol Biol. (1990) 37:509–11. doi: 10.1016/0960-0760(90)90394-Z
28. Karalis K, Goodwin G, Majzoub JA. Cortisol blockade of progesterone: a possible molecular mechanism involved in the initiation of human labor. Nat Med. (1996) 2:556–60. doi: 10.1038/nm0596-556
29. McCormick PD, Razel AJ, Spelsberg TC, Coulam CB. Absence of high-affinity binding of progesterone (R 5020) in human placenta and fetal membranes. Placenta Suppl. (1981) 3:123–32.
30. Shanker YG, Rao AJ. Progesterone receptor expression in the human placenta. Mol Hum Reprod. (1998) 5:481–6. doi: 10.1093/molehr/5.5.481
31. Palomares K, Wang B, Parobchak N, Rosen T. 77: Epigenetic regulation of progesterone receptor isoform-A (PR-A) in the human placenta. Am J Obstet Gynecol. (2016) 214:S56. doi: 10.1016/j.ajog.2015.10.096
32. Oh SY, Chong JK, Park I, Romero R, Sohn YK, Kyung CM, et al. Progesterone receptor isoform (A/B) ratio of human fetal membranes increases during term parturition. Am J Obstet Gynecol. (2005) 193:1156–60. doi: 10.1016/j.ajog.2005.05.071
33. Mills AA, Yonish B, Feng L, Schomberg DW, Heine RP, Murtha AP. Characterization of progesterone receptor isoform expression in fetal membranes. Am J Obstet Gynecol. (2006) 195:998–1003. doi: 10.1016/j.ajog.2006.06.040
34. Livak KJ, Schmittgen TD. Analysis of relative gene expression data using real-time quantitative PCR and the 2-ΔΔCT method. Methods. (2001) 25:402–8. doi: 10.1006/meth.2001.1262
35. Schneider CA, Rasband WS, Eliceiri KW. NIH Image to ImageJ: 25 years of image analysis. Nat Methods. (2012) 9:671–5. doi: 10.1038/nmeth.2089
36. Goldman S, Weiss A, Almalah I, Shalev E. Progesterone receptor expression in human decidua and fetal membranes before and after contractions: possible mechanism for functional progesterone withdrawal. Mol Hum Reprod. (2005) 11:269–77. doi: 10.1093/molehr/gah161
37. Tan H, Yi L, Rote NS, Hurd WW, Mesiano S. Progesterone receptor-A and -B have opposite effects on proinflammatory gene expression in human myometrial cells: implications for progesterone actions in human pregnancy and parturition. J Clin Endocrinol Metab. (2012) 97:719–30. doi: 10.1210/jc.2011-3251
38. Younes MA, Besch NF, Besch PK. Estradiol and progesterone binding in human term placental cytosol. Am J Obstet Gynecol. (1981) 141:170–4. doi: 10.1016/S0002-9378(16)32586-8
39. Khan-Dawood FS, Dawood MY. Estrogen and progesterone receptor and hormone levels in human myometrium and placenta in term pregnancy. Am J Obstet Gynecol. (1984) 150:501–5. doi: 10.1016/S0002-9378(84)90428-9
40. Abd-Elnaeim MMM, Derar IR, Wilsher S, Allen WR, Leiser R, Schuler G. Immunohistochemical localization of oestrogen receptors α and β, progesterone receptor and aromatase in the equine placenta. Reprod Domest Anim. (2009) 44:312–9. doi: 10.1111/j.1439-0531.2008.01073.x
41. Sinn HP, Schneeweiss A, Keller M, Schlombs K, Laible M, Seitz J, et al. Comparison of immunohistochemistry with PCR for assessment of ER, PR, and Ki-67 and prediction of pathological complete response in breast cancer. BMC Cancer. (2017) 17:1–10. doi: 10.1186/s12885-017-3111-1
Keywords: placenta, equine, progesterone, receptors, PRA, PRB
Citation: Nagy AM, Sathe SR, Atta AH, Hammam AMM and Hsu WH (2021) Characterization of Nuclear Progesterone Receptor Isoforms in the Term Equine Placenta. Front. Vet. Sci. 8:660177. doi: 10.3389/fvets.2021.660177
Received: 28 January 2021; Accepted: 08 March 2021;
Published: 01 April 2021.
Edited by:
Arumugam Kumaresan, National Dairy Research Institute (ICAR), IndiaReviewed by:
Cecilia Dall'Aglio, University of Perugia, ItalyFirdous Ahmad Khan, St. George's University, Grenada
Copyright © 2021 Nagy, Sathe, Atta, Hammam and Hsu. This is an open-access article distributed under the terms of the Creative Commons Attribution License (CC BY). The use, distribution or reproduction in other forums is permitted, provided the original author(s) and the copyright owner(s) are credited and that the original publication in this journal is cited, in accordance with accepted academic practice. No use, distribution or reproduction is permitted which does not comply with these terms.
*Correspondence: Walter H. Hsu, whsu@iastate.edu