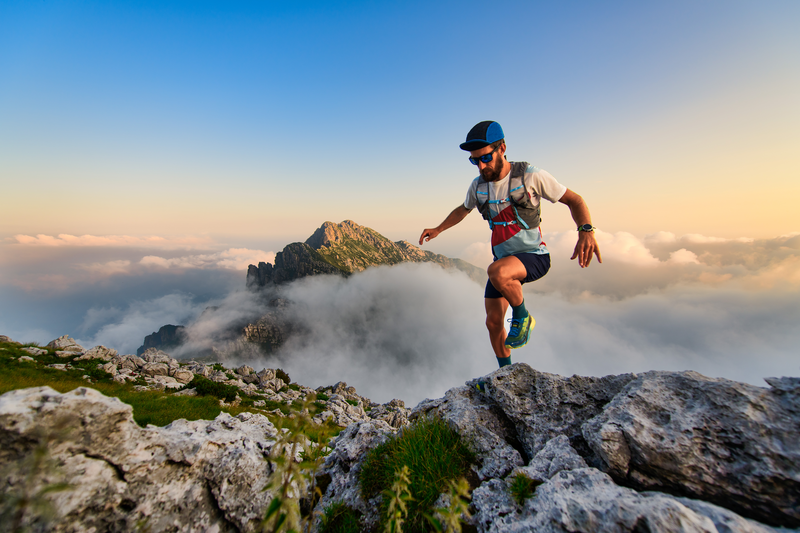
94% of researchers rate our articles as excellent or good
Learn more about the work of our research integrity team to safeguard the quality of each article we publish.
Find out more
BRIEF RESEARCH REPORT article
Front. Vet. Sci. , 26 March 2021
Sec. Parasitology
Volume 8 - 2021 | https://doi.org/10.3389/fvets.2021.659786
This article is part of the Research Topic Current Knowledge on Pathogenic and Endosymbiotic Tick-Borne Bacteria View all 13 articles
Spiroplasma are vertically-transmitted endosymbionts of ticks and other arthropods. Field-collected Ixodes persulcatus have been reported to harbour Spiroplasma, but nothing is known about their persistence during laboratory colonisation of this tick species. We successfully isolated Spiroplasma from internal organs of 6/10 unfed adult ticks, belonging to the third generation of an I. persulcatus laboratory colony, into tick cell culture. We screened a further 51 adult male and female ticks from the same colony for presence of Spiroplasma by genus-specific PCR amplification of fragments of the 16S rRNA and rpoB genes; 100% of these ticks were infected and the 16S rRNA sequence showed 99.8% similarity to that of a previously-published Spiroplasma isolated from field-collected I. persulcatus. Our study shows that Spiroplasma endosymbionts persist at high prevalence in colonised I. persulcatus through at least three generations, and confirms the usefulness of tick cell lines for isolation and cultivation of this bacterium.
Ixodid ticks naturally harbour a variety of bacterial symbionts that may be obligately or facultatively intracellular and are transovarially transmitted. These include species of the genera Rickettsia, Coxiella, Midichloria and Spiroplasma that occur with high frequency (1–4) and less common or well-characterised species of the genus Francisella (1, 3) and Occidentia (5). The insect symbionts Cardinium, Wolbachia, Arsenophonus and Rickettsiella have also been detected in or isolated from ticks (3, 6–10) but it is unclear whether or not their presence results from parasitism by insects such as the wasp Ixodiphagus hookeri (7, 9) or cohabiting mites (author's unpublished observations), and they are not known to be transovarially transmitted in ticks. Most studies of occurrence of bacterial symbionts in ticks are based on molecular detection in DNA extracted from individual or pooled ticks sampled directly from the field. Some recognised or putative tick symbionts have been isolated into culture, in either mammalian or tick cells; these include several species of Rickettsia (11–16), Francisella (17), several strains of Spiroplasma (10, 18–21) and one isolate each of Arsenophonus, Occidentia and Rickettsiella (5, 8, 10). In all cases, the unfed or partially-fed ticks had been collected from the field, and bacteria were isolated directly from homogenised/macerated whole ticks or aseptically-dissected internal organs, or from eggs laid by engorged female ticks.
Ixodes persulcatus, a tick species distributed widely from the eastern Baltic coast to Japan (22–25), has been reported to harbour symbionts including the Montezuma agent, now called Candidatus Lariskella arthropodarum (26–28), Coxiella and Spiroplasma spp. (29), as well as human and livestock pathogens including tick-borne encephalitis virus (TBEV), Kemerovo virus, Alongshan virus, Anaplasma phagocytophilum, Candidatus Neoehrlichia mikurensis, Ehrlichia muris, Rickettsia helvetica, Rickettsia heilongjiangensis, Candidatus Rickettsia tarasevichiae, Borrelia miyamotoi, Borrelia burgdorferi sensu lato, Theileria equi and several species of Babesia (25, 30–38). Co-infections with multiple pathogens and symbionts are common (29, 35, 39). There is a single report of isolation into culture of bacterial symbionts from Japanese I. persulcatus: R. helvetica and a Spiroplasma were isolated from field-caught adult male ticks into an Ixodes scapularis cell line (10).
Research on transmission of tick-borne pathogens of medical and veterinary interest depends largely on ticks maintained in laboratory colonies. However, few studies have assessed such ticks for presence of symbionts, despite the potential influence of the latter on the ability of ticks to harbour (40) and/or transmit pathogens. Prevalence of Candidatus Midichloria mitochondrii determined by molecular methods was found to be lower in Ixodes ricinus ticks from laboratory colonies than in field ticks, and to decrease (albeit in a small sample size) with increasing numbers of tick generations (2). A subsequent study, using a more sensitive assay, revealed the presence of extremely low levels of Ca. M. mitochondrii DNA in 60% of >10th generation laboratory colony I. ricinus (41). Ixodes arboricola were screened for bacterial symbionts by PCR and higher incidences were found in field-collected ticks than in laboratory colony ticks of three genera: Rickettsiella (28.0 vs. 0%), Midichloria (1.3 vs. 0%) and Spiroplasma (16.0 vs. 5.6%) (3). Both groups harboured similarly high levels of Rickettsia (96.0 vs. 100%), suggesting that transovarial transmission was highly efficient for Rickettsia, less efficient for Spiroplasma and might not occur for Rickettsiella. Both lower absolute numbers of bacteria including the symbionts Spiroplasma and Midichloria, and more limited diversity of bacterial species, were reported in midguts of I. ricinus ticks from a laboratory colony compared to wild-caught ticks, and extremely low numbers of bacteria (<100 organisms per midgut) were found in Rhipicephalus microplus ticks from a closed colony in Brazil (42). A bacterial symbiont, later identified as a Cardinium sp. (43), was isolated from first-generation adult I. scapularis reared in the laboratory from field-caught adults (6). Rickettsia raoultii was isolated from eggs laid by the first generation of adult Dermacentor reticulatus reared in the laboratory from field-caught ticks (21). However, we could not find any report of in vitro isolation of a bacterial symbiont from laboratory colony ticks maintained for additional generations.
Here we report isolation and preliminary genetic characterisation of a Spiroplasma from third-generation adult male and female I. persulcatus, originally collected in Siberia (Irkutsk Oblast, Russian Federation) and maintained in a laboratory colony for over 4 years.
Unfed adult I. persulcatus ticks were collected from vegetation by flagging near Irkutsk, (Irkutsk Oblast, Russian Federation) at Talsy (52.024381 N, 104.657681 E) and Ust-Ordynsky (52.700295 N, 104.905164 E) in May 2015. The ticks were subsequently maintained as a laboratory colony through three generations in the tick rearing facility of the Institute of Parasitology, Biology Centre, Czech Academy of Sciences (BCCAS). All animal experiments were in accordance with the Animal Protection Law of the Czech Republic (§17, Act No. 246/1992 Sb) and with the approval of the Czech Academy of Sciences (approval no. 161/2010). All instars were fed to engorgement on guinea pigs or gerbils, incubated for moulting or oviposition at 24°C, 96% relative humidity (RH) and stored following moult or larval hatching under the same conditions. To obtain separate groups of unfed adult male and female ticks, nymphs were visually inspected following engorgement, and males were sorted from females according to their size as male nymphs are approximately one third smaller. Unfed adult male and female ticks were transferred by courier to the Tick Cell Biobank, University of Liverpool, where they were stored at 15°C, 100% RH for 19 days until used for Spiroplasma isolation or seven months until used for DNA extraction.
Five male and five female unfed adult I. persulcatus ticks were surface-sterilised by immersion in 0.1% benzalkonium chloride for 5 min, 70% ethanol for 1 min and 2 x 1 min rinses in sterile deionised water. After drying on sterile filter paper, the ticks were embedded in wax and their internal organs (as much as possible of midgut, salivary glands, synganglion, Malpighian tubules, rectal sac, fat body, testes/ovary) were dissected out as described previously (21). Each tick was dissected in a separate drop of Hank's balanced salt solution and the dissecting instruments were sterilised in 70% ethanol between ticks. The internal organs from each tick were inoculated into a separate culture of tick cells in a sealed, flat-sided tube (Nunc, Thermo-Fisher) and incubated at 28°C. Four embryo-derived tick cell lines were used for Spiroplasma isolation: Rhipicephalus microplus BME/CTVM23 (13) and BME26 (44), I. ricinus IRE11 (45) and Ixodes scapularis IDE2 (46). BME/CTVM23 and BME26 cells were grown in complete L-15 and L-15B media respectively, (47) and IRE11 and IDE2 cells were grown in complete L-15B300 medium (48); all media contained 100 units/ml penicillin and 100 μg/mL streptomycin. Medium was changed weekly by removal and replacement of of the medium volume and cultures were monitored by inverted microscope examination. Giemsa-stained cytocentrifuge smears were prepared as described previously (13) from all cultures on day 53 post inoculation (p.i.) and examined for presence of bacteria. All cultures were cryopreserved in vapour phase liquid nitrogen as described previously (21) on day 90 p.i.
On day 65 p.i., the cells in each culture were resuspended and 200 μL aliquots were centrifuged at 15,000 × g for 5 min. DNA was extracted from the cell pellets using a DNeasy blood and tissue Mini Kit (Qiagen) following the manufacturer's instructions. DNA extracts were screened for presence of Spiroplasma using PCR assays amplifying fragments of the 16S rRNA (16S rRNA; ~500 bp) and RNA polymerase beta subunit (rpoB; ~1443 bp) genes (49, 50). Amplicons were visualised by agarose gel electrophoresis, and positive PCR products were purified using a PureLink Quick Gel Extraction and PCR Purification Combo kit (ThermoFisher) following the manufacturer's instructions and submitted for Sanger sequencing in both directions (Eurofins Genomics, Germany). Phylogenetic analyses were conducted with MEGA X using the maximum likelihood method based on the Kimura 2-parameter model and including all sites (51, 52). The nucleotide substitution model was selected according to the Bayesian information criterion (BIC) implemented in Mega X (53). Confidence values for individual branches of the resulting trees were determined by bootstrap analysis with 500 replicates. Two separate phylogenetic trees based on available 16S rRNA and rpoB sequences of Spiroplasma spp. isolated or detected in ixodid ticks were inferred. It was not possible to include all these Spiroplasma variants in both phylogenies because published sequences of both gene fragments amplified in this study were not available for some of them. Moreover, the rpoB analysis was performed with a shorter fragment (<600 bp) corresponding to the fragment available from many of these published sequences. The published sequences used in the analyses are shown in the phylogenetic trees.
DNA was extracted from 10 ticks (four male and six female) remaining from the batch shipped to Liverpool, 7 months after receipt, using a DNeasy blood and tissue Mini Kit (Qiagen) according to the manufacturer's instructions with overnight lysis. DNA was extracted from a further 17 male and 24 female ticks from the same generation maintained in the BCCAS colony, using a DNeasy blood and tissue Mini Kit (Qiagen) with the following modifications. Briefly, the ticks were homogenised individually in 200 μL of ATL buffer (Qiagen) for 2 min at 30 shakes/s in a Tissue Lyser II (Qiagen). After brief centrifugation and addition of 20 μL of proteinase K, the samples were incubated at 56°C for 30 min. The remaining steps of DNA extraction were done according to the manufacturer's instructions. To confirm species identity of the ticks screened in Liverpool, a fragment of the tick 16S rRNA gene was amplified using primer pairs 16S+1/16S-1 as described previously (54). To detect Spiroplasma, DNA from all ticks was PCR-screened using the specific assays for fragments of the Spiroplasma 16S rRNA and rpoB genes as described above. Randomly-selected positive amplicons were purified and sequenced as above (Liverpool ticks) or enzymatically purified using Exonuclease I FastAP and Thermosensitive Alkaline Phosphatase (ThermoFisher Scientific) and submitted for Sanger sequencing (SeqMe, Czech Republic) (BCCAS ticks), and analysed as described above.
When the tick cell cultures were examined by Giemsa-stained cytocentrifuge smear on day 53 p.i., bacteria resembling Spiroplasma were seen in cells that had received organs from 1/5 male and 5/5 female I. persulcatus ticks (Table 1). In all cases, the Spiroplasma were intracellular and concentrated in cytoplasmic vacuoles, but the appearance differed between the various tick cell lines (Figure 1). In the R. microplus cell lines (Figures 1A,B) and IDE2 (not shown), most vacuoles containing Spiroplasma also contained homogenous, light blue- or pink-staining background material, whereas in IRE11 cells (Figures 1C,D) such material was absent in most vacuoles containing Spiroplasma. It was not possible to determine whether this was due to differences between the cell lines or the Spiroplasma isolates, although in previous studies background material was visible in Spiroplasma-containing vacuoles in cells of the tick cell lines BME/CTVM23 and DALBE3 (21) but not of the tick cell lines IRE11, IRE/CTVM19 or IDE2 (20).
Table 1. Detection of Spiroplasma by microscopy and PCR analysis of tick cell lines inoculated with internal organs from male and female Ixodes persulcatus ticks.
Figure 1. Morphology of the Spiroplasma sp. isolated from Ixodes persulcatus ticks. (A–D). Spiroplasma (arrows) in tick cell lines inoculated with internal organs from male and female I. persulcatus ticks, day 53 post inoculation. (A) BME/CTVM23 cells inoculated with male tick #304 (Spiroplasma strain Irkutsk1). (B) BME26 cells inoculated with female tick #308 (Spiroplasma strain Irkutsk4). (C,D). IRE11 cells inoculated with female tick #310 (Spiroplasma strain Irkutsk5). Giemsa-stained cytocentrifuge smears; scale bars = 10 μm.
PCR amplification of fragments of the Spiroplasma-specific 16S rRNA and rpoB genes from DNA extracted on day 65 p.i. confirmed the presence of Spiroplasma in the six microscopically-positive cultures, and in both cases failed to amplify any products from DNA extracted from the four microscopically-negative cultures (Table 1). To determine the Spiroplasma infection rate in adult ticks of the parent colony, the 10 ticks remaining in Liverpool (four males, six females) and a further 41 ticks (12 males, 12 females fed as nymphs on guinea pigs and five males, 12 females fed as nymphs on gerbils) from the same generation of the BCCAS colony were screened using the Spiroplasma 16S rRNA and rpoB PCR assays. All of the ticks were positive for Spiroplasma by one or both assays, and amplification and sequencing of a 430 bp fragment of the tick 16S rRNA gene confirmed the species identity of the ticks tested in Liverpool as I. persulcatus (99.8% similarity to I. persulcatus from Omsk, Siberia, Russia, Genbank accession no. MH790201.1).
Sequence analysis revealed that, for the Spiroplasma 16S rRNA gene, all six culture isolates (designated Irkutsk1-6) and five representative tick samples screened in Liverpool were identical to each other, and identical to eight representative tick samples screened at BCCAS apart from one ambiguous nucleotide at position 105 (Table 2A). All sequences showed 99.8% similarity (99.5% query cover) to the only sequence from an I. persulcatus-derived Spiroplasma available in Genbank at the time of writing (LC388762.1) (10); interestingly, the only mismatch between our sequences and that of the Japanese isolate (10) was also at position 105 (Table 2A). The 16S rRNA sequence of the Irkutsk strains isolated from I. persulcatus was identical to several other Spiroplasma strains isolated from hard ticks: Spiroplasma sp. Bratislava 1 (KP967685, from Slovakian I. ricinus), Spiroplasma sp. 1033 (LC388770, from Japanese Haemaphysalis kitaokai) and Spiroplasma sp. 135 (LC388760 and LC388759, from Japanese Ixodes monospinosus) (10, 20) (Table 2A). Moreover, the Irkutsk 16S rRNA sequence showed 99.1–99.3% similarity to those of spiroplasmas isolated from North American Ixodes pacificus (Spiroplasma ixodetis, NR_104852), Spanish Dermacentor marginatus (Spiroplasma sp. strain DMAR11, MG859280) and Dutch D. reticulatus (Spiroplasma sp. strain DRET8, MG859282) (21, 55) (Table 2A). For the rpoB gene, all sequences obtained from the six culture isolates and 11 representative whole ticks were identical. At the time of writing, we could not find any published rpoB sequences from I. persulcatus-derived Spiroplasma for comparison, and most of those derived from other hard tick species were shorter than 600 bp. Considering the query cover higher than 99%, the rpoB sequence of the Irkutsk strains showed 99.9% similarity to the spiroplasmas isolated from I. ricinus (Spiroplasma sp. Bratislava1, KP967687) and Dermacentor spp. (Spiroplasma strain DMAR11, MG859278 and Spiroplasma strain DRET8, MG859277) (Table 2B), and 99.3% similarity to S. ixodetis (DQ313832). With a query cover of 43%, the sequences amplified in this study were identical to shorter sequences from spiroplasmas detected by PCR in other hard tick species (GenBank accession numbers MK267073-MK267077, MK267081-MK267085 and MK267097) (4), and also to Spiroplasma strain DMAR11 (MG859278) and Spiroplasma sp. Bratislava1 (KP967687) that showed polymorphisms in the longer gene fragment (Table 2B).
Table 2A. Polymorphisms in sequences detected in the Ixodes persulcatus-derived Spiroplasma sp. in this study compared to other tick-borne Spiroplasma spp.
Table 2B. Polymorphisms in sequences detected in the Ixodes persulcatus-derived Spiroplasma sp. in this study compared to other tick-borne Spiroplasma spp.
Phylogenetic analysis based on 16S rRNA sequences derived from Spiroplasma sp. strain Irkutsk1 and two representative whole ticks revealed that the I. persulcatus spiroplasmas clustered together with, but were not identical to, S. ixodetis (55) and most of the spiroplasmas from other hard ticks (Figure 2A). Similarly, the phylogeny obtained with the rpoB sequences showed tight clustering of the I. persulcatus Spiroplasma with most other tick-borne Spiroplasma sequences (Figure 2B).
Figure 2. Phylogenetic analysis of Spiroplasma gene sequences obtained from third generation Ixodes persulcatus colony ticks. Sequences amplified from DNA extracted from representative adult Ixodes persulcatus ticks at the University of Liverpool (Liverpool tick) and the Institute of Parasitology, Biology Centre, Czech Academy of Sciences (BCCAS tick) and from a representative Spiroplasma-infected tick cell culture previously inoculated with internal organs from an adult I. persulcatus (Spiroplasma sp. strain Irkutsk1) were compared with published sequences from other Spiroplasma spp. or strains derived from ixodid tick species. The evolutionary analysis was inferred using the maximum likelihood method and Kimura 2-parameter model within the Mega X software. The trees are drawn to scale, with branch lengths measured in the number of substitutions per site. GenBank accession numbers of the sequences used in this analysis are shown in brackets following each Spiroplasma species or strain and before the tick species host. Sequences obtained in this study are marked in bold. (A) Tree constructed from 15 16S rRNA nucleotide sequences and a total of 461 positions in the final dataset. (B) Tree constructed from 19 rpoB nucleotide sequences and a total of 588 positions in the final dataset.
The Spiroplasma 16S rRNA and rpoB gene sequences obtained in the present study were deposited in GenBank under accession numbers MW492370, MW498416, MW498417, MW528409-MW528411.
Colonisation in the laboratory has been previously reported to result in decrease or loss of the microbial symbiont Ca. M. mitochondrii in I. ricinus (2, 41), whereas Coxiella-like endosymbionts were detected at high prevalence in Ornithodoros rostratus, Amblyomma americanum, Dermacentor silvarum and R. microplus ticks maintained in laboratory colonies for unspecified numbers of generations (55). In the case of the I. persulcatus Spiroplasma in the present study, after three generations in the laboratory, 100% of whole adult ticks (21 males, 30 females) were PCR-positive for this endosymbiont. Moreover, 5/5 female ticks and 1/5 male ticks harboured sufficient levels of viable bacteria to allow in vitro isolation in tick cell lines. Admittedly, the sensitivity of this technique for detection of infection with Spiroplasma is unknown, so it is possible that the remaining four male ticks could also have harboured Spiroplasma but either at a level insufficient to allow isolation, or in an organ or tissue that was inadvertently not included in the inoculum, or in a state of viability not conducive to in vitro isolation. Tissue tropism of the symbiont Ca. M. mitochondrii in I. ricinus ticks was found to be highly specific to certain organs (56); further study is needed to determine the tissue tropism of Spiroplasma spp. in Ixodes spp. ticks.
The prevalence of Spiroplasma in the original Siberian field ticks from which the laboratory colony was initiated in 2015 is unknown, and therefore it is impossible to determine whether colonisation resulted in maintenance of, or increase in, the infection rate. However, it can be concluded that laboratory colonisation does not have a negative effect on occurrence of Spiroplasma in I. persulcatus, at least over three generations. There have only been two reports of detection of Spiroplasma in I. persulcatus ticks. Using 16S amplicon pyrosequencing, Spiroplasma were detected in salivary glands of at least 5/6 male and 5/6 female unfed I. persulcatus collected in the field in Japan (29), and Spiroplasma was successfully isolated into arthropod cell culture from 1/30 questing adult I. persulcatus collected in Japan (10). In contrast, no Spiroplasma or other mollicutes were recorded in questing I. persulcatus collected in the Novosibirsk area of Russia and examined by 16S metagenomic profiling (four pools of 87–120 ticks) (32), and Spiroplasma were not detected by species-specific 16S rRNA PCR in three questing I. persulcatus ticks collected in Finland (3).
Spiroplasma infection rates determined by molecular analysis vary widely in other Ixodes spp. ticks collected from the field in different geographical areas. Prevalence of Spiroplasma in I. ricinus nymphs and adults ranged from 0–0.3% in UK [(21); author's unpublished data] through 5–6% in Hungary and Czech Republic (57, 58) to 23–30% in The Netherlands, France, Switzerland and Spain (3, 59). The bacterium was detected in 10% of Ixodes uriae from Russia (3), 14–16% of I. arboricola from Belgium (3, 60) and 100% of Ixodes ovatus from Japan (29). The type species S. ixodetis was isolated from 7/30 pools representing 600 I. pacificus from USA, suggesting a prevalence between 1.2 and 23% (61). Considering this level of variation between species and geographical location, the infection rates of 100% in whole ticks and 60% following in vitro isolation in the present study suggest that Spiroplasma survives well-under laboratory colony conditions, in both male and female I. persulcatus ticks.
Presence of Spiroplasma in laboratory colony ticks could affect their ability to be infected experimentally with, and/or transmit, tick-borne pathogens, and therefore their use in this context. A recent study examined correlations between presence of Spiroplasma in field-collected I. ricinus in Switzerland, and presence in these ticks of bacterial pathogens and symbionts (40). Negative correlations were found between Spiroplasma and the pathogens Rickettsia spp. and Borrelia valaisiana in individual I. ricinus, but positive correlations were found between Spiroplasma and the symbionts Lariskella and Rickettsiella at the population level. Further studies are needed to examine whether presence of Spiroplasma in Ixodes spp. ticks has any effect on acquisition, replication or transmission of tick-borne arboviruses such as TBEV or protozoa such as Babesia spp., or indeed any effect on the viability of the ticks themselves.
The molecular analysis revealed almost no differences between the Spiroplasma isolated from colonised I. persulcatus of Russian origin and cultured for 2.5 months in cell lines derived from heterologous tick species (I. ricinus, I. scapularis and R. microplus), Spiroplasma DNA detected in whole ticks from the same colony and the Spiroplasma isolated into I. scapularis cells from Japanese I. persulcatus (10). Ambiguity was seen in a single nucleotide in the ~476 bp fragment of the 16S rRNA gene amplified in the present study, and the same nucleotide showed a difference when compared with the sequence from the Japanese isolate. The rpoB gene fragment analysed in our study was longer than the 16S rRNA sequences, providing more phylogenetic information, although the shorter length of most of the published sequences from other tick species (4) reduced the coverage available for comparison. The overall topology of the tree and the relationship between strains in the tick-borne Spiroplasma branch were very close to the results based on the 16S rRNA gene, although neither of these gene fragments are sufficient to confidently separate Spiroplasma strains or species. Nevertheless, as reported previously (21) it is clear that the spiroplasmas harboured by different Ixodes spp. ticks are not identical, and also differ from those harboured by Dermacentor spp. ticks from broadly contiguous geographic regions.
In conclusion, our study has shown that efficient vertical transmission of Spiroplasma can be maintained in I. persulcatus ticks under laboratory colony conditions for at least three generations, and has confirmed that co-cultivation of internal organs with tick cell lines is a simple and effective technique for in vitro isolation of intracellular tick symbionts such as Spiroplasma spp. Further molecular analysis of the cultured Spiroplasma strains derived from I. persulcatus, either by Sanger sequencing of additional genes or by whole genome sequencing, is required to clarify the phylogenetic relationships between them and Spiroplasma harboured by I. persulcatus of different geographical origins and by other tick species, and to facilitate an accurate taxonomic classification of these genotypes.
The original sequences generated for this study are publicly available in the NCBI Genbank repository under accession numbers MW492370, MW498416, MW498417, MW528409-MW528411.
The animal study was reviewed and approved by Czech Academy of Sciences (approval no. 161/2010).
AB, VH, JE, TV, MP, and LB-S carried out the experimental work. AB, VH, AP, and LB-S analysed the data. JC, IK, and DR carried out the field work. LB-S conceived the study and drafted the manuscript. AB, VH, JC, DR, and AP revised the manuscript. All authors reviewed and agreed to the final version.
Funding was provided by the United Kingdom Biotechnology and Biological Sciences Research Council grant BB/P024270/1 (AB, LB-S), Czech Science Foundation 20-14325S and 20-30500S, Ministry of Education, Youth and Sports project FIT (Pharmacology, Immunotherapy, nanoToxicology) CZ.02.1.01/0.0/0.0/15 003/0000495 (VH, TV, MP, JE, DR), National Agency for Agricultural Research, The Ministry of Agriculture of Czech Republic grant QK1920258 (VH) and State scientific theme of the Russian Academy of Sciences No. 0416-2021-003 (IK).
The authors declare that the research was conducted in the absence of any commercial or financial relationships that could be construed as a potential conflict of interest.
We would like to thank the Tick Cell Biobank and Profs Ulrike Munderloh and Timothy Kurtti (University of Minnesota) for providing the tick cell lines used in this study, and Elena K. Doroshchenko, Oksana V. Lisak and Olga V. Suntsova for help with tick collection.
1. Noda H, Munderloh UG, Kurtti TJ. Endosymbionts of ticks and their relationship to Wolbachia spp. and tick-borne pathogens of humans and animals. Appl Env Microbiol. (1997) 63:3926–32. doi: 10.1128/AEM.63.10.3926-3932.1997
2. Lo N, Beninati T, Sassera D, Bouman EAP, Santagati S, Gern L, et al. Widespread distribution and high prevalence of an alpha-proteobacterial symbiont in the tick Ixodes ricinus. Environ Microbiol. (2006) 8:1280–7. doi: 10.1111/j.1462-2920.2006.01024.x
3. Duron O, Binetruy F, Noel V, Cremaschi J, McCoy KD, Arnathau C, et al. Evolutionary changes in symbiont community structure in ticks. Molec Ecol. (2017) 26:2905–21. doi: 10.1111/mec.14094
4. Binetruy F, Bailly X, Chevillon C, Martin OY, Bernasconi MV, Duron O. Phylogenetics of the Spiroplasma ixodetis endosymbiont reveals past transfers between ticks and other arthropods. Ticks Tick Borne Dis. (2019) 10:575–84. doi: 10.1016/j.ttbdis.2019.02.001
5. Mediannikov O, Nguyen T, Bell-Sakyi L, Padmanabhan R, Fournier P, Raoult D. Genome sequence and description of Occidentia massiliensis gen. nov., sp. nov., a new member of the family Rickettsiaceae. Standards Genomic Sci. (2014) 9:9. doi: 10.1186/1944-3277-9-9
6. Kurtti TJ, Munderloh UG, Andreadis TG, Magnarelli LA, Mather TN. Tick cell culture isolation of an intracellular prokaryote from the tick Ixodes scapularis. J Invert Pathol. (1996) 67:318–21. doi: 10.1006/jipa.1996.0050
7. Tijsse-Klasen E, Braks M, Scholte E-J, Sprong H. Parasites of vectors - Ixodiphagus hookeri and its Wolbachia symbionts in ticks in the Netherlands. Parasites Vectors. (2011) 4:228. doi: 10.1186/1756-3305-4-228
8. Mediannikov O, Subramanian G, Sekeyova Z, Bell-Sakyi L, Raoult D. Isolation of Arsenophonus nasoniae from Ixodes ricinus ticks in Slovakia. Ticks Tick Borne Dis. (2012) 3:366–9. doi: 10.1016/j.ttbdis.2012.10.016
9. Plantard O, Bouju-Albert A, Malard M-A, Hermouet A, Capron G, Verheyden H. Detection of Wolbachia in the tick Ixodes ricinus is due to the presence of the Hymenoptera endoparasitoid Ixodiphagus hookeri. PLoS ONE. (2012) 7:e30692. doi: 10.1371/journal.pone.0030692
10. Thu MJ, Qiu Y, Kataoka-Nakamura C, Sugimoto C, Katakura K, Isoda N, et al. Isolation of Rickettsia, Rickettsiella, and Spiroplasma from questing ticks in Japan using arthropod cells. Vector-Borne Zoonot Dis. (2019) 19:474–85. doi: 10.1089/vbz.2018.2373
11. Simser JA, Palmer AT, Munderloh UG, Kurtti TJ. Isolation of a spotted fever group rickettsia, Rickettsia peacockii, in a Rocky Mountain wood tick, Dermacentor andersoni, cell line. Appl Env Microbiol. (2001) 67:546–52. doi: 10.1128/AEM.67.2.546-552.2001
12. Mattila JT, Burkhardt NY, Hutcheson HJ, Munderloh UG, Kurtti TJ. Isolation of cell lines and a rickettsial endosymbiont from the soft tick Carios capensis (Acari: Argasidae: Ornithodorinae). J Med Entomol. (2007) 44:1091–101. doi: 10.1093/jmedent/44.6.1091
13. Alberdi MP, Nijhof AM, Jongejan F, Bell-Sakyi L. Tick cell culture isolation and growth of Rickettsia raoultii from Dutch Dermacentor reticulatus ticks. Ticks Tick Borne Dis. (2012) 3:349–54. doi: 10.1016/j.ttbdis.2012.10.020
14. Ferrari FAG, Goddard J, Moraru GM, Smith WEC, Varela-Stokes AS. Isolation of “Candidatus Rickettsia andeanae” (Rickettsiales: Rickettsiaceae) in embryonic cells of naturally infected Amblyomma maculatum (Ixodida: Ixodidae). J Med Entomol. (2013) 50:1118–25. doi: 10.1603/ME13010
15. Kurtti TJ, Felsheim RF, Burkhardt NY, Oliver JD, Heu CC, Munderloh UG. Rickettsia buchneri sp. nov., a rickettsial endosymbiont of the blacklegged tick Ixodes scapularis. Int J Syst Evol Microbiol. (2015) 65:965–70. doi: 10.1099/ijs.0.000047
16. Santibáñez S, Portillo A, Palomar AM, Bell-Sakyi L, Romero L, Oteo JA. Isolation and maintenance of Rickettsia raoultii in a Rhipicephalus sanguineus tick cell line. Microbes Infect. (2015) 17:866–9. doi: 10.1016/j.micinf.2015.09.018
17. Niebylski ML, Peacock MG, Fischer ER, Porcella SF, Schwan TG. Characterization of an endosymbiont infecting wood ticks, Dermacentor andersoni, as a member of the genus Francisella. Appl Environ Microbiol. (1997) 63:3933–40. doi: 10.1128/AEM.63.10.3933-3940.1997
18. Yunker CE, Tully JG, Cory J. Arthropod cell lines in the isolation and propagation of tickborne spiroplasmas. Curr Microbiol. (1987) 15:45–50. doi: 10.1007/BF01577213
19. Henning K, Greiner-Fischer S, Hotzel H, Ebsen M, Theegarten D. Isolation of a Spiroplasma sp. from an Ixodes tick. Int J Med Microbiol. (2006) 296:157–61. doi: 10.1016/j.ijmm.2006.01.012
20. Bell-Sakyi L, Palomar AM, Kazimirova M. Isolation and propagation of a Spiroplasma sp. from Slovakian Ixodes ricinus ticks in Ixodes spp. cell lines. Ticks Tick Borne Dis. (2015) 6:601–6. doi: 10.1016/j.ttbdis.2015.05.002
21. Palomar AM, Premchand-Branker S, Alberdi P, Belova O, Moniuszko-Malinowska A, Kahl O, et al. Isolation of known and potentially pathogenic tick-borne microorganisms from European ixodid ticks using tick cell lines. Ticks Tick Borne Dis. (2019) 10:628–38. doi: 10.1016/j.ttbdis.2019.02.008
22. Yamaguti N, Tipton VJ, Keegan HL, Toshioka S. Ticks of Japan, Korea, and the Ryukyu Islands. Brigham Young University Science Bulletin, Biological Series. (1971) 15:1. Available online at: https://scholarsarchive.byu.edu/byuscib/vol15/iss1/1 (accessed May 22, 2020).
23. Shpynov S. Ixodes persulcatus, a major vector of Alphaproteobacteria in Russia. Ticks Tick Borne Dis. (2012) 3:304–6. doi: 10.1016/j.ttbdis.2012.10.029
24. Cerný J, Buyannemekh B, Needham T, Gankhuyag G, Dashzeveg Oyuntsetseg D. Hard ticks and tick-borne pathogens in Mongolia – a review. Ticks Tick Borne Dis. (2019) 10:101268. doi: 10.1016/j.ttbdis.2019.101268
25. Jaenson GT, Wilhelmsson P. First records of tick-borne pathogens in populations of the taiga tick Ixodes persulcatus in Sweden. Parasites Vectors. (2019) 12:559. doi: 10.1186/s13071-019-3813-0
26. Mediannikov O, Ivanov LI, Nishikawa M, Saito R, Sidelnikov IN, Zdanovskaia NI, et al. Microorganism “Montezuma” of the order Rickettsiales: the potential causative agent of tick-borne disease in the Far East of Russia. Zh Mikrobiol Epidemiol Immunobiol. (2004) 1:7–13.
27. Eremeeva ME, Oliveira A, Moriarity J, Robinson JB, Tokarevich NK, Antyukova LP, et al. Detection and identification of bacterial agents in Ixodes persulcatus Schulze ticks from the North Western Region of Russia. Vector-Borne Zoonot Dis. (2007) 7:426–36. doi: 10.1089/vbz.2007.0112
28. Mukhacheva TA, Kovalev SY. Bacteria of the family ‘Candidatus Midichloriaceae' in sympatric zones of Ixodes ticks: genetic evidence for vertical transmission. Microb Ecol. (2017) 74:185–93. doi: 10.1007/s00248-017-0932-z
29. Qiu Y, Nakao R, Ohnuma A, Kawamori F, Sugimoto C. Microbial population analysis of the salivary glands of ticks; a possible strategy for the surveillance of bacterial pathogens. PLoS ONE. (2014) 9:e103961. doi: 10.1371/journal.pone.0103961
30. Süss J. Tick-borne encephalitis 2010: epidemiology, risk areas, and virus strains in Europe and Asia – an overview. Ticks Tick Borne Dis. (2011) 2:2–15. doi: 10.1016/j.ttbdis.2010.10.007
31. Yi S, Jiang H, Cao W, Fu W, Ju W, Wang X. Prevalence of Candidatus Rickettsia tarasevichiae-like bacteria in ixodid ticks at 13 sites on the Chinese–Russian border. J Med Entomol. (2014) 51:1304–7. doi: 10.1603/ME13189
32. Kurilshokov A, Livanona NN, Fomenko NV, Tupikin AE, Rar VA, Kabilov MR, et al. Comparative metagenomic profiling of symbiotic bacterial communities associated with Ixodes persulcatus, Ixodes pavlovskyi and Dermacentor reticulatus ticks. PLoS ONE. (2015) 10:e0131413. doi: 10.1371/journal.pone.0131413
33. Igolkina Y, Bondarenko E, Rar V, Epikhina T, Vysochina N, Pukhovskaya N, et al. Genetic variability of Rickettsia spp. in Ixodes persulcatus ticks from continental and island areas of the Russian Far East. Ticks Tick Borne Dis. (2016) 7:1284–9. doi: 10.1016/j.ttbdis.2016.06.005
34. Sormunen JJ, Penttinen R, Klemola T, Hänninen J, Vuorinen I, Laaksonen M, et al. Tick-borne bacterial pathogens in southwestern Finland. Parasites Vectors. (2016) 9:168. doi: 10.1186/s13071-016-1449-x
35. Livanova NN, Fomenko NV, Akimov IA, Ivanov MJ, Tikunova NV, Armstrong R, et al. Dog survey in Russian veterinary hospitals: tick identification and molecular detection of tick-borne pathogens. Parasites Vectors. (2018) 11:591. doi: 10.1186/s13071-018-3161-5
36. Narankhajid M, Yeruult C, Gurbadam A, Battsetseg J, Aberle SW, Bayartogtokh B, et al. Some aspects on tick species in Mongolia and their potential role in the transmission of equine piroplasms, Anaplasma phagocytophilum and Borrelia burgdorferi L. Parasitol Res. (2018) 117:3557–66. doi: 10.1007/s00436-018-6053-x
37. Rar V, Livanova N, Sabitova Y, Igolkina Y, Tkachev S, Tikunov A, et al. Ixodes persulcatus/pavlovskyi natural hybrids in Siberia: occurrence in sympatric areas and infection by a wide range of tick-transmitted agents. Ticks Tick Borne Dis. (2019) 10:101254. doi: 10.1016/j.ttbdis.2019.05.020
38. Kholodilov IS, Litov AG, Klimentov AS, Belova OA, Polienko AE, Nikitin NA. Isolation and characterization of Alongshan virus in Russia. Viruses. (2020) 12:362. doi: 10.3390/v12040362
39. Popov VL, Korenberg EI, Nefedova VV, Han VC, Wen JW, Kovalevskii YV, et al. Ultrastructural evidence of the ehrlichial developmental cycle in naturally infected Ixodes persulcatus ticks in the course of coinfection with Rickettsia, Borrelia, and a flavivirus. Vector-Borne Zoonot Dis. (2007) 7:699–716. doi: 10.1089/vbz.2007.0148
40. Aivelo T, Norberg A, Tschirren B. Bacterial microbiota composition of Ixodes ricinus ticks: the role of environmental variation, tick characteristics and microbial interactions. Peer J. (2019) 7:e8217. doi: 10.7717/peerj.8217
41. Cafiso A, Sassera D, Romeo C, Serra V, Hervet C, Bandi C, et al. Midichloria mitochondrii, endosymbiont of Ixodes ricinus: evidence for the transmission to the vertebrate host during the tick blood meal. Ticks Tick Borne Dis. (2019) 10:5–12. doi: 10.1016/j.ttbdis.2018.08.008
42. Guizzo MG, Neupane S, Kucera M, Perner J, Frantová H, da Silva Vaz I, et al. Poor unstable midgut microbiome of hard ticks contrasts with abundant and stable monospecific microbiome in ovaries. Front Cell Infect Microbiol. (2020) 8:211. doi: 10.3389/fcimb.2020.00211
43. Morimoto S, Kurtti TJ, Noda H. In vitro cultivation and antibiotic susceptibility of a Cytophaga- like intracellular symbiote isolated from the tick Ixodes scapularis. Curr Microbiol. (2006) 52:324–9. doi: 10.1007/s00284-005-0349-7
44. Kurtti TJ, Munderloh UG, Ahlstrand GG, Johnson RC. Borrelia burgdorferi in tick cell culture: growth and cellular adherence. J Med Entomol. (1988) 25:256–61. doi: 10.1093/jmedent/25.4.256
45. Simser JA, Palmer AT, Fingerle V, Wilske B, Kurtti TJ, Munderloh UG. Rickettsia monacensis sp. nov., a spotted fever group rickettsia, from ticks (Ixodes ricinus) collected in a European city park. Appl Env Microbiol. (2002) 68:4559–66. doi: 10.1128/AEM.68.9.4559-4566.2002
46. Munderloh UG, Liu Y, Wang M, Chen C, Kurtti TJ. Establishment, maintenance and description of cell lines from the tick Ixodes scapularis. J Parasitol. (1994) 80:533–43. doi: 10.2307/3283188
47. Bell-Sakyi L. Ehrlichia ruminantium grows in cell lines from four ixodid tick genera. J Comp Pathol. (2004) 130:285–93. doi: 10.1016/j.jcpa.2003.12.002
48. Munderloh UG, Jauron SD, Fingerle V, Leitritz L, Hayes SF, Hautman JM, et al. Invasion and intracellular development of the human granulocytic ehrlichiosis agent in tick cell culture. J Clin Microbiol. (1999) 37:2518–24. doi: 10.1128/JCM.37.8.2518-2524.1999
49. Fukatsu T, Nikoh N. Endosymbiotic microbiota of the bamboo pseudococcid Antonina crawii (Insecta, Homoptera). Appl Environ Microbiol. (2000) 66:643–50. doi: 10.1128/AEM.66.2.643-650.2000
50. Haselkorn TS, Markow TA, Moran NA. Multiple introductions of the Spiroplasma bacterial endosymbiont into Drosophila. Mol Ecol. (2009) 18:1294–305. doi: 10.1111/j.1365-294X.2009.04085.x
51. Kumar S, Stecher G, Li M, Knyaz C, Tamura K. MEGA X: molecular evolutionary genetics analysis across computing platforms. Mol Biol Evol. (2018) 35:1547–9. doi: 10.1093/molbev/msy096
52. Kimura M. A simple method for estimating evolutionary rate of base substitutions through comparative studies of nucleotide sequences. J Mol Evol. (1980) 16:111–20. doi: 10.1007/BF01731581
53. Schwarz G. Estimating the dimension of a model. Ann Stat. (1978) 6:461–4. doi: 10.1214/aos/1176344136
54. Black WC, Piesman J. Phylogeny of hard- and soft-tick taxa (Acari: Ixodida) based on mitochondrial 16S rDNA sequences. Proc Natl Acad Sci USA. (1994) 91:10034–8. doi: 10.1073/pnas.91.21.10034
55. Duron O, Noel V, McCoy KD, Bonazzi M, Sidi-Boumedine K, Morel O, et al. The recent evolution of a maternally-inherited endosymbiont of ticks led to the emergence of the Q fever pathogen, Coxiella burnetii. PLoS Path. (2015) 11:e1004892. doi: 10.1371/journal.ppat.1004892
56. Olivieri E, Epis S, Castelli M, Boccazzi IV, Romeo C, Desiro A, et al. Tissue tropism and metabolic pathways of Midichloria mitochondrii suggest tissue-specific functions in the symbiosis with Ixodes ricinus. Ticks Tick Borne Dis. (2019) 10:1070–7. doi: 10.1016/j.ttbdis.2019.05.019
57. Hornok S, Meli ML, Perreten A, Farkas R, Willi B, Beugnet F, et al., Hofmann-Lehmann R. Molecular investigation of hard ticks (Acari: Ixodidae) and fleas (Siphonaptera: Pulicidae) as potential vectors of rickettsial and mycoplasmal agents. Vet Microbiol. (2010) 140:98–104. doi: 10.1016/j.vetmic.2009.07.013
58. Klubal R, Kopecky J, Nesvorna M, Sparagano OAE, Thomayerova J, Hubert J. Prevalence of pathogenic bacteria in Ixodes ricinus ticks in Central Bohemia. Exp Appl Acarol. (2016) 68:127–37. doi: 10.1007/s10493-015-9988-y
59. Krawczyk AI, van Duijvendijk GLA, Swart A, Heylen D, Jaarsma RI, Jacobs FHH, et al. Effect of rodent density on tick and tick-borne pathogen populations: consequences for infectious disease risk. Parasites Vectors. (2020) 13:34. doi: 10.1186/s13071-020-3902-0
60. Van Oosten AR, Duron O, Heylen DJA. Sex ratios of the tick Ixodes arboricola are strongly female-biased, but there are no indications of sex-distorting bacteria. Ticks Tick Borne Dis. (2018) 9:307–13. doi: 10.1016/j.ttbdis.2017.11.004
Keywords: tick cell line, endosymbiont, Spiroplasma, tick colony, Ixodes persulcatus
Citation: Beliavskaia A, Hönig V, Erhart J, Vyhlidalova T, Palus M, Cerny J, Kozlova I, Ruzek D, Palomar AM and Bell-Sakyi L (2021) Spiroplasma Isolated From Third-Generation Laboratory Colony Ixodes persulcatus Ticks. Front. Vet. Sci. 8:659786. doi: 10.3389/fvets.2021.659786
Received: 28 January 2021; Accepted: 26 February 2021;
Published: 26 March 2021.
Edited by:
Cornelia Silaghi, Friedrich-Loeffler-Institute, GermanyReviewed by:
Alessandra Cafiso, University of Milan, ItalyCopyright © 2021 Beliavskaia, Hönig, Erhart, Vyhlidalova, Palus, Cerny, Kozlova, Ruzek, Palomar and Bell-Sakyi. This is an open-access article distributed under the terms of the Creative Commons Attribution License (CC BY). The use, distribution or reproduction in other forums is permitted, provided the original author(s) and the copyright owner(s) are credited and that the original publication in this journal is cited, in accordance with accepted academic practice. No use, distribution or reproduction is permitted which does not comply with these terms.
*Correspondence: Lesley Bell-Sakyi, bC5iZWxsLXNha3lpQGxpdmVycG9vbC5hYy51aw==
Disclaimer: All claims expressed in this article are solely those of the authors and do not necessarily represent those of their affiliated organizations, or those of the publisher, the editors and the reviewers. Any product that may be evaluated in this article or claim that may be made by its manufacturer is not guaranteed or endorsed by the publisher.
Research integrity at Frontiers
Learn more about the work of our research integrity team to safeguard the quality of each article we publish.