- 1Institute of Bacterial Infections and Zoonoses (IBIZ), Friedrich-Loeffler-Institute, Federal Research Institute for Animal Health, Jena, Germany
- 2Department of Immunology, Fraunhofer Institute for Cell Therapy and Immunology, Leipzig, Germany
- 3Institute of Animal Hygiene and Veterinary Public Health, University of Leipzig, Leipzig, Germany
The zoonosis Q fever is caused by the obligate intracellular bacterium Coxiella burnetii. Besides the main transmission route via inhalation of contaminated aerosols, ticks are discussed as vectors since the first isolation of the pathogen from a Dermacentor andersonii tick. The rare detection of C. burnetii in ticks and the difficult differentiation of C. burnetii from Coxiella-like endosymbionts (CLEs) are questioning the relevance of ticks in the epidemiology of Q fever. In this review, literature databases were systematically searched for recent prevalence studies concerning C. burnetii in ticks in Europe and experimental studies evaluating the vector competence of tick species. A total of 72 prevalence studies were included and evaluated regarding DNA detection methods and collection methods, country, and tested tick species. Specimens of more than 25 different tick species were collected in 23 European countries. Overall, an average prevalence of 4.8% was determined. However, in half of the studies, no Coxiella-DNA was detected. In Southern European countries, a significantly higher prevalence was observed, possibly related to the abundance of different tick species here, namely Hyalomma spp. and Rhipicephalus spp. In comparison, a similar proportion of studies used ticks sampled by flagging and dragging or tick collection from animals, under 30% of the total tick samples derived from the latter. There was no significant difference in the various target genes used for the molecular test. In most of the studies, no distinction was made between C. burnetii and CLEs. The application of specific detection methods and the confirmation of positive results are crucial to determine the role of ticks in Q fever transmission. Only two studies were available, which assessed the vector competence of ticks for C. burnetii in the last 20 years, demonstrating the need for further research.
Introduction
Coxiella burnetii as the causative agent of the zoonosis Q fever is distributed worldwide, except New Zealand. This infectious disease may have a significant impact on animal welfare, human health, and economies (1, 2). Coxiella burnetii is a gram-negative bacterium that replicates as an obligate intracellular pathogen under acidic and microaerophilic conditions in phagolysosome-like compartments of cells, predominantly macrophages (3). Infection of hosts mainly occurs due to inhalation of contaminated dust and aerosols (4). During infection, C. burnetii shows a tropism for the reproductive tissue and the mammary gland. Therefore, C. burnetii is primarily shed during parturition and via the milk (5). The considered main reservoirs for human infections are domestic ruminants, whereas other species, such as dogs or horses, can play a minor role as carriers (6). In their birth products, large amounts of infectious agents can be detected (7, 8). As the infective dose by inhalation is <10 bacteria, farmers, and veterinarians are especially at high risk of acquiring this disease through contact with infected animals and their products (9, 10). Coxiella burnetii develops spore-like forms, which are resistant to environmental stressors such as desiccation or sunlight. Therefore, the bacteria survive under adverse conditions over long periods in the soil or other dry substances (11).
An infection in ruminants, also termed coxiellosis, is often asymptomatic and not noticed until human Q fever cases occur (12). Decrease in fertility and increase of abortion and stillbirth are common indications of an ongoing Q fever disease in herds or flocks of ruminants (13, 14).
Outbreaks of Q fever, as seen in the Netherlands in 2007–2010, clearly show the huge impact of this infectious disease not only on agricultural economics but also on human health (15, 16). Acute human Q fever infection presents mainly flu-like symptoms with possible complications concerning the lung and liver. Although 60% of acute cases remain asymptomatic, in 1–5% of cases, chronic Q fever may develop (17, 18). This often affects the heart valves as endocarditis. Antibiotic treatment is mandatory over months and, in some cases, up to several years (17–19). Besides the long and difficult treatment, the disease can also be fatal in acute and chronic cases. Although the mortality of acute Q fever was assessed to be 1% in a study on hospitalized patients, the mortality in chronic cases has been reported as 13% and even up to 38% during the Dutch outbreak mentioned earlier (1, 18, 20).
The bacterium is known as an infectious agent since the 1930s when it caused an outbreak of query fever among abattoir workers. In the years after its first isolation from a Dermacentor andersonii tick (21), early experimental studies showed horizontal transmission of the agent from ticks to mammalian hosts and suggested that several tick species, e.g., Haemaphysalis humerosa, Haemaphysalis bispinosa, and Rhipicephalus sanguineus, may be capable of transmitting C. burnetii (22–25). Although transstadial transmission was shown for most of the examined tick species, the transovarial transmission was reported rarely (25, 26). Later, it was demonstrated that Hyalomma dromedarii infected by inoculation excreted C. burnetii with their saliva (27). Duron et al. summarized the vector competence data for seven hard tick species (28). Hence, since its first detection, C. burnetii has been discussed as a ruminant-associated tick-borne bacterium.
During outbreaks of Q fever, ticks are regularly tested for C. burnetii. Until today, no C. burnetii-positive ticks associated with outbreaks have been documented. Human infection after tick exposure has been reported, but due to the predominant infection route by inhalation, the source of infection remains unclear (29, 30).
Besides pathogen transmission, ticks are host to many endosymbionts and harbor a diverse microbiome (31). Although C. burnetii was the only known member of the genus Coxiella for a long time, closely related bacteria were found in recent studies, referred to as Coxiella-like endosymbionts (CLEs) (32, 33). This heterogenic group of bacteria with different genome sizes and gene content shows up to 97% genome identity with C. burnetii (33–37). Genetically, CLEs are classified into four clades (A–D), and C. burnetii belongs to clade A, which otherwise contains CLE associated with soft ticks (38).
Coxiella-like endosymbionts were detected in many different tick species, and for some species, an obligatory mutualism was proven (39–41). As obligate hematophagous arthropods, ticks are dependent on external vitamin B synthesis, likely supplied by the harbored bacteria (40, 42). Furthermore, a positive effect on fecundity was shown for R. sanguineus. Treatment with ofloxacin resulted in a significantly lower egg mass, hatching rate, and viability of larvae in this tick species (39). Similar results were shown for Haemaphysalis longicornis, Rhipicephalus microplus, and Rhipicephalus haemaphysaloides, which were treated with tetracycline and kanamycin, respectively, indicating the influence of CLE on reproduction (43–45).
CLEs seem to be apathogenic, but single studies showed pathogenic potential in particular cases. Fatal infection with CLE was occasionally observed in different bird species (46–48). Furthermore, a skin-associated inflammation caused by the tick-borne bacterium Candidatus Coxiella massiliensis was described (49).
The polymerase chain reaction was established in the 1980s and was subsequently used as the predominant detection method. Until then, ticks were primarily tested for the occurrence of C. burnetii and related pathogens with staining methods and animal infection experiments (50–52). Because C. burnetii is difficult to isolate and cultivate as an obligate intracellular organism, molecular diagnostic using PCR is the method of choice for its detection. Various protocols target several plasmids and chromosomal genes, for example, IS1111, icd, com1, sodB, and GroEL/htpB (53–55). The target gene IS1111 is a transposase-like insertion sequence, and the amount of copies from 7 to 110 per genome varies between isolates. Thus, the use for quantification is limited, but due to the higher number of targets per bacterium, this signal amplification leads to a higher sensitivity compared with single-copy targets (53). In contrast, icd, encoding for the isocitrate dehydrogenase gene, and com1, encoding for the C. burnetii outer membrane protein 1, are single-copy genes allowing quantification (53, 54). The commonly used target genes for C. burnetii cross-react with CLE and may lead to misidentification (28, 56). In a study by Duron, roughly one-third of CLE-positive ticks were positive for the IS1111 element (57). Furthermore, IS1111 was shown to be the most unspecific marker for detection of C. burnetii in CLE-positive ticks, followed by GroEL/htpAB (for chaperone heat shock protein), whereas icd was not amplified in the samples (56). However, the icd sequence of a novel CLE derived from Carios capensis soft ticks was >90% similar to C. burnetii (58). As a consequence, usage of a target gene of low specificity could lead to an overestimation of the role of ticks as host and vector of C. burnetii. To distinguish between C. burnetii and CLE, there is no specific method available, owing to the fact that the group of CLE is very heterogeneous. Therefore, the most reliable method is the sequencing of PCR-positive samples using highly conserved genes such as rrs or groEL (56, 59).
Ticks (Ixodida) are obligate blood-feeding ectoparasites with a global distribution. A total of 67 tick species are reported in Europe and Northern Africa (60). Out of these, only a small number, i.e., 15–17 hard tick species, depending on their confirmed taxonomic status as a distinct species or subspecies, have been found to harbor either C. burnetii or CLE (28, 61). When considering a particular tick species to serve as a vector for a given pathogen, certain aspects of the tick's life cycle should be considered in addition to the sole finding of molecular traces or even morphological structures within an examined tick. This information will help to understand further whether this tick species has vector competence, defined as the capability to transmit a given pathogen horizontally or vertically, which is an essential aspect of the vector capacity. The latter is more relevant for determining the infection risk, as it includes parameters such as longevity, feeding behavior (duration, frequency, and preferred blood source), population density, and frequency of host contact encounters. In this regard, ticks, which are feeding on different hosts in every life stage (three-host-ticks) with a large geographical range, high population densities, and promiscuous feeding behavior are relevant, when it comes to pathogen transmission. The most common hard tick of Europe, the castor bean tick Ixodes ricinus, is fulfilling these criteria, making it the most important vector tick species for bacterial and viral tick-borne diseases, e.g., Borrelia burgdorferi s.l. or tick-borne encephalitis virus, in Europe (62). Such vector-borne agents, some with a wide range of host species, may have their reservoir in wildlife, leading to a sylvatic transmission cycle between arthropods and wild animals. In addition, transmission can also occur in an urban or domestic cycle, including pets, livestock, or humans. Although I. ricinus is ubiquitous in Europe, other relevant tick species are not, and only for some geo-referenced distribution maps are available, e.g., for both Dermacentor species (63). However, the geographical distribution of a particular tick species is not static but rather subject to permanent habitat changes due to increased movement of humans, animals, and goods and, very importantly, the increasing ambient temperatures as major drivers. The high frequency of recent introductions of Hyalomma spp. ticks into temperate Europe is impressive sign of a very menacing trend of potent vector tick species moving into new areas (64, 65).
Recent prevalence studies using molecular methods aim at determining the percentage of ticks carrying C. burnetii among other diverse tick-borne pathogens. This allows surveillance of C. burnetii in ticks and benefits the identification of a potential risk of acquiring a Q fever infection by a tick-bite for humans or domestic animals.
Different methods exist for the sampling of ticks. As most European hard ticks seek hosts by questing on the vegetation and naturally intend to be wiped up by a passing host, the mainly used collection method is flagging or dragging. Using this method, a cotton blanket is dragged or flagged over the vegetation, considering that both methods favor different tick species (66). Ticks are stripped off and are hindered in their motion by the weave. The success of this method depends on the texture of the blanket and the structure of the vegetation (67). Activity peaks of most questing ticks are in spring and autumn. However, other studies observed a trend to a unimodal peak with the highest activity in the spring months (68, 69). Ticks can also be removed directly from their host. This method is used to examine the burden of pathogens associated with these animals and for the sampling of hunting ticks, such as Hyalomma spp., which are less likely to be collected by flagging (70).
This review gives a comprehensive overview of the present literature on C. burnetii in ticks and discusses the vector competence of European tick species for the Q fever agent. To this end, data of prevalence studies conducted in Europe concerning the occurrence of C. burnetii in ticks were systematically compiled and analyzed regarding the spatial occurrence, molecular detection method used, and the frequency of detection per tick species, as well as the detection of CLE. Furthermore, experimental approaches to investigate the vector competence of tick species for C. burnetii were collected. The determination of the vector competence of ticks is important to assess the role of ticks as a reservoir in Q fever epidemiology.
Materials and Methods
Studies about C. burnetii in ticks were classified into two different categories. One group investigates the prevalence of ticks for C. burnetii in Europe. The other group discusses the vector competence of ticks based on experimental approaches on ticks infected under laboratory conditions. For identification of studies, the databases Pubmed and Web of Science were systematically searched for articles in English, published between 2000 and 2020. Search terms were “coxiella tick,” “coxiella-like,” “coxiella vector competence.” The search was completed on October 27, 2020. Additional studies were included using Google Scholar. References were sorted by the use of Endnote X7 (Clarivate Analytics, Philadelphia, USA). Only studies using DNA detection methods for Coxiella spp. on hard ticks obtained in Europe were included as prevalence studies. The definition of Europe used in this study refers to a geographical demarcation as von Strahlenberg's, including all countries of which the major part is situated between the Urals and the Bosphorus. Regions were defined according to the United Nations geoscheme for Europe in Southern Europe, Eastern Europe, Northern Europe, and Western Europe (71).
Further criteria for eligibility as prevalence studies were stated information of the number of tested ticks, the number of Coxiella-positive ticks, or amount and description of tick pools and at a minimum description of the tick genus or species name.
Data were extracted regarding information about the country in which the ticks were obtained. Furthermore, the number of tested and Coxiella-positive ticks and tick pools and the collection and detection method were included in the analysis. All publications were read, and data were extracted by two persons independently.
The proportion of positive ticks was calculated as the ratio of positive ticks to the total number of tested ticks. Prevalence was defined as the proportion of positive ticks multiplied by 100. When ticks were tested in pools, the proportion was estimated as minimum infection rate (MIR) using the formula
Studies were evaluated, and confidence interval was calculated using Excel 2016 (Microsoft Corporation, Redmond, USA). For statistical analysis, the Kruskal–Wallis test and Mann–Whitney U test for data lacking normal distribution were performed using SPSS V.22 (IBM, Armonk, USA), and results were considered significant in the case of p < 0.05.
Results
A total of 590 studies were found using the mentioned search terms. Additionally, two studies using the platform Google Scholar were included. Based on the title and abstract, 502 studies were excluded. Main reasons for exclusion were non-European studies (n = 272), no hard ticks tested (n = 120), or reviews (n = 44); 66 studies were excluded for other reasons (Figure 1: PRISMA analysis). After full-text analysis, 16 further studies were removed. Finally, 72 publications in which the prevalence of C. burnetii in ticks collected in European countries were examined, compiled, and evaluated. Additionally, two vector competence studies were included.
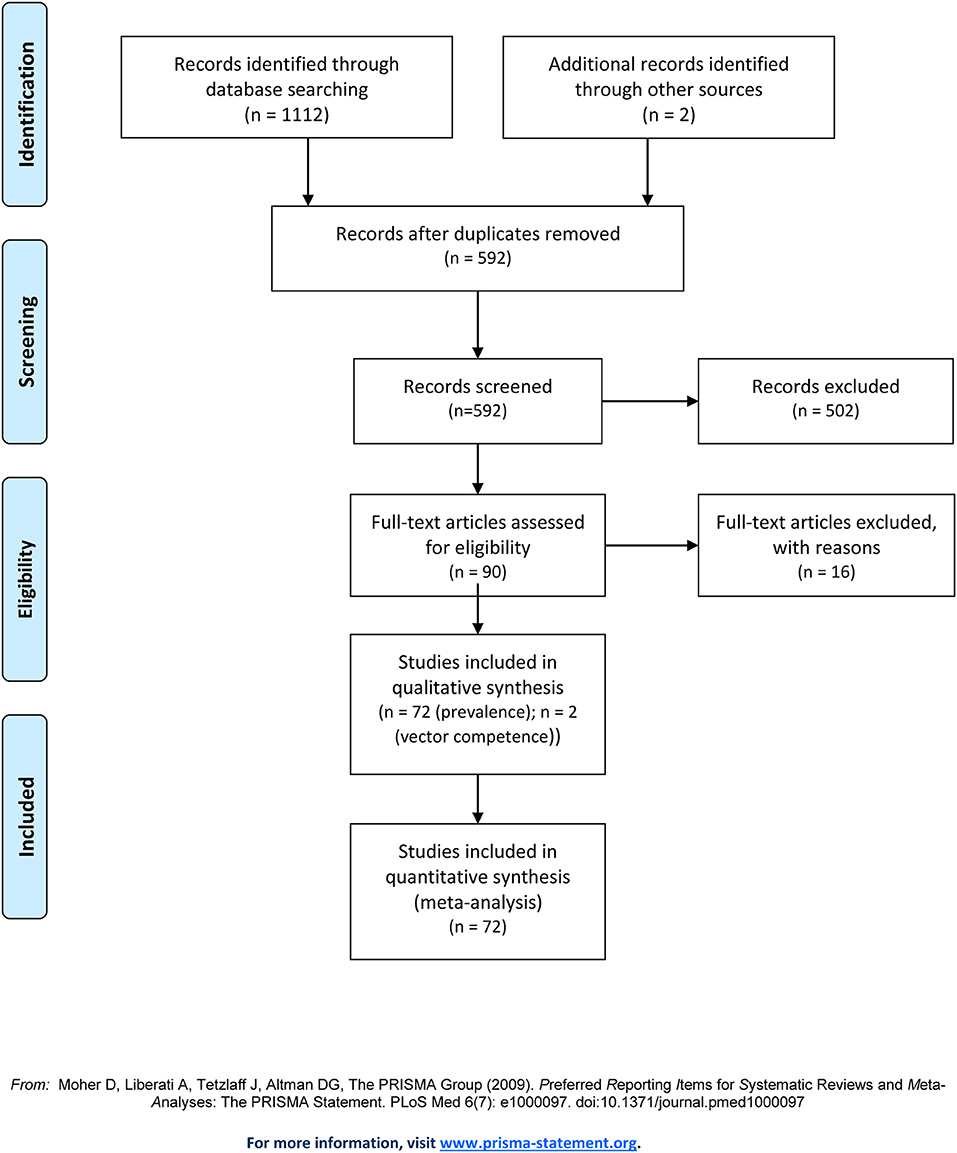
Figure 1. PRISMA flow chart for selection of studies, including the reasons for exclusion from the analysis.
Prevalence Studies in Europe—A Systematic Analysis
Prevalence studies were analyzed by country, the number of tested ticks, tick species, the method used for tick collection, and the method used for detection of C. burnetii (Supplementary Table 1). The ticks tested in these studies were sampled between 1994 and 2018. In more than half of the studies (n = 44), ticks were collected between the years 2011 and 2013. Studies were performed in 23 of 45 different European countries.
Most collection areas were rural, single studies collected ticks in recreational areas in urban settings (72, 73). Furthermore, ticks were sampled from vegetation and animals on European islands (74–77).
In total, 115,265 ticks were tested in all 72 analyzed studies, of which 62,889 were sampled in a single and thus largest study performed in Switzerland (78). Excluding this study, on average, 689 ticks were sampled per study, ranging from 18 to 7,050 samples. The mean prevalence of C. burnetii-DNA over all evaluated studies was 4.8%. In half of the studies (n = 38), no C. burnetii-positive tick was identified. The highest prevalence was determined to be 54.2% in a Spanish study (79). Of the 34 studies with positive results, 10 (29.4%) confirmed the results with the sequencing of at least one PCR amplicon. Sequencing could not be performed in some cases because of low DNA yield (80). Ticks were sampled by flagging or dragging from the vegetation or removed from animals.
Most studies simultaneously gathered further information, e.g., about tick infestation of local animal species or other tick-borne pathogens. In addition, the seroprevalence for C. burnetii or other tick-borne agents of captive domestic and wild animals was examined (79–82).
Country
There were major differences in prevalence between different countries and regions in Europe (Figure 2, Supplementary Figure 1). The majority of studies were performed in Southern Europe (n = 30), whereas the number of prevalence studies per country was highest for Slovakia (n = 12). A high prevalence was found in studies in Southern Europe, especially in Spain (10.1%) and Portugal (10.6%), but also in Poland, a high proportion of ticks was positive (8.5%). In ticks tested in Northern Europe, no C. burnetii was detected (83–86).
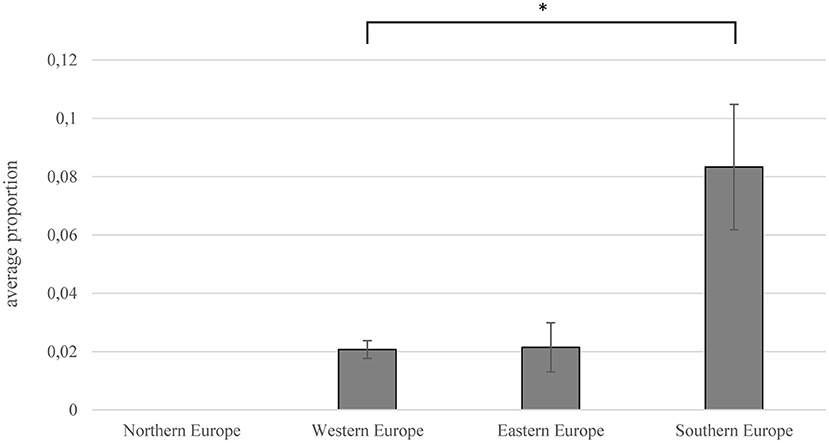
Figure 2. Prevalence (ratio of C. burnetii-positive ticks to total number of tested ticks) depending on European regions. Error bars show average confidence interval; significance was proven using Mann–Whitney U-test (*p = 0.013).
The highest prevalence within single studies was determined in Spain and Italy. González et al. detected Coxiella-DNA in over 50% of the 236 tested ticks (79). In studies from Italy and France, more than 30% of tested ticks were found to be positive for C. burnetii-DNA (87–89). The mean prevalence in Southern Europe (8.3%) was significantly higher than in the rest of Europe (Mann–Whitney U test; p = 0.031), especially in Western Europe (2.1%) (Mann–Whitney U test; p = 0.013).
Tick Species
More than 25 tick species were examined in all studies (Table 1). In Amblyomma spp., Haemaphysalis inermis, Hyalomma scupense, Hyalomma truncatum, Ixodes festai, and Ixodes hexagonus, no molecular detection of C. burnetii-specific DNA was reported. Furthermore, in Haemaphysalis hispanica, Hyalomma aegyptium, Hyalomma lusitanicum, Ixodes ventalloi, Rhipicephalus pusillus, and Rhipicephalus thuranicus, Coxiella-DNA was detected, but the results were not sequenced for confirmation or discriminated from CLE.
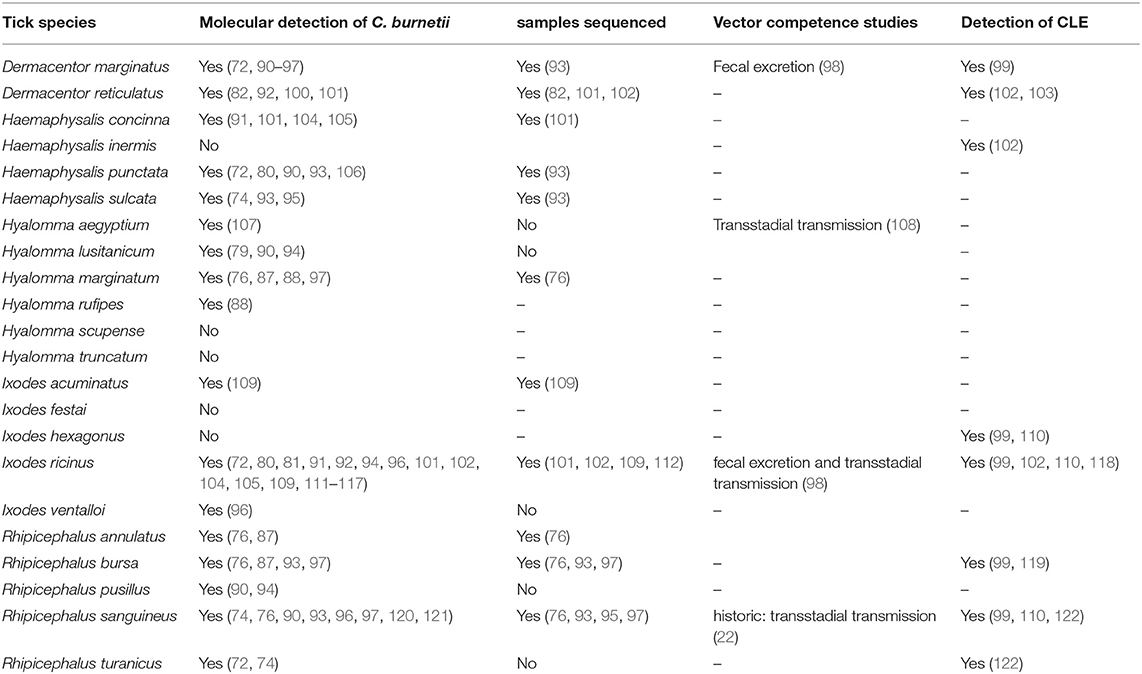
Table 1. Selected tick species collected in Europe and detection of C. burnetii and CLE (Reference).
Differences in detecting C. burnetii-positive ticks were noticed for the tick genera tested (Figure 3). The highest prevalence was observed in Hyalomma spp. ticks (11.3%). Of the six species tested, Hyalomma rufipes, Hyalomma marginatum, H. lusitanicum, and H. aegyptium were positive for C. burnetii. The most abundant and most often infected species was H. lusitanicum, in which 17.7% of ticks were PCR positive for C. burnetii. Furthermore, 6.0% of all tested Rhipicephalus spp. were positive for C. burnetii-DNA, whereas Dermacentor spp. harbored Coxiella-DNA in 1.4% of samples. By far, the most tested ticks belonged to the genus of Ixodes spp. (81.4%). Of these ticks, only 0.4% carried C. burnetii-DNA. The second least Coxiella-infested tick species was Haemaphysalis spp. with 1.2% positive samples. Of Amblyomma spp., only 11 negative ticks were flagged or removed from birds, which is not surprising, as this genus is not endemic in Europe (77, 90).
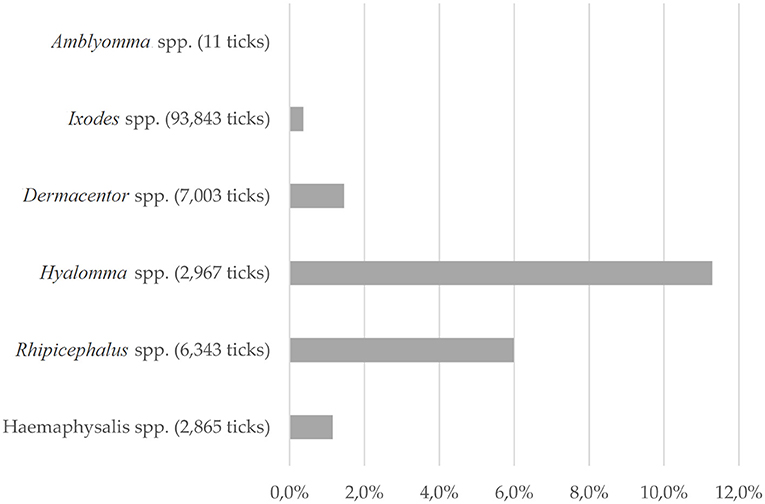
Figure 3. Tick species: Proportion of C. burnetii-positive ticks of the ticks tested, depending on tick genera. For 2,233 ticks, only total numbers were described.
Collection Method
The most common methods for prevalence studies were sampling from animals (n = 29, 40.3%; 11,283 ticks) or from the vegetation by dragging or flagging a piece of cotton (n = 27, 37.5%, 83,476 ticks). Overall, in the evaluated studies, 72.4% of all tested ticks were derived from vegetation. In 19.4% (n = 14; 19,939) of the examined studies, a combination of sampling from animals or humans and flagging was performed. Data in this review were not divided into the different collection methods used. Two studies examined the occurrence of pathogens in ticks exclusively collected from humans (123, 124). The highest mean prevalence for C. burnetii was found in studies in which ticks were removed from infected animals with 6.6%, whereas the mean prevalence in ticks collected from vegetation was 2.8% (Figure 4A). Of all tested ticks removed by flagging, 0.5% were positive for C. burnetii-DNA (Figure 4B). Tested animals ranged from pets and livestock to trapped rodents or game. In some studies, ticks were removed from birds to examine their role as hosts and vehicle for tick species (81, 88, 125).
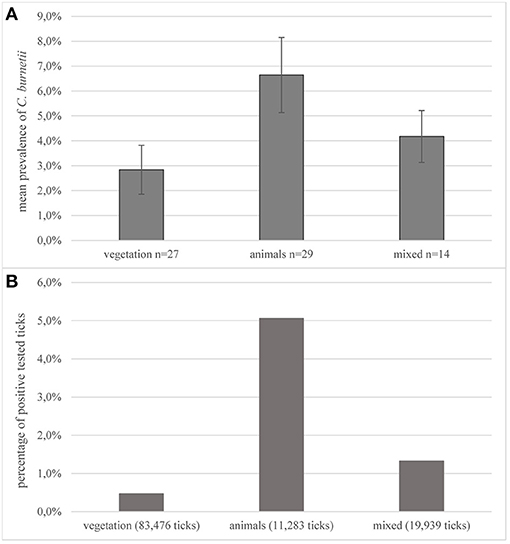
Figure 4. Collection methods. (A) Average prevalence of C. burnetii in studies on ticks depending on the collection method. Error bars show average confidence intervals. n = number of studies; (B) percentage of positively tested ticks of the total number of tested ticks in all studies, depending on the collection methods. Number in parentheses = total number of sampled ticks with this method.
C. burnetii was detected in two H. marginatum, feeding on humans in Sardinia. Contrary, in two studies that tested human-derived ticks exclusively, all were negative for C. burnetii-specific DNA (76, 123, 124).
The only study that was directly associated with a Q fever outbreak was performed in the Netherlands. Almost 3,000 ticks were sampled from nature, domestic animals, and wildlife. No C. burnetii-positive questing tick was found, whereas five female ticks collected from animals were positive for IS1111 and com1 target sequences, suggesting uptake of blood from a bacteremic host (111). Further investigations revealed that these positive ticks were collected from recently vaccinated sheep. This might indicate a low risk of field infection during the outbreak.
Detection Method
Conventional PCR and real-time PCR were applied as a detection method in most of the studies. The predominant target genes for PCR detection were fragments of the insertion sequence IS1111 (n = 37, 51.4%, 33,626 ticks) or the genes com1 (n = 14, 19.4%, 10,725 ticks), icd (n = 12, 16.7%, 17,631 ticks), sodB (n = 5, 6.9%, 3,502 ticks), and htpAB (n = 4, 5.6%, 3,192 ticks). Single studies used more than one target gene for identification (75, 85, 100, 126, 127). Of studies, which used sodB as the target gene for C. burnetii, an average prevalence of 7.3% was reported (Figure 5A). Of the studies using PCR targeting the com1 fragment, the mean prevalence was 0.8%. The most frequently used target IS1111 resulted in an average prevalence of 5.7%. Of all ticks tested with IS1111, 3.0% were positive, which is the highest percentage (Figure 5B). The largest sampling size of 62,889 ticks, exclusively I. ricinus, was collected in a study in Switzerland. No C. burnetii-positive tick was detected by using ompA as the target gene in this study (78). Also, 16S ribosomal RNA (rRNA) sequencing was used for the detection of Coxiella spp. in eight studies; one of them detected C. burnetii-positive ticks (102).
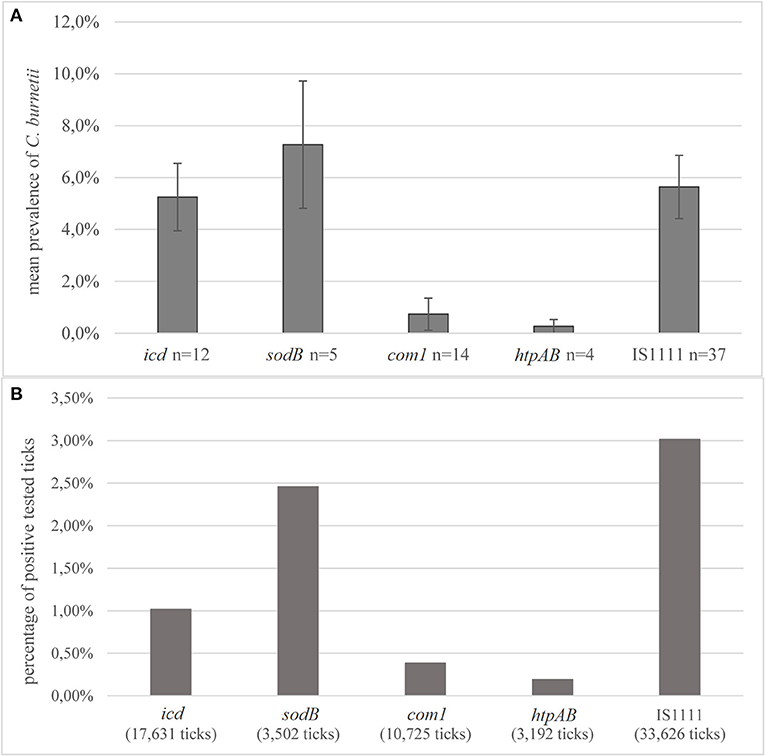
Figure 5. Detection methods (A) average prevalence of C. burnetii in PCR detection depending on the target genes. Error bars show average confidence intervals. n = number of studies; (B) percentage of positively tested ticks of the total number of tested ticks in all studies, depending on the detection method. In two studies, 567 ticks were collected from humans and tested negative. Number in parentheses = total number of ticks tested with this method.
Before PCR detection, some studies used the hemocyte test for visual detection of Coxiella spp. and morphologically similar agents in the hemolymph (91, 104). In these studies, C. burnetii was detected by PCR in four hemocyte-positive ticks, but also in two hemocyte-negative ticks.
Furthermore, studies used non-molecular methods in addition to PCR, such as cultivation in cells or embryonated eggs, resulting in the isolation of Spiroplasma spp. but not C. burnetii (126).
Coxiella-Like Endosymbionts
Some authors considered CLE and searched specifically for these endosymbionts or detected CLE by sequencing positive PCR products. Furthermore, sequencing of 16S rRNA was used to evaluate the tick microbiome (99, 103). In seven studies, CLEs were detected additionally or exclusively using sequencing in a variety of tick species, or Coxiella was only identified on genus level (99, 102, 103, 110, 119, 122). Nine tick species were found to harbor CLE in these studies.
Experimental Vector Competence Studies
In the decades after the first isolation of C. burnetii from a tick, experimental transmission studies were conducted on various tick species. However, only two recent investigations on the vector competence of ticks under laboratory conditions were found in the literature.
In one study, the vector competence of the tick H. aegyptium was proven, including the transstadial transmission over all life stages (108). Larvae were fed on infected guinea pigs, and 5.6% tested positive after molting into nymphs. After feeding on an infected host, 28.9% of nymphs molted into adults remained positive. In addition, reinfection of uninfected guinea pigs was shown in this study.
For I. ricinus, a transstadial transmission from nymphs to adults was shown using an in vitro feeding system, enabling better differentiation between the transmission via saliva or feces (98). The transstadial transmission rate from nymphs to adults was determined to be 25%, but horizontal transfer into the blood was not shown, whereas the ticks excreted infectious feces during feeding and after molting.
Discussion
Higher Prevalence in the Mediterranean Region Might Be Associated With Regional Distribution of Different Tick Species
Duron et al. found an average prevalence of 5% of C. burnetii in ticks after evaluation of 60 studies conducted worldwide (28). The data of the present study show a similar mean prevalence of studies on ticks in Europe. Here, an update is provided, enhancing the insight into the prevalence of C. burnetii in various tick species, demonstrating that there is a huge variation between the results of different studies depending on the country, tested species, method of collection, and C. burnetii detection method applied.
Evaluating the studies, a gradient from North to South was noticed, resulting in a higher prevalence in the Mediterranean region, followed by Eastern Europe. Most positive samples were obtained in Southern Europe, especially Portugal, Spain, and Greece. Here, species of the genera Hyalomma, Rhipicephalus, and Haemaphysalis are the most abundant vector ticks. Due to climate change associated with global warming, the spread of arthropods is expected (128), which could possibly lead to an increased role of pathogen transmission by these tick species in Western and Northern Europe. The tick species H. marginatum was detected in the last years more often in Central Europe and hibernation of this species was recently observed in Germany (64). Since in most of the studies, no confirmation of positive results by specific methods for C. burnetii was performed, an inadvertent detection of CLE cannot be excluded. However, this differentiation is extremely important because CLEs seem to have less if at all pathogenic potential than C. burnetii.
Coxiella burnetii is rarely found in ticks in most of the regions in Europe. It is presumed that ticks play a minor role in Q fever transmission, also considering that no validated human cases of C. burnetii infection via ticks have been reported. Foci in which ticks may play a role as the natural reservoir for Q fever seem to exist, but these hot spots are hard to detect. Similar patterns of regional foci were described for tick-borne encephalitis virus (129, 130). The absence of C. burnetii-infected ticks in endemic areas is often explained by narrow hot spots (131). That causes less frequent surveillance of this pathogen in major prevalence studies, mainly focusing on well-described tick-borne infectious agents such as Anaplasma phagocytophilum or B. burgdorferi s.l. Under these circumstances, there is a lack of data in certain countries and regions. The evaluation of European studies shows that occurrence of C. burnetii is highly dependent on the tick species. The most abundant tick species in Europe, I. ricinus, seems to be infected with C. burnetii very rarely. Occasional reports of successful isolation of C. burnetii from field-collected I. ricinus support the theory of less relevance in Q fever transmission (132). In the past, a correlation was presumed between the occurrence of Q fever and the abundance of Dermacentor marginatus (133). Considering the low prevalence in this species, this theory was not proven by the data collected here from the analyzed studies. However, there seems to be an association of C. burnetii with ticks of the genus Hyalomma spp. and Rhipicephalus spp., naturally present in regions with ambient temperatures, e.g., the Mediterranean region. This is also supported by the in vivo experimental study performed with H. aegyptium (108). As Rhipicephalus spp. is highly infected with CLE, positive results should be confirmed by sequencing (41, 119).
Prevalence studies targeting C. burnetii in ticks are conducted worldwide, and positive ticks were found on all continents except Antarctica (134–138). Comparably with European studies, there are divergent findings in the different investigations. Recently, a study conducted in China using 16S rRNA sequencing reported a prevalence of 40–96% depending on tested species (139). High prevalence was also found in several countries, e.g., Argentina, Egypt, or Nigeria (140–142). Apart from that, in studies in Japan or Reunion Island, no Coxiella-positive ticks were determined (143, 144). Despite the origin of the first isolate, C. burnetii is reported rarely from ticks in North America (145, 146).
A Combination of Different Collection Methods Supports a Realistic Depiction of Reality
Different collection methods were used in the studies, but the most common is removing from animals or flagging. Simple detection of the DNA of a pathogen does not prove the vector competence of a certain tick species (92, 147). Ticks can also carry a pathogen without the ability to transmit it (93). These arthropods feed on bacteraemic/viraemic/protozooaemic animals and are likely to take up any agent circulating in the blood of the host. The slightly higher prevalence of C. burnetii in ticks collected from animals compared with ticks from vegetation argues in favor of such an uptake. The procedure of flagging of questing ticks, therefore, seems to be more appropriate for information regarding the prevalence and to discriminate between the role of ticks as a reservoir or as an accidental host (148). Additionally, data about the local seroprevalence of C. burnetii in animal host species could be included. A combination of both origins, vegetation, and animals, might also be of interest, especially in the context of outbreak surveillance. Moreover, only exophilic and questing ticks can be obtained using the dragging method. That excludes, for example, premature life stages of Dermacentor spp. or preferably hunting ticks, e.g., Hyalomma spp. As it is known that C. burnetii genomes found in ticks genetically cluster with samples isolated from wildlife, the inclusion of hunted animals can increase the knowledge of potentially sylvatic cycles (149). The sampling of ticks from migratory birds can give insight into the movement of ticks and the pathogens or microbial communities they carry. Examination of avian ticks and tick-borne diseases can also help monitor the spread of these ticks and their pathogenic cargo (150). The introduced tick species might not be adapted to the climate and availability of host species in this country; therefore, its survival is unclear. Sampling on farm animals and game favors a higher rate of adult ticks, as this life stage is more frequently feeding on larger animals (112). Examination of ticks removed from human patients also might be of interest regarding the zoonotic potential of the disease. In a study by Dubourg et al., patients showing scalp eschar were examined, and removed ticks were tested for a wide range of tick-borne pathogens. Of the 11 ticks, mainly D. marginatus, two carried C. burnetii, whereas Rickettsia slovaca was the most prevalent pathogen (151). Simultaneous infection of human patients with C. burnetii and other tick-borne pathogens were described, but it cannot be excluded that the temporal connection between the infections is random, as Q fever is endemic worldwide, and infection might have been caused by inhalation (30). To determine the significance of ticks in Q fever transmission, it might be of major interest to perform prevalence studies in areas of active Q fever outbreaks or in known Coxiella-positive herds. This is important to prove any association between infected animals or humans and the local arthropods. Furthermore, identification of tick life stage is important to analyze the possibility of transovarial transmission. Coxiella burnetii is considered one of the most relevant pathogens and should be prioritized in the examination of wildlife (152).
Prevalence Studies Should Include Specific C. burnetii Detection Methods
There was no significant difference noticed between the various target genes used in the evaluated studies. The determined prevalence depends, among other things, on the sensitivity of the used target gene. Some assays, especially when using IS1111, detect lower amounts of DNA and thus are more sensitive than others. This relation can be described more precisely by the use of standardized controls such as plasmids. Because of the close genetic relationship to CLE, the high specificity of molecular methods is required. Because frequently used PCR target gene sequences, e.g., IS1111, were also detected in endosymbionts, there is a need for specific methods to distinguish between these species. This would rule out an overestimation of the dissemination of C. burnetii within the tick population. The majority of studies detecting commonly used target genes for the detection of C. burnetii did not confirm the results with sequencing. Particularly, the IS1111 fragment is a common target for C. burnetii detection in ticks, which is known to be less specific but highly sensitive for the pathogen (57). The specificity for detection of C. burnetii in tick samples is limited in all commonly used target genes; thus, the use of single targets is not recommended (56, 58). Positive results in PCR should be interpreted carefully, and sequence confirmation should be mandatory, preferably using long targets for increased specificity (56, 153). Due to low DNA yield, sequencing might not be possible. In these cases, the combination of different target genes for detection could be a valid method to minimize the risk of unspecific results. Thus, there is a need to develop specific assays for differentiation between C. burnetii and CLE, which might also be applicable for higher sample sizes and poor DNA yield.
Unclear Pathogenic Potential of Coxiella-Like Endosymbionts Should Not Be Neglected
In seven studies, CLEs were detected in tick samples, mainly using sequencing of 16s rRNA. These endosymbionts are distributed in several hard and soft tick species and represent a large proportion of the microbiome of some species (33, 154). This leads to the presumption that the specificity of some molecular methods may not be sufficient to distinguish between C. burnetii and endosymbionts. Several Amblyomma spp. and Rhipicephalus spp. were shown to be CLE carriers in up to 100% of analyzed tick samples (41). In another study with more than 50 different tick species of hard and soft ticks, more than two-thirds of the species were found to harbor CLE (38). Coxiella-like endosymbionts seem to be associated with some genera, for example, Rhipicephalus spp. or Ornithodoros spp., in which CLEs were detected with a high prevalence, whereas only a few positive samples originated from Ixodes spp. Binetruy et al. found CLE to be present in 11 of 24 species of the genus Amblyomma (155). Recent phylogenetic analysis revealed a close and apparently ancient alliance between Rhipicephalus spp. and their CLE (156). The main survival strategy of CLE is the vertical transmission via the egg, but also horizontal transfer, possibly via co-feeding, was proven (156, 157). Recent results based on genome sequencing have shown that certain CLEs seem to have evolved from an ancestor capable of infection of immune cells (158). Hence, a loss of pathogenic potential was suggested. In contrast, C. burnetii was reported to have its phylogenetic origin in CLE (38).
Novel tick-borne pathogens are emerging, and as the evolution of C. burnetii is closely linked to endosymbionts, the possibility of other Coxiella spp. being pathogenic should not be neglected, considering that bird infections or human skin infections were reported (38, 49, 103, 158). Increasing the knowledge on evolutionary processes and the pathogenic potential of CLE could likewise also contribute to a better understanding of the epidemiology of C. burnetii.
Little is known about the impact CLE might have on pathogen transmission. A reduced infection rate of R. haemaphysaloides with CLE correlated with a lower rate of transstadial transmission of Babesia microti. Candidatus Midichloria mitochondrii, another tick endosymbiont, is known to influence the occurrence and ability to detect tick-borne pathogens in I. ricinus (159, 160).
Experimental Studies Are Needed to Assess the Vector Competence of Further Tick Species
In the past, seven tick species were shown to be competent vectors for C. burnetii (28). There is little recent research exploring the vector competence of ticks under laboratory conditions. Experimental studies prove transstadial transmission and successful reinfection of guinea pigs by H. aegyptium ticks (108) and transstadial transmission from nymphs to adults in I. ricinus (98). Recently, a transstadial transmission from nymphs to adults and a subsequent excretion with saliva were concluded in naturally infected H. lusitanicum (161). There is a lack of vector competence studies on different tick species, focusing on the transstadial and transovarial transmission of pathogens. However, those studies are limited by the low rate of transmission and consequently incomplete knowledge of epidemiological cycles (162). Furthermore, studies are missing, which describe the level and duration of bacteremia in C. burnetii-infected hosts, which are necessary to estimate the actual vector capacity under laboratory conditions. As a mainly airborne pathogen, the potential infection routes may be via inhalation of feces besides the injection of saliva during the tick bite (98, 108). Infected feces might also contaminate the wound and thus causing an infection, as it is known from the transmission of Trypanosoma cruzi by bed bugs or Rickettsia prowazekii and lice (163, 164).
Besides the need for information regarding the prevalence of C. burnetii in ticks, the risk of acquiring Q fever by a tick bite cannot finally be determined. In different studies, the correlation between coxiellosis and the abundance of ticks as a risk factor was examined. Predominantly, no significant correlation was found (165–167), whereas other investigations concluded an association between tick infestation and seroprevalence (168, 169).
Most tick species in Europe are spreading, and the increased risk of tick-borne diseases accompanies this. For this reason, extensive and focused monitoring of ticks and their microbial burden is crucial, as well as further research on possibly tick-borne diseases and the pathogenic potential of already known bacteria.
Conclusions
The evaluation of European studies shows a significantly higher prevalence of Coxiella spp. in ticks in Mediterranean countries. This is likely to be driven by the abundance of different tick species in these countries, i.e., Hyalomma spp. and Rhipicephalus spp. In the context of global warming, the geographical distribution of these tick species changes and thus the epidemiology of Q fever in currently temperate Europe. Based on the large number of studies, which failed to detect C. burnetii DNA in tick samples, ticks carrying C. burnetii seem to be restricted to certain regions. Positive results have to be interpreted carefully because no distinction between C. burnetii and CLE was made in most of these studies. Planning of a prevalence study on ticks should, therefore, in particular, focus on the choice of detection methods for specific results. Methods should aim to differentiate C. burnetii and CLE using sequencing or at least to use different target genes in positive samples.
To address the questions on the role ticks play as a reservoir in Q fever transmission, vector competence studies using different and relevant tick species should be performed.
Data Availability Statement
The original contributions presented in the study are included in the article/Supplementary Material, further inquiries can be directed to the corresponding author/s.
Author Contributions
SK and KM-S designed the study, SK conducted the literature search, SK, GM, SU, MP, and KM-S performed the data extraction, and SK analyzed the data and drafted the manuscript. All authors contributed to the article and approved the submitted version.
Funding
This study was supported by the German Federal Ministry for Education and Research (BMBF) via the Research Network of Zoonotic Infectious Diseases, project Q-GAPS (Q fever—German Interdisciplinary Program for Research), grant numbers 01KI1726G and 01KI1726C, and project TBENAGER (Tick-Borne Encephalitis in Germany), grant number 01KI1728D.
Conflict of Interest
The authors declare that the research was conducted in the absence of any commercial or financial relationships that could be construed as a potential conflict of interest.
Supplementary Material
The Supplementary Material for this article can be found online at: https://www.frontiersin.org/articles/10.3389/fvets.2021.655715/full#supplementary-material
Supplementary Figure 1. Forest plots of the included prevalence studies.
Supplementary Table 1. Overview on included prevalence studies and the collected data.
References
1. van Roeden SE, Wever PC, Kampschreur LM, Gruteke P, van der Hoek W, Hoepelman AIM, et al. Chronic Q fever-related complications and mortality: data from a nationwide cohort. Clin Microbiol Infect. (2019) 25:1390–8. doi: 10.1016/j.cmi.2018.11.023
2. van Asseldonk MAPM, Prins J, Bergevoet RHM. Economic assessment of Q fever in the Netherlands. Prev Vet Med. (2013) 112:27–34. doi: 10.1016/j.prevetmed.2013.06.002
3. Baca OG, Li YP, Kumar H. Survival of the Q fever agent Coxiella burnetii in the phagolysosome. Trends Microbiol. (1994) 2:476–80. doi: 10.1016/0966-842x(94)90651-3
4. Melenotte C, Lepidi H, Nappez C, Bechah Y, Audoly G, Terras J, et al. Mouse model of Coxiella burnetii aerosolization. Infect Immun. (2016) 84:2116–23. doi: 10.1128/iai.00108-16
5. Arricau Bouvery N, Souriau A, Lechopier P, Rodolakis A. Experimental Coxiella burnetii infection in pregnant goats: excretion routes. Vet Res. (2003) 34:423–33. doi: 10.1051/vetres:2003017
6. Roest HI, van Solt CB, Tilburg JJ, Klaassen CH, Hovius EK, Roest FT, et al. Search for possible additional reservoirs for human Q fever, The Netherlands. Emerg Infect Dis. (2013) 19:834–5. doi: 10.3201/eid1905.121489
7. Roest H-J, van Gelderen B, Dinkla A, Frangoulidis D, van Zijderveld F, Rebel J, et al. Q fever in pregnant goats: pathogenesis and excretion of Coxiella burnetii. PLoS ONE. (2012) 7:e48949. doi: 10.1371/journal.pone.0048949
8. Guatteo R, Joly A, Beaudeau F. Shedding and serological patterns of dairy cows following abortions associated with Coxiella burnetii DNA detection. Vet Microbiol. (2012) 155:430–3. doi: 10.1016/j.vetmic.2011.09.026
9. Groten T, Kuenzer K, Moog U, Hermann B, Maier K, Boden K. Who is at risk of occupational Q fever: new insights from a multi-profession cross-sectional study. BMJ Open. (2020) 10:e030088. doi: 10.1136/bmjopen-2019-030088
10. Schimmer B, Schotten N, van Engelen E, Hautvast JL, Schneeberger PM, van Duijnhoven YT. Coxiella burnetii seroprevalence and risk for humans on dairy cattle farms, the Netherlands, 2010–2011. Emerg Infect Dis. (2014) 20:417–25. doi: 10.3201/eid2003.131111
11. Evstigneeva AS, Ul'yanova TY, Tarasevich IV. The survival of Coxiella burnetii in soils. Euras Soil Sci. (2007) 40:565–8. doi: 10.1134/S1064229307050122
12. Cutler SJ, Bouzid M, Cutler RR. Q fever. J Infect. (2007) 54:313–8. doi: 10.1016/j.jinf.2006.10.048
13. To H, Htwe KK, Kako N, Kim HJ, Yamaguchi T, Fukushi H, et al. Prevalence of Coxiella burnetii infection in dairy cattle with reproductive disorders. J Vet Med Sci. (1998) 60:859–61. doi: 10.1292/jvms.60.859
14. Macías-Rioseco M, Riet-Correa F, Miller MM, Sondgeroth K, Fraga M, Silveira C, et al. Bovine abortion caused by Coxiella burnetii: report of a cluster of cases in Uruguay and review of the literature. J Vet Diagn Invest. (2019) 31:634–9. doi: 10.1177/1040638719856394
15. van der Hoek W, Morroy G, Renders NH, Wever PC, Hermans MH, Leenders AC, et al. Epidemic Q fever in humans in the Netherlands. Adv Exp Med Biol. (2012) 984:329–64. doi: 10.1007/978-94-007-4315-1_17
16. Schneeberger PM, Wintenberger C, van der Hoek W, Stahl JP. Q fever in the Netherlands – 2007–2010: what we learned from the largest outbreak ever. Med Mal Infect. (2014) 44:339–53. doi: 10.1016/j.medmal.2014.02.006
18. Kampschreur LM, Delsing CE, Groenwold RH, Wegdam-Blans MC, Bleeker-Rovers CP, de Jager-Leclercq MG, et al. Chronic Q fever in the Netherlands 5 years after the start of the Q fever epidemic: results from the Dutch chronic Q fever database. J Clin Microbiol. (2014) 52:1637–43. doi: 10.1128/jcm.03221-13
19. van Roeden SE, Bleeker-Rovers CP, de Regt MJA, Kampschreur LM, Hoepelman AIM, Wever PC, et al. Treatment of chronic Q Fever: clinical efficacy and toxicity of antibiotic regimens. Clin Infect Dis. (2018) 66:719–26. doi: 10.1093/cid/cix886
20. Kampschreur LM, Wegdam-Blans MC, Thijsen SF, Groot CA, Schneeberger PM, Hollander AA, et al. Acute Q fever related in-hospital mortality in the Netherlands. Neth J Med. (2010) 68:408–13.
21. Davis GE, Cox HR, Parker RR, Dyer RE. A filter-passing infectious agent isolated from ticks. Public Health Reports (1896–1970) (1938) 53:2259–82. doi: 10.2307/4582746
22. Smith DJW. Studies in the epidemiology of Q fever. 8. The transmission of Q fever by the tick Rhipicephalus sanguineus. Aust J Exp Biol Med. (1941) 19:133–6. doi: 10.1038/icb.1941.21
23. Smith DJW. Studies in the epidemiology of Q fever. 11. Experimental infection of the ticks Haemaphysalis bispinosa and Ornithodoros sp. with Rickettsia burneti. Aust J Exp Biol Med Sci. (1942) 20:295–6. doi: 10.1038/icb.1942.47
24. Philip CB. Observations on experimental Q fever. J Parasitol. (1948) 34:457–64. doi: 10.2307/3273312
25. Smith DJW. Studies in the epidemiology of Q fever. 3. Transmission of Q fever by the tick Haemaphysalis humerosa. Aust J Exp Biol Med Sci. (1940) 18:103–18. doi: 10.1038/icb.1940.11
26. Eldin C, Mélenotte C, Mediannikov O, Ghigo E, Million M, Edouard S, et al. From Q Fever to Coxiella burnetii Infection: a Paradigm Change. Clin Microbiol Rev. (2017) 30:115–90. doi: 10.1128/cmr.00045-16
27. Rehacek J, Brezina R. Detection of Coxiella burnetii in saliva of experimentally infected ticks, Hyalomma dromedarii Koch. Bull World Health Organ. (1968) 39:974–7.
28. Duron O, Sidi-Boumedine K, Rousset E, Moutailler S, Jourdain E. The importance of ticks in Q Fever transmission: what has (and has not) been demonstrated? Trends Parasitol. (2015) 31:536–52. doi: 10.1016/j.pt.2015.06.014
29. Nett R, Book E, Anderson A. Q Fever with unusual exposure history: a classic presentation of a commonly misdiagnosed disease. Case Rep. Infect. Dis. (2012) 2012:916142. doi: 10.1155/2012/916142
30. Rolain JM, Gouriet F, Brouqui P, Larrey D, Janbon F, Vene S, et al. Concomitant or consecutive infection with Coxiella burnetii and tickborne diseases. Clin Infect Dis. (2005) 40:82–8. doi: 10.1086/426440
31. Duron O, Hurst GD. Arthropods and inherited bacteria: from counting the symbionts to understanding how symbionts count. BMC Biol. (2013) 11:45. doi: 10.1186/1741-7007-11-45
32. Noda H, Munderloh UG, Kurtti TJ. Endosymbionts of ticks and their relationship to Wolbachia spp. and tick-borne pathogens of humans and animals. Appl Environ Microbiol. (1997) 63:3926–32. doi: 10.1128/aem.63.10.3926-3932.1997
33. Zhong J. Coxiella-like endosymbionts. Adv Exp Med Biol. (2012) 984:365–79. doi: 10.1007/978-94-007-4315-1_18
34. Seo MG, Lee SH, VanBik D, Ouh IO, Yun SH, Choi E, et al. Detection and genotyping of Coxiella burnetii and Coxiella-like bacteria in horses in South Korea. PLoS ONE. (2016) 11:e0156710. doi: 10.1371/journal.pone.0156710
35. Gottlieb Y, Lalzar I, Klasson L. Distinctive genome reduction rates revealed by genomic analyses of two Coxiella-like endosymbionts in ticks. Genome Biol Evol. (2015) 7:1779–96. doi: 10.1093/gbe/evv108
36. Ramaiah A, Dasch GA. Genome sequence of Coxiella-like endosymbiont strain CLE-RmD, a bacterial agent in the cattle tick (Rhipicephalus microplus) Deutsch strain. Genome Announc. (2018) 6:e00003–18. doi: 10.1128/genomeA.00003-18
37. Tsementzi D, Castro Gordillo J, Mahagna M, Gottlieb Y, Konstantinidis KT. Comparison of closely related, uncultivated Coxiella tick endosymbiont population genomes reveals clues about the mechanisms of symbiosis. Environ Microbiol. (2018) 20:1751–64. doi: 10.1111/1462-2920.14104
38. Duron O, Noel V, McCoy KD, Bonazzi M, Sidi-Boumedine K, Morel O, et al. The recent evolution of a maternally-inherited endosymbiont of ticks led to the emergence of the Q fever pathogen, Coxiella burnetii. PLoS Pathog. (2015) 11:e1004892. doi: 10.1371/journal.ppat.1004892
39. Ben-Yosef M, Rot A, Mahagna M, Kapri E, Behar A, Gottlieb Y. Coxiella-like endosymbiont of Rhipicephalus sanguineus is required for physiological processes during ontogeny. Front Microbiol. (2020) 11:493. doi: 10.3389/fmicb.2020.00493
40. Smith TA, Driscoll T, Gillespie JJ, Raghavan R. A Coxiella-like endosymbiont is a potential vitamin source for the Lone Star tick. Genome Biol Evol. (2015) 7:831–8. doi: 10.1093/gbe/evv016
41. Machado-Ferreira E, Vizzoni VF, Balsemão-Pires E, Moerbeck L, Gazeta GS, Piesman J, et al. Coxiella symbionts are widespread into hard ticks. Parasitol Res. (2016) 115:4691–9. doi: 10.1007/s00436-016-5230-z
42. Duron O, Morel O, Noël V, Buysse M, Binetruy F, Lancelot R, et al. Tick-bacteria mutualism depends on B vitamin synthesis pathways. Curr Biol. (2018) 28:1896–902.e5. doi: 10.1016/j.cub.2018.04.038
43. Zhang CM, Li NX, Zhang TT, Qiu ZX, Li Y, Li LW, et al. Endosymbiont CLS-HI plays a role in reproduction and development of Haemaphysalis longicornis. Exp Appl Acarol. (2017) 73:429–38. doi: 10.1007/s10493-017-0194-y
44. Li LH, Zhang Y, Zhu D. Effects of antibiotic treatment on the fecundity of Rhipicephalus haemaphysaloides ticks. Parasit Vec. (2018) 11:242. doi: 10.1186/s13071-018-2807-7
45. Guizzo MG, Parizi LF, Nunes RD, Schama R, Albano RM, Tirloni L, et al. A Coxiella mutualist symbiont is essential to the development of Rhipicephalus microplus. Sci Rep. (2017) 7:17554. doi: 10.1038/s41598-017-17309-x
46. Vapniarsky N, Barr BC, Murphy B. Systemic Coxiella-like infection with myocarditis and hepatitis in an eclectus parrot (Eclectus roratus). Vet Pathol. (2012) 49:717–22. doi: 10.1177/0300985811409251
47. Woc-Colburn AM, Garner MM, Bradway D, West G, D'Agostino J, Trupkiewicz J, et al. Fatal coxiellosis in Swainson's Blue Mountain Rainbow Lorikeets (Trichoglossus haematodus moluccanus). Vet Pathol. (2008) 45:247–54. doi: 10.1354/vp.45-2-247
48. Flanders AJ, Rosenberg JF, Bercier M, Leissinger MK, Black LJ, Giglio RF, et al. Antemortem diagnosis of coxiellosis in a Blue and Gold Macaw (Ara ararauna). J Avian Med Surg. (2017) 31:364–72. doi: 10.1647/2016-224
49. Angelakis E, Mediannikov O, Jos SL, Berenger JM, Parola P, Raoult D. Candidatus Coxiella massiliensis infection. Emerg Infect Dis. (2016) 22:285–8. doi: 10.3201/eid2202.150106
50. Rehácek J, Brezina R, Kovácová E, Zupancicová M. Haemocyte test-an easy, quick and reliable method for the detection of rickettsiae in ticks. Acta Virol. (1971) 15:237–40.
51. Rehácek J, Zupancicová M, Ac P, Tarasevic IV, Jablonskaja VA, Pospísil R, et al. Ricettsioses studies. 2. Natural foci of rickettsioses in east Slovakia. Bull World Health Organ. (1976) 53:31–8.
52. Rehacek J, Kovacova E, Lisak V, Rumin W. Occurrence of Coxiella burnetii, Rickettsia slovaca, and organisms resembling bacillary rickettsiae in their natural foci in Slovakia 20 years after their first detection. Folia Parasitol (Praha) (1990) 37:285–6.
53. Klee SR, Tyczka J, Ellerbrok H, Franz T, Linke S, Baljer G, et al. Highly sensitive real-time PCR for specific detection and quantification of Coxiella burnetii. BMC Microbiol. (2006) 6:2. doi: 10.1186/1471-2180-6-2
54. Brennan RE, Samuel JE. Evaluation of Coxiella burnetii antibiotic susceptibilities by real-time PCR assay. J Clin Microbiol. (2003) 41:1869–74. doi: 10.1128/jcm.41.5.1869-1874.2003
55. Stein A, Raoult D. Detection of Coxiella burnetti by DNA amplification using polymerase chain reaction. J Clin Microbiol. (1992) 30:2462–6. doi: 10.1128/jcm.30.9.2462-2466.1992
56. Jourdain E, Duron O, Severine B, Gonzalez-Acuna D, Sidi-Boumedine K. Molecular methods routinely used to detect Coxiella burnetii in ticks cross-react with Coxiella-like bacteria. Infect Ecol Epidemiol. (2015) 5:29230. doi: 10.3402/iee.v5.29230
57. Duron O. The IS1111 insertion sequence used for detection of Coxiella burnetii is widespread in Coxiella-like endosymbionts of ticks. FEMS Microbiol Lett. (2015) 362:fnv132. doi: 10.1093/femsle/fnv132
58. Reeves WK, Loftis AD, Sanders F, Spinks MD, Wills W, Denison AM, et al. Borrelia, Coxiella, and Rickettsia in Carios capensis (Acari: Argasidae) from a brown pelican (Pelecanus occidentalis) rookery in South Carolina, USA. Exp Appl Acarol. (2006) 39:321–9. doi: 10.1007/s10493-006-9012-7
59. Duron O, Jourdain E, McCoy KD. Diversity and global distribution of the Coxiella intracellular bacterium in seabird ticks. Ticks Tick Borne Dis. (2014) 5:557–63. doi: 10.1016/j.ttbdis.2014.04.003
60. Estrada-Peña A, Mihalca AD, Petney TN. Ticks of Europe and North Africa: A Guide to Species Identification. Basel: Springer International Publishing (2017). p. 404.
61. Duron O, Binetruy F, Noël V, Cremaschi J, McCoy KD, Arnathau C, et al. Evolutionary changes in symbiont community structure in ticks. Mol Ecol. (2017) 26:2905–21. doi: 10.1111/mec.14094
62. Rizzoli A, Silaghi C, Obiegala A, Rudolf I, Hubálek Z, Földvári G, et al. Ixodes ricinus and its transmitted pathogens in urban and peri-urban areas in Europe: new hazards and relevance for public health. Front Public Health. (2014) 2:251. doi: 10.3389/fpubh.2014.00251
63. Rubel F, Brugger K, Pfeffer M, Chitimia-Dobler L, Didyk YM, Leverenz S, et al. Geographical distribution of Dermacentor marginatus and Dermacentor reticulatus in Europe. Ticks Tick Borne Dis. (2016) 7:224–33. doi: 10.1016/j.ttbdis.2015.10.015
64. Chitimia-Dobler L, Schaper S, Rieß R, Bitterwolf K, Frangoulidis D, Bestehorn M, et al. Imported Hyalomma ticks in Germany in 2018. Parasit Vec. (2019) 12:134. doi: 10.1186/s13071-019-3380-4
65. Duscher GG, HodŽić A, Hufnagl P, Wille-Piazzai W, Schötta AM, Markowicz MA, et al. Adult Hyalomma marginatum tick positive for Rickettsia aeschlimannii in Austria, October 2018. Euro Surveill. (2018) 23:1800595. doi: 10.2807/1560-7917.Es.2018.23.48.1800595
66. Dantas-Torres F, Lia RP, Capelli G, Otranto D. Efficiency of flagging and dragging for tick collection. Exp Appl Acarol. (2013) 61:119–27. doi: 10.1007/s10493-013-9671-0
67. Tack W, Madder M, De Frenne P, Vanhellemont M, Gruwez R, Verheyen K. The effects of sampling method and vegetation type on the estimated abundance of Ixodes ricinus ticks in forests. Exp Appl Acarol. (2011) 54:285–92. doi: 10.1007/s10493-011-9444-6
68. Schulz M, Mahling M, Pfister K. Abundance and seasonal activity of questing Ixodes ricinus ticks in their natural habitats in southern Germany in 2011. J Vector Ecol. (2014) 39:56–65. doi: 10.1111/j.1948-7134.2014.12070.x
69. Randolph SE, Green RM, Hoodless AN, Peacey MF. An empirical quantitative framework for the seasonal population dynamics of the tick Ixodes ricinus. Int J Parasitol. (2002) 32:979–89. doi: 10.1016/s0020-7519(02)00030-9
70. Sherifi K, Rexhepi A, Berxholi K, Mehmedi B, Gecaj RM, Hoxha Z, et al. Crimean-Congo hemorrhagic fever virus and Borrelia burgdorferi sensu lato in ticks from Kosovo and Albania. Front Vet Sci. (2018) 5:38. doi: 10.3389/fvets.2018.00038
71. United Nations Statistics Division. (1999). Available online at: https://unstats.un.org/unsd/methodology/m49/ (accessed November 26, 2020).
72. Mancini F, Di Luca M, Toma L, Vescio F, Bianchi R, Khoury C, et al. Prevalence of tick-borne pathogens in an urban park in Rome, Italy. Ann Agric Environ Med. (2014) 21:723–7. doi: 10.5604/12321966.1129922
73. Minichová L, Hamšíková Z, Mahríková L, Slovák M, Kocianová E, Kazimírová M, et al. Molecular evidence of Rickettsia spp. in ixodid ticks and rodents in suburban, natural and rural habitats in Slovakia. Parasit Vec. (2017) 10:158. doi: 10.1186/s13071-017-2094-8
74. Satta G, Chisu V, Cabras P, Fois F, Masala G. Pathogens and symbionts in ticks: a survey on tick species distribution and presence of tick-transmitted micro-organisms in Sardinia, Italy. J Med Microbiol. (2011) 60(Pt 1):63–8. doi: 10.1099/jmm.0.021543-0
75. Grech-Angelini S, Stachurski F, Vayssier-Taussat M, Devillers E, Casabianca F, Lancelot R, et al. Tick-borne pathogens in ticks (Acari: Ixodidae) collected from various domestic and wild hosts in Corsica (France), a Mediterranean island environment. Transbound Emerg Dis. (2020) 67:745–57. doi: 10.1111/tbed.13393
76. Chisu V, Foxi C, Mannu R, Satta G, Masala G. A five-year survey of tick species and identification of tick-borne bacteria in Sardinia, Italy. Ticks Tick Borne Dis. (2018) 9:678–81. doi: 10.1016/j.ttbdis.2018.02.008
77. Pascucci I, Di Domenico M, Capobianco Dondona G, Di Gennaro A, Polci A, Capobianco Dondona A, et al. Assessing the role of migratory birds in the introduction of ticks and tick-borne pathogens from African countries: an Italian experience. Ticks Tick Borne Dis. (2019) 10:101272. doi: 10.1016/j.ttbdis.2019.101272
78. Pilloux L, Baumgartner A, Jaton K, Lienhard R, Ackermann-Gaumann R, Beuret C, et al. Prevalence of Anaplasma phagocytophilum and Coxiella burnetii in Ixodes ricinus ticks in Switzerland: an underestimated epidemiologic risk. New Microbes New Infect. (2019) 27:22–6. doi: 10.1016/j.nmni.2018.08.017
79. González J, González MG, Valcárcel F, Sánchez M, Martín-Hernández R, Tercero JM, et al. Prevalence of Coxiella burnetii (Legionellales: Coxiellaceae) infection among wildlife species and the tick Hyalomma lusitanicum (Acari: Ixodidae) in a meso-Mediterranean ecosystem. J Med Entomol. (2020) 57:551–6. doi: 10.1093/jme/tjz169
80. Knap N, Zele D, Glinsek Biskup U, Avsic-Zupanc T, Vengust G. The prevalence of Coxiella burnetii in ticks and animals in Slovenia. BMC Vet Res. (2019) 15:368. doi: 10.1186/s12917-019-2130-3
81. Berthová L, Slobodník V, Slobodník R, Olekšák M, Sekeyová Z, Svitálková Z, et al. The natural infection of birds and ticks feeding on birds with Rickettsia spp. and Coxiella burnetii in Slovakia. Exp Appl Acarol. (2016) 68:299–314. doi: 10.1007/s10493-015-9975-3
82. Tokarevich NK, Panferova YA, Freylikhman OA, Blinova OV, Medvedev SG, Mironov SV, et al. Coxiella burnetii in ticks and wild birds. Ticks Tick Borne Dis. (2019) 10:377–85. doi: 10.1016/j.ttbdis.2018.11.020
83. Wallmenius K, Pettersson JH, Jaenson TG, Nilsson K. Prevalence of Rickettsia spp., Anaplasma phagocytophilum, and Coxiella burnetii in adult Ixodes ricinus ticks from 29 study areas in central and southern Sweden. Ticks Tick Borne Dis. (2012) 3:100–6. doi: 10.1016/j.ttbdis.2011.11.003
84. Quarsten H, Skarpaas T, Fajs L, Noraas S, Kjelland V. Tick-borne bacteria in Ixodes ricinus collected in southern Norway evaluated by a commercial kit and established real-time PCR protocols. Ticks Tick Borne Dis. (2015) 6:538–44. doi: 10.1016/j.ttbdis.2015.04.008
85. Michelet L, Delannoy S, Devillers E, Umhang G, Aspan A, Juremalm M, et al. High-throughput screening of tick-borne pathogens in Europe. Front Cell Infect Microbiol. (2014) 4:103. doi: 10.3389/fcimb.2014.00103
86. Tijsse-Klasen E, Hansford KM, Jahfari S, Phipps P, Sprong H, Medlock JM. Spotted fever group rickettsiae in Dermacentor reticulatus and Haemaphysalis punctata ticks in the UK. Parasit Vec. (2013) 6:212. doi: 10.1186/1756-3305-6-212
87. Mancini F, Vescio MF, Toma L, Di Luca M, Severini F, Caccio SM, et al. Detection of tick-borne pathogens in ticks collected in the suburban area of Monte Romano, Lazio Region, Central Italy. Ann Ist Super Sanita. (2019) 55:143–50. doi: 10.4415/ann_19_02_06
88. Toma L, Mancini F, Di Luca M, Cecere JG, Bianchi R, Khoury C, et al. Detection of microbial agents in ticks collected from migratory birds in central Italy. Vector Borne Zoon. Dis. (2014) 14:199–205. doi: 10.1089/vbz.2013.1458
89. Desjardins I, Joulié A, Pradier S, Lecollinet S, Beck C, Vial L, et al. Seroprevalence of horses to Coxiella burnetii in an Q fever endemic area. Vet Microbiol. (2018) 215:49–56. doi: 10.1016/j.vetmic.2017.11.012
90. Toledo A, Jado I, Olmeda AS, Casado-Nistal MA, Gil H, Escudero R, et al. Detection of Coxiella burnetii in ticks collected from Central Spain. Vector Borne Zoon. Dis. (2009) 9:465–8. doi: 10.1089/vbz.2008.0070
91. Spitalska E, Kocianova E. Detection of Coxiella burnetii in ticks collected in Slovakia and Hungary. Eur J Epidemiol. (2003) 18:263–6. doi: 10.1023/a:1023330222657
92. Bonnet S, de la Fuente J, Nicollet P, Liu X, Madani N, Blanchard B, et al. Prevalence of tick-borne pathogens in adult Dermacentor spp. ticks from nine collection sites in France. Vector Borne Zoon. Dis. (2013) 13:226–36. doi: 10.1089/vbz.2011.0933
93. Varela-Castro L, Zuddas C, Ortega N, Serrano E, Salinas J, Castellà J, et al. On the possible role of ticks in the eco-epidemiology of Coxiella burnetii in a Mediterranean ecosystem. Ticks Tick Borne Dis. (2018) 9:687–94. doi: 10.1016/j.ttbdis.2018.02.014
94. Santos-Silva MM, Melo P, Santos N, Antunes S, Duarte LR, Ferrolho J, et al. PCR screening of tick-borne agents in sensitive conservation areas, Southeast Portugal. Mol Cell Probes. (2017) 31:42–5. doi: 10.1016/j.mcp.2016.11.005
95. Chaligiannis I, Fernandez de Mera IG, Papa A, Sotiraki S, de la Fuente J. Molecular identification of tick-borne pathogens in ticks collected from dogs and small ruminants from Greece. Exp Appl Acarol. (2018) 74:443–53. doi: 10.1007/s10493-018-0237-z
96. Santos AS, de Bruin A, Veloso AR, Marques C, Pereira da Fonseca I, de Sousa R, et al. Detection of Anaplasma phagocytophilum, Candidatus Neoehrlichia sp., Coxiella burnetii and Rickettsia spp. in questing ticks from a recreational park, Portugal. Ticks Tick Borne Dis. (2018) 9:1555–64. doi: 10.1016/j.ttbdis.2018.07.010
97. Chisu V, Loi F, Foxi C, Chessa G, Masu G, Rolesu S, et al. Coexistence of tick-borne pathogens in ticks collected from their hosts in Sardinia: an update. Acta Parasitol. (2020). doi: 10.1007/s11686-020-00240-z
98. Körner S, Makert GR, Mertens-Scholz K, Henning K, Pfeffer M, Starke A, et al. Uptake and fecal excretion of Coxiella burnetii by Ixodes ricinus and Dermacentor marginatus ticks. Parasit Vec. (2020) 13:75. doi: 10.1186/s13071-020-3956-z
99. Papa A, Tsioka K, Kontana A, Papadopoulos C, Giadinis N. Bacterial pathogens and endosymbionts in ticks. Ticks Tick Borne Dis. (2017) 8:31–5. doi: 10.1016/j.ttbdis.2016.09.011
100. Sprong H, Fonville M, Docters van Leeuwen A, Devillers E, Ibañez-Justicia A, Stroo A, et al. Detection of pathogens in Dermacentor reticulatus in northwestern Europe: evaluation of a high-throughput array. Heliyon. (2019) 5:e01270. doi: 10.1016/j.heliyon.2019.e01270
101. Tomanovic S, Chochlakis D, Radulovic Z, Milutinovic M, Cakic S, Mihaljica D, et al. Analysis of pathogen co-occurrence in host-seeking adult hard ticks from Serbia. Exp Appl Acarol. (2013) 59:367–76. doi: 10.1007/s10493-012-9597-y
102. Spitalska E, Sparagano O, Stanko M, Schwarzova K, Spitalsky Z, Skultety L, et al. Diversity of Coxiella-like and Francisella-like endosymbionts, and Rickettsia spp., Coxiella burnetii as pathogens in the tick populations of Slovakia, Central Europe. Ticks Tick Borne Dis. (2018) 9:1207–11. doi: 10.1016/j.ttbdis.2018.05.002
103. Zhang Y-K, Yu Z-J, Wang D, Bronislava V, Branislav P, Liu J-Z. The bacterial microbiome of field-collected Dermacentor marginatus and Dermacentor reticulatus from Slovakia. Parasit Vec. (2019) 12:325. doi: 10.1186/s13071-019-3582-9
104. Spitalska E, Kocianova E. Tick-borne microorganisms in southwestern Slovakia. Ann N Y Acad Sci. (2003) 990:196–200. doi: 10.1111/j.1749-6632.2003.tb07362.x
105. Spitalská E, Kocianová E, Výrosteková V. Natural focus of Coxiella burnetii and Rickettsiae of spotted fever group in southwestern Slovakia. Biologia. (2002) 57:585–91.
106. Barandika JF, Hurtado A, Garcia-Sanmartin J, Juste RA, Anda P, Garcia-Perez AL. Prevalence of tick-borne zoonotic bacteria in questing adult ticks from northern Spain. Vector Borne Zoon. Dis. (2008) 8:829–35. doi: 10.1089/vbz.2008.0023
107. Pa?tiu AI, Matei IA, Mihalca AD, D'Amico G, Dumitrache MO, Kalmár Z, et al. Zoonotic pathogens associated with Hyalomma aegyptium in endangered tortoises: evidence for host-switching behaviour in ticks? Parasit Vec. (2012) 5:301. doi: 10.1186/1756-3305-5-301
108. Siroky P, Kubelova M, Modry D, Erhart J, Literak I, Spitalska E, et al. Tortoise tick Hyalomma aegyptium as long term carrier of Q fever agent Coxiella burnetii- evidence from experimental infection. Parasitol Res. (2010) 107:1515–20. doi: 10.1007/s00436-010-2037-1
109. Pascucci I, Di Domenico M, Dall'Acqua F, Sozio G, Cammà C. Detection of Lyme disease and Q Fever agents in wild rodents in Central Italy. Vector Borne Zoon. Dis. (2015) 15:404–11. doi: 10.1089/vbz.2015.1807
110. Vila A, Estrada-Peña A, Altet L, Cusco A, Dandreano S, Francino O, et al. Endosymbionts carried by ticks feeding on dogs in Spain. Ticks Tick Borne Dis. (2019) 10:848–52. doi: 10.1016/j.ttbdis.2019.04.003
111. Sprong H, Tijsse-Klasen E, Langelaar M, De Bruin A, Fonville M, Gassner F, et al. Prevalence of Coxiella burnetii in ticks after a large outbreak of Q fever. Zoon Publ Health. (2012) 59:69–75. doi: 10.1111/j.1863-2378.2011.01421.x
112. Reye AL, Stegniy V, Mishaeva NP, Velhin S, Hubschen JM, Ignatyev G, et al. Prevalence of tick-borne pathogens in Ixodes ricinus and Dermacentor reticulatus ticks from different geographical locations in Belarus. PLoS ONE. (2013). 8:e54476. doi: 10.1371/journal.pone.0054476
113. Smetanová K, Schwarzová K, Kocianová E. Detection of Anaplasma phagocytophilum, Coxiella burnetii, Rickettsia spp., and Borrelia burgdorferi s. l. in ticks, and wild-living animals in western and middle Slovakia. Ann N Y Acad Sci. (2006) 1078:312–5. doi: 10.1196/annals.1374.058
114. Szymanska-Czerwinska M, Galinska EM, Niemczuk K, Zasepa M. Prevalence of Coxiella burnetii infection in foresters and ticks in the south-eastern Poland and comparison of diagnostic methods. Ann Agric Environ Med. (2013) 20:699–704. Available online at: http://www.aaem.pl/Prevalence-of-Coxiella-burnetii-infection-in-foresters-in-the-south-eastern-Poland,72000,0,2.html
115. Kazimírová M, Hamšíková Z, Špitalská E, Minichová L, Mahríková L, Caban R, et al. Diverse tick-borne microorganisms identified in free-living ungulates in Slovakia. Parasit Vect. (2018) 11:495. doi: 10.1186/s13071-018-3068-1
116. Bielawska-Drozd A, Cieslik P, Zakowska D, Glowacka P, Wlizlo-Skowronek B, Zieba P, et al. Detection of Coxiella burnetii and Francisella tularensis in tissues of wild-living animals and in ticks of north-west Poland. Pol J Microbiol. (2018) 67:529–34. doi: 10.21307/pjm-2018-059
117. Hildebrandt A, Franke J, Schmook G, Pauliks K, Krämer A, Straube E. Diversity and coexistence of tick-borne pathogens in Central Germany. J Med Entomol. (2011) 48:651–5. doi: 10.1603/ME10254
118. Schabereiter-Gurtner C, Lubitz W, Rölleke S. Application of broad-range 16S rRNA PCR amplification and DGGE fingerprinting for detection of tick-infecting bacteria. J Microbiol Methods. (2003) 52:251–60. doi: 10.1016/s0167-7012(02)00186-0
119. Raele DA, Galante D, Pugliese N, De Simone E, Cafiero MA. Coxiella-like endosymbiont associated to the “Anatolian brown tick” Rhipicephalus bursa in Southern Italy. Microbes Infect. (2015) 17:799–805. doi: 10.1016/j.micinf.2015.09.011
120. Socolovschi C, Reynaud P, Kernif T, Raoult D, Parola P. Rickettsiae of spotted fever group, Borrelia valaisiana, and Coxiella burnetii in ticks on passerine birds and mammals from the Camargue in the south of France. Ticks Tick Borne Dis. (2012) 3:355–60. doi: 10.1016/j.ttbdis.2012.10.019
121. Bogunovic D, Stević N, Sidi-Boumedine K, Misic D, Tomanović S, Kulišić Z, et al. Molecular evidence of Q Fever agent Coxiella burnetii in ixodid ticks collected from stray dogs in Belgrade (Serbia). Acta Veterin. (2018) 68:257–68. doi: 10.2478/acve-2018-0023
122. Bernasconi MV, Casati S, Peter O, Piffaretti JC. Rhipicephalus ticks infected with Rickettsia and Coxiella in Southern Switzerland (Canton Ticino). Infect Genet Evol. (2002) 2:111–20. doi: 10.1016/s1567-1348(02)00092-8
123. Beltrame A, Laroche M, Degani M, Perandin F, Bisoffi Z, Raoult D, et al. Tick-borne pathogens in removed ticks Veneto, northeastern Italy: a cross-sectional investigation. Travel Med Infect Dis. (2018) 26:58–61. doi: 10.1016/j.tmaid.2018.08.008
124. Kalmár Z, Dumitrache M, d'amico G, Matei I, Ionică A, Gherman C, et al. Multiple tick-borne pathogens in Ixodes ricinus ticks collected from humans in Romania. Pathogens. (2020) 9:390. doi: 10.3390/pathogens9050390
125. Pajoro M, Pistone D, Varotto Boccazzi I, Mereghetti V, Bandi C, Fabbi M, et al. Molecular screening for bacterial pathogens in ticks (Ixodes ricinus) collected on migratory birds captured in northern Italy. Folia Parasitol (Praha). (2018) 65:2018.008. doi: 10.14411/fp.2018.008
126. Henning K, Greiner-Fischer S, Hotzel H, Ebsen M, Theegarten D. Isolation of Spiroplasma sp. from an Ixodes tick. Int J Med Microbiol. (2006) 296(Suppl 40):157–61. doi: 10.1016/j.ijmm.2006.01.012
127. Andersson MO, Tolf C, Tamba P, Stefanache M, Radbea G, Frangoulidis D, et al. Molecular survey of neglected bacterial pathogens reveals an abundant diversity of species and genotypes in ticks collected from animal hosts across Romania. Parasit Vec. (2018) 11:144. doi: 10.1186/s13071-018-2756-1
128. Williams HW, Cross DE, Crump HL, Drost CJ, Thomas CJ. Climate suitability for European ticks: assessing species distribution models against null models and projection under AR5 climate. Parasit Vec. (2015) 8:440. doi: 10.1186/s13071-015-1046-4
129. Wallenhammar A, Lindqvist R, Asghar N, Gunaltay S, Fredlund H, Davidsson Å, et al. Revealing new tick-borne encephalitis virus foci by screening antibodies in sheep milk. Parasit Vec. (2020) 13:185. doi: 10.1186/s13071-020-04030-4
130. Zeman P. Objective assessment of risk maps of tick-borne encephalitis and Lyme borreliosis based on spatial patterns of located cases. Int J Epidemiol. (1997) 26:1121–9. doi: 10.1093/ije/26.5.1121
131. Pluta S, Hartelt K, Oehme R, Mackenstedt U, Kimmig P. Prevalence of Coxiella burnetii and Rickettsia spp. in ticks and rodents in southern Germany. Ticks Tick Borne Dis. (2010) 1:145–7. doi: 10.1016/j.ttbdis.2010.04.001
132. Rehacek J, Kaaserer B, Urvolgyi J, Lukacova M, Kovacova E, Kocianova E. Isolation of Coxiella burnetii and of an unknown rickettsial organism from Ixodes ricinus ticks collected in Austria. Eur J Epidemiol. (1994) 10:719–23. doi: 10.1007/bf01719288
133. Liebisch A. Das Q-Fieber als Naturherdinfektion in Süddeutschland. Bundesgesundheitsblatt. (1977) 20:185–91.
134. Cooper A, Stephens J, Ketheesan N, Govan B. Detection of Coxiella burnetii DNA in wildlife and ticks in northern Queensland, Australia. Vector Borne Zoon Dis. (2013) 13:12–6. doi: 10.1089/vbz.2011.0853
135. Davoust B, Marié JL, Pommier de Santi V, Berenger JM, Edouard S, Raoult D. Three-toed sloth as putative reservoir of Coxiella burnetii, Cayenne, French Guiana. Emerg Infect Dis. (2014) 20:1760–1. doi: 10.3201/eid2010.140694
136. Ullah Q, El-Adawy H, Jamil T, Jamil H, Qureshi ZI, Saqib M, et al. Serological and molecular investigation of Coxiella burnetii in small ruminants and ticks in Punjab, Pakistan. Int J Environ Res Public Health. (2019) 16. doi: 10.3390/ijerph16214271
137. Noda AA, Rodríguez I, Miranda J, Contreras V, Mattar S. First molecular evidence of Coxiella burnetii infecting ticks in Cuba. Ticks Tick Borne Dis. (2016) 7:68–70. doi: 10.1016/j.ttbdis.2015.08.008
138. Hornok S, Abichu G, Meli ML, Tánczos B, Sulyok KM, Gyuranecz M, et al. Influence of the biotope on the tick infestation of cattle and on the tick-borne pathogen repertoire of cattle ticks in Ethiopia. PLoS ONE. (2014) 9:e106452. doi: 10.1371/journal.pone.0106452
139. Ni J, Lin H, Xu X, Ren Q, Aizezi M, Luo J, et al. Coxiella burnetii is widespread in ticks (Ixodidae) in the Xinjiang areas of China. BMC Vet Res. (2020) 16:317. doi: 10.1186/s12917-020-02538-6
140. Loftis AD, Reeves WK, Szumlas DE, Abbassy MM, Helmy IM, Moriarity JR, et al. Rickettsial agents in Egyptian ticks collected from domestic animals. Exp Appl Acarol. (2006) 40:67. doi: 10.1007/s10493-006-9025-2
141. Pacheco RC, Echaide IE, Alves RN, Beletti ME, Nava S, Labruna MB. Coxiella burnetii in ticks, Argentina. Emerg Infect Dis. (2013) 19:344–6. doi: 10.3201/eid1902.120362
142. Reye AL, Arinola OG, Hübschen JM, Muller CP. Pathogen prevalence in ticks collected from the vegetation and livestock in Nigeria. Appl Environ Microbiol. (2012) 78:2562–8. doi: 10.1128/aem.06686-11
143. Andoh M, Andoh R, Teramoto K, Komiya T, Kaneshima T, Takano A, et al. Survey of Coxiella burnetii in ticks collected from dogs in Japan. J Vet Med Sci. (2013) 75:1115–7. doi: 10.1292/jvms.12-0570
144. Cardinale E, Esnault O, Beral M, Naze F, Michault A. Emergence of Coxiella burnetii in ruminants on Reunion Island? Prevalence and risk factors. PLoS Negl Trop Dis. (2014) 8:e3055. doi: 10.1371/journal.pntd.0003055
145. Nelder MP, Reeves WK, Adler PH, Wozniak A, Wills W. Ectoparasites and associated pathogens of free-roaming and captive animals in zoos of South Carolina. Vector Borne Zoon. Dis. (2009) 9:469–77. doi: 10.1089/vbz.2008.0008
146. Sanders DM, Parker JE, Walker WW, Buchholz MW, Blount K, Kiel JL. Field collection and genetic classification of tick-borne Rickettsiae and Rickettsiae-like pathogens from South Texas: Coxiella burnetii isolated from field-collected Amblyomma cajennense. Ann N Y Acad Sci. (2008) 1149:208–11. doi: 10.1196/annals.1428.022
147. Estrada-Pena A, Gray JS, Kahl O, Lane RS, Nijhof AM. Research on the ecology of ticks and tick-borne pathogens-methodological principles and caveats. Front Cell Infect Microbiol. (2013) 3:29. doi: 10.3389/fcimb.2013.00029
148. Bolanos-Rivero M, Carranza-Rodriguez C, Rodriguez NF, Gutierrez C, Perez-Arellano JL. Detection of Coxiella burnetii DNA in peridomestic and wild animals and ticks in an endemic region (Canary Islands, Spain). Vector Borne Zoon. Dis. (2017) 17:630–4. doi: 10.1089/vbz.2017.2120
149. González-Barrio D, Jado I, Fernández-de-Mera IG, Del Rocio Fernández-Santos M, Rodríguez-Vargas M, García-Amil C, et al. Genotypes of Coxiella burnetii in wildlife: disentangling the molecular epidemiology of a multi-host pathogen. Environ Microbiol Rep. (2016) 8:708–14. doi: 10.1111/1758-2229.12431
150. Michelet L, Joncour G, Devillers E, Torina A, Vayssier-Taussat M, Bonnet SI, et al. Tick species, tick-borne pathogens and symbionts in an insular environment off the coast of Western France. Ticks Tick Borne Dis. (2016) 7:1109–15. doi: 10.1016/j.ttbdis.2016.08.014
151. Dubourg G, Socolovschi C, Del Giudice P, Fournier PE, Raoult D. Scalp eschar and neck lymphadenopathy after tick bite: an emerging syndrome with multiple causes. Eur J Clin Microbiol Infect Dis. (2014) 33:1449–56. doi: 10.1007/s10096-014-2090-2
152. Ciliberti A, Gavier-Widén D, Yon L, Hutchings MR, Artois M. Prioritisation of wildlife pathogens to be targeted in European surveillance programmes: expert-based risk analysis focus on ruminants. Prev Vet Med. (2015) 118:271–84. doi: 10.1016/j.prevetmed.2014.11.021
153. Mori M, Mertens K, Cutler SJ, Santos AS. Critical aspects for detection of Coxiella burnetii. Vector Borne Zoon. Dis. (2017) 17:33–41. doi: 10.1089/vbz.2016.1958
154. Dupraz M, Toty C, Devillers E, Blanchon T, Elguero E, Vittecoq M, et al. Population structure of the soft tick Ornithodoros maritimus and its associated infectious agents within a colony of its seabird host Larus michahellis. Int J Parasitol Parasites Wildl. (2017) 6:122–30. doi: 10.1016/j.ijppaw.2017.05.001
155. Binetruy F, Buysse M, Lejarre Q, Barosi R, Villa M, Rahola N, et al. Microbial community structure reveals instability of nutritional symbiosis during the evolutionary radiation of Amblyomma ticks. Mol Ecol. (2020) 29:1016–29. doi: 10.1111/mec.15373
156. Coimbra-Dores MJ, Jaarsma RI, Carmo AO, Maia-Silva M, Fonville M, da Costa DFF, et al. Mitochondrial sequences of Rhipicephalus and Coxiella endosymbiont reveal evidence of lineages co-cladogenesis. FEMS Microbiol Ecol. (2020) 96(6). doi: 10.1093/femsec/fiaa072
157. Klyachko O, Stein BD, Grindle N, Clay K, Fuqua C. Localization and visualization of a Coxiella-type symbiont within the lone star tick, Amblyomma americanum. Appl Environ Microbiol. (2007) 73:6584–94. doi: 10.1128/aem.00537-07
158. Brenner AE, Muñoz-Leal S, Sachan M, Labruna MB, Raghavan R. Ticks convert pathogenic Coxiella into endosymbionts. bioRxiv. (2020). doi: 10.1101/2020.12.29.424491
159. Li L-H, Zhang Y, Zhu D, Zhou X-N. Endosymbionts alter larva-to-nymph transstadial transmission of Babesia microti in Rhipicephalus haemaphysaloides ticks. (2018) 9:1415. doi: 10.3389/fmicb.2018.01415
160. Gofton AW, Oskam CL, Lo N, Beninati T, Wei H, McCarl V, et al. Inhibition of the endosymbiont “Candidatus Midichloria mitochondrii” during 16S rRNA gene profiling reveals potential pathogens in Ixodes ticks from Australia. Parasit Vec. (2015) 8:345. doi: 10.1186/s13071-015-0958-3
161. González J, González MG, Valcárcel F, Sánchez M, Martín-Hernández R, Tercero JM, et al. Transstadial transmission from nymph to adult of Coxiella burnetii by naturally infected Hyalomma lusitanicum. Pathogens. (2020) 9:884. doi: 10.3390/pathogens9110884
162. Eisen L. Vector competence studies with hard ticks and Borrelia burgdorferi sensu lato spirochetes: a review. Ticks Tick Borne Dis. (2020) 11:101359. doi: 10.1016/j.ttbdis.2019.101359
163. Salazar R, Castillo-Neyra R, Tustin AW, Borrini-Mayorí K, Náquira C, Levy MZ. Bed bugs (Cimex lectularius) as vectors of Trypanosoma cruzi. Am J Trop Med Hyg. (2015) 92:331–5. doi: 10.4269/ajtmh.14-0483
164. Houhamdi L, Fournier P-E, Fang R, Lepidi H, Raoult D. An experimental model of human body louse infection with Rickettsia prowazekii. J Infect Dis. (2002) 186:1639–46. doi: 10.1086/345373
165. Selmi R, Mamlouk A, Ben Yahia H, Abdelaali H, Ben Said M, Sellami K, et al. Coxiella burnetii in Tunisian dromedary camels (Camelus dromedarius): Seroprevalence, associated risk factors and seasonal dynamics. Acta Trop. (2018) 188:234–9. doi: 10.1016/j.actatropica.2018.09.008
166. Cikman A, Aydin M, Gulhan B, Karakecili F, Ozcicek A, Kesik O, et al. The seroprevalence of Coxiella burnetii in Erzincan, Turkey: identification of the risk factors and their relationship with geographical features. (2017) 54:157–63. Available online at: https://www.jvbd.org/downloadpdf.asp?
167. Menadi SE, Mura A, Santucciu C, Ghalmi F, Hafsi F, Masala G. Seroprevalence and risk factors of Coxiella burnetii infection in cattle in northeast Algeria. Trop Anim Health Prod. (2019) 52:935–42. doi: 10.1007/s11250-019-02083-x
168. van Engelen E, Schotten N, Schimmer B, Hautvast JL, van Schaik G, van Duijnhoven YT. Prevalence and risk factors for Coxiella burnetii (Q fever) in Dutch dairy cattle herds based on bulk tank milk testing. Prev Vet Med. (2014) 117:103–9. doi: 10.1016/j.prevetmed.2014.08.016
Keywords: ticks, Coxiella, prevalence, molecular detection, Coxiella-like, endosymbionts, vector
Citation: Körner S, Makert GR, Ulbert S, Pfeffer M and Mertens-Scholz K (2021) The Prevalence of Coxiella burnetii in Hard Ticks in Europe and Their Role in Q Fever Transmission Revisited—A Systematic Review. Front. Vet. Sci. 8:655715. doi: 10.3389/fvets.2021.655715
Received: 19 January 2021; Accepted: 22 February 2021;
Published: 26 April 2021.
Edited by:
Sandra Diaz Sanchez, University of Castilla-La Mancha, SpainReviewed by:
Sandra Antunes, Universidade NOVA de Lisboa, PortugalQuan Liu, Foshan University, China
Copyright © 2021 Körner, Makert, Ulbert, Pfeffer and Mertens-Scholz. This is an open-access article distributed under the terms of the Creative Commons Attribution License (CC BY). The use, distribution or reproduction in other forums is permitted, provided the original author(s) and the copyright owner(s) are credited and that the original publication in this journal is cited, in accordance with accepted academic practice. No use, distribution or reproduction is permitted which does not comply with these terms.
*Correspondence: Katja Mertens-Scholz, katja.mertens-scholz@fli.de