Erratum: Evaluation of Three Medetomidine-Based Anesthetic Protocols in Free-Ranging Wild Boars (Sus scrofa)
- 1Department of Forestry and Wildlife Management, Inland Norway University of Applied Sciences, Koppang, Norway
- 2Wildlife Diseases Unit, French Agency for Biodiversity, Gap, France
- 3François Sommer Foundation, Paris, France
- 4Vetsuisse Faculty, Veterinary Public Health Institute, University of Bern, Bern, Switzerland
- 5Department of Wildlife, Fish and Environmental Studies, Swedish University of Agricultural Sciences, Umeå, Sweden
Three medetomidine-based drug protocols were compared by evaluating time courses, reliability and physiological effects in wild boars. A total of 21 cage-trapped wild boars (Sus scrofa) were immobilized using one of the following drug combinations; MTZ: medetomidine (0.2 mg/kg) + tiletamine-zolazepam (2.0 mg/kg), MK: medetomidine (0.15 mg/kg) + ketamine (5 mg/kg), and MKB: medetomidine (0.1 mg/kg) + ketamine (5.0 mg/kg) + butorphanol (0.2 mg/kg). Induction time, recovery time, and physiological variables were recorded and arterial blood gas analysis measured twice, before and after 15 min of oxygen supplementation (0.5–1.0 L/min). For reversal, 4 mg of atipamezole per mg of medetomidine was administered intramuscularly. The boars recovered in the cage and were released once ataxia resolved. The MK group had significantly longer recovery times (mean 164 min ± 79 SD) compared to the other groups. MKB elicited longer and incomplete induction compared to the other groups (mean induction time 20 min ± 10 SD), decreasing the efficiency of the capture and increasing the risk of hyperthermia. Both ketamine-based protocols required additional ketamine intramuscularly to prolong the anesthesia after 20–40 min from induction. Agreement between the pulse oximeter and the blood gas analyzer was low, with the pulse oximeter underestimating the real values of arterial oxyhemoglobin saturation, particularly at higher readings. Mild acute respiratory acidosis (PaCO2 45–60 mmHg) and mild to moderate hypoxemia (PaO2 69–80 mmHg) occurred in most boars, regardless of the treatment group but especially in the MKB group. The acid-base status improved and hypoxemia resolved in all boars during oxygen supplementation, with the PaO2 rising above the physiological reference range (81.6–107.7 mmHg) in many individuals. MK and MKB induced safe and reliable immobilization of wild boars for at least 20 min. Supplemental oxygen delivery is recommended in order to prevent hypoxemia in wild boars immobilized with the protocols used in the present study. Long and ataxic recoveries occurred in most animals, regardless of the protocol, but especially in the MKB group.
Introduction
Wild boars (Sus scrofa) are frequently captured and either physically or chemically restrained for research and management purposes (1, 2). In order to reduce the stress and safety risks for both humans and animals, chemical immobilization is the preferred method of restraint. Anesthesia is a more complex state than chemical immobilization and is defined as “drug-induced unconsciousness that is characterized by controlled but reversible depression of the central nervous system and perception. In this state, the patient is not arousable by noxious stimulation” (3). Most recommended drug protocols for wild boar anesthesia are based on one of the cyclohexamine anesthetic drug, ketamine or tiletamine (4–6). Tiletamine, which is only available in a 1:1 ratio with the benzodiazepine agonist zolazepam, is more potent and has a longer elimination half-life than ketamine. The main side effects of cyclohexamines include muscle rigidity and dose-dependent prolonged, ataxic recoveries (1, 7). By choosing a balanced anesthetic combination that includes an alpha-2 adrenoceptor agonist, such as xylazine or medetomidine, these side effects can be alleviated (4). Medetomidine is more potent than xylazine and is the preferred alpha-2 adrenoceptor agonist. Medetomidine improves muscle relaxation and potentiates the primary anesthetic drugs so that its dose can be reduced. Additionally, the effects of medetomidine can be antagonized, making the cyclohexamine combinations partly reversible. Butorphanol, an opioid, has been used as an adjunct to further improve anesthesia and reduce dose requirements of the other drugs used in a combination (8–10).
Anesthetized wild boars are prone to hyperthermia (8) and hypoxemia (11) and the body temperature and blood oxygenation of immobilized animals should be frequently monitored. Pulse-oximeters are valuable tools for assessing oxygen saturation in immobilized wildlife, especially in field settings, where blood gas analysis is not routinely conducted. Nevertheless, the tendency of pulse oximeters to either underestimate or overestimate arterial oxyhemoglobin saturation and to lack in accuracy has been previously described in several mammalian species (12–14). Such inaccuracy requires that pulse oximetry is validated with the simultaneous use of a blood gas analyzer. Most anesthetized wildlife require oxygen supplementation in order to correct hypoxemia (5, 13), which could otherwise cause multiple organ dysfunction, including but not limiting to the central nervous system (15). Ultimately, such a physiological impact might also lead to biased results of the ecological studies dependent on capturing representative animals.
The aim of the present study was to evaluate three medetomidine-based protocols for anesthesia of trapped wild boars, to assess the effects of supplemental oxygen and to evaluate the reliability of pulse oximetry in this species compared to blood gas analysis.
Materials and Methods
Study Area and Period
The study was conducted in the National Estate of Chambord (47°36′ N, 1°31′ E, France), a 5,439 ha enclosed park located at low elevation (72–128 meters above sea level). Wild boars are fed daily throughout the year to maintain high densities. The study was carried out in September 2015 and in April 2017, with an ambient temperature of 6.0–17.5°C and a barometric pressure (PB) of 754–763 mmHg (16) during the captures.
Capture Methods and Drug Combinations
A total of 10 (2 × 1 × 1 meter) cage traps were set in the forest and baited with corn and pies daily 1 week prior the start of the captures. Traps were inspected early every morning during the capturing period. Traps were triggered by the animal when stepping on a bottom platform in the center of the trap, connected to a drop gate at the entry. In case of more than one wild boar present in the same trap, the animals were separated and moved into other cages prior to injection of the drugs. The body mass of the animals was estimated from a distance in order to adjust drug doses. The wild boars were injected with the anesthetic combination intramuscularly (IM) in the thigh muscle group using a automatic spring-loaded pole syringe including a multiuse 10 ml syringe and 30 × 2 mm needles (Dan-Inject®, Børkop DK-7080, Denmark). While the needle was changed for every injection, the syringe was not sterilized in between the injections. A total of two operators carried out the injections. Stress of the wild boars was not scored however it was higher at the time of the injection, with a remarkable stress response (i.e., charging and biting the cage). The cages were then covered with a tarp to minimize stress during induction of the drug effect.
Three different drug combinations were formulated following previous findings in similar studies in wild boar chemical immobilizations (17, 18), therefore the wild boars were divided into the following groups:
• MTZ group (medetomidine-tiletamine-zolazepam): 0.2 mg/kg of medetomidine (Zalopine®, Orion Pharma Animal Health, Turku 20101, Finland, 10 mg/ml) + 2 mg/kg of tiletamine-zolazepam (Zoletil Forte® Vet, Virbac S.A., Carros 06510, France, prepared by dissolving the powder in sterile water to a total drug concentration of 50 mg/ml).
• MK group (medetomidine-ketamine): 0.15 mg/kg of medetomidine + 5 mg/kg of ketamine (Narketan10®, Vetoquinol Ireland Ltd, Dublin 6, Ireland, 100 mg/ml).
• MKB group (medetomidine-ketamine-butorphanol): 0.1 mg/kg of medetomidine + 5 mg/kg of ketamine + 0.2 mg/kg of butorphanol (Alvegesic®, Dechra, Shrewsbury SY4 4AS, UK, 10 mg/ml).
Monitoring and Handling
The time from the first pole syringe injection to complete immobilization (induction time) was recorded. Induction problems were defined as animal not being recumbent after 10 min or not completely immobilized 20 min post injection. Depending on animal behavior, these animals were injected with an additional half or full dose 20 min after the first injection. Once anesthesia was achieved, the animals were approached, blindfolded and placed in lateral recumbency outside the trap. Rectal temperature (Tr) was immediately measured with a digital thermometer (Wellkang Ltd, CT16 1PW, UK) placed 10 cm deep into the rectum and then monitored every 10 min. Hyperthermia was considered as at least two consecutive measures above 39.5°C. Heart rate (HR), respiratory rate (RR) and peripheral oxyhemoglobin saturation (SpO2) were recorded every 10 min from induction to recovery using a stethoscope and a a portable pulse oximeter (Nellcor® NPB-40, Nellcor Inc., Pleasanton, California, USA) with the transmissive probe placed over the lingual artery. The pulse oximeter showed the pulse amplitude at all times by means of a subjective bar graph, reflecting the quality of the reading, allowing the researchers to promptly identify the risk of inaccurate readings. The depth of the immobilization was assessed every 5 min by monitoring the palpebral and corneal reflexes, the movements of the ears, HR, RR, and occasional muscle tremor. The wild boars were classified as juveniles (<12 months of age), subadults (12–24 months of age) or adults (>24 months of age) by assessing the eruption of the incisor teeth. Body mass was measured with an analog spring scale and all captured boars were ear tagged for individual identification.
On completion of the procedures, all animals received 4 mg of atipamezole (Antisedan®, Zoetis, Parsippany NJ 07054, USA, 5 mg/ml) per mg of medetomidine in the thigh muscle group to reverse the effects of the alpha-2 adrenoceptor agonist, as recommended in previous studies (19). Time between the initial drug injection and the reversal of the anesthesia was recorded (capture duration). In case of more than one animal captured in the same trap, they were moved to individual wooden boxes to recover alone prior to be released once ataxia was no longer present. Times of first sign of recovery (head lifting), standing, walking, and leaving the site were recorded. Recovery time was defined as the time from atipamezole injection to coordinated walking or release from the individual box.
Sample Collection and Processing
After induction of complete immobilization, an arterial blood sample was collected anaerobically from the femoral artery using heparinized 1 ml syringes (Smiths Medical ASD, Inc., Keene NH 03431, USA) and 20 × 0.6 mm needles. The sample was immediately analyzed using an i-STAT®1 Portable Clinical Analyzer and i-STAT® CG8+ and Chem8+ cartridges (Abbott Laboratories, Abbott Park IL 60064–6048, USA). The analyzer was kept in an insulated box to maintain a temperature between 16 and 30°C. Measured variables included pH, arterial partial pressure of oxygen (PaO2), arterial partial pressure of carbon dioxide (PaCO2), lactate, sodium (Na), potassium (K), chloride (Cl), ionized calcium (iCa), hematocrit (Hct), glucose and urea (BUN). PaO2, PaCO2 and pH were corrected based on Tr. Calculated values included base excess (BE), bicarbonate (), total carbon dioxide (TCO2), arterial oxyhemoglobin saturation (SaO2), hemoglobin (Hb), and anion gap (AG). After the arterial blood sampling, oxygen was delivered from a portable oxygen concentrator (Philips Respironics EverGo, Advanced Aeromedical, Inc., Virginia Beach VA 2345, USA) at a flow rate of 0.5–1 L/min via a bi-prong nasal cannula (Minton Medical Supplies PTY, Ltd., Collingwood VIC 3066, Australia) placed 10 cm deep into the nostrils. Further, a second arterial sample was taken after 15 min and processed as mentioned above to assess the effects of the supplemental oxygen. The SpO2 values obtained via pulse oximetry at the time of the arterial blood samples were recorded and any mismatch between the HR measured with the pulse oximeter and the HR counted by means of the stethoscope was ruled out, to further ensure more accurate readings from the machine. In case of a HR mismatch or a low quality of pulse detected by the pulse oximeter, the probe site was flushed with sterile crystalloid solution, wiped and the probe was reattached. The alveolar-arterial oxygen tension difference (P(A−a)O2) prior to oxygen delivery was estimated for the Tr corrected values, based on calculation of the alveolar partial pressure of oxygen (PAO2) calculated from the alveolar gas equation [PAO2 = FiO2 (PB - PH2O) - (PaCO2/RQ)], where PB = barometric pressure detected by the iSTAT® at the time of the capture, FiO2 = fraction of inspired oxygen (0.21) and PH2O = saturated vapor pressure for water at 37°C (47 mmHg). The respiratory quotient (RQ) was assumed to be 1.1 for wild boars (2). Since the FiO2 was unknown at the time of the second arterial sample, P(A−a)O2 was not calculated. Hypoxemia was defined as mild (PaO2 80–70 mmHg; SaO2 97–90%), moderate (PaO2 69–60; SaO2 89–78%), or severe (PaO2 ≤ 59 mmHg; SaO2 ≤ 77%) according to the iSTAT® PaO2 and SaO2. Hypercapnia was defined as mild (PaCO2 45–60 mmHg), moderate (PaCO2 61–75 mmHg) and severe (PaCO2 > 75 mmHg) according to the iSTAT® PaCO2.
Statistical Analysis
Microsoft Excel 2019 was used to conduct simple descriptive statistics including mean and standard deviation (SD) for body mass, capture parameters (drug doses, induction time, capture time, recovery time), anesthesia variables (Tr, HR, RR, SpO2), and all variables from blood gas, hematological, and serum biochemistry analyses, for every animal and within protocol groups. Kurtosis, skewness and their standard error were also calculated to confirm that the data within each protocol group were normally distributed.
R-3.5.3 (20) software was used to conduct a Kruskal-Wallis test by rank to investigate significant differences among protocol groups. A Dunn's test with simple Bonferroni method for p-value adjustment was used as post-hoc. Paired samples Wilcoxon test was used for the blood gas, hematological and serum biochemistry variables between the first and the second sample. Further, all simultaneous SpO2-SaO2 sets of values were matched and Lin's concordance correlation coefficient (ρc) was calculated to validate the concordance between the SpO2 values from the pulse-oximeter to the “gold standard” SaO2 values from the iSTAT® analyzer, with a strong correlation considered for |ρc| > 0.9. Agreement between saturation measurements was evaluated by use of Bland-Altman analysis. In this analysis, the mean of the two measurements is plotted against the difference in the measurements, and at least 95% of the elements must lay within the upper and lower limits of agreement (LoA) to suggest a fair agreement. Acceptable agreement was considered a priori for |LoA| < 5% between paired SaO2-SpO2 values. We will refer to this threshold as clinically acceptable limit (CAL). A Wilcoxon rank sum test was used to determine significant differences between paired SpO2 and SaO2 sets of values. Non-parametric analysis results were considered statistically significant at p < 0.05.
Results
Efficiency and Reliability of the Capture
A total of 32 animals triggered the traps during the study period: 31 wild boars, including 10 piglets which were immediately released, and a young red deer. Trapping of multiple animals in the same cage occurred in three cases and all the individuals suitable for the study were immobilized at the same time. Twenty-one wild boars were anesthetized (8 with MTZ, 7 with MK and 6 with MKB), monitored, and released in the study area. No evidence of incomplete emptying of the pole syringe was recorded. Animals in the MTZ group were younger, with a lower body mass, than those in the other two groups. Mean (± SD) body mass, drug doses, induction time, capture time, recovery time and induction problems are presented in Table 1.
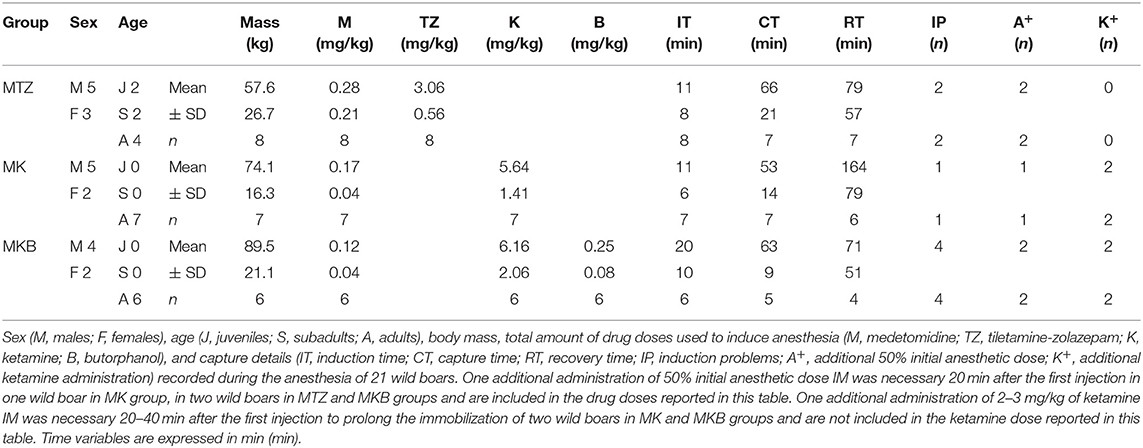
Table 1. Drug doses and capture time course data of cage-trapped wild boars anesthetized using medetomidine-tiletamine-zolazepam (MTZ; n = 8) or medetomidine-ketamine (MK; n = 7) or medetomidine-ketamine-butorphanol (MKB; n = 6).
Average initial dose of MTZ was 1.5 times higher than initially planned because lower doses were associated with induction problems (in two out of eight animals, both recumbent over 10 min after the first injection). The average actual MK and MKB doses were higher than the targeted dose by 11.7 and 12.3%, respectively, due to inaccurate body mass estimation. Overall, a second pole-syringe injection with an additional half induction dose was necessary 20 min after the first injection in 5 out of 21 boars: 2 out of 8 in the MTZ group, 1 out of 7 in the MK group and 2 out 6 in the MKB group. A higher number of induction problems occurred in the MKB group: 4 out of 6, all recumbent by 10 min from the initial injection, but not completely immobilized within 20 min. In contrast, only 1 induction problem was recorded (1 out of 7 wild boars, not fully induced by 20 min from the injection) in the MK group. No significant differences in induction times were detected among the groups.
Partial arousal during handling were more frequent in the MK (in 3 out of 7 boars) and the MKB (4 out of 6) groups than in the MTZ group (1 out of 8), requiring additional 2–3 mg/kg of ketamine IM 20–40 min from the previous full or half dose in two cases in the MK group and in other two cases in the MKB group to prolong the anesthesia.
Pre-release mortality occurred in 2 out of 32 captured animals (6.2%), with none of them being anesthetized as both deaths occurred in piglets, due to trauma by the trap closure mechanism before the inspection of the traps.
Mild to moderate ataxia was observed in all animals during the recovery period, requiring a delay in the release of animals after the antidote administration (up to 4.3 h later). Mean recovery times did not differ significantly among groups, however only 2 out of 7 individuals of the MK group were fit to be released within 1.5 h from the injection of atipamezole compared to the 6 out of 8 and the 4 out of 6 in MTZ and MKB groups, respectively. No post-release mortality was reported during the 30 days following the capture.
Physiological Variables
Oxygen was delivered in only 17 out of 21 animals due to the occurrence of multiple trapped and immobilized animals at a time and the availability of only one portable oxygen concentrator. Despite the pulse amplitude and quality detected by the pulse oximeter were subjectively good, there was no acceptable correlation between pulse-oximetry values for SpO2 and blood gas analysis for SaO2 (|ρc| < 0.2). Agreement was below both the statistical and clinical thresholds, since only 93.7 and 65.6% of the values lied within the LoA and the CAL intervals, respectively, in the Bland-Altman plot. Pulse-oximetry underestimated blood oxygenation in 63% of cases regardless of the group and to a higher extent for higher SpO2 values. Concordance, correlation and agreement between SpO2 and SaO2 measurements are presented in Figures 1–3.
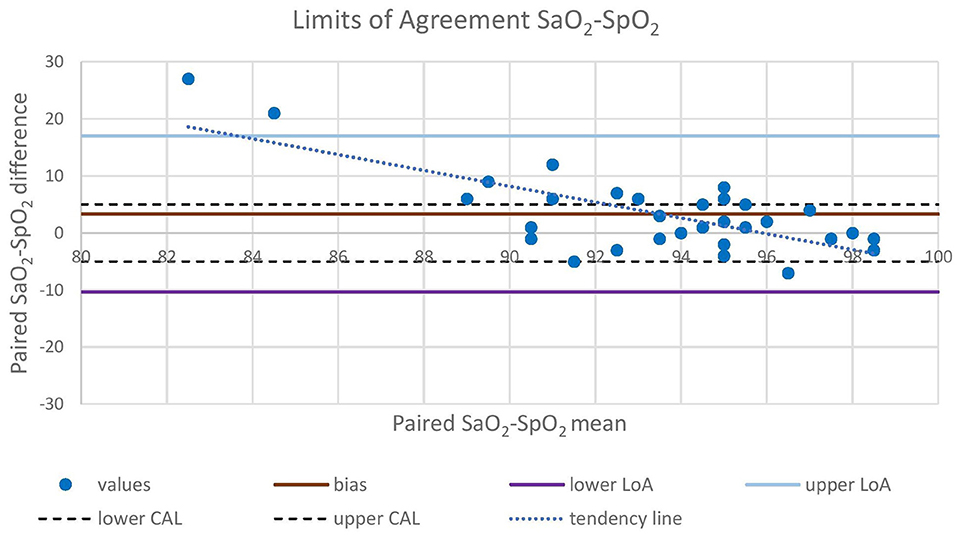
Figure 1. Bland-Altman analysis plots the difference between concurrent SaO2 and SpO2 values (Y-axis) against the mean between concurrent SaO2 and SpO2 values (X-axis). Mean difference (bias), upper LoA (bias + 1.96 SD), and lower LoA (bias – 1.96 SD) between paired values are represented with straight lines. Upper CAL (5%) and lower CAL (−5%) are represented with dashed lines. LoA, Limit of Agreement; CAL, Clinically Acceptable Limit; SaO2, arterial oxyhemoglobin saturation; SpO2, peripheral oxyhemoglobin saturation.
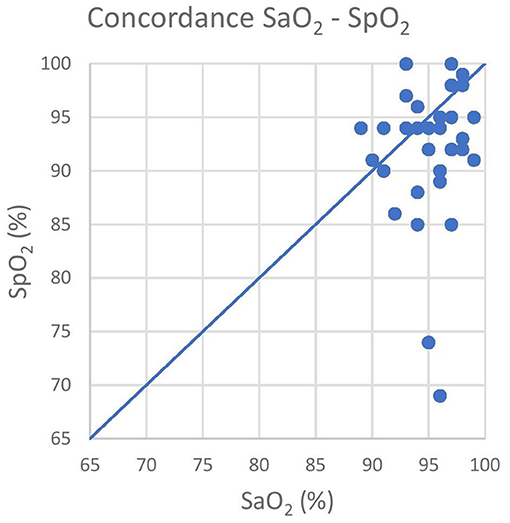
Figure 2. The graph shows the poor concordance between the pulse-oximeter (Y-axis) and the iSTAT (X-axis) results. The blue line represents the equality line, that is for the absolute concordance. SaO2, arterial oxyhemoglobin saturation; SpO2, peripheral oxyhemoglobin saturation.
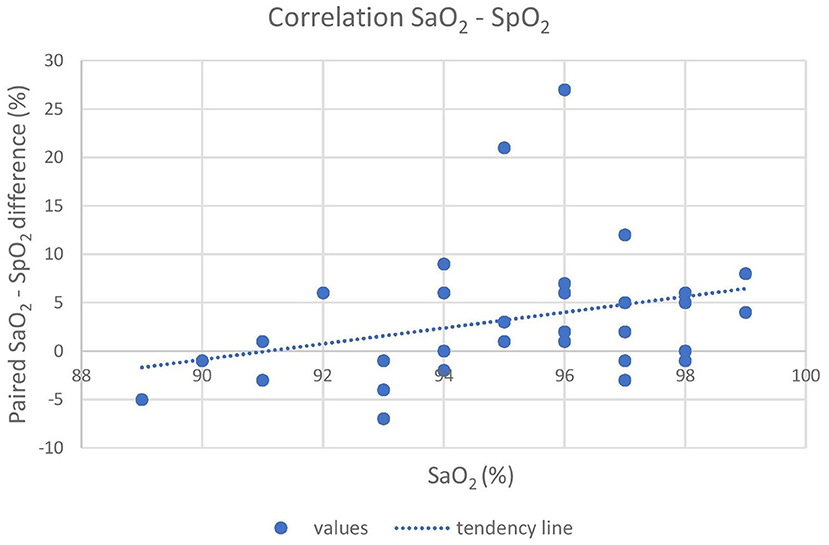
Figure 3. The graph shows the poor correlation between the pulse-oximeter and the iSTAT (X-axis) results. The difference between SaO2, and SpO2 is reported on the Y-axis. A tendency of the pulse-oximeter to underestimate actual SaO2, especially at high readings, is shown. SaO2, arterial oxyhemoglobin saturation; SpO2, peripheral oxyhemoglobin saturation.
Mean Tr, HR and RR trends were significantly different among protocol groups. Average Tr was higher (p = 0.005) in wild boars in the MKB group compared to the MTZ group, with a decreasing trend over time in both groups. Hyperthermia occurred in 3 out of 7 boars in the MK group and in 3 out of 6 boars in the MKB group. Hyperthermia was seen only in 1 out of 8 animals in the MTZ group. Mean HR was higher (p = 0.048) in the MK group compared to the other groups, remaining constant or decreasing over time in the MTZ and MK groups. RR was increasing in all animals, with significantly lower mean values (p = 0.042) in the MK group compared to the MTZ group. Details of Tr, HR and RR are reported in Table 2.
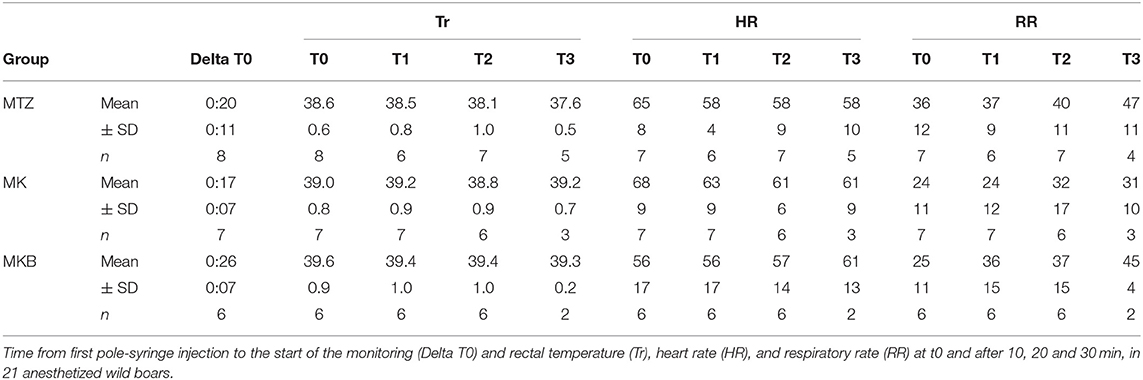
Table 2. Physiological variables of cage-trapped wild boars anesthetized using medetomidine-tiletamine-zolazepam (MTZ; n = 8) or medetomidine-ketamine (MK; n = 7) or medetomidine-ketamine-butorphanol (MKB; n = 6).
Arterial Blood Gas Analysis
Twenty out of 21 anesthetized wild boars were sampled for blood gas, electrolyte, and hematological analysis (8 from the MTZ group and 6 each for the MK and MKB groups). For two of those animals (MK and MKB groups) we were not able to perform an arterial sample within the timeframe. Three individuals (1 from the MTZ group and 2 from the MKB group) were excluded from the blood gas analysis due to artifacts in one of the two arterial samples (i.e., mixed venous/arterial blood sample or prolonged exposure to ambient air). The comparison between the first and the second arterial sample was conducted only for those individuals which received oxygen supplementation. Hypoxemia occurred regardless of the group, although with differences in frequency and extent. All wild boars in the MKB group developed mild hypoxemia, except one individual which developed moderate hypoxemia (69 mmHg). Mild hypoxemia occurred in three out of seven animals in the MTZ group, and in one out of six individuals in the MK group. The alveolar-arterial oxygen tension difference was increased in all groups, with higher values (23 vs. 19 vs. 19 mmHg) in the MKB group. Acidemia and mild hypercapnia were seen in all groups. Lactate was below 6 mmol/L in all wild boars, with no significant differences among the groups. At the time of the second arterial sample, Tr was significantly higher in the MKB group than in MTZ group and K2+ was significantly higher in the MKB group compared to the MTZ and MK groups. Details of the complications occurred during the immobilizations are reported in Table 3.
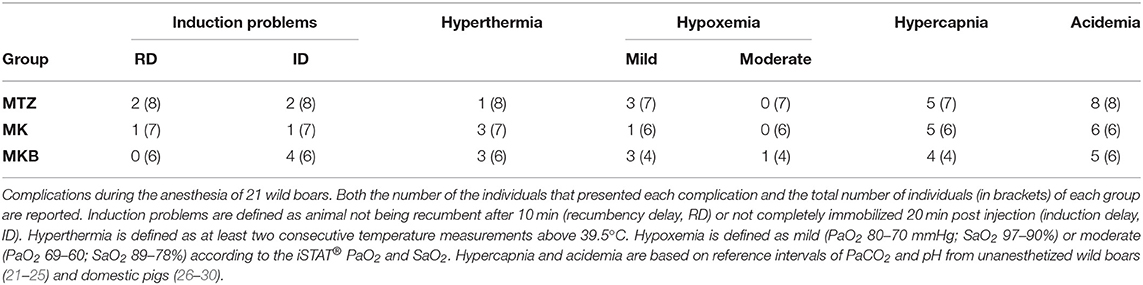
Table 3. Complications during the anesthesia of cage-trapped wild boars anesthetized using medetomidine-tiletamine-zolazepam (MTZ; n = 8) or medetomidine-ketamine (MK; n = 7) or medetomidine-ketamine-butorphanol (MKB; n = 6).
No statistically significant changes in any blood variable in the three groups were identified after the oxygen delivery period. Also, pH, lactate, , and especially PaO2 and SaO2 improved during oxygen supplementation. Hypoxemia and hypercapnia improved by the time of the second sample also in animals that did not receive supplemental oxygen. At the time of the second sample, the mean PaO2 and PaCO2 were 117 and 52 mmHg in animals receiving supplemental oxygen and 99 and 48 mmHg in the ones who did not receive any supplementation. Details of the measured variables related to blood gas analysis, hematology and serum biochemistry are presented in Table 4.
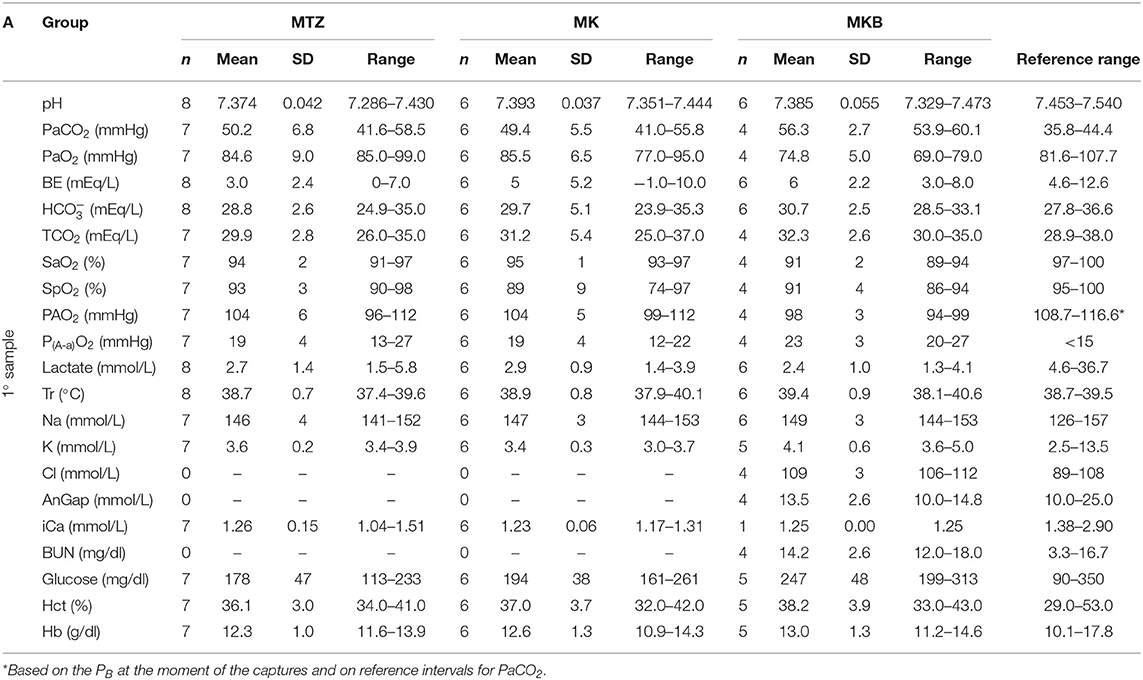
Table 4A. Blood gas, hematological and serum biochemistry variables before oxygen supplementation of cage-trapped wild boars anesthetized using medetomidine-tiletamine-zolazepam (MTZ; n = 8) or medetomidine-ketamine (MK; n = 6) or medetomidine-ketamine-butorphanol (MKB; n = 6).
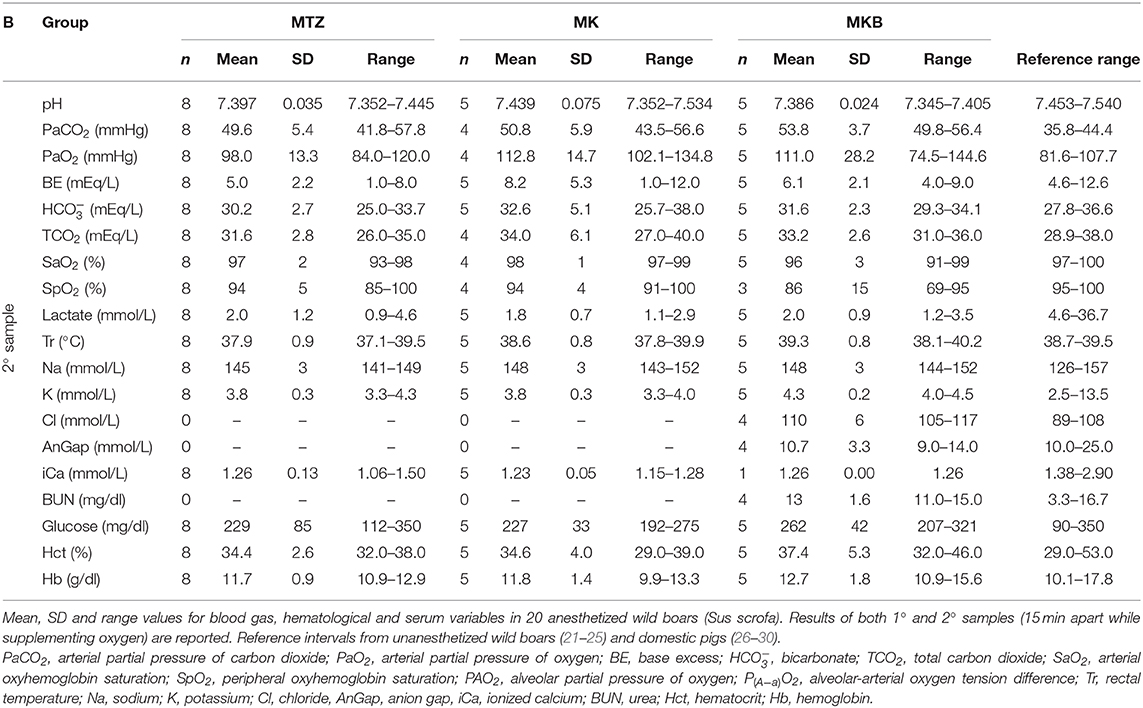
Table 4B. Blood gas, hematological and serum biochemistry variables after oxygen supplementation in 20 anesthetized wild boars.
Discussion
Efficiency and Reliability of the Capture
Short inductions must be pursued when immobilizing wild ungulates, since animals anesthetized with only one injection present a lower risk of developing stress, hyperthermia, tachycardia, and slower recoveries (6). Although the drug protocols evaluated in this study did not result in significantly different induction times, the anesthetic efficiency (percentage of immobilized wild boars with a single injection) was lower than previously reported with the same drug combinations (18, 19, 31). In fact, a second injection was often necessary, particularly in the MKB group. This difference is possibly due to the large volume of the MKB combination (>6 ml), which may have led to delayed absorption of the drugs. Another reason for prolonged induction times could be an incorrect drug placement into the subcutaneous fat, although efficacy and onset between subcutaneous and IM injections were not significantly different in similar studies in domestic pigs (32). Furthermore, studies on wild boars found a direct relationship between the number of animals captured in the same trap and the required drug dose to achieve complete immobilization, due to the high levels of stress of the captured animals (33). Thus, the event of more than one animal caught in the same trap could have led to delayed induction, although it occurred only in three captures.
The majority of the induction problems occurred in MKB and MTZ (when the planned dose was administered) groups, whereas animals from MK group underwent a smoother induction. The hypothesis of an inadequate dose of ketamine and tiletamine-zolazepam leading to induction problems is supported by previous studies in wild boars and domestic pigs in which higher doses were adopted, achieving a relatively rapid induction (4, 18, 34). However, the depth of immobilization for boars injected with ketamine-based protocols was lighter, and a second dose of anesthetic was required after 20–40 min in two cases in each group to carry out further procedures. This reflects the shorter half-life of ketamine compared to tiletamine-zolazepam.
The administration of extra doses of ketamine, especially in the MK group, likely contributed to the difference in recovery time among groups. Nonetheless, the time of achievement of coordination after atipamezole administration was longer than previously reported in all three groups (17, 19). The addition of butorphanol at the present dose might have masked the effects of ketamine in MKB group, consequently reducing the duration of the recovery time compared to MK protocol, as previously documented (18). However, butorphanol did not elicit any clear improvement on the depth of the anesthesia, contrarily to previous findings (4), probably due to the lower dose of medetomidine given in MKB group. In the light of similar studies in suids, we can speculate that quality and reliability of the induction with ketamine-based combinations heavily depends on the ratio between the doses of the dissociative anesthetic and the sedatives (35).
Ataxia and rough, long recoveries occurred after atipamezole administration regardless of the drug protocol. This is consistent with other studies on cyclohexamine anesthesia in this species (1, 4, 7, 34) and it could be attributed to the effects of the active metabolites of ketamine (i.e., norketamine), whose half-life is considerably longer than ketamine in humans (36) and in domestic animals (37). However, the species-specific pharmacokinetics of ketamine and its metabolites is not completely understood in the wild boar yet, leaving such hypothesis at the speculative level. Although tiletamine seems not to be converted in active substances in pigs, its duration of action is more extended than ketamine (38), leading to similar effects. Adding butorphanol to the MK protocol did not clearly improve the quality of the recovery after reversal. The effects of butorphanol on the recovery have been debated in a number of studies on wild boars and domestic pigs (4, 18, 19), with no univocal answer.
It is important to consider that the differences in both age and average body mass among groups may have influenced the variables related to capture and immobilization. In fact the initial dose was increased by 150% in MTZ, since induction problems were noticed. Although neither sex nor age has been confirmed to affect the required doses and induction in wild boars to date, the physiologic responses in this study must be interpreted carefully. Nevertheless, no difference which could be elicited by such bias occurred in the present study.
The pre-release mortality reported here (6.2%) falls within the previously reported 1.6–10.6% range for this species (1, 33), with no fatalities occurring during the anesthesia in the present study.
Physiological Variables
Hyperthermia occurred most frequently in MK and MKB groups. A higher Tr associated with a longer induction time in the MKB group than in the MTZ group is consistent with the findings of a pilot study by one of the authors (Arnemo, unpublished data).
In wild boars and in domestic pigs, marked bradycardia and transient pulmonary and systemic hypertension have been reported when using medetomidine as sedative (8, 9, 19, 34). In the MKB protocol, butorphanol was likely to further contribute to the bradycardia due to its confirmed cardiodepressant properties in mammals (39). Nevertheless, both ketamine and tiletamine elicit an increase in HR when added to the anesthetic protocol, easing such profound bradycardia, as reported in similar studies (4).
The RR was approximately ~50% lower than reported in other studies on wild boars and domestic pigs (8, 19), although similar values are reported in warthogs (Phacochoerus africanus) immobilized with similar combinations (31). Contrarily to what is reported in literature for pigs undergoing ketamine administration (10, 35), no wild boar in the current study developed tachypnea once fully sedated. In fact, boars anesthetized with MK showed a lower RR compared to the other groups. A drop of both Tr and HR, and an increase of RR over time frequently occurs in suids immobilized with the same drug protocols (8, 19, 34).
In this study, we corroborate previous findings (12, 13, 40) where the agreement between SaO2 (iSTAT) and SpO2 (pulse oximeter) was poor, particularly at low values of SpO2 (Figures 1, 2). This could be due to several factors, including species-specific differences and to the use of alpha-2 adrenoceptor agonist drugs (41). Although the SaO2 underestimation at high values that resulted in this study is clinically safer than its overestimation, researchers should also take into account other indicators (i.e., color of mucus membranes and changes in respiratory rate and tidal volume) when assessing hypoxemia in this species. Underestimation at high saturation levels is consistent in brown bears (Ursus arctos) (14), musk oxen (Ovibos moschatus) (13) and white-tailed deer (Odocoileus virginianus) (12) and is associated with overestimation at low levels. Further, although low in accuracy, the pulse oximeter can be reliably used to monitor the trend of SpO2 over time during the immobilization.
Arterial Blood Gas Analysis
Simple acute respiratory acidosis occurred in all three groups in the current study. This is the most common acid-base disorder during anesthesia in spontaneous ventilation (42), with hypercapnia and hypoxemia, usually due to hypoventilation caused by a decrease in respiratory rate and/or tidal volume. This disorder has been reported for all three protocols when used in domestic pigs (4, 34), and it was especially prevalent in boars anesthetized with MKB in the current study. The boars immobilized with MKB also presented higher P(A−a)O2 compared to the other groups. A number of studies on animals immobilized with similar protocols confirm that ventilation-alveolar perfusion mismatch is another remarkable cause of hypoxemia, mainly due to alpha-2-agonist-induced pulmonary vasoconstriction and prolonged recumbency (43, 44), and would be consistent with the increased P(A−a)O2 of the wild boars in this study, regardless of the group. Additionally, the degree of medetomidine-induced increase in pulmonary resistance is considered to be higher in suids than in carnivores (19, 45), and is more severe when butorphanol is added to the combination (17, 46). The mild non-significant differences in the current study among protocol groups, are consistent with previous findings in pigs anesthetized with MKB, as this protocol is reported to elicit a remarkable depressant effect on blood gas and acid-base status (5, 19) compared to MTZ (18) or MK (4). The hypoxia detected in the wild boars at the time of the first arterial blood sample was only mild (and moderate in one individual), milder than in several other species undergoing anesthesia in the field, although different drug protocols and capture methods were used (14). In addition to this, the supplemental oxygen flow was deliberately very conservative considering the size of the immobilized animals (47) due to the mild grade of the hypoxemia encountered. A more pronounced increase of PaO2 (~172 mmHg) is reported in anesthetized pigs in spontaneous ventilation with a FiO2 of 0.5 and an intratracheal oxygen flow of 30–50 ml/kg/min (42). Being the oxygen flow not intratracheal and only about 5–20 ml/kg/min in this study, it might have not produced a clinically significant increase in PAO2. The cardiopulmonary effects of prolonged lateral recumbency, the presence of right-to-left intrapulmonary shunting and diffusion impairment could not be determined in this study. All these factors might have contributed to the fact that no significant change in PaO2 nor SaO2 were detected following oxygen supplementation.
Although the wild boars did not experience hypoxemia of clinically concerning levels in any group, oxygen supplementation resolved the mild to moderate hypoxemia in all wild boars in the present study, hence we recommend it for all three combinations assessed here. Oxygen has been previously recommended for the MKB protocol (5, 17). Although most animals were still acidemic after oxygen supplementation, the pH slightly improved in the MTZ and to a higher extent in MK group despite the significantly lower RR and the sustained hypercapnia. This might be explained by the marked decrease in lactate over time in the wild boars anesthetized with MK.
Both the respiratory acidosis and the hypercapnia occurred following the administration of the drug combinations in this study were mild and of limited clinical impact compared to other studies in anesthetized wildlife (12–14). This reflects the safety of such capture and drug combinations in regards to the respiratory and metabolic status in wild boars. In spontaneous ventilation circumstances, hypercapnia, and thus respiratory acidosis, resolves only following a change in minute ventilation (RR and/or tidal volume). The fact that the average levels of PaCO2 did not change significantly over time indirectly suggests that minute ventilation is likely to remain stable in animals immobilized with these drug combinations.
Bicarbonates and BE increased slightly over time, this is perfectly predictable as the metabolic compensation usually begins within 10 min from the start of the acute respiratory acidosis (19, 48).
Limits of the Research
The exiguous number of the animals anesthetized with the MTZ, the MK and the MKB drug combinations leads to significant statistical limitations in this study, risking not to detect significant differences in all measured variables. Extending the research to a larger sample and including a larger control sample of wild boars not receiving supplemental oxygen would result in a more reliable interpretation and a more solid inference to a wider population. Additionally, the results in this study are highly dependent of the doses of the anesthetic combinations used, therefore they should be interpreted carefully as different dose ratios of the drug combinations may have resulted in different outcomes.
Wildlife capture inevitably leads to heterogeneity in regards to age, body mass, sex and number of individuals in each group. A more standardized study sample would decrease the risks of statistical bias. The implementation of a standard scoring system to assess the anesthetic depth would have increased rigor and statistical relevance, helping to draw more objective conclusions on the quality of the anesthesia.
Although portable analyzers such as i-STAT® are the most accurate instrument available in field settings to measure blood gases, they present some limitations compared to benchtop analyzers that are considered to be the gold-standard. Further, i-STAT® calculates SaO2 following algorithms based on a pre-set oxyhemoglobin saturation curve, which is species-specific. Hence a validation of the accuracy of i-STAT® for blood gas variables in wild boars would be recommendable.
As the bar graph on the pulse oximeter display evaluating the pulse amplitude is assessed subjectively by the reader, further inaccuracy due to anomalies in the probe-oxyhemoglobin interface (e.g., unsuitable probe site, medetomidine-induced vasoconstriction, movements, etc.) could not be always ruled out.
Conclusions
Evaluating both advantages and disadvantages of the three anesthetic protocols, we have demonstrated that ketamine-based protocols were reliable to safely immobilize wild boars to carry out short procedures. The choice of the drug protocol depends on the purpose and the expected duration of the procedures, making MK and MKB combinations effective and reliable for procedures shorter than 40 min. After that time, a supplemental dose of ketamine may be required. Regarding the choice between these two protocols, it is important to consider their impact on induction time, arterial blood gases, pH and recovery time. Despite all three protocols combined with the capture method in this study elicited minimal impact on the respiratory function, we strongly recommend the use of supplemental oxygen to prevent and treat hypoxemia in wild boars, especially if the MKB protocol is used at the reported doses. Ataxia and rough recoveries seem to be a common occurrence in wild boar anesthesia, regardless of the drug protocol adopted. In case of longer procedures, MTZ combination at the increased dose would be preferred for wild boar anesthesia, due to its milder cardiopulmonary and hematological effects.
The pulse-oximeter readings did not correlate with the arterial oxyhemoglobin saturation measured with iSTAT®, underestimating the real values in most cases and to a higher extent at higher readings. Further studies are recommended on the species-specific hemoglobin dissociation curve and the accuracy of pulse-oximeters in wild boars immobilized with similar protocols. Due to the limitations of the present study, further research with a higher number of wild boars anesthetized with the same drug combinations is needed to corroborate the findings and to extend the conclusion to the population level. Additional research should assess whether the combination of the same drugs, but with different doses, would elicit a lower incidence of induction problems and prevent the requirement for an additional anesthetic dose.
Data Availability Statement
The original contributions presented in the study are included in the article/supplementary material, further inquiries can be directed to the corresponding author/s.
Ethics Statement
The animal study was reviewed and approved by SR participated to a training course provided by the French National Museum of Natural History (Muséum National d'Histoire Naturelle, MNHN) and the French National Centre for Scientific Research (Centre National de la Recherche Scientifique, CNRS), in collaboration with the French Office for Biodiversity (Office Français pour la Biodiversité, OFB). At the end of the course, SR passed successfully the exam and was awarded an official permit for capture, anesthesia, handling, sample collection and any other use of the animals for scientific purposes.
Author Contributions
The study was conceived and designed by SR, JA, and AE. SR planned, organized and led the captures. SR, BF, AE, SK, and DB carried out the field sampling and data collection. Data was prepared by BF, AE, DB, and JM. JM analyzed the data and drafted the manuscript. SR and JM contributed to interpretation of the results. All authors contributed to the article and approved the submitted version.
Funding
The capture of wild boars was funded by the François Sommer Foundation, the National Hunting and wildlife Agency (now French Agency for Biodiversity) and the Chambord National Estate (agreement n°2014/DER/03). The arterial blood gas sampling and analysis was funded by the Ecophysiology Research Group, Inland Norway University of Applied Sciences. The first author was funded by a postgraduate scholarship from ERASMUS plus.
Conflict of Interest
The authors declare that the research was conducted in the absence of any commercial or financial relationships that could be construed as a potential conflict of interest.
Acknowledgments
The authors wish to thank the National Hunting and Wildlife Agency (Office National de Chasse et de la Faune Sauvage, ONCFS) in agreement with Domaine National de Chambord and François Sommer Foundation, the veterinarians, biologists, technicians, park rangers, and students who participated in the field work.
Abbreviations
MTZ, medetomidine-tiletamine-zolazepam; MK, medetomidine-ketamine; MKB, medetomidine-ketamine-butorphanol; Tr, rectal temperature; HR, heart rate; SpO2, peripheral oxyhemoglobin saturation (pulse-oximeter); RR, respiratory rate; PaO2, arterial partial pressure of oxygen; PaCO2, arterial partial pressure of carbon dioxide; SaO2, arterial oxyhemoglobin saturation (iSTAT®); LoA, Limit of Agreement; CAL, Clinically Acceptable Limit.
References
1. Fournier P, Fournier-Chambrillon C, Maillard D, Klein F. Zoletil immobilization of wild boar (Sus scrofa L.). Ibex J Mt Ecol. (1995) 3:134–6.
2. Jakobsen K, Thorbekt G. The respiratory quotient in relation to fat deposition in fattening-growing pigs. Br J Nutr. (1993) 69:333–43. doi: 10.1079/BJN19930037
3. Tranquilli WJ, Grimm KA. Introduction: use, definitions, history, concepts, classification, and considerations for anesthesia and analgesia. In: Veterinary Anesthesia and Analgesia. 5th ed. Ames: Lumb & Jones (2015). pp. 1-10.
4. Nishimura R, Sakaguchi M, Sasaki N, Tamura H, Takeuchi A. Medetomidine-ketamine and medetomidine-butorphanol-ketamine anaesthesia in pigs. J Vet Anaesth. (1991) 18:177–9. doi: 10.1111/j.1467-2995.1991.tb00540.x
5. Lehmann HS, Blache D, Drynan E, Tshewang P, Blignaut DJC, Musk GC. Optimum drug combinations for the sedation of growing boars prior to castration. Animals. (2017) 7:61. doi: 10.3390/ani7080061
6. Sweitzer RA, Gonzales BJ, Gardner I, Van Vuren D, Waithman JD, Boyce WM. A modified panel trap and immobilization technique for capturing multiple wild pig. Wildl Soc Bull. (1997) 25:699–705.
7. Heinonen ML, Raekallio MR, Oliviero C, Ahokas S, Peltoniemi OAT. Comparison of azaperone-detomidine-butorphanol-ketamine and azaperone-tiletamine-zolazepam for anaesthesia in piglets. Vet Anaesth Analg. (2009) 36:151–7. doi: 10.1111/j.1467-2995.2008.00443.x
8. Barasona JA, López-Olvera JR, Beltrán-Beck B, Gortázar C, Vicente J. Trap-effectiveness and response to tiletamine-zolazepam and medetomidine anaesthesia in Eurasian wild boar captured with cage and corral traps. BMC Vet Res. (2013) 9:107. doi: 10.1186/1746-6148-9-107
9. Ugarte CE, O'Flaherty K. The use of a medetomidine, butorphanol and atropine combination to enable blood sampling in young pigs. N Z Vet J. (2005) 53:249–52. doi: 10.1080/00480169.2005.36554
10. Green CJ, Knight J, Precious S, Simpkin S. Ketamine alone and combined with diazepam or xylazine in laboratory animals: a 10 year experience. Lab Anim. (1981) 15:163–70. doi: 10.1258/002367781780959107
11. Hurst RJ, Oritsland NA, Watts PD. Body mass, temperature and cost of walking in polar bears. Acta Physiol Scand. (1982) 115:391–5. doi: 10.1111/j.1748-1716.1982.tb07096.x
12. Mich PM, Wolfe LL, Sirochman TM, Sirochman MA, Davis TR, Lance WR, et al. Evaluation of intramuscular butorphanol, azaperone, and medetomidine and nasal oxygen insufflation for the chemical immobilization of white-tailed deer, Odocoileus virginianus. J Zoo Wildl Med. (2008) 39:480–7. doi: 10.1638/2007-0150.1
13. Lian M, Björck S, Arnemo JM, Esteruelas NF, Angel M, Minsaas SC, et al. Severe hypoxemia in muskoxen (Ovibos moschatus) immobilized with etorphine and xylazine corrected with supplemental nasal oxygen. J Wildl Dis. (2017) 53:356–60. doi: 10.7589/2016-04-085
14. Fahlman Å, Caulkett NA, Arnemo JM, Neuhaus P, Ruckstuhl KE. Efficacy of a portable oxygen concentrator with pulsed delivery for treatment of hypoxemia during anesthesia of wildlife. J Zoo Wildl Med. (2012) 43:67–76. doi: 10.1638/2011-0064.1
15. Busl KM, Greer DM. Hypoxic-ischemic brain injury: pathophysiology, neuropathology and mechanisms. NeuroRehabilitation. (2010) 26:5–13. doi: 10.3233/NRE-2010-0531
16. Chambord Weather. (2021). Available online at: https://www.worldweatheronline.com/ (accessed September 20, 2020).
17. Arnemo JM. Chemical Immobilization and Anaesthesia of Wild Boars. DDA News, Orion Pharma Animal Health, Turku (2004).
18. Enqvist K. Medetomidine/tiletamine-zolazepam and medetomidine/butorphanol/tiletamine-zolazepam: a comparison of two anesthetic regimens for surgical implantation of intraperitoneal radiotransmitters in free ranging juvenile European wild boar (Sus scrofa scrofa). in Proceedings of the Annual Conference-American Association of Zoo Veterinarians, 17th-21st September. New Orleans; American Association of Zoo Veterinarians. American Association of Zoo Veterinarians Annual Proceedings (2000). p. 303–06
19. Sakaguchi M, Nishimura R, Sasaki N, Ishiguro T, Tamura H, Takeuchi A. Anesthesia induced in pigs by use of a combination of medetomidine, butorphanol, and ketamine and its reversal by administration of atipamezole. Am J Vet Res. (1996) 57:529–34.
20. R-3.5.3. Core Team. R: A Language and Environment for Statistical Computing. R Foundation for Statistical Computing. (2019) Vienna. URL. Available online at: https://www.R-project.org/ (accessed June 05, 2020).
21. Harapin I, Bedrica L, HahnI V, Graèner D. Haematological and biochemical values in blood of wild boar (Sus scrofa ferus). Vet Arh. (2003) 73:333–43.
22. Casas-Dìaz E, Closa-Sebastià F, Marco I, Lavìn S, Bach-Raich E, Cuenca R. Hematologic and biochemical reference intervals for wild boar (Sus scrofa) captured by cage trap. Vet Clin Pathol. (2015) 44:215–22. doi: 10.1111/vcp.12250
23. Desjardins Bergonso TH, Chiacchio SB, Gonçalves RC, Takahira RK, Laurenti Ferreira DO, Rotter Curotto SM, et al. Reference values of the urea and creatinine in wild boar (sus scrofa scrofa, linnaeus, 1758) raised in captivity. Arch Vet Sci. (2010) 15:190–5. doi: 10.5380/avs.v15i4.17265
24. Vidal D, Naranjo V, Mateo R, Gortazar C, De la Fuente J. Analysis of serum biochemical parameters in relation to mycobacterium bovis infection of European wild boars (Sus scrofa) in Spain. Eur J Wildl Res. (2006) 52:301–4. doi: 10.1007/s10344-006-0062-2
25. Shender LA, Botzler RG, George TL. Analysis of serum and whole blood values in relation to helminth and ectoparasite infections of feral pigs in texas. J Wildl Dis. (2002) 38:385–94. doi: 10.7589/0090-3558-38.2.385
26. Department of Veterinary Pathology ISU. Reference Intervals from Clinical Pathology Laboratory. (2011). Available online at: https://www.vetmed.iastate.edu/vpath/services/diagnostic-services/clinical-pathology/testing-and-fees/reference-intervals (accessed September 07, 2020).
27. Cabral Gianotti G, Krüger Beheregaray W, Passos Bianchi S, Santos Mombach V, Bonfim Carregaro A, Contesini EA. Suíno como modelo experimental na pesquisa biomédica: valores fisiológicos normais. Acta Sci Vet. (2009) 38:133–7. doi: 10.22456/1679-9216.16610
28. Buzzard BL, Edwards-Callaway LN, Engle TE, Rozell TG, Dritz SS. Evaluation of blood parameters as an early assessment of health status in nursery pigs. J Swine Health Prod. (2013) 21:148–51.
29. Reece WO. Chapter 14: Body temperature and its regulation. In: Reece WO, editor. Duke's Physiology of Domestic Animals. 13th ed. Ames: Wiley-Blackwell (2015), p. 150.
30. Jackson PGG, Cockcroft PD. Appendix 3, Laboratory Reference Values: Biochemistry in Clinical Examination of Farm Animals. Osney Mead: Blackwell Science Ltd (2002). pp. 303–5.
31. Hewlett J, Buss P, Olea-Popelka F, Koeppel K, Neiffer D, Hausler G, et al. Evaluation of a partially reversible immobilization protocol using medetomidine, butorphanol, zolazepam-tiletamine, and ketamine in free-ranging warthogs (Phacochoerus africanus) in Kruger National Park, South Africa. J Zoo Wildl Med. (2020) 17:80–7. doi: 10.1638/2019-0110
32. Swindle MM, Smith CA. Swine in the Laboratory. Surgery, Anesthesia, Imaging, and Experimental Techniques. 3rd ed. Boca Raton: CRC Press (2016).
33. Fenati M, Monaco A, Guberti V. Efficiency and safety of xylazine and tiletamine/zolazepam to immobilize captured wild boars (Sus scrofa L. 1758): analysis of field results. Eur J Wildl Res. (2008) 54:269–74. doi: 10.1007/s10344-007-0140-0
34. Lee JY, Jee HC, Jeong SM, Park CS, Kim MC. Comparison of anaesthetic and cardiorespiratory effects of xylazine or medetomidine in combination with tiletamine/zolazepam in pigs. Vet Rec. (2010) 167:245–9. doi: 10.1136/vr.c3611
35. Santos M, Bertràn de Lis BT, Tendillo FJ. Effects of intramuscular dexmedetomidine in combination with ketamine or alfaxalone in swine. Vet Anaesth Analg. (2016) 43:81–5. doi: 10.1111/vaa.12259
36. Zanos P, Moaddel R, Morris PJ, Riggs LM, Highland JN, Georgiou P, et al. Ketamine and ketamine metabolite pharmacology: insights into therapeutic mechanisms. Pharmacol Rev. (2018) 70:621–60. doi: 10.1124/pr.117.015198
37. Berry SH. Injectable anesthetics. In: Veterinary Anesthesia and Analgesia. 5th ed. Ames: Lumb & Jones (2015). pp. 277–96.
38. Kumar A, Mann HJ, Remmel RP. Pharmacokinetics of tiletamine and zolazepam (Telazol) in anesthetized pigs. J Vet Pharmacol Ther. (2006) 29:587–9. doi: 10.1111/j.1365-2885.2006.00798.x
39. KuKanich B, Wiese AJ. Opioids. In: Veterinary Anesthesia and Analgesia. 5th ed. Ames: Lumb & Jones (2015). pp. 207–26.
40. Schmitt PM, Göbel T, Trautvetter E. Evaluation of pulse oximetry as a monitoring method in avian anesthesia. J Avian Med Surg. (1998) 12:91–9.
42. Biteli EGF, Nunes N, Lopes PCF, Silva PES, Ido CK, Silva HRA, et al. Blood gas analysis in pigs submitted to different concentrations of nitrous oxide or oxygen, under different ventilatory modalities. Arq Bras Med Vet Zootec. (2019) 71:35–43. doi: 10.1590/1678-4162-10210
43. Fahlman Å, Arnemo JM, Swenson JE, Pringle J, Brunberg S, Nyman G. Physiologic evaluation of capture and anesthesia with medetomidine-zolazepam-tiletamine in brown bears (Ursus arctos). J Zoo Wildl Med. (2011) 42:1–11. doi: 10.1638/2008-0117.1
44. Fahlman Å, Pringle J, Arnemo JM, Swenson JE, Brunberg S, Nyman G. Treatment of hypoxemia during anesthesia of brown bears (Ursus arctos). J Zoo Wildl Med. (2010) 41:161–4. doi: 10.1638/2009-0036.1
45. Hayashi K, Nishimura R, Yamaki A, Kim HY, Matsunaga S, Sasaki N, et al. Cardiopulmonary effects of medetomidine, medetomidine-midazolam and medetomidine-midazolam-atipamezole in dogs. J Vet Med Sci. (1995) 57:99–104. doi: 10.1292/jvms.57.99
46. Sakaguchi M, Nishimura R, Sasaki N, Ishiguro T, Tamura H, Takeuchi A. Cardiopulmonary effects of a combination of medetomidine and butorphanol in atropinized pigs. J Vet Med Sci. (1993) 55:497–9. doi: 10.1292/jvms.55.497
Keywords: butorphanol, blood gas, oxygen, anesthesia, capture, medetomidine, Sus scrofa, wild boar (Sus scrofa L.)
Citation: Morelli J, Rossi S, Fuchs B, Richard E, Barros DSB, Küker S, Arnemo JM and Evans AL (2021) Evaluation of Three Medetomidine-Based Anesthetic Protocols in Free-Ranging Wild Boars (Sus scrofa). Front. Vet. Sci. 8:655345. doi: 10.3389/fvets.2021.655345
Received: 18 January 2021; Accepted: 03 March 2021;
Published: 29 March 2021.
Edited by:
Michele Ann Miller, Stellenbosch University, South AfricaReviewed by:
Leith Carl Rodney Meyer, University of Pretoria, South AfricaGareth Edward Zeiler, University of Pretoria, South Africa
Copyright © 2021 Morelli, Rossi, Fuchs, Richard, Barros, Küker, Arnemo and Evans. This is an open-access article distributed under the terms of the Creative Commons Attribution License (CC BY). The use, distribution or reproduction in other forums is permitted, provided the original author(s) and the copyright owner(s) are credited and that the original publication in this journal is cited, in accordance with accepted academic practice. No use, distribution or reproduction is permitted which does not comply with these terms.
*Correspondence: Jacopo Morelli, jacmorelli@gmail.com