- 1Centro de Biotecnología Genómica, Instituto Politécnico Nacional, Reynosa, Mexico
- 2Facultad de Medicina Veterinaria y Zootecnia, Universidad Autónoma de Tamaulipas, Ciudad Victoria, Mexico
In this work, the antimicrobial resistance profile of Escherichia coli strains (n = 248) isolated from bovine feces and carcass samples from Tamaulipas, Mexico, was evaluated. Susceptibility to 12 antibiotics conventionally used in human and veterinary treatments was determined according to Clinical and Laboratory Standards Institute guidelines. Genes encoding resistance to tetracycline (tetA and tetB), streptomycin (strA), aminoglycoside (aadA), and β-lactamase (blaTEM and blaSHV) were investigated by PCR. Also, stx1, stx2, eae, bfp, and hlyA encoding virulence factors were determined. Of the isolates, 85.9% were confirmed as E. coli strains. Among the 213 E. coli isolates tested, 94.8% (202/213) showed resistance for at least one antimicrobial, mainly ampicillin (83.0%; 177/213), cephalothin (76.0%; 162/213), and tetracyclines (69.0%; 147/213). In all the other antibiotics tested, the resistance percentage was below 36%. A multidrug-resistant phenotype was found in 72.7% of the tested strains. The presence of the tet gene (tetA or tetB) was detected in 43.1% of the isolates, the strA gene in 17.3%, and aadA1 in 51.6%. The blaTEM and blaSHV genes were found in 10.3 and 0.4% of the isolates, respectively. stx1 was detected in 4.2% of isolates, stx2 in 7.0, and hlyA in 2.8%. The virulence genes, eae and bfp, were not detected in any strain. These results indicate that Tamaulipas food products of bovine origin can be a source of multiresistant E. coli strains for the environment and exposure for consumers.
Introduction
Antimicrobial resistance (AMR) is a complex multifactorial process driven by numerous and varied factors (1). A causal relationship has been demonstrated with the increased use of antimicrobials in veterinary medicine for treating and preventing disease and for growth promotion (2). Antibiotic-resistant bacteria can be transferred directly from animals to humans and can spread to the soil, food, and groundwater by the application of manure to agricultural fields (3–5). The emergence of AMR in food-producing animals is of concern since these zoonotic bacteria pose a risk to human health via the food chain (6, 7). Resistant proportions in the sentinel organism Escherichia coli are often referred to as “prevalence” of resistance (8). E. coli is known as a dangerous pathogen to human health. It contributes to the dissemination of AMR, and it is a good indicator of transmission pathways because it is ubiquitous, shares the same niche as other enteric pathogens, and can be transferred by the same route (7, 9, 10). E. coli strains can colonize cattle at any age (11), and bovine carriers are often asymptomatic. Consequently, these enteric pathogens, when shed in the feces, are a source of contamination of water and food products (12). Analysis of antibiotic resistance in microbial isolates from agricultural and other environments could help us recognize resistance gene reservoirs that may be present in bacterial populations associated with food production animals (13). Monitoring of AMR in E. coli may provide information on antimicrobial abuse and the dynamics of transmission and development of AMR pathogens. Only a few reports from México about the AMR profiles of E. coli isolates in food-producing animals are available. Amezquita et al. (14), in northwestern Mexico, examined the AMR profiles of Shiga toxin-producing E. coli (STEC) O157 and non-O157 from cattle, sheep, and poultry. Multidrug resistance profiles were identified in 42% of the non-susceptible STEC strains. However, in northeast Mexico, no information has been generated regarding the possible multidrug resistance in cattle (feces or carcasses) and its potential risk for human health. The objective of this work was to investigate the AMR profile in E. coli isolated from cattle in northeast Mexico.
Materials and Methods
Sample Collection
Samples were collected from 124 cattle from a bovine slaughterhouse located in the center of the state of Tamaulipas, Mexico. This slaughterhouse receives cattle from nearby municipalities. One specimen was randomly selected per farm for the fecal sample and the carcass sample later to be taken.
Fecal Samples
A total of 124 bovine fecal samples were collected by direct rectal retrieval using disposable gloves. Each glove was inverted and sealed immediately after collection.
Carcass Samples
A total of 124 carcass samples were collected by sterile sponge from each carcass 12 h after evisceration: a minimum of 100 cm2 from inside the round area from the navel–plate–brisket–foreshank areas. All carcass samples were collected according to the national regulations described in NOM-SSA1-1994 (15). All samples were placed in separate sterile plastic bags to prevent cross-contamination, labeled, and transported in ice to the laboratory.
Isolation and Identification of E. coli
Twenty-five grams of each sample was enriched by inoculation in 225 ml of peptone water. They were then homogenized and incubated at 37°C. After 24 h, the samples were plated onto eosin methylene blue and were incubated at 37°C for 18–24 h. Six colonies of presumptive E. coli strains were randomly picked from each sample, and each was subsequently transferred separately to tryptic soy agar and incubated for 24 h at 37°C to obtain a pure culture. All suspect colonies were confirmed by biochemical identification, including the sugar fermentation test, the indole production test, and the citrate test (16).
Antimicrobial Susceptibility Testing
Antimicrobial susceptibility was tested by the agar disc diffusion method, following the Clinical and Laboratory Standards Institute guidelines (17). The antimicrobials used were tetracycline (TE; 30 μg), ampicillin (AM; 10 μg), levofloxacin (LEV; 5 μg), cephalothin (CF; 30 μg), cefotaxime (30 μg), gentamicin (GE; 10 μg), cefepime (30 μg), trimethoprim/sulfamethoxazole (25 μg), netilmicin (NET, 30 μg), amikacin (30 μg), ceftriaxone (30 μg), and streptomycin (STR, 30 μg) (Sensi-disc BBL). These antimicrobials represent the major classes of antimicrobial drugs relevant in both veterinary and human medicine. Each E. coli strain colony was evaluated based on the diameter of the clear inhibition zone, and the results were interpreted following the criteria provided by the CLSI. E. coli ATCC 25922 was used as a control.
Antibiotic-Resistant Gene Detection
Bacterial DNA was obtained from a pure culture on tryptic soy agar (BD Becton Dickinson & Co., Cuautitlán Izcalli, Mexico) by lysis of a bacterial cell suspension at 95°C for 15 min, followed by centrifugation at 13,000 × g for 3 min. Six antibiotic resistance genes were screened by PCR: genes encoding tetracycline efflux pump (tetA and tetB), streptomycin phosphotransferases (strA), aminoglycoside adenyltransferase (aadA), and beta-lactamase resistance (blaTEM and blaSHV) (18–20). PCR conditions were performed in a total volume of 25 μl containing 1× buffer, 25 mM MgCl2, 10 mM dNTPs (Bioline), 10 mM of each primer, 5 U of Taq polymerase (Uniparts), and sterile water. PCR amplification was conducted following these conditions: initial denaturation at 95°C for 7 min, followed by 30 cycles of denaturation at 95°C for 45 s, annealing at 42 at 59°C for 45 s, and extension at 72°C for 45 s and a final cycle of amplification at 72°C for 7 min. The PCR products were analyzed by gel electrophoresis in 2.5% agarose (Sigma Aldrich, Germany), followed by visualization on a transilluminator.
Detection of Virulence Factors
The detection of virulence factor genes was performed according to the method described by Canizalez et al. (21). PCR was carried out using target gene-specific primers, including shiga toxin (stx1 and stx2), intimin (eaeA), bundle-forming pilus (bfp), and enterohemolysin A (hlyA). The PCR reaction mixture contained buffer 1X, MgCl2 25 mM, dNTPs 10 mM, primer 10 mM, and Taq DNA polymerase 5 U. The volume of this mix was adjusted to 25 μl with sterile water. The PCR amplification conditions were as follows: initial denaturation at 95°C for 1 min, followed by 30 cycles of denaturation at 95°C for 45 s, annealing at 54–60°C for 45 s, and extension at 72°C for 45 s and a final cycle of amplification at 72°C for 7 min. Verification of PCR products was performed in horizontal electrophoresis using 2.5% agarose gel with 0.5× TBE and Sybr gold at 100 V for 45 min. A molecular marker was run concurrently (100 pb Promega). The negative control consisted of all contents of the reaction mixture excluding template DNA, which was substituted with 1 μl sterile water. The DNA bands were visualized and photographed under UV light.
Results
Isolation and Identification of E. coli
Out of 248 samples tested, E. coli strains were detected in 85.9% (213/248); 87.9% (109/124) were confirmed as E. coli strains in fecal samples, and 83.8% (104/124) were from carcass samples (Table 1).
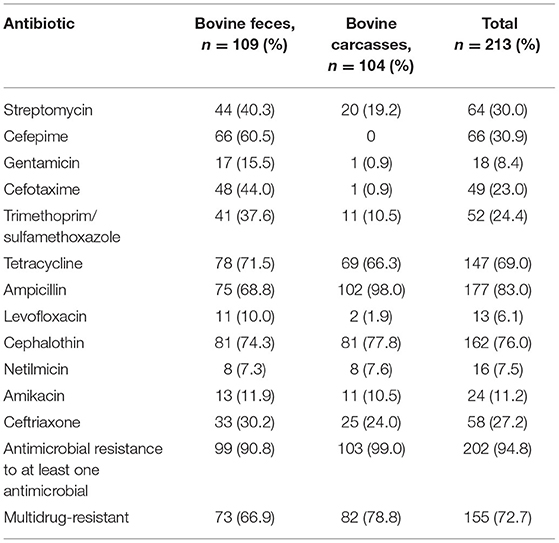
Table 1. Prevalence of phenotypical antimicrobial resistance in E. coli recovered from bovine feces and carcasses.
Antimicrobial Susceptibility Testing
Among the 213 E. coli isolates tested, 94.8% (202/213) showed resistance for at least one antimicrobial, mainly AM (83.0%; 177/213), CF (76.0%; 162/213), and TE (69.0%; 147/213) (Table 1). The percentage of resistance to the other antimicrobial agents was in all cases below 36%. The results showed resistance to AM in 68.8% of fecal strains (75/109) and 98.0% of carcass strains (102/104). Phenotypic resistance to CF E. coli was confirmed in 74.3% of fecal isolates (81/109) and 77.8% of carcass isolates (81/104). The isolates that showed resistance to TE were 71.5% of fecal strains (78/109) and 66.3% of carcass strains (69/104). The percentage of sensitivity to LEV (90.6%; 193/213), GE (85.9%; 183/213), and NET (80.7%; 172/213) shown in this work was higher than 80%. Fifty-seven antibiogram resistance patterns were detected in this study (46 fecal and 11 carcasses; Table 2). The detection of 72.7% (155/213) strains which showed a MDR phenotype (104 fecal and 82 carcasses) is remarkable.
Antibiotic-Resistant Gene Detection
Of the isolates, 19.2% (41/213) were negative for all the resistant genes tested. The tet(A) and tet(B) genes were detected in 43.1% of isolates (92/213; 35 fecal and 57 carcasses). Both tet genes were detected in 12.6% (27/213) of isolates (17 fecal and 10 carcasses). The strA and aadA1 genes were detected in 17.3% (37/213; eight fecal and 29 carcasses) and 51.6% (110/213; six fecal and 104 carcasses) of isolates, respectively. β-Lactamic genes were detected in 10.3% (22/213; 18 fecal and four carcasses) from blaTEM and in 0.4% (1/213; one fecal and zero carcasses) from blaSHV (Table 3).
Detection of Virulence Factors
Of the isolates, 14.0% (30/213) showed one or two genes encoding a virulence factor (stx1, stx2, or hlyA). The stx genes were carried by 11.2% (24/213; 12 fecal and 12 carcasses) of the studied isolates. From these stx positives, 4.2% (9/213; three fecal and six carcasses) were stx1, and 7.0% (15/213; nine fecal and six carcasses) were stx2. Only three strains showed both genes stx1 and stx2 (1.4%; 3/213). However, hlyA was detected in only 2.8% (6/213; four fecal and two carcasses). None of the isolates contained the virulence genes eaeA and bfp.
Discussion
Previous studies show that antimicrobial resistance varies widely depending on the countries or animal from which the microorganisms have been isolated. In this study, E. coli strains with resistance to AM (83.0%), CF (76.0%), and TE (69.0%) were the most prevalent. Overall, other studies with E. coli strains from bovine samples showed a range of antimicrobial resistance to AM between 6.0 and 95.7% and to TE between 10.6 and 95% (8, 22–26). Globally, TE and AM are widely used in animal production, disease prevention, and growth promotion. Their combined resistance is often due to the colocation of different determinants in the same mobile genetic elements (plasmids, transposons, and/or integrons), and this has contributed to the selection of multidrug-resistant isolates worldwide (23). Fifty-seven antibiogram patterns of resistance were detected in this study (46 fecal and 11 carcasses) (Table 2). In the current study, an overall prevalence of MDR among the isolates (72.7%; Table 1) was high compared to previous studies worldwide, for example, an MDR of 44.4% in Egypt (27), 69.0% in Portugal (28), 56.0% in France (29), 13.7% in South Africa (30), and 37.1% in Jordan (24). In Mexico, an AMR study in the northwest reported an MDR of 42% (14). This might be due to inappropriate or excessive use of antibiotics for therapeutic and prophylactic treatment (31, 32). These results suggest that bovines may represent an important reservoir of antibiotic-resistant bacteria, be a route of spread, and represent a health risk. Of the 147 E. coli strains with phenotypic resistance to TE, 38.0% (56/147) showed the gene tetA and 24.4% (36/147) the gene tetB. While the tetA gene had a similar prevalence in both types of samples (fecal, 27/109 and carcasses, 29/104) for the tetB gene, the prevalence was markedly higher in carcass samples compared to that in fecal samples (fecal, 8/109 and carcasses, 28/104). Of the 64 strains with phenotypic resistance to STR, 57.8% (37/64) have the resistance gene strA and 100% (64/64) the resistance gene aadA-IV. The 46 strains identified with the aadA-IV gene did not show phenotypic resistance to STR (Table 3). These resistance genes may be transferable from E. coli to other bacteria in the gut and subsequently disseminated into the environment. The virulence factors of E. coli strains were frequently associated with MDR. In the association between antimicrobial resistance and virulence factors, the most remarkable was resistance to AM, CF, TE, and STR in all strains with stx1 virulence factors (9/9); 100% (15/15) of strains stx2 showed resistance to at least one antimicrobial. Of these, 40% (6/15) showed resistance to four. One strain harboring hlyA was not resistant to any antibiotic tested (6.6%; 1/15), and the rest of the hlyA-harboring strains had antimicrobial resistance to two or more antibiotics (93.3%; 14/15). E. coli harboring stx and eae genes might represent a high zoonotic risk, as these genes are often found in human pathogenic enterohemorrhagic E. coli strains (26, 33, 34). In this study, the stx genes and the hlyA gene were present in our isolates, whereas aea and bfp were not detected. The gene stx2 codes for a potent toxin, often associated with the development of hemorrhagic colitis and hemolytic uremic syndrome in humans and was frequently associated with more severe human diseases than stx1 (26, 35).
Conclusions
In this study, we detected a high prevalence of E. coli strains with resistance (82.0%) and MDR (72.7%) with AM, CF, and TE being the least effective antibiotics. Although the prevalence of virulence factors was low, the strains harboring the virulence factors were frequently associated with MDR. These results suggest that bovines may represent an important reservoir of antibiotic-resistant bacteria and show the need to enforce the regulations in the use of antimicrobials in food-producing animals.
Data Availability Statement
The raw data supporting the conclusions of this article will be made available by the authors, without undue reservation.
Author Contributions
AM-V and JV-V participated in samples processing and laboratory analysis. LL-Z participated in samples acquisition and processing. HB-G and GR participated in data analysis and first draft. VB-G participated in study design and revised and approved the final manuscript. All authors contributed to the article and approved the submitted version.
Conflict of Interest
The authors declare that the research was conducted in the absence of any commercial or financial relationships that could be construed as a potential conflict of interest.
Acknowledgments
AM-V, GR, and VB-G are COFAA and EDI scholarship recipients from the Instituto Politécnico Nacional and members of the National Researchers System (SNI) from CONACyT.
References
1. Benedict KM, Gow SP, McAllister TA, Bookers CW, Hannon SJ, Checkley SL, et al. Antimicrobial resistance in Escherichia coli recovered from feedlot cattle and associations with antimicrobial use. PLOS ONE. (2015) 10:e143995. doi: 10.1371/journal.pone.0143995
2. Carr CM, Hersom KC, Jeong N, DiLorenzo J, Scheffler G, Faniola S, et al. Antibiotic Use and Resistance for Beef Cattle Producers. UF/IFAS (2019). Available online at: https://edis.ifas.ufl.edu
3. Heuer H, Schmitt H, Smalla K. Antibiotic resistance gene spread due to manure application on agricultural fields. Curr Opin Microbiol. (2011) 14:236–43. doi: 10.1016/j.mib.2011.04.009
4. Novais C, Freitas AR, Silveira E, Antunes P, Silva R, Coque TM, et al. Spread of multidrug-resistant Enterococcus to animals and humans: an underestimated role for the pig farm environment. J. Antimicrobial Chemother. (2013) 68:2746–54. doi: 10.1093/jac/dkt289
5. Wichmann F, Udikovic N, Andrew S, Handelsman J. Diverse antibiotic resistance genes in dairy cow manure. mBio. (2014) 5:e01017–3. doi: 10.1128/mBio.01017-13
6. Hammerum AM, Heuer OE. Human health hazards from antimicrobial-resistant Escherichia coli of animal origin. Clin Infect Dis. (2009) 48:916–21. doi: 10.1086/597292
7. Anes J, Nguyen SV, Eshwar AK, McCabe E, Macori G, Hurley D, et al. Molecular characterization of multidrug resistant Escherichia coli of bovine origin. Vet Microbiol. (2020) 242:108566. doi: 10.1016/j.vetmic.2019.108566
8. Hesp A, Veldman K, Van der Goot J, Mevius D, Van Schaik G. Monitoring antimicrobial resistance trends in commensal Escherichia coli from livestock, the Netherlands 1998 to 2016. Euro Surveill. (2019) 24:1800438. doi: 10.2807/1560-7917.ES.2019.24.25.1800438
9. Mercat M, Clermont O, Massot M, Ruppe E, de Garine M, Miguel E, et al. Escherichia coli population structure and antibiotic resistance at a buffalo/cattle interface in southern Africa. Appl Environ Microbiol. (2016) 82:1459–67. doi: 10.1128/AEM.03771-15
10. Robertson K, Green A, Allen L, Ihry T, White P, Chen W-S, et al. Foodborne outbreaks reported to the U.S. Food Safety and Inspection Service, fiscal years 2007 through 2012. J Food Protection. (2016) 79:442–7. doi: 10.4315/0362-028X.JFP-15-376
11. Baehler AA, Moxley RA. Escherichia coli O157:H7 induces attaching-effacing lesions in large intestinal mucosal explants from adult cattle. FEMS Microbiol Lett. (2000) 185:239–42. doi: 10.1111/j.1574-6968.2000.tb09068.x
12. Naylor SW, Gally DL, Low JC. Enterohaemorrhagic E. coli in veterinary medicine. Int J Med Microbiol. (2005) 295:419–41. doi: 10.1016/j.ijmm.2005.07.010
13. Barlow RS, Fegan N, Gobius KS. A comparison of antibiotic resistance integrons in cattle from separate beef meat production systems at slaughter. J Appl Microbiol. (2008) 104:651–8. doi: 10.1111/j.1365-2672.2007.03572.x
14. Amezquita BA, Quiñones B, Soto M, Lee BG, Yambao JC, Lugo OY, et al. Antimicrobial resistance profiles of shiga toxin producing Escherichia coli O157 and non-O157 recovered from domestic farm animals in rural communities in Northwestern Mexico. Antimicrob Resist Infection Control. (2016) 5:1. doi: 10.1186/s13756-015-0100-5
15. NOM-109-SSA1-1994-Procedimientos para la toma, manejo y transporte de muestras de alimentos para su análisis microbiológico. Diario Oficial de la Federacion (1994).
16. Brenner DJ, Krieg NR, Staley JT. Bergey's Manual of Systematic Bacteriology, Vol. 2. 2nd ed. Berlin: Springer (2008). p. 546–51.
17. Clinical and Laboratory Standards Institute. M100. Performance Standards for Antimicrobial Susceptibility Testing. 27th ed. Wayne: Clinical and Laboratory Standards Institute (2017). Available online at: https://clsi.org/standards/products/microbiology/documents/m100/
18. Jouini A, Vinue L, Slama KB, Saenz Y, Klibi N, Hammami S, et al. Characterization of CTX-M and SHV extended-spectrum B-lactamases and associated resistance genes in Escherichia coli strains of food samples in Tunisa. J Antimicrob Chemother. (2007) 60:1137–41. doi: 10.1093/jac/dkm316
19. Boerlin P, Travis R, Gyles CL, Reid R, Janecko N, Lim H, et al. Antimicrobial resistance and virulence genes of Escherichia coli isolates from swine in Ontario. Appl Environ Microbiol. (2005) 71:6753–61. doi: 10.1128/AEM.71.11.6753-6761.2005
20. Saenz Y, Briñas L, Dominguez E, Ruiz J, Zarazaga M, Vila J, et al. Mechanisms of resistance in multiple-antibiotic-resistant Escherichia coli strains of human, animal, and food origins. Antimicrob Agents Chemother. (2004) 48:3996–4001. doi: 10.1128/AAC.48.10.3996-4001.2004
21. Canizalez A, Gonzalez E, Vidal JE, Flores H, Leon N. Prevalence and antibiotic resistance profiles of diarrheagenic Escherichia coli strains isolated from food items in northwestern Mexico. Int J Food Microbiol. (2013) 164:36–45. doi: 10.1016/j.ijfoodmicro.2013.03.020
22. Adenipekum EO, Jackson CR, Oluwadun A, Iwalokun BA, Frye JG, Barrett JB, et al. Prevalence and antimicrobial resistance in Escherichia coli from food animals in Lagos, Nigeria. Microb Drug Resist. (2015) 21:358–65. doi: 10.1089/mdr.2014.0222
23. Alonso CA, Zarazaga M, Ben R, Jouini A, Ben K, Torres C. Antibiotic resistance in Escherichia coli in husbandry animals: the African perspective. Lett Appl Microbiol. (2017) 64:318–34. doi: 10.1111/lam.12724
24. Obaidat MM, Bani AE, Davis MA, Roess AA. Major diseases, extensive misuse, and high antimicrobial resistance of Escherichia coli in large-and small- scale dairy cattle farms in Jordan. J Dairy Sci. (2018) 101:2324–34. doi: 10.3168/jds.2017-13665
25. Sobur A, Momen AA, Salker R, Taufiqur AMM, Lutful K, Rahman MT. Antibiotic resistant Escherichia coli and Salmonella spp. associated with dairy cattle and farm environment having public health significance. Vet World. (2019) 12:984–93. doi: 10.14202/vetworld.2019.984-993
26. Gwida M, Awad A, El-Ashker M, Hotzel H, Monecke S, Ehricht R, et al. Microarray based detection of resistance and virulence factors in commensal Escherichia coli from livestock and farmers in Egypt. Vet Microbiol. (2020) 240:108539. doi: 10.1016/j.vetmic.2019.108539
27. Ramadan H, Jackson CR, Frye JG, Hiott LM, Samir M, Awad A, et al. Antimicrobial resistance, genetic diversity and multilocus sequence typing of Escherichia coli from humans, retail chicken and ground beef in Egypt. Pathogens. (2020) 9:357. doi: 10.3390/pathogens9050357
28. Amador P, Fernandes R, Prudêncio C, Duarte I. Prevalence of antibiotic resistance genes in multidrug-resistant enterobacteriaceae on portuguese livestock manure. Antibiotics. (2019) 8:23. doi: 10.3390/antibiotics8010023
29. Bourély C, Cazeau G, Jarrige N, Jouy E, Haenni M, Lupo A, et al. Co-resistance to amoxicillin and tetracycline as an indicator of multidrug resistance in Escherichia coli isolates from animals. Front Microbiol. (2019) 10:2288. doi: 10.3389/fmicb.2019.02288
30. Bumunangg EW, McAllister TA, Zaheer R, Ortega R, Stanford K, King R, et al. Characterization of non-O157 Escherichia coli from cattle faecal samples in the north-west province of South Africa. Microorganisms. (2019) 7:272. doi: 10.3390/microorganisms7080272
31. Hamid M, Tefera Y, Eguale T, Worku Y. Escherichia coli O157:H7: prevalence, identification and antimicrobial resistance in cattle slaughter at Addis Ababa Municipal Abattior, Ethiopia. Int J Adv Res Biol Sci. (2018) 5:10. doi: 10.22192/ijarbs.2018.05.10.014
32. Majalija S, Francis O, Sarah W, Lubowa M, Vudriko P, Nakamya F. Antibiotics susceptibility profiles of faecal Escherichia coli isolates from Dip-Litter broilers chickens in Northern and Central Uganda. Vet Res. (2010) 3:75–80. doi: 10.3923/vr.2010.75.80
33. Chandran A, Hatha AAM, Varghese S, Sheeja KM, Guardabassi L, Schwarz S, et al. Pet animals as reservoirs of antimicrobial-resistant bacteria. J Antimicrob Chemother. (2004) 54:321–32. doi: 10.1093/jac/dkh332
34. Selim SA, Ahmed SF, Abdel MH, Zakaria AM, Klena JD, Pangallo D. Prevalence and characterization of shiga-toxin O157:H7 and Non-O157:H7 enterohemorrhagic Escherichia coli isolated from different sources. Biotechnol Biotechnol Equip. (2013) 27:3834–42. doi: 10.5504/BBEQ.2013.0031
Keywords: bovine, antimicrobial resistance, MDR, Escherichia coli, ESBL
Citation: Martínez-Vázquez AV, Vázquez-Villanueva J, Leyva-Zapata LM, Barrios-García H, Rivera G and Bocanegra-García V (2021) Multidrug Resistance of Escherichia coli Strains Isolated From Bovine Feces and Carcasses in Northeast Mexico. Front. Vet. Sci. 8:643802. doi: 10.3389/fvets.2021.643802
Received: 18 December 2020; Accepted: 02 March 2021;
Published: 23 April 2021.
Edited by:
Lixing Huang, Jimei University, ChinaReviewed by:
Everlon Cid Rigobelo, Universidade Estadual Paulista, BrazilWeiwei Zhang, Ningbo University, China
Copyright © 2021 Martínez-Vázquez, Vázquez-Villanueva, Leyva-Zapata, Barrios-García, Rivera and Bocanegra-García. This is an open-access article distributed under the terms of the Creative Commons Attribution License (CC BY). The use, distribution or reproduction in other forums is permitted, provided the original author(s) and the copyright owner(s) are credited and that the original publication in this journal is cited, in accordance with accepted academic practice. No use, distribution or reproduction is permitted which does not comply with these terms.
*Correspondence: Virgilio Bocanegra-García, vbocanegg@hotmail.com; vbocanegra@ipn.mx