- 1Department of Biological Science and Technology, Yonsei University, Wonju, South Korea
- 2Department of Biomedical Laboratory Science, Yonsei University, Wonju, South Korea
Caspases, a family of cysteine protease enzymes, are a critical component of apoptotic cell death, but they are also involved in cellular differentiation. The expression of caspases during apoptotic processes in reproductive tissues has been shown in some species; however, the expression and regulation of caspases in the endometrium and placental tissues of pigs has not been fully understood. Therefore, we determined the expression of caspases CASP3, CASP6, CASP7, CASP8, CASP9, and CASP10 in the endometrium throughout the estrous cycle and pregnancy. During the estrous cycle, the expression of all caspases and during pregnancy, the expression of CASP3, CASP6, and CASP7 in the endometrium changed in a stage-specific manner. Conceptus and chorioallantoic tissues also expressed caspases during pregnancy. CASP3, cleaved-CASP3, and CASP7 proteins were localized to endometrial cells, with increased levels in luminal and glandular epithelial cells during early pregnancy, whereas apoptotic cells in the endometrium were limited to some scattered stromal cells with increased numbers on Day 15 of pregnancy. In endometrial explant cultures, the expression of some caspases was affected by steroid hormones (estradiol-17β and/or progesterone), and the cytokines interleukin-1β and interferon-γ induced the expression of CASP3 and CASP7, respectively. These results indicate that caspases are dynamically expressed in the endometrium throughout the estrous cycle and at the maternal-conceptus interface during pregnancy in response to steroid hormones and conceptus signals. Thus, caspase action could be important in regulating endometrial and placental function and epithelial cell function during the implantation period in pigs.
Introduction
The structure and function of the uterus changes significantly during the reproductive cycle and pregnancy in mammalian species. The degree of change in the endometrium during the cycle varies by species, with the most dramatic changes found in humans and non-human primates, which form a hemochorial type placenta (1–3). In pigs, which form a true epitheliochorial type placenta, the endometrium also undergoes morphological and functional change during the estrous cycle and pregnancy (4). During the estrous cycle in pigs, endometrial change is affected mainly by the ovarian steroid hormones estrogen and progesterone (5, 6), and during early pregnancy, it is driven by conceptus-derived signals, including estrogen and the cytokines interleukin-1β (IL1B), interferon-δ (IFND), and interferon-γ (IFNG), in addition to ovarian steroid hormones (4, 7, 8).
Apoptosis, a programmed cell death, plays a critical role in a variety of physiological processes in multicellular organisms. For example, it maintains functional tissue homeostasis by eliminating unwanted or dysfunctional cells (9, 10). Apoptosis occurs in the endometrium during the estrous cycle and pregnancy to regulate endometrial homeostasis (11, 12). In the human endometrium, apoptotic cell death is observed in endometrial epithelial and stromal cells, with a higher apoptotic rate in the late secretory to early proliferative phases than in the late proliferative to mid-secretory phases of the menstrual cycle (13). In pigs, cells undergoing apoptosis are detected mainly in endometrial stroma during the estrous cycle and early pregnancy and in luminal epithelial cells at the proestrus phase of the estrous cycle, but apoptotic cell death does not occur as dramatically in pigs as it does in primates during the reproductive cycle (14).
Apoptotic cell death is induced by intrinsic and extrinsic pathways. The intrinsic pathway is mediated by various intracellular stress and mitochondrial factors, whereas the extrinsic pathway is triggered by extracellular death signals, such as tumor necrosis factor (TNF) superfamily members: TNF-α, Fas ligand (FASLG), and TNF-related apoptosis-inducing ligand (TRAIL, also known as TNFSF10) (15, 16). The two pathways result in the activation of caspases, which are cytoplasmic cysteine protease enzymes, to induce apoptotic cell death. Caspases play essential roles in apoptosis and inflammation and are divided into two groups, initiator caspases (CASP8, CASP9, and CASP10) and executioner caspases (CASP3, CASP6, and CASP7) (9, 17, 18). Once the executioner caspases are activated by the initiator caspases, they recognize the aspartic residue of various intracellular target proteins and cleave them to cause apoptotic cell death. In that way, caspases are used as a representative marker for cells in which apoptosis has occurred. However, the apoptotic signaling pathway that activates caspases also plays an important role in the differentiation of various cell types, such as immune cells, trophoblasts, spermatocytes, epithelial cells, and stem cells (19, 20). It has been suggested that caspase activation is locally regulated during cellular remodeling without causing apoptotic cell death and that transient caspase activity is used for cell fate determination (10, 21).
Although endometrial changes during the estrous cycle and pregnancy involve the apoptotic process and the function of caspases is essential during apoptotic cell death and cellular differentiation, the pattern of caspase expression in the endometrium during the estrous cycle and pregnancy is not fully understood in pigs. We hypothesized that caspases are expressed in the endometrium during the estrous cycle and at the maternal-conceptus interface during pregnancy to regulate apoptosis and cellular differentiation. Therefore, we determined in pigs (1) the expression of caspases (CASP3, CASP6, CASP7, CASP8, CASP9, and CASP10) in the endometrium during the estrous cycle and pregnancy, conceptus tissues during early pregnancy, and chorioallantoic tissues during mid- to late pregnancy; (2) the localization of caspases and apoptotic cells in the endometrium; and (3) the regulation of caspase expression by the steroid hormones estrogen and progesterone and by the cytokines IL1B and IFNG in endometrial tissues.
Materials and Methods
Animals and Tissue Preparation
All experimental procedures involving animals were conducted in accordance with the Guide for the Care and Use of Research Animals in Teaching and Research and approved by the Institutional Animal Care and Use Committee of Yonsei University and the National Institute of Animal Science. Sexually mature Landrace and Yorkshire crossbred female gilts of similar age (6–8 months) and weight (100–120 kg) were assigned randomly to either cyclic or pregnant status, as described previously (22). Gilts assigned to the pregnant uterus status group were artificially inseminated with fresh boar semen at the onset of estrus (Day 0) and 12 h later. The reproductive tracts of the gilts were obtained immediately after slaughter on Days 0, 3, 6, 9, 12, 15, or 18 of the estrous cycle or Days 10, 12, 15, 30, 60, 90, or 114 of pregnancy (n = 3–6/day/status). Pregnancy was confirmed by the presence of apparently normal filamentous conceptuses in uterine flushings on Days 10, 12, and 15 and the presence of embryos and placenta on later days of pregnancy. Conceptus tissues were obtained from uterine flushings on Days 12 and 15 of pregnancy. Uterine flushings were obtained by introducing and recovering 25 ml of phosphate-buffered saline (PBS; pH 7.4) into each uterine horn. Chorioallantoic tissues were obtained on Days 30, 60, 90, and 114 of pregnancy (n = 3–4/day). Endometrial tissues from prepubertal gilts (n = 8; approximately 6 months of age) that had not undergone the estrous cycle, with no corpus luteum formed, were obtained from a local slaughterhouse. Endometrium, dissected free of myometrium, was collected from the middle portion of each uterine horn, snap-frozen in liquid nitrogen, and stored at −80°C prior to RNA extraction. For immunohistochemistry, cross-sections of the endometrium were fixed in 4% paraformaldehyde in PBS (pH 7.4) for 24 h and then embedded in paraffin as previously described (23).
Explant Cultures
To determine the effects of steroid hormones, IL1B, and IFNG on the expression of caspase mRNA in the endometrium, endometrial tissue was dissected from the myometrium and placed into warm phenol red-free Dulbecco's modified Eagle's medium/F-12 (DMEM/F-12) (Sigma) containing penicillin G (100 IU/ml) and streptomycin (0.1 mg/ml), as described previously (23–25) with some modifications. The endometrium was minced with scalpel blades into small pieces (2–3 mm3), and 500 mg were placed into T25 flasks with serum-free modified DMEM/F-12 containing 10 μg/ml insulin (Sigma), 10 ng/ml transferrin (Sigma), and 10 ng/ml hydrocortisone (Sigma). To analyze the effect of steroid hormones on the expression of caspases, endometrial explants from immature gilts, immediately after mincing, were cultured with rocking in the presence of increasing doses of estradiol-17β (E2; 0, 5, 50, or 500 pg/ml; Sigma) or progesterone (P4; 0, 0.3, 3, or 30 ng/ml; Sigma) for 24 h in an atmosphere of 5% CO2 in air at 37°C. The doses were chosen to encompass the full concentration range of physiological levels of E2 and P4 in the endometrium during the estrous cycle and pregnancy (8). To analyze the effect of IL1B on CASP3 and the effect of IFNG on CASP7 expression, endometrial explant tissues from Day 12 of the estrous cycle were treated with E2 (10 ng/ml), P4 (30 ng/ml), and increasing doses of IL1B (0, 1, 10, and 100 ng/ml; Sigma) or IFNG (0, 1, 10, and 100 ng/ml; R&D Systems, Minneapolis, MN, USA) at 37°C for 24 h. To determine the effect of the steroid hormones on the expression of CASP3 during the implantation period, endometrial explant tissues from Day 12 of the estrous cycle were treated with ethanol (control), E2 (10 ng/ml; Sigma, USA), P4 (30 ng/ml; Sigma, USA), P4+E2, P4+E2+ICI182,780 (ICI; an estrogen receptor antagonist; 200 ng/ml; Tocris Bioscience, Ellisville, MO, USA), or P4+E2+RU486 (RU; a progesterone receptor antagonist; 30 ng/ml; Sigma, USA) for 24 h. The explant tissues were then harvested, and total RNA was extracted for a real-time RT-PCR analysis to determine the expression levels of caspase mRNA. These experiments were conducted using endometrium from three gilts on Day 12 of the estrous cycle in triplicate and eight immature gilts.
Total RNA Extraction, Reverse Transcription-Polymerase Chain Reaction (RT-PCR), and Cloning of Porcine Caspase cDNA
Total RNA was extracted from endometrial and conceptus tissues using TRIzol reagent (Invitrogen, Carlsbad, CA, USA) according to the manufacturer's recommendations, as described previously (22). The quantity of RNA was assessed spectrophotometrically, and RNA integrity was validated following electrophoresis in 1% agarose gel. Four micrograms of total RNA from endometrial, conceptus, and chorioallantoic tissues were treated with DNase I (Promega, Madison, WI, USA) and reverse transcribed using SuperScript II Reverse Transcriptase (Invitrogen) to obtain cDNA. The cDNA templates were then diluted at a 1:4 ratio with sterile water and amplified by PCR using Taq polymerase (Takara Bio, Shiga, Japan) and specific primers based on porcine caspase mRNA sequences. The PCR conditions, sequences of primer pairs for caspases, and expected product sizes are listed in Supplementary Table 1. The PCR products were separated on 2% agarose gel and visualized by ethidium bromide staining. The identity of each amplified PCR product was verified by sequence analysis after cloning into the pCRII vector (Invitrogen).
Quantitative Real-Time RT-PCR
To analyze the levels of caspase expression in the endometrial and chorioallantoic tissues, real-time RT-PCR was performed using an Applied Biosystems StepOnePlus System (Applied Biosystems, Foster City, CA, USA) with the SYBR Green method, as described previously (22). Complementary DNA was synthesized from 4 μg of total RNA isolated from different uterine endometrial and chorioallantoic tissues, and the newly synthesized cDNA (total volume of 21 μl) was diluted 1:4 with sterile water and used for PCR. Power SYBR Green PCR Master Mix (Applied Biosystems) was used for the PCR reactions. The final reaction volume of 20 μl contained 2 μl of cDNA, 10 μl of 2× Master mix, 2 μl of each primer, and 4 μl of distilled H2O. The annealing temperature and number of cycles for PCR were the same for all products obtained. The results are reported as expression relative to that detected on Day 0 of the estrous cycle, that on Day 30 of pregnancy in chorioallantoic tissues, or that in control explant tissues after normalization of the transcript amount to the geometric mean of endogenous porcine ribosomal protein L7 (RPL7) and ubiquitin B (UBB), and TATA binding protein (TBP) controls, all using the 2−ΔΔCT method as previously described (26).
Immunohistochemical Analysis
To identify the type(s) of porcine endometrial cells expressing CASP3, cleaved-CASP3, CASP7, poly (ADP-ribose) polymerase (PARP1), an enzyme that is cleaved during apoptosis and used as a hallmark for apoptosis (27), and cleaved-PARP1, sections were immunostained. Sections (5 μm thick) were deparaffinized and rehydrated in an alcohol gradient. Tissue sections were boiled in citrate buffer (pH 6.0) for 10 min. Then, they were washed with PBST (PBS with 0.1% Tween-20) three times, and a peroxidase block was performed with 0.5% (v/v) H2O2 in methanol for 30 min. Tissue sections were then blocked with 10% normal goat serum for 30 min at room temperature. Rabbit polyclonal anti-CASP3 antibody (5 μg/ml; Cell Signaling, Danvers, MA, USA), rabbit polyclonal anti-cleaved-CASP3 antibody (5 μg/ml; Cell Signaling), mouse monoclonal anti-CASP7 antibody (5 μg/ml; Enzo Life Sciences, Farmingdale, NY, USA), rabbit polyclonal anti-PARP1 antibody (1 μg/ml; Santa Cruz Biotechnology, Santa Cruz, CA, USA), or rabbit monoclonal anti-cleaved-PARP1 antibody (1 μg/ml; GeneTex, Irvine, CA, USA) were added and incubated overnight at 4°C in a humidified chamber. For each tissue tested, purified normal rabbit IgG or mouse IgG was substituted for the primary antibody as a negative control. Tissue sections were washed with PBST three times. Biotinylated goat anti-rabbit or anti-mouse secondary antibody (1 μg/ml; Vector Laboratories, Burlingame, CA, USA) was added and incubated for 1 h at room temperature. Following washes with PBST, a streptavidin peroxidase conjugate (Invitrogen) was added to the tissue sections, which were then incubated for 10 min at room temperature. The sections were washed with PBST, and aminoethyl carbazole substrate (Invitrogen) was added to the tissue sections, which were then incubated for 10 min at room temperature. The tissue sections were washed in water, counterstained with Mayer's hematoxylin, and coverslipped. Images were captured using an Eclipse TE2000-U microscope (Nikon, Seoul, Korea) and processed with Adobe Photoshop CS6 software (Adobe Systems, Seattle, WA, USA).
TUNEL Assay and Immunofluorescence
Apoptotic cells in endometrial tissue sections were analyzed using the terminal deoxynucleotidyl transferase-mediated dUTP nick end labeling (TUNEL) assay with an In Situ Cell Death Detection Kit (Roche Diagnostics, Mannheim, Germany) used according to the manufacturer's recommendations, as described previously (28). Endometrial tissue sections (5 μm thick) were deparaffinized and rehydrated in an alcohol gradient. The sections were then boiled with 0.1 M citrate buffer (pH 6.0) for 3 min, cooled at room temperature for 10 min, and then washed three times in PBS. For a positive control for TUNEL staining, the sections were treated with DNase I (3 U/ml; Promega) in 50 mM Tris-HCl (pH 7.5), 10 mM MgCl2, and 1 mg/ml bovine serum albumin (BSA; Bovogen Biologicals, Melbourne, Australia) for 10 min at room temperature and then washed with PBS. Tissue sections were then blocked with 0.1 M Tris-HCl (pH 7.5) containing 3% (w/v) BSA and 20% (v/v) normal bovine serum for 30 min at room temperature. The TUNEL reaction was performed according to the kit instructions. After the TUNEL reactions, tissue sections were washed with PBS. The tissue sections were counterstained with 4',6-diamidino-2-phenylindole (DAPI), and fluorescence images were captured using an Eclipse TE2000-U microscope (Nikon, Seoul, Korea) with Adobe Photoshop CS6 software (Adobe Systems, Seattle, WA, USA).
Statistical Analysis
Data from real-time RT-PCR for caspase expression were subjected to ANOVA using the general linear models procedures in SAS (Cary, NC, USA). As sources of variation, the model included day, pregnancy status (cyclic or pregnant, Days 12 and 15 post-estrus), and their interactions to evaluate steady-state levels of caspase mRNA. Data from real-time RT-PCR performed to assess the effects of day of the estrous cycle (Days 0, 3, 6, 9, 12, 15, and 18) and pregnancy (Days 10, 12, 15, 30, 60, 90, and 114) and the effects of day of pregnancy (Days 30, 60, 90, and 114) on chorioallantoic tissues were analyzed using a least squares regression analysis. The effects of E2, P4, IL1B, and IFNG doses on explant cultures were analyzed by one-way ANOVA followed by Tukey's post-test. Data from real-time RT-PCR to assess the effects of steroid hormones and their receptor antagonists on explant culture were analyzed by preplanned orthogonal contrasts (control vs. E2; control vs. P4; P4 vs. P4+E2; P4+E2 vs. P4+E2+ICI; and P4+E2 vs. P4+E2+RU). Data are presented as means with standard error of the mean. A P-value <0.05 was considered significant, and P-values 0.05–0.10 were considered to indicate a trend toward significance.
Results
Expression of Caspase mRNA in the Endometrium During the Estrous Cycle and Pregnancy
In real-time RT-PCR analyses, we found that CASP3, CASP6, CASP7, CASP8, CASP9, and CASP10 mRNA was expressed in the endometrium during the estrous cycle and pregnancy (Figure 1). During the estrous cycle, the steady-state levels of CASP3 (quadratic, P = 0.0572), CASP6 (quadratic, P < 0.01), CASP7 (linear, P < 0.05), CASP8 (quadratic, P < 0.01), and CASP10 (quadratic, P < 0.05) mRNA changed, with the highest levels of CASP3, CASP6, CASP7, and CASP8 in the proestrus phase and that of CASP10 in the proestrus to metestrus phase. On Days 12 and 15 post-estrus, the expression of CASP3 was affected by day (P < 0.05), status (P < 0.01), and the day x status interaction (P < 0.05). The expression of CASP6 was affected by the day x status interaction (P < 0.05), that of CASP7 was affected by day (P < 0.05), that of CASP8 was affected by status (P < 0.01), and that of CASP9 was affected by day (P < 0.01). The expression of CASP10 was not affected by day, status, or the day x status interaction. During pregnancy, the steady-state levels of CASP3 (linear, P = 0.0526), CASP6 (cubic, P = 0.073), CASP7 (linear, P < 0.05), and CASP10 (quadratic, P < 0.05), but not CASP8 or CASP9 mRNA, changed with the highest levels on Day 12 for CASP3, on Day 15 for CASP7, and Day 60 for CASP6 and CASP10.
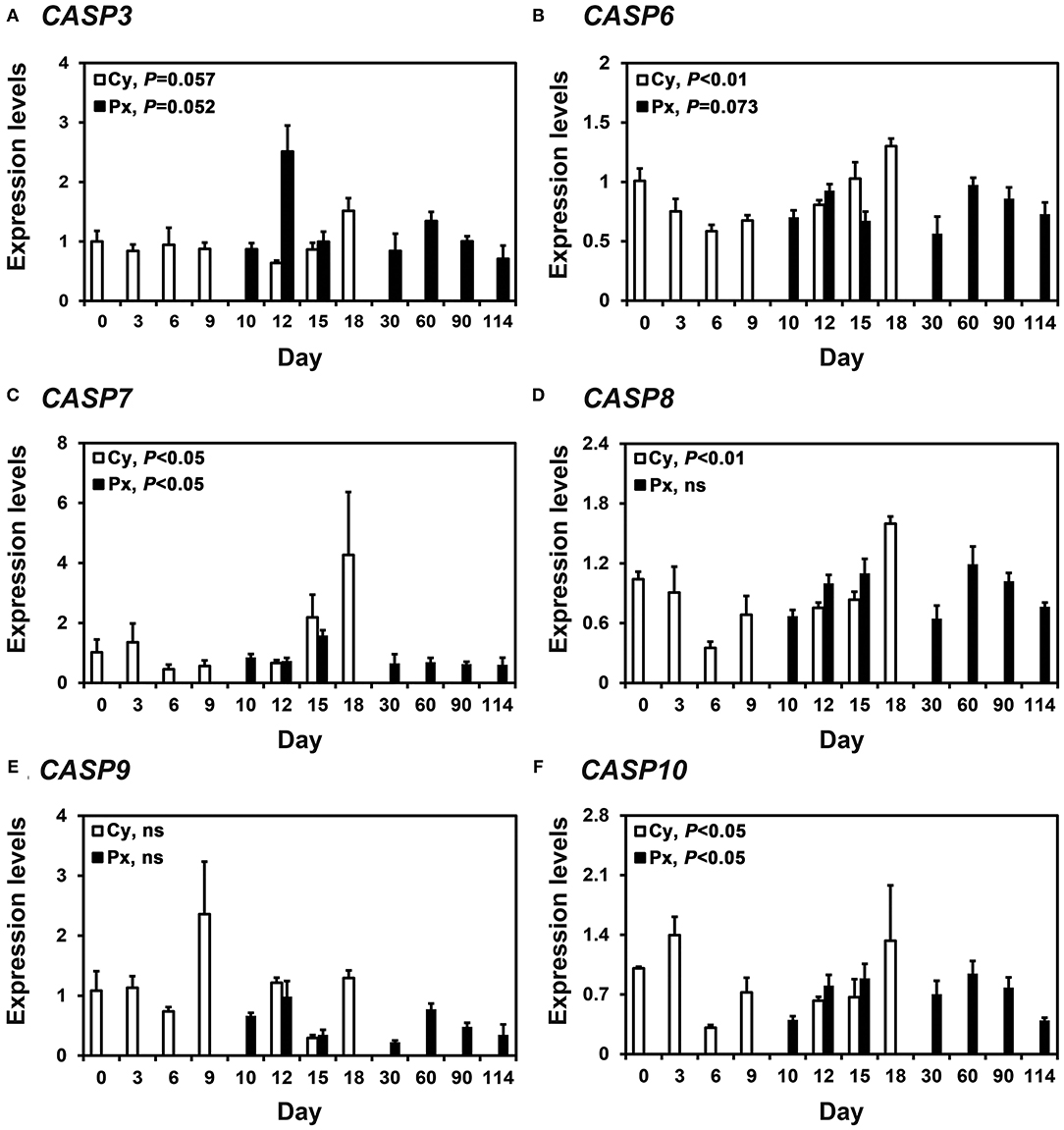
Figure 1. Expression of CASP3 (A), CASP6 (B), CASP7 (C), CASP8 (D), CASP9 (E), and CASP10 (F) mRNA in the endometrium during the estrous cycle and pregnancy in pigs. Endometrial tissue samples from cyclic (Cy) and pregnant (Px) gilts were analyzed by real-time RT-PCR, and data are reported as the expression relative to that detected on Day 0 of the estrous cycle after normalization to the transcript amount of the endogenous RPL7, UBB, and TBP mRNAs. Data are presented as the mean with standard error. Statistical significances for the effect of day during the estrous cycle and pregnancy are indicated; ns, not significant.
Expression of Caspase mRNA in Conceptuses During Early Pregnancy and Chorioallantoic Tissues in Later Stages of Pregnancy
In RT-PCR analysis using cDNAs from conceptuses from Days 12 and 15 of pregnancy, we found that CASP3, CASP6, CASP7, CASP8, and CASP10 mRNA but not CASP9 mRNA in conceptuses from both days of early pregnancy (Figure 2A). These caspases were also detectable in endometrial tissues from same days. In addition, we performed real-time RT-PCR analyses to determine whether the expression of CASP3, CASP6, CASP7, CASP8, CASP9, and CASP10 mRNA changed in chorioallantoic tissues during pregnancy. The abundance of CASP3, CASP6, CASP7, CASP8, CASP9, and CASP10 mRNA in chorioallantoic tissues changed, with the highest levels on Day 30 for CASP3 and at term for CASP6, CASP7, CASP8, CASP9, and CASP10 (linear effect of day for CASP6, CASP7, CASP8, CASP9, and CASP10, P < 0.01; quadratic effect of day for CASP3, P < 0.01) (Figure 2B).
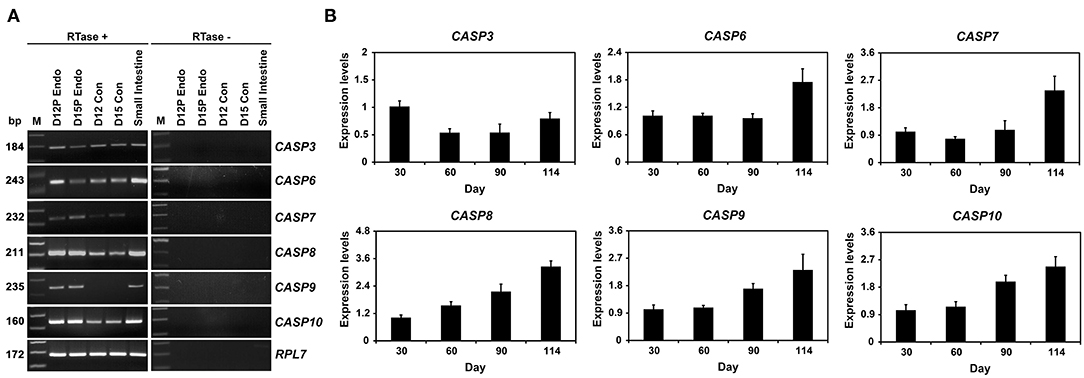
Figure 2. Expression of CASP3, CASP6, CASP7, CASP8, CASP9, and CASP10 by conceptuses from Days 12 and 15 of pregnancy (A) and chorioallantoic tissues during mid- to late pregnancy (B). (A) RT-PCR analyses of CASP3, CASP6, CASP7, CASP8, CASP9, and CASP10 mRNA in conceptuses on Days 12 and 15 of pregnancy were performed using total RNA preparations. RPL7 was used as a positive control. RTase ±, with (+) or without (–) reverse transcriptase; M, molecular marker; D12 Endo, endometrium on day 12 of pregnancy; D12 Con, Day 12 conceptus; D15 Con, Day 15 conceptus. (B). Real-time RT-PCR analyses of the expression of CASP3, CASP6, CASP7, CASP8, CASP9, and CASP10 mRNA in chorioallantoic tissues on Days 30, 60, 90, and 114 of pregnancy. Data are reported as expression relative to that detected on Day 30 of pregnancy after normalization to the transcript amount of the endogenous RPL7, UBB, and TBP mRNAs control, and data are presented as means with standard errors.
Localization of CASP3, Cleaved-CASP3, and CASP7 Proteins in the Endometrium on Days 12 and 15 Post-estrus
Having determined that CASP3, CASP6, CASP7, CASP8, CASP9, and CASP10 mRNA was present in the endometrium during the estrous cycle and pregnancy and in conceptuses and chorioallantoic tissues during pregnancy and that the expression of CASP3 and CASP7 mRNA was highest during early pregnancy, we next determined the cellular localization of the CASP3, cleaved-CASP3 (an active form), and CASP7 proteins in the endometrium on Days 12 and 15 post-estrus using immunohistochemistry (Figure 3). CASP3 proteins were mainly detected in endometrial luminal (LE) and glandular epithelial (GE) cells and in scattered stromal cells, with stronger signal intensity on Days 12 and 15 of pregnancy than during the estrous cycle, and they were localized subcellularly to both the cytoplasm and the nucleus (Figure 3A). The active form of CASP3, cleaved-CASP3 protein, was localized primarily to the nucleus of LE cells and some stromal cells in the endometrium on Days 12 and 15 of pregnancy (Figure 3B). Both CASP3 and cleaved-CASP3 proteins were detected in the small intestine used as a positive control. CASP7 protein was localized to the cytoplasm of LE and stromal cells in the endometrium, but only on Day 15 of pregnancy (Figure 3C). Trophectoderm cells in conceptuses were also positive for CASP7 protein on Day 15 of pregnancy (Figure 3C). CASP7 protein was detected in the lymph node used as a positive control. Immunohistochemistry for cleaved-CASP7 was not done due to the lack of an appropriate antibody to detect porcine cleaved-CASP7 protein.
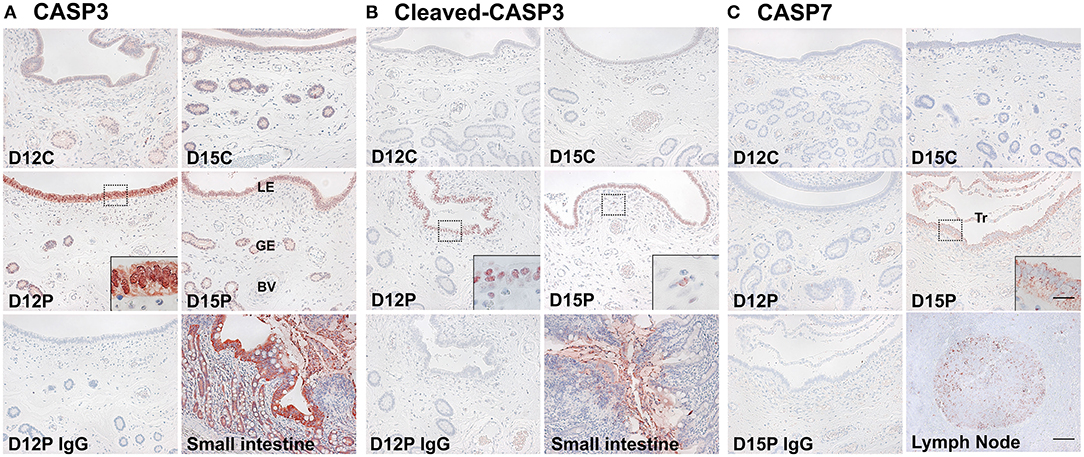
Figure 3. Immunohistochemical localization of CASP3 (A), cleaved-CASP3 (B), and CASP7 (C) proteins in the endometrium on Days 12 and 15 post-estrus. Representative uterine sections from Days 12 or 15 of pregnancy immunostained with normal IgG are shown as negative controls, and tissue sections from the small intestine and lymph node serve as positive controls for CASP3, cleaved-CASP3, and CASP7 immunostaining. D, Day; C, estrous cycle; P, pregnancy; LE, luminal epithelium; GE, glandular epithelium; BV, blood vessel; Tr, trophectoderm. Bars = 100 μm and 20 μm in insets.
TUNEL Staining and PARP Cleavage Analysis for in situ Apoptotic Cell Death in the Endometrium During the Estrous Cycle and Pregnancy
Because CASP3 and CASP7 proteins were localized to endometrial epithelial and stromal cells during the estrous cycle and pregnancy, we determined whether cells expressing CASP3 and CASP7 were undergoing apoptotic cell death. Because apoptotic cells undergo DNA degradation and PARP1, an enzyme involved in DNA repair, is cleaved by caspases (29), we performed the TUNEL assay and immunostaining of PARP1 and cleaved-PARP1 in endometrial tissues from pregnant pigs. We found that apoptotic cells in the endometrium during pregnancy were predominantly in stromal cells, not in epithelial cells, with many apoptotic cells found on Day 15 of pregnancy and very few cells found during the later stages of pregnancy (Figure 4A). PARP1 protein was localized to most cell types in the endometrium on Days 12 and 15 of the estrous cycle and pregnancy (Figure 4B), but cleaved-PARP1, a marker for apoptotic cells, was localized primarily to stromal cells on Day 15 of pregnancy (Figure 4C). The PARP1 and cleaved-PARP1 proteins were also detected in the ovary used as a positive control.
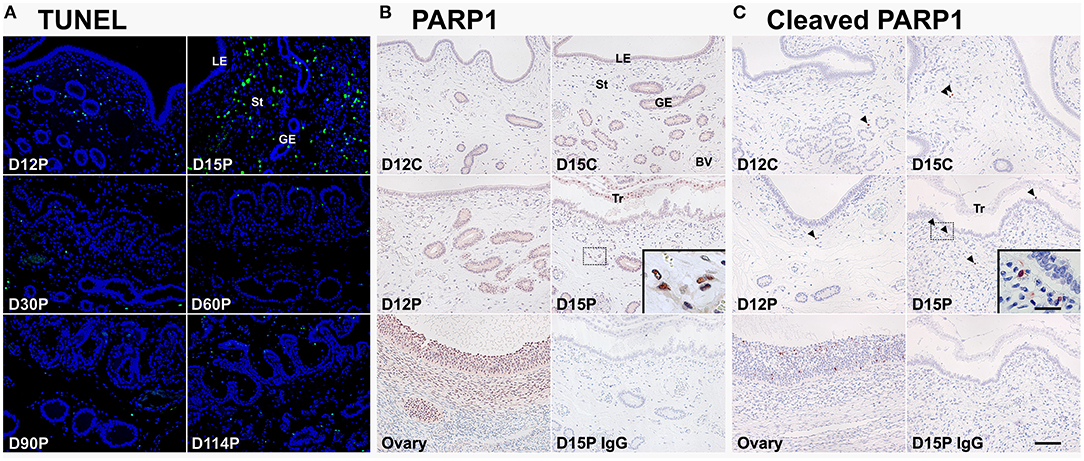
Figure 4. TUNEL staining (A) and immunohistochemical localization of PARP1 (B) and cleaved-PARP1 (C) proteins for in situ apoptotic cell death in the endometrium during the estrous cycle and pregnancy. Cells undergoing apoptosis in the endometrium during pregnancy were localized using the TUNEL assay (green), and tissue morphology is shown by DAPI staining. Representative uterine sections from Day 15 of pregnancy immunostained with normal IgG are shown as negative control and tissue sections from the ovary serve as positive controls for PARP1 and cleaved-PARP1 immunostaining. D, day; P, pregnancy; LE, luminal epithelium; GE, glandular epithelium; St, stroma; BV, blood vessel; Tr, trophectoderm. Arrowheads indicate cleaved-PARP1-positive cells. Bar = 100 μm and 20 μm in inset.
Effects of the Steroid Hormones E2 and P4 on Caspase Expression in Endometrial Tissue of Prepubertal Gilts
Because the expression of caspases changed during the estrous cycle and because E2 from the ovary and P4 from the corpus luteum regulate the expression of many endometrial genes during the cycle (4, 8), we hypothesized that E2 and P4 might affect the expression of caspases in the endometrium. Therefore, we obtained endometrial tissues from immature gilts, which had not been exposed to cyclical ovarian hormones, and treated them with increasing doses of E2 or P4. We found that the expression of CASP7 mRNA was decreased by E2 (0 vs. 500 pg/ml, P < 0.05), but the expression of CASP3, CASP6, CASP8, CASP9, and CASP10 mRNA was unaffected by E2 (Figure 5). The expression of CASP7 (0 vs. 30 ng/ml, P < 0.05), CASP8 (0 vs. 3 ng/ml and 0 vs. 30 ng/ml, P < 0.01), and CASP10 (0 vs. 3 ng/ml, P < 0.01; 0 vs. 30 ng/ml, P < 0.05), but not CASP3, CASP6, and CASP9, was affected by P4 treatment (Figure 6).
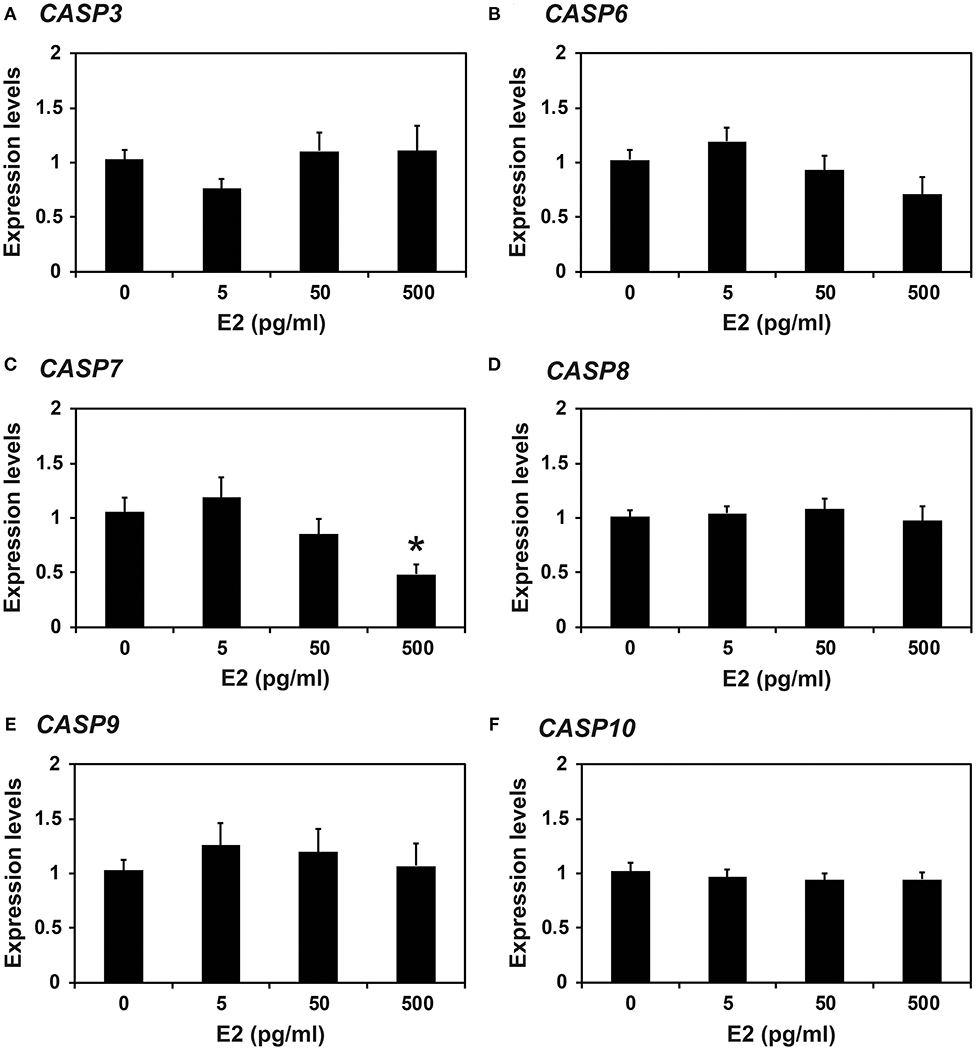
Figure 5. Effect of estradiol on CASP3 (A), CASP6 (B), CASP7 (C), CASP8 (D), CASP9 (E), and CASP10 (F) mRNA in endometrial explant cultures. Endometrial explants from immature gilts were cultured at 37°C in DMEM/F-12 with increasing doses of estradiol-17β (E2; 0, 5, 50, and 500 pg/ml) for 24 h. Experiments were performed with endometria from eight gilts. The abundance of mRNA, determined by real-time RT-PCR, is relative to that of CASP3, CASP6, CASP7, CASP8, CASP9, and CASP10 mRNA in the control group of endometrial explants (0 pg/ml E2) after normalization to the transcript amount of RPL7, UBB, and TBP mRNAs. Data are presented as the mean with standard error. The asterisk denotes statistically significant difference when values were compared with the control group: *P < 0.05.
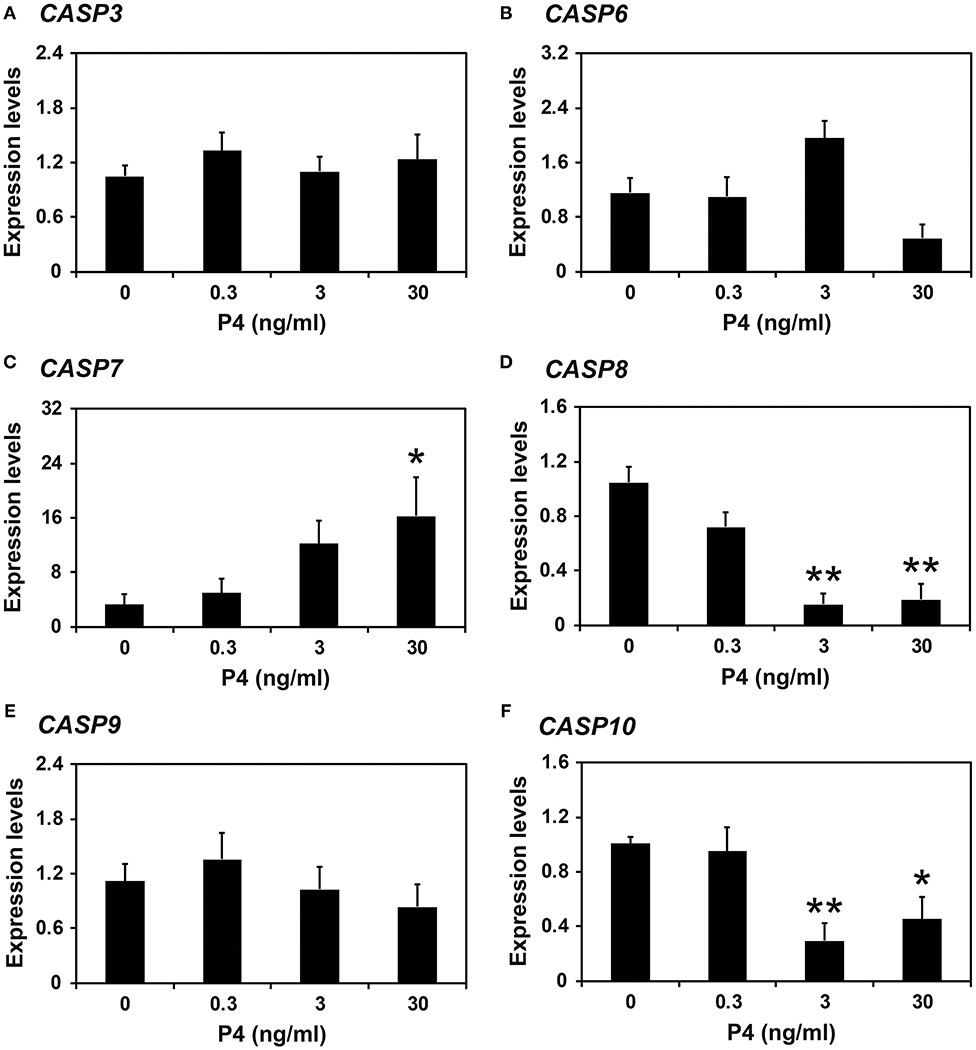
Figure 6. Effect of progesterone on CASP3 (A), CASP6 (B), CASP7 (C), CASP8 (D), CASP9 (E), and CASP10 (F) mRNA in endometrial explant cultures. Endometrial explants from immature gilts were cultured at 37°C in DMEM/F-12 with increasing doses of progesterone (P4; 0, 0.3, 3, and 30 ng/ml) for 24 h. Experiments were performed with endometria from eight gilts. The abundance of mRNA, determined by real-time RT-PCR, is relative to that of CASP3, CASP6, CASP7, CASP8, CASP9, and CASP10 mRNA in the control group of endometrial explants (0 ng/ml P4) after normalization to the transcript amount of RPL7, UBB, and TBP mRNAs. Data are presented as the mean with standard error. The asterisks denote statistically significant differences when values were compared with the control group: *P < 0.05; **P < 0.01.
Effects of IL1B and Steroid Hormones on CASP3 and the Effect of IFNG on CASP7 Expression in Endometrial Tissues
Because the expression of CASP3 and CASP7 was highest on Days 12 and 15 of pregnancy, respectively, and porcine conceptuses secrete estrogen and IL1B2 into the uterine lumen on Day 12 and IFND and IFNG on Day 15 (4, 8), we assumed that the expression of CASP3 on Day 12 could be affected by estrogen and IL1B and that of CASP7 on Day 15 of pregnancy could be affected by IFNG. We treated endometrial explant tissues from Day 12 of the estrous cycle with increasing doses of IL1B and steroid hormones and found that IL1B induces the expression of CASP3 (0 vs. 1 ng/ml, P < 0.05; Figure 7A), but steroid hormones and their receptor antagonists do not affect the expression of CASP3 (Figure 7B). When increasing doses of IFNG were administered, the IFNG induced the expression of CASP7 (0 vs. 10 pg/ml, 0 vs. 100 pg/ml; P < 0.01) (Figure 7C).
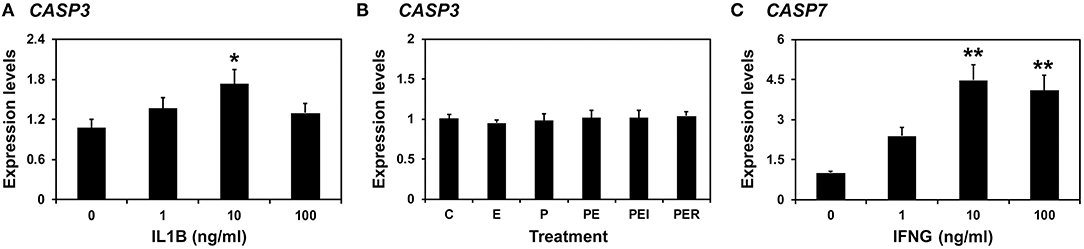
Figure 7. Effects of IL1B (A) and steroid hormones (B) on the expression of CASP3 and the effect of IFNG on CASP7 (C) in endometrial explant cultures. Endometrial explants from gilts on Day 12 of the estrous cycle were cultured (A) with increasing doses of IL1B (0, 1, 10, and 100 ng/ml) in the presence of with E2 (estradiol-17β; 10 ng/ml) and P4 (progesterone; 30 ng/ml), (B) with steroid hormones [control (C), E2 (E), P4 (P), E2 + P4 (PE), E2+P4+ICI (I, an estrogen receptor antagonist) (PEI), or E2+P4+RU (R; a progesterone receptor antagonist) (PER)], or (C) with increasing doses of IFNG (0, 1, 10, and 100 ng/ml) in the presence of with E2 (10 ng/ml) and P4 (30 ng/ml). The abundance of mRNA expression, determined by real-time RT-PCR analyses, was relative to that for CASP3 and CASP7 mRNA in the control group of endometrial explants after normalization to the transcript amounts of RPL7, UBB, and TBP mRNAs. Data are presented as means with standard error. These treatments were performed in triplicate using tissues obtained from each of three gilts. The asterisks denote statistically significant differences when values were compared with the control group: *P < 0.05; **P < 0.01.
Discussion
The significant findings of this study in pigs were: (1) caspases CASP3, CASP6, CASP7, CASP8, CASP9, and CASP10 were expressed in the endometrium during the estrous cycle and pregnancy in a stage- and pregnancy status–specific manner; (2) conceptuses on Days 12 and 15 of pregnancy and chorioallantoic tissues from Day 30 of pregnancy to term expressed caspases, except CASP9, on Days 12 and 15 of pregnancy; (3) CASP3, cleaved-CASP3, and CASP7 proteins were localized to endometrial cells, with increased signal intensity in LE and GE cells during early pregnancy; (4) apoptotic cells in the endometrium were localized to some scattered stromal cells, with increased numbers on Day 15 of pregnancy; (5) E2 and P4 affected the expression of some caspases in endometrial tissues; and (6) IL1B and IFNG upregulated the expression of CASP3 and CASP7, respectively, in endometrial explant tissues.
Caspases are essential mediators of apoptosis and play an important role in a variety of biological processes (9, 10, 17). Two groups of caspases, initiator caspases and executioner caspases, are activated during the pathway to apoptotic activation. Caspases are expressed in the endometrium during the reproductive cycle and pregnancy and that they mediate apoptotic cell death in various species (2, 30, 31). However, the expression of all initiator and executioner caspases in the endometrium throughout the estrous/menstrual cycle and at the maternal-conceptus interface during pregnancy has not been fully studied in any species. The results of this study indicate the variable expression of the initiator and executioner caspases in the endometrium during the estrous cycle and pregnancy and in conceptus/chorioallantoic tissues throughout pregnancy in pigs.
During the estrous cycle, the expression of caspases CASP3, CASP6, CASP7, CASP8, and CASP10 changed with the stage of the cycle, with the highest levels in the proestrus phase for CASP3, CASP6, and CASP7 and in the proestrus to metestrus phase for CASP8 and CASP10. These data indicate that the expression of caspases is dynamically regulated in the endometrium during the estrous cycle and may be related to cyclic remodeling of this tissue in pigs. The incidence of apoptotic cell death in LE cells was previously shown by TUNEL assay to be highest in the estrus phase in pigs (14), suggesting that caspases expressed in the proestrus phase could cause apoptotic cell death in the endometrium in the estrus phase. In bovine endometrium, CASP3 expression does not change during the estrous cycle, but active forms of CASP3 proteins increase at the follicular and early luteal phases compared with the mid- to late luteal phase (2). Furthermore, CASP8 expression in the bovine endometrium increases toward the follicular phase from the luteal phase (31). Thus, it seems that the endometrial expression of some caspases increases in pigs and cows as the cycle moves toward the estrus phase.
The pattern of caspase expression in the endometrium during the estrous cycle led us to postulate that the expression of caspases and the activation of apoptotic signaling could be related to cyclical changes in the endometrium triggered by the actions of steroid hormones from the ovary. In this study, we found that P4 decreased the expression of CASP8 and CASP10 in endometrial explant tissues. Because the endometrial expression of CASP8 and CASP10 was low at the diestrus phase and high at the proestrus to metestrus phase of the estrous cycle, it is likely that P4 causes the decreased levels of CASP8 and CASP10 expression in the endometrium at the diestrus phase of the cycle in pigs. However, P4 increased the expression of CASP7, whereas E2 decreased the expression of CASP7 in endometrial explant tissues, even though the endometrial expression of CASP7 was high in the proestrus phase of the cycle, when plasma levels of P4 and E2 decrease and increase, respectively (8). These data indicate that the regulation of CASP7 expression in the endometrium during the estrous cycle is much more complex than can be explained by the simple action of P4 and E2 and thus needs further analysis.
Although the levels of caspase expression during pregnancy have not been much studied in any species, it has been shown that the levels of active Casp3 protein in the rat endometrium are highest at mid-pregnancy (30). In this study, the endometrial expression of caspases CASP3, CASP6, CASP7, and CASP10 during pregnancy changed, with the highest levels occurring during early pregnancy for CASP3 and CASP7 and during mid-pregnancy for CASP6 and CASP10, suggesting that the expression of caspases is pregnancy stage–specific and varies with the type of caspase. In particular, we observed that the expression of CASP3 and CASP7 was highest on Days 12 and 15 of pregnancy, respectively, which is the period when conceptuses interact with the endometrium for implantation (4, 7, 8). Because the implanting porcine conceptus secretes estrogen and IL1B on Day 12 of pregnancy and type I and II IFNs, IFND and IFNG, around Day 15 of pregnancy (4, 8), we postulated that estrogen and/or IL1B might be responsible for inducing CASP3 expression in the endometrium on Day 12 and that IFNG might be responsible for inducing CASP7 expression on Day 15 of pregnancy. Indeed, our results performed using endometrial explant culture revealed increased expression of CASP3 in response to IL1B but not E2, whereas CASP7 expression was stimulated by IFNG. These data indicate that the expression of CASP3 and CASP7 in the endometrium during early pregnancy in pigs is induced by conceptus-derived IL1B and IFNG, respectively.
Because CASP3 and CASP7 are well-known executioner caspases during apoptosis (9, 17), we determined which cell type(s) expressed CASP3 and CASP7 proteins in the endometrium during early pregnancy and whether the cells expressing CASP3 and CASP7 were undergoing apoptotic cell death. Our results show that CASP3 and active CASP3 proteins were predominantly localized to LE and stromal cells on Days 12 and 15 of pregnancy and that the CASP7 protein was primarily localized to LE cells on Day 15 of pregnancy. Interestingly, however, results from the TUNEL assay and cleaved-PARP1 staining show that only stromal cells in the endometrium, not epithelial cells, were undergoing apoptosis during early pregnancy. These data suggest that CASP3 and CASP7 might not be involved in endometrial epithelial apoptosis during early pregnancy in pigs.
Executioner caspases CASP3, CASP6, and CASP7 play critical roles in both apoptotic cell death and cell differentiation in various cell types, such as keratinocytes, muscle cells, neurons, and stem cells (18). Also, the initiator caspase CASP8, which is expressed by cytotrophoblast cells, is involved in the differentiation of cytotrophoblast cells into the syncytiotrophoblast layer in human placental villi (32–34). Thus, the increased endometrial expression of CASP3 and CASP7 in response to conceptus-derived signals at the time of conceptus implantation could be expected to act on epithelial cell differentiation instead of activating apoptosis. Indeed, at the time of implantation LE cells of the porcine endometrium show various aspects of differentiated cellular characteristics: changed morphology (35–37), increased production of secretory proteins, including fibroblast growth factor 7 (38) and secreted phosphoprotein 1 (39), and increased expression of immunity-related molecules, including interferon α/β receptor 1 and 2 (40), interferon gamma receptor 1 and 2 (25), cysteine-X-cysteine motif chemokine ligand 12 (41), TNF superfamily 10 (28), and cytotoxic T-lymphocyte-associated protein 4 (Yoo and Ka, unpublished data). The expression of most of those molecules in endometrial epithelial cells is induced by conceptus signals, estrogen, IL1B, or IFNG, and those molecules play important roles in conceptus implantation. Thus, it is likely that CASP3 and CASP7 are also involved in activating the differentiation process of endometrial LE cells in response to conceptus-derived signals. However, the nature of the differentiated cellular characteristics mediated by CASP3 and CASP7 in endometrial LE cells still needs further study.
Our results also show that caspases were expressed in conceptus tissues during early pregnancy and in chorioallantoic tissues during mid- to late pregnancy. In particular, the levels of CASP6, CASP7, CASP8, CASP9, and CASP10 expression in chorioallantoic tissues increased as the pregnancy came to term. However, as determined by TUNEL assay in this study, apoptotic cells were barely detectable in chorioallantoic tissues during pregnancy. It has been shown that CASP3 proteins levels in porcine placental tissue are higher on Day 30 than on Days 60, 80, and 90 of pregnancy, what coincides with the expression pattern of CASP3 mRNA in this study (42). In ovine placentas, CASP3 and CASP9 proteins increase toward term, and the levels of active CASP3 and CASP9 are increased in placentas with intrauterine growth restriction pregnancy compared with normal pregnancy (43). In bovine placental tissues obtained at parturition, CASP3 and CASP8 mRNA and proteins are expressed (44), and CASP8 and CASP10 are expressed in human placental villi at term (33, 45). Thus, the expression of caspases in placental tissues is common among mammalian species and increases toward term. In addition, because accumulated evidence shows that apoptotic cell death increases in the placenta when pregnancy complications occur, such as intrauterine growth restriction, preeclampsia and preterm premature rupture of membranes in humans (46, 47), and in placental tissues derived from somatic cell nuclear transfer–cloned embryos in pigs (48), it is likely that caspases are important in regulating placental function and are activated in situations of inappropriate placental development during pregnancy.
Conclusion
In conclusion, the results of this study in pigs show that caspases are expressed in the endometrium, with differential expression patterns throughout the estrous cycle and pregnancy, and in the conceptus and chorioallantoic tissues during pregnancy; CASP3 and CASP7 are localized primarily to endometrial epithelial cells during early pregnancy; the steroid hormones E2 and P4 regulate the expression of caspases in endometrial tissues; and IL1B and IFNG induce the expression of CASP3 and CASP7, respectively, in endometrial tissues. These results suggest that caspases dynamically expressed in the endometrium and at the maternal-conceptus interface could play important roles in the establishment and maintenance of pregnancy in pigs by regulating apoptosis and epithelial differentiation.
Data Availability Statement
The original contributions presented in the study are included in the article/Supplementary Material, further inquiries can be directed to the corresponding author/s.
Ethics Statement
The animal study was reviewed and approved by Institutional Animal Care and Use Committee of Yonsei University.
Author Contributions
WJ, MH, B-YJ, and HK: conceptualization, methodology. IY, JH, MK, SL, and YC: investigation. WJ, IY, and HK: data analysis, writing—original draft preparation. MH and B-YJ: writing—reviewing and editing. HK: supervision, funding acquisition. All authors contributed to the article and approved the submitted version.
Funding
This work was supported by The National Research Foundation Grant funded by the Korean Government (#2019R1A2C1004670), Republic of Korea.
Conflict of Interest
The authors declare that the research was conducted in the absence of any commercial or financial relationships that could be construed as a potential conflict of interest.
Supplementary Material
The Supplementary Material for this article can be found online at: https://www.frontiersin.org/articles/10.3389/fvets.2021.641916/full#supplementary-material
References
1. Reynolds LP, Borowicz PP, Vonnahme KA, Johnson ML, Grazul-Bilska AT, Wallace JM, et al. Animal models of placental angiogenesis. Placenta. (2005) 26:689–708. doi: 10.1016/j.placenta.2004.11.010
2. Arai M, Yoshioka S, Tasaki Y, Okuda K. Remodeling of bovine endometrium throughout the estrous cycle. Anim Reprod Sci. (2013) 142:1–9. doi: 10.1016/j.anireprosci.2013.08.003
3. Bazer FW. Pregnancy recognition signaling mechanisms in ruminants and pigs. J Anim Sci Biotechnol. (2013) 4:23. doi: 10.1186/2049-1891-4-23
4. Bazer FW, Johnson GA. Pig blastocyst-uterine interactions. Differentiation. (2014) 87:52–65. doi: 10.1016/j.diff.2013.11.005
5. Oberleithner H, Riethmuller C, Ludwig T, Shahin V, Stock C, Schwab A, et al. Differential action of steroid hormones on human endothelium. J Cell Sci. (2006). 119(Pt 9):1926–32. doi: 10.1242/jcs.02886
6. Annie L, Gurusubramanian G, Roy VK. Estrogen and progesterone dependent expression of visfatin/NAMPT regulates proliferation and apoptosis in mice uterus during estrous cycle. J Steroid Biochem Mol Biol. (2019) 185:225–36. doi: 10.1016/j.jsbmb.2018.09.010
7. Geisert RD, Whyte JJ, Meyer AE, Mathew DJ, Juarez MR, Lucy MC, et al. Rapid conceptus elongation in the pig: an interleukin 1 beta 2 and estrogen-regulated phenomenon. Mol Reprod Dev. (2017) 84:760–74. doi: 10.1002/mrd.22813
8. Ka H, Seo H, Choi Y, Yoo I, Han J. Endometrial response to conceptus-derived estrogen and interleukin-1beta at the time of implantation in pigs. J Anim Sci Biotechnol. (2018) 9:44. doi: 10.1186/s40104-018-0259-8
9. Crawford ED, Wells JA. Caspase substrates and cellular remodeling. Annu Rev Biochem. (2011) 80:1055–87. doi: 10.1146/annurev-biochem-061809-121639
10. Nakajima YI, Kuranaga E. Caspase-dependent non-apoptotic processes in development. Cell Death Differ. (2017) 24:1422–30. doi: 10.1038/cdd.2017.36
11. Song J, Rutherford T, Naftolin F, Brown S, Mor G. Hormonal regulation of apoptosis and the Fas and Fas ligand system in human endometrial cells. Mol Hum Reprod. (2002) 8:447–55. doi: 10.1093/molehr/8.5.447
12. Joswig A, Gabriel HD, Kibschull M, Winterhager E. Apoptosis in uterine epithelium and decidua in response to implantation: evidence for two different pathways. Reprod Biol Endocrinol. (2003) 1:44. doi: 10.1186/1477-7827-1-44
13. Kokawa K, Shikone T, Nakano R. Apoptosis in the human uterine endometrium during the menstrual cycle. J Clin Endocrinol Metab. (1996) 81:4144–7. doi: 10.1210/jcem.81.11.8923873
14. Okano A, Ogawa H, Takahashi H, Geshi M. Apoptosis in the porcine uterine endometrium during the estrous cycle, early pregnancy and post partum. J Reprod Dev. (2007) 53:923–30. doi: 10.1262/jrd.18139
15. Fluhr H, Wenig H, Spratte J, Heidrich S, Ehrhardt J, Zygmunt M. Non-apoptotic Fas-induced regulation of cytokines in undifferentiated and decidualized human endometrial stromal cells depends on caspase-activity. Mol Hum Reprod. (2011) 17:127–34. doi: 10.1093/molehr/gaq082
16. Spratte J, Princk H, Schutz F, Rom J, Zygmunt M, Fluhr H. Stimulation of chemokines in human endometrial stromal cells by tumor necrosis factor-alpha and interferon-gamma is similar under apoptotic and non-apoptotic conditions. Arch Gynecol Obstet. (2018) 297:505–12. doi: 10.1007/s00404-017-4586-3
17. Riedl SJ, Shi Y. Molecular mechanisms of caspase regulation during apoptosis. Nat Rev Mol Cell Biol. (2004) 5:897–907. doi: 10.1038/nrm1496
18. Shalini S, Dorstyn L, Dawar S, Kumar S. Old, new and emerging functions of caspases. Cell Death Differ. (2015) 22:526–39. doi: 10.1038/cdd.2014.216
19. Levy R, Nelson DM. To be, or not to be, that is the question. Apoptosis in human trophoblast Placenta. (2000) 21:1–13. doi: 10.1053/plac.1999.0450
20. Yi CH, Yuan J. The Jekyll and Hyde functions of caspases. Dev Cell. (2009) 16:21–34. doi: 10.1016/j.devcel.2008.12.012
21. Solier S, Fontenay M, Vainchenker W, Droin N, Solary E. Non-apoptotic functions of caspases in myeloid cell differentiation. Cell Death Differ. (2017) 24:1337–47. doi: 10.1038/cdd.2017.19
22. Lee S, Yoo I, Han J, Ka H. Antimicrobial peptides cathelicidin, PMAP23, and PMAP37: Expression in the endometrium throughout the estrous cycle and at the maternal-conceptus interface during pregnancy and regulation by steroid hormones and calcitriol in pigs. Theriogenology. (2021) 160:1–9. doi: 10.1016/j.theriogenology.2020.10.034
23. Seo H, Kim M, Choi Y, Lee CK, Ka H. Analysis of lysophosphatidic acid (LPA) receptor and LPA-induced endometrial prostaglandin-endoperoxide synthase 2 expression in the porcine uterus. Endocrinology. (2008) 149:6166–75. doi: 10.1210/en.2008-0354
24. Han J, Yoo I, Lee S, Jung W, Kim HJ, Hyun SH, et al. Atypical chemokine receptors 1, 2, 3 and 4: Expression and regulation in the endometrium during the estrous cycle and pregnancy and with somatic cell nucleus transfer-cloned embryos in pigs. Theriogenology. (2019) 129:121–9. doi: 10.1016/j.theriogenology.2019.02.021
25. Yoo I, Seo H, Choi Y, Jang H, Han J, Lee S, et al. Analysis of interferon-gamma receptor IFNGR1 and IFNGR2 expression and regulation at the maternal-conceptus interface and the role of interferon-gamma on endometrial expression of interferon signaling molecules during early pregnancy in pigs. Mol Reprod Dev. (2019) 86:1993–2004. doi: 10.1002/mrd.23287
26. Livak KJ, Schmittgen TD. Analysis of relative gene expression data using real-time quantitative PCR and the 2(-Delta Delta C(T)) method. Methods. (2001) 25:402–8. doi: 10.1006/meth.2001.1262
27. Germain M, Affar EB, D'Amours D, Dixit VM, Salvesen GS, Poirier GG. Cleavage of automodified poly(ADP-ribose) polymerase during apoptosis. Evidence for involvement of caspase-7. J Biol Chem. (1999) 274:28379–84. doi: 10.1074/jbc.274.40.28379
28. Yoo I, Kye YC, Han J, Kim M, Lee S, Jung W, et al. Uterine epithelial expression of the tumor necrosis factor superfamily: a strategy for immune privilege during pregnancy in a true epitheliochorial placentation species. Biol Reprod. (2020) 102:828–42. doi: 10.1093/biolre/ioz233
29. Soldani C, Scovassi AI. Poly(ADP-ribose) polymerase-1 cleavage during apoptosis: an update. Apoptosis. (2002) 7:321–8. doi: 10.1023/a:1016119328968
30. Shooner C, Caron PL, Frechette-Frigon G, Leblanc V, Dery MC, Asselin E. TGF-beta expression during rat pregnancy and activity on decidual cell survival. Reprod Biol Endocrinol. (2005) 3:20. doi: 10.1186/1477-7827-3-20
31. Groebner AE, Schulke K, Unterseer S, Reichenbach HD, Reichenbach M, Buttner M, et al. Enhanced proapoptotic gene expression of XAF1, CASP8 and TNFSF10 in the bovine endometrium during early pregnancy is not correlated with augmented apoptosis. Placenta. (2010) 31:168–77. doi: 10.1016/j.placenta.2009.12.017
32. Black S, Kadyrov M, Kaufmann P, Ugele B, Emans N, Huppertz B. Syncytial fusion of human trophoblast depends on caspase 8. Cell Death Differ. (2004) 11:90–8. doi: 10.1038/sj.cdd.4401307
33. Ka H, Hunt JS. FLICE-inhibitory protein: expression in early and late gestation human placentas. Placenta. (2006) 27:626–34. doi: 10.1016/j.placenta.2005.08.004
34. Longtine MS, Chen B, Odibo AO, Zhong Y, Nelson DM. Caspase-mediated apoptosis of trophoblasts in term human placental villi is restricted to cytotrophoblasts and absent from the multinucleated syncytiotrophoblast. Reproduction. (2012) 143:107–21. doi: 10.1530/REP-11-0340
35. Stroband HW, Taverne N, Langenfeld K, Barends PM. The ultrastructure of the uterine epithelium of the pig during the estrous cycle and early pregnancy. Cell Tissue Res. (1986) 246:81–9. doi: 10.1007/BF00219003
36. Cencic A, Guillomot M, Koren S, La Bonnardiere C. Trophoblastic interferons: do they modulate uterine cellular markers at the time of conceptus attachment in the pig? Placenta. (2003) 24:862–9. doi: 10.1016/s0143-4004(03)00135-8
37. Jalali BM, Lukasik K, Witek K, Baclawska A, Skarzynski DJ. Changes in the expression and distribution of junction and polarity proteins in the porcine endometrium during early pregnancy period. Theriogenology. (2020) 142:196–206. doi: 10.1016/j.theriogenology.2019.09.041
38. Ka H, Jaeger LA, Johnson GA, Spencer TE, Bazer FW. Keratinocyte growth factor is up-regulated by estrogen in the porcine uterine endometrium and functions in trophectoderm cell proliferation and differentiation. Endocrinology. (2001) 142:2303–10. doi: 10.1210/endo.142.6.8194
39. Garlow JE, Ka H, Johnson GA, Burghardt RC, Jaeger LA, Bazer FW. Analysis of osteopontin at the maternal-placental interface in pigs. Biol Reprod. (2002) 66:718–25. doi: 10.1095/biolreprod66.3.718
40. Jang H, Choi Y, Yoo I, Han J, Kim M, Ka H. Characterization of interferon alpha and beta receptor IFNAR1 and IFNAR2 expression and regulation in the uterine endometrium during the estrous cycle and pregnancy in pigs. Theriogenology. (2017) 88:166–73. doi: 10.1016/j.theriogenology.2016.09.025
41. Han J, Jeong W, Gu MJ, Yoo I, Yun CH, Kim J, et al. Cysteine-X-cysteine motif chemokine ligand 12 and its receptor CXCR4: expression, regulation, and possible function at the maternal-conceptus interface during early pregnancy in pigs. Biol Reprod. (2018). doi: 10.1093/biolre/ioy147
42. Sanchis EG, Cristofolini AL, Fiorimanti MR, Barbeito CG, Merkis CI. Apoptosis and cell proliferation in porcine placental vascularization. Anim Reprod Sci. (2017) 184:20–8. doi: 10.1016/j.anireprosci.2017.06.009
43. Monson T, Wright T, Galan HL, Reynolds PR, Arroyo JA. Caspase dependent and independent mechanisms of apoptosis across gestation in a sheep model of placental insufficiency and intrauterine growth restriction. Apoptosis. (2017) 22:710–8. doi: 10.1007/s10495-017-1343-9
44. Kamemori Y, Wakamiya K, Nishimura R, Hosaka Y, Ohtani S, Okuda K. Expressions of apoptosis-regulating factors in bovine retained placenta. Placenta. (2011) 32:20–6. doi: 10.1016/j.placenta.2010.10.016
45. Dubova EA, Pavlov KA, Aleksandrova NV, Baev OR, Shchyogolev AI, Sukhikh GT. Caspase expression in placental terminal villi in spontaneous and induced pregnancy. Bull Exp Biol Med. (2014) 158:92–6. doi: 10.1007/s10517-014-2700-0
46. Tanir HM, Sener T, Artan S, Kaytaz B, Sahin-Mutlu F, Ozen ME. Programmed cell death (apoptosis) in placentas from normal pregnancy and pregnancy complicated by term (t) and preterm (p) premature rupture of membranes (PROM). Arch Gynecol Obstet. (2005) 273:98–103. doi: 10.1007/s00404-005-0028-8
47. Longtine MS, Chen B, Odibo AO, Zhong Y, Nelson DM. Villous trophoblast apoptosis is elevated and restricted to cytotrophoblasts in pregnancies complicated by preeclampsia, IUGR, or preeclampsia with IUGR. Placenta. (2012) 33:352–9. doi: 10.1016/j.placenta.2012.01.017
Keywords: pig, endometrium, apoptosis, caspase, differentiation
Citation: Jung W, Yoo I, Han J, Kim M, Lee S, Cheon Y, Hong M, Jeon B-Y and Ka H (2021) Expression of Caspases in the Pig Endometrium Throughout the Estrous Cycle and at the Maternal-Conceptus Interface During Pregnancy and Regulation by Steroid Hormones and Cytokines. Front. Vet. Sci. 8:641916. doi: 10.3389/fvets.2021.641916
Received: 15 December 2020; Accepted: 25 January 2021;
Published: 12 February 2021.
Edited by:
Dariusz Jan Skarzynski, Institute of Animal Reproduction and Food Research (PAS), PolandReviewed by:
Beenu Moza Jalali, Institute of Animal Reproduction and Food Research (PAS), PolandAgnieszka Blitek, Institute of Animal Reproduction and Food Research of PAS, Poland
Copyright © 2021 Jung, Yoo, Han, Kim, Lee, Cheon, Hong, Jeon and Ka. This is an open-access article distributed under the terms of the Creative Commons Attribution License (CC BY). The use, distribution or reproduction in other forums is permitted, provided the original author(s) and the copyright owner(s) are credited and that the original publication in this journal is cited, in accordance with accepted academic practice. No use, distribution or reproduction is permitted which does not comply with these terms.
*Correspondence: Hakhyun Ka, aGthJiN4MDAwNDA7eW9uc2VpLmFjLmty