- Center of Excellence for Poultry Science, University of Arkansas, Fayetteville, AR, United States
Proteins are considered the most expensive nutrients in commercial modern broiler production, and their dietary inclusion at low levels is pivotal to minimize feed costs and reduce nitrogen waste. The quest for an environmentally friendly source of proteins that favor the formulation of low protein diets without compromising broiler health, welfare, and growth performance has become a hotspot in nutrition research. Due to its high protein content, the naturally growing Spirulina microalgae is considered a promising nutrient source. The purpose of the present study was, therefore, to determine the effects of Spirulina supplementation on liver bacterial translocation, hematological profile, and circulating inflammatory and redox markers in broilers fed a low-protein diet. One-day-old Ross 708 male broilers (n = 180) were randomly assigned into one of three experimental treatments: standard diet as a control, low protein diet, and low protein diet supplemented with 100 g/kg of Spirulina. Target molecular markers were measured in the peripheral blood circulation using real-time quantitative PCR. Reducing dietary proteins increased bacterial translocation and systemic inflammation as indicated by proportions of basophils among blood leukocytes. The expression levels of circulating pro-inflammatory cytokines [interleukin (IL)-3, IL-6, IL-4, IL-18, and tumor necrosis factor-α], chemokines (CCL-20), and NOD-like receptor family pyrin domain containing 3 inflammasome were significantly upregulated in birds fed the low protein diet compared with the control. The inclusion of Spirulina reversed these effects, which indicates that Spirulina reduces systemic inflammation- and bacterial translocation-induced by a low protein diet and could be a promising alternative protein source in poultry diets.
Introduction
Global poultry meat production is projected to increase by 32% by 2030 and 59% by 2050 from production in 2012, with low- and middle-income countries making up most of this demand (1). The cost of feed, particularly proteins, and availability of feedstuffs are by far the most important factors in poultry production sustainability and competitiveness. Indeed, soybean meal (SBM) is the preferred source of protein in broiler diets, as it is the only economically feasible protein source currently used in many countries. However, the continuous increase in global demand for high-quality animal protein, which consequently needs an improved animal production and abundant animal feeds, may inevitably surpass the SBM supply. This may, in turn, affect the inflation and the market price of SBM, which is linearly rising. Furthermore, there has also been a precedent set to limit animal-derived protein sources for animal feed in the European Union via Regulation (EC) No. 999/2001 (2). Thus, numerous innovative and effective approaches will need to be implemented to breach this looming protein gap to keep up with the worldwide demand for poultry meat.
Reducing crude protein (CP) in broiler diets has been extensively studied, yet the underlying issues of increased fat deposition and decreased performance are still prevalent (3–5). Implementing the ideal protein concept, computer least-cost formulation, and commercially produced crystalline amino acids allow for the lowest dietary CP diet thus far (6, 7). The reduction of CP with supplemental crystalline amino acids alleviates the demand for SBM, yet alternative protein sources will also be important for meeting the anticipated increase in demand for protein in animal and poultry feed. Alternatives for SBM in broiler diets have been investigated, including traditional and novel options such as canola meal, blood meal, meat and bone meal, fish meal, peameal, sunflower meal, insect meal, and numerous algae species (8–12). Many of the trials involving algae in broiler feed have focused on growth performance or meat quality with limited mechanistic explanations for their beneficial effects. Algae inclusion rates of up to 100 g/kg have maintained or increased performance parameters in chickens reared under conventional conditions (13–15).
Spirulina (Athrospira sp.) platensis is a filamentous spiral-shaped blue-green cyanobacterium that grows naturally in warm and alkaline aquatic media and is particularly interesting as a possible animal feed protein source due to its high level of CP (43–70%) and balanced amino acid profile. In addition to essential fatty acids, vitamins, minerals, and pigments (16), Spirulina contains several compounds shown to have antioxidant, anti-inflammatory, immune-modulating, and probiotic properties (17–20). Spirulina is also considered a nutritionally safe feed ingredient with no risk of mineral toxicity and free of the major algal toxin, microcystins (21, 22). Spirulina use as a feed ingredient for multiple species has been outlined (23), and one of the confounding issues put forth is the underlying mechanisms by which Spirulina impacts them. The purpose of this study was, therefore, to determine hematological parameters, inflammatory markers, and oxidative stress in broilers fed a low CP diet with and without Spirulina.
Materials and Methods
Animals and Treatment
A total of 180 1-day-old male (Ross 708) broiler chicks were obtained from a commercial hatchery and randomly allotted to one of the 15-floor pens (5 pens/diet; 12 birds/pen) in an environmentally controlled pilothouse. Chicks were reared in pens top-dressed with ~4 cm of fresh wood shavings, and the temperature gradually decreased from 32°C on day 1 to 20°C on day 27. The trial was conducted from February to March 2020. Birds received 23 h of light until day 10; then, light duration was decreased to 18 h for the remainder of the trial. Birds were given ad libitum access to feed (Table 1) and water throughout the trial. A standard corn–SBM basal diet (3,250 kcal/kg−1, 21% CP) was fed to all birds until day 14, at which point experimental diets were introduced until 37 days of age. The experimental diets included an industry-standard level protein (~20% CP) corn/SBM control (SCP), reduced (~17%) CP corn/SBM diet (LCP), and LCP diet where Spirulina was included at the level of 100 g/kg (SP-LCP). All experimental diets were isocaloric and met all essential amino acid needs set forth by the primary breeder. Both low CP diets were formulated to be isonitrogenous.
Blood Sampling and Hematological Analysis
On day 37, blood (3 ml) was collected from non-fasted birds (10 birds/treatment; 2 birds/pen), via the brachial wing vein, in ethylenediaminetetraacetic acid-coated tubes and immediately placed on ice. The hematologic profile of 1 ml of whole blood samples was measured using the Cell-Dyn 3500 automated hematology analyzer calibrated for chicken blood (Abbott Diagnostics, Abbott Park, IL) within 3 h of sampling. Data collected included the percent of heterophils, lymphocytes, monocytes, eosinophils, and basophils and the calculated heterophil to lymphocyte (H/L) ratio. For gene expression analysis, 250 μl of blood was added to tubes containing 750 μl of TRIzol LS reagent according to manufacturer's recommendations (Life Technologies Corporation, CA, US). The birds' bodyweights were 2.61 ± 0.07, 2.60 ± 0.07, and 2.72 ± 0.08 kg for SCP, LCP, and SP-LCP, respectively.
Liver Bacterial Translocation
As previously described by Tellez et al. (24), a section of the right liver was aseptically removed from 30 chickens (10 birds/treatment), placed into sterile sampling containers, and homogenized. Samples were then diluted 1:4 based on tissue weight with sterile 0.9% saline. Liver samples were then transferred to sterile 96-well Bacti flat-bottom culture plates and diluted 10-fold before being plated on tryptic soy agar to evaluate total counts of Enterobacteriaceae per gram of tissue. Samples were incubated under aerobic conditions at 37°C for 24 h.
RNA Isolation, Reverse Transcription, and Quantitative Real-Time PCR
Total RNA was isolated from whole blood samples using Trizol LS reagent (ThermoFisher Scientific, Rockford, IL) according to manufacturer's recommendations. Take 3 Micro-Volume Plate using Synergy HT multimode microplate reader (BioTek, Winooski, VT) determined RNA concentrations and purity. Real-time quantitative PCR (Applied Biosystems 7500 Real-Time PCR System) was performed using 5 μl of complementary DNA, 1 μl of each forward and reverse specific primers, and 10 μl of SYBR Green Master Mix (ThermoFisher Scientific, Rockford, IL, United States) in a total 25-μl reaction. Oligonucleotide primers used for chicken cytokines, chemokines, inflammasomes, anti-oxidative, and 18S (housekeeping) genes are summarized in Table 2. The real-time quantitative PCR cycling conditions were 50°C for 2 min, 95°C for 10 min followed by 40 cycles of a two-step amplification program (95°C for 15 s and 58°C for 1 min). At the end of the amplification, melting curve analysis was applied using the dissociation protocol from the Sequence Detection system to exclude contamination with unspecific PCR products. 18S RNA was used to normalize the relative expression of targeted genes via the 2−ΔΔCt method (25). All values were compared relative with those in the SCP dietary control group.
Statistical Analysis
All data were analyzed as a complete randomized design with one-way ANOVA in JMP Pro v 15.0 (SAS Institute, Cary, NC, United States). Post-hoc analysis assessment through multiple Dunnett comparisons was used when appropriate. Differences were considered significant at P ≤ 0.05.
Results
Hematological Analysis
Reducing CP by 3% in the diet (LCP) increased lymphocyte percent (P < 0.01) while decreasing that of heterophils (P = 0.026) and thereby resulted in a significantly lower H/L ratio (P = 0.014) (Table 3). The LCP group exhibited a significantly higher percent of basophil but no differences in monocyte or eosinophil percent compared with the SCP group. The inclusion of Spirulina reversed basophil percent to a similar level as the SCP (control) group. Spirulina inclusion had no impact on the H/L ratio or the percent of heterophils, lymphocytes, monocytes, or eosinophils (Table 3).
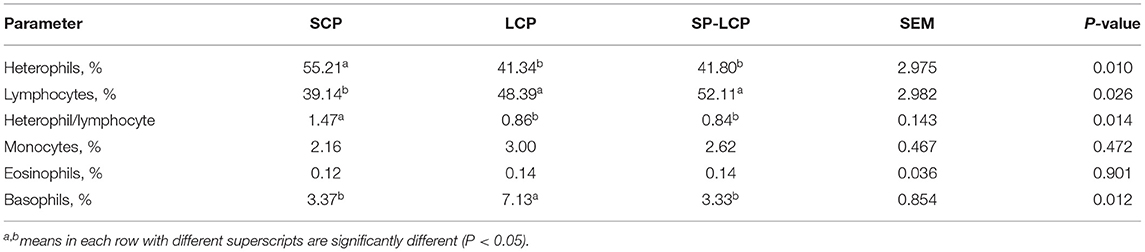
Table 3. Effects of a standard corn/soy (SCP), low crude protein (LCP), and Spirulina included low crude protein diet (SP-LCP) on blood lymphocyte profiles of broilers on d 37.
Liver Bacterial Translocation
The presence of bacteria in the liver reflects bacterial translocation from the gastrointestinal tract. Bacterial counts in the liver were elevated (P < 0.05) by the LCP diet compared with birds fed with the SCP control diet (3.34 ± 0.7 vs. 2.44 ± 0.4 log10 CFU/g). Bacterial translocation was ameliorated in birds fed the Spirulina LCP diet to levels (1.34 ± 0.4 log10 cfu/g) lower than in the liver of birds fed the SCP control diet.
Circulating Inflammation-, Toll-Like Receptor-, and Antioxidant-Associated Markers
The LCP diet upregulated (P < 0.05) the expression of pro-inflammatory cytokines interleukin-6 (IL-6), interleukin-3 (IL-3), interleukin-18 (IL-18), and tumor necrosis factor-alpha (TNF-α), as indicated by expression of lipopolysaccharide-induced TNF-α factor (Figures 1A–D, 2A) and that of the regulatory cytokine interleukin-10 (IL-10) and interleukin-4 (IL-4) (Figure 2D). Similarly, the LCP diet upregulated the expression of circulating chemokine C-C motif ligand 20CCL-20 (Figure 3A) but not that of C-C motif ligand 4 or C-X-C motif ligand 14 (Figures 3B–D). LCP supplementation increased the messenger RNA (mRNA) abundance of NOD-like receptor family pyrin domain-containing 3 but not NOD-like receptor family CARD domain-containing 3, NOD-like receptor family CARD domain-containing 5, and nucleotide-binding oligomerization domain and leucine-rich repeat-containing X1 inflammasomes (Figures 4A–D). LCP diet upregulated the expression of myeloid differentiation primary response protein 88 (MyD88) but not that of toll-like receptors 3, 4, and 21 (TLR-3, TLR-4, TLR-21, and MyD88) (Figures 5A–D). Spirulina inclusion reversed the expression of all of the markers mentioned earlier to levels being expressed in birds fed with the SCP control diet (Figures 1–4).
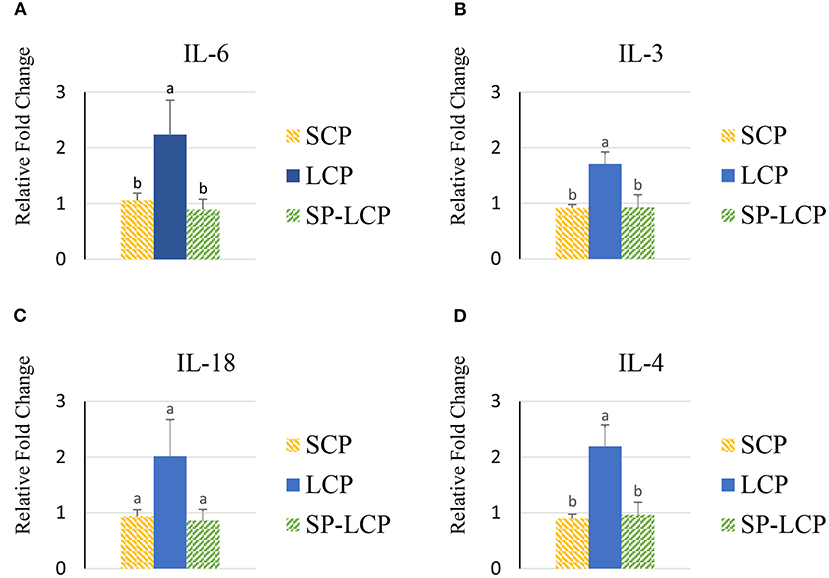
Figure 1. Effects of Spirulina on circulating cytokine expression profile. Relative mRNA abundance of circulating IL-6 (A), IL-3 (B), IL-18 (C), and IL-4 (D) in broilers on day (d) 37 were determined by real-time qPCR. Treatments include standard corn/soy (SCP) diet as control and low crude protein without (LCP) or with Spirulina (SP-LCP). Data are presented as mean ± SEM (n = 8/group). Means with different letters are significantly different (P < 0.05).
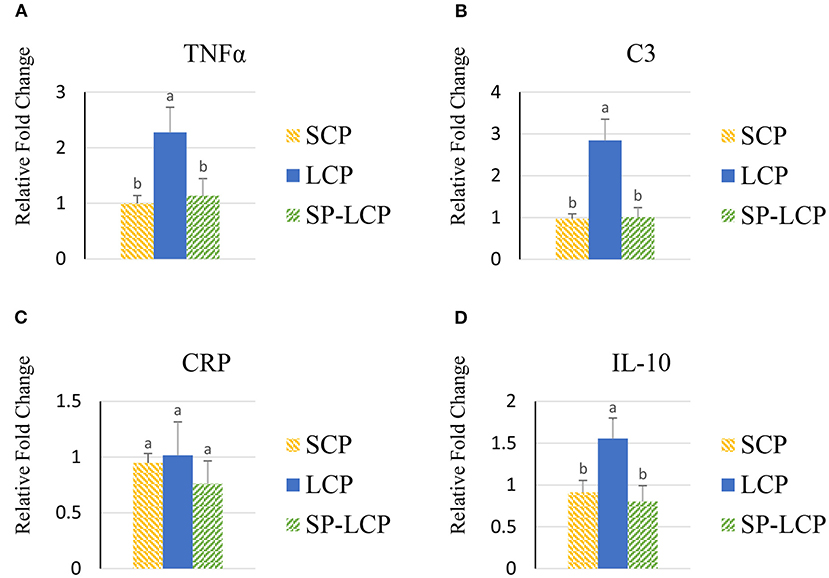
Figure 2. Effects of Spirulina on circulating TNFα, C3, CRP, and IL-10 expression. Relative mRNA abundance of circulating TNF-α (A), C3 (B), CRP (C), and IL-10 (D) expression in broilers on day (d) 37 were determined by real-time qPCR. Treatments include standard corn/soy (SCP) diet as control and low crude protein without (LCP) or with Spirulina (SP-LCP). Data are presented as mean ± SEM (n = 8/group). Means with different letters are significantly different (P < 0.05).
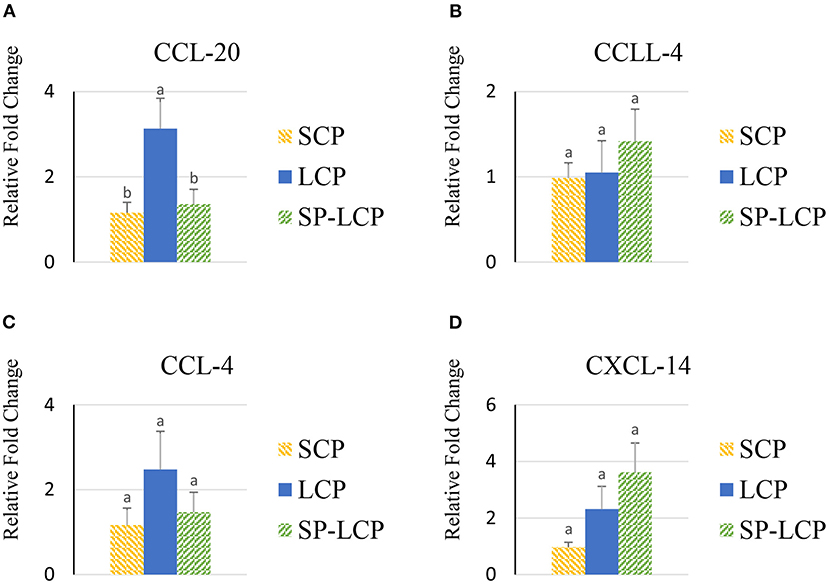
Figure 3. Effects of Spirulina on circulating chemokine expression profile. Relative mRNA abundance of circulating chemokine (C-C motif) ligand 20 (CCL-20) (A), chemokine-like ligand 4 (CCLL-4) (B), chemokine (C-C motif) ligand 4 (CCL-4) (C), and chemokine (C-X-C motif) ligand 14 (CXCL-14) (D) in broilers on day (d) 37 were determined by real-time qPCR. Treatments include standard corn/soy (SCP) diet as control and low crude protein without (LCP) or with Spirulina (SP-LCP). Data are presented as mean ± SEM (n = 8/group). Means with different letters are significantly different (P < 0.05).
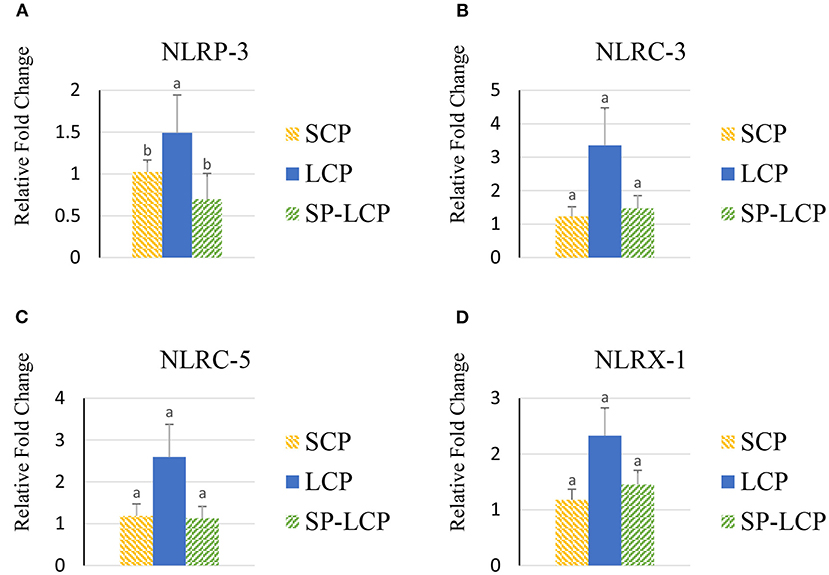
Figure 4. Effects of Spirulina on circulating inflammasome expression. Relative mRNA abundance of NOD-like receptor family pyrin domain-containing 3 (NLRP-3) (A), NOD-like receptor family CARD domain-containing 3 (NLRC-3) (B), NOD-like receptor family CARD domain-containing 5 (NLRC-5) (C), and nucleotide-binding oligomerization domain, leucine-rich repeat-containing X1 (NLRX-1) (D) in broilers on day (d) 37 were determined by real-time qPCR. Treatments include standard corn/soy (SCP) diet as control and low crude protein without (LCP) or with Spirulina (SP-LCP). Data are presented as mean ± SEM (n = 8/group). Means with different letters are significantly different (P < 0.05).
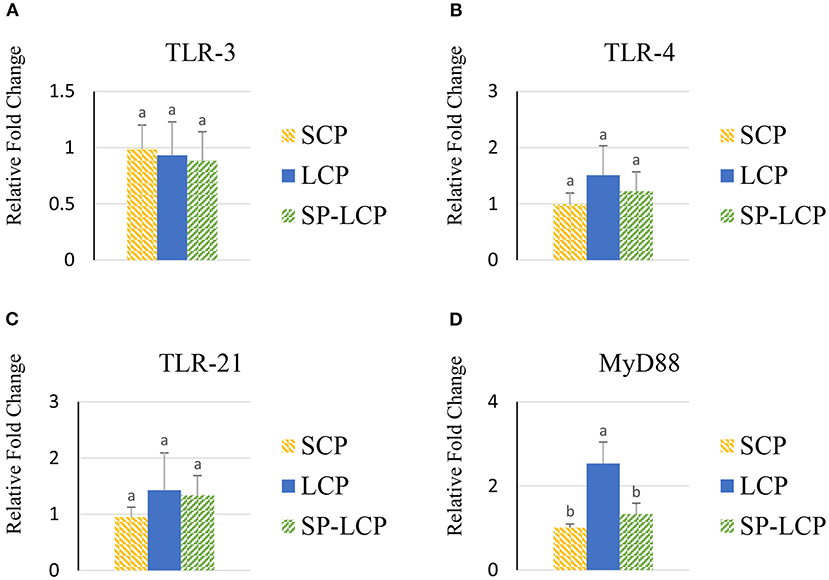
Figure 5. Effects of Spirulina on circulating toll-like receptor expression. Relative mRNA abundance of toll-like receptor 3 (TLR-3) (A), toll-like receptor 4 (TLR-4) (B), toll-like receptor 21 (TLR-21) (C), and myeloid differentiation primary response protein 88 (MyD88) (D) in the blood of broilers on day (d) 37 were determined by real-time qPCR. Treatments include standard corn/soy (SCP) diet as control and low crude protein without (LCP) or with Spirulina (SP-LCP). Data are presented as mean ± SEM (n = 8/group). Means with different letters are significantly different (P < 0.05).
The LCP diet upregulated the blood expression of glutathione peroxidase 1 GPx-1 but not that of GPx-3 or superoxide dismutase 1 and 2 (SOD-1/2) compared with the control (SCP) group (Figures 6A–D). The inclusion of Spirulina reduced GPx-1 mRNA abundance to the control level; however, it non-significantly increased further the expression of GPx-3 and SOD-2 genes (Figures 6A,B,D).
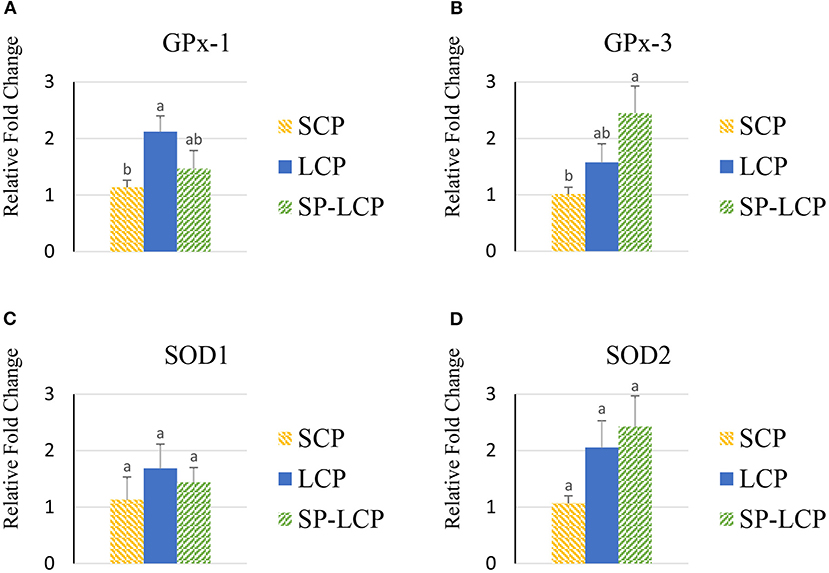
Figure 6. Effects of Spirulina on the expression of circulating antioxidant defense system. Relative mRNA abundance of glutathione peroxidase 1 (GPx-1) (A), glutathione peroxidase 3 (GPx-3) (B), superoxide dismutase 1 (SOD1) (C), and superoxide dismutase 2 (SOD2) (D) in the blood of broilers on day (d) 37 were determined by real-time qPCR. Treatments include standard corn/soy (SCP) diet as control and low crude protein without (LCP) or with Spirulina (SP-LCP). Data are presented as mean ± SEM (n = 8/group). Means with different letters are significantly different (P < 0.05).
Discussion
Inflammation is a response to stress and can occur in multiple levels of severity, from immediate acute responses to long-term chronic conditions. There is an intertwining relationship between inflammatory response and oxidative stress, in which inflammation elevates free radicals to levels exceeding cell threshold, thus inducing oxidative stress (26). Stress-induced inflammation can be associated with a plethora of factors, including dietary nutrient composition, bacterial or viral infection, environmental factors, obesity, aging, and numerous diseases (27–33).
There is limited information on how low CP diets and Spirulina impact inflammatory biomarkers in broiler chickens. It is important to remember that “low” or “high” CP level is relative regarding species, age, environment, and other factors. Excessive dietary protein intake can cause undigested protein or amino acids to enter the hindgut, increasing the amounts of opportunistic bacteria such as Clostridium perfringens in the ileum and ceca of broilers (34). Excess dietary nutrients/metabolites could cause metabolic (meta)-inflammation, which is different from pathological and physiological inflammation, and typically occurs in modern broilers production systems due to several factors such as ingredients used in the diet, nutrient excess in the diet, and high feed intake (35). All forms of inflammation lead to an upregulation of certain inflammatory biomarkers (36). However, the mechanisms mentioned earlier could not be the case in our study, as, surprisingly, the LCP diet led to an increase in basophil concentration and inflammation-associated biomarkers.
Basophil concentrations vary widely between avian species but are more highly concentrated in birds than their mammalian counterparts (37). Basophils are highly granular leukocytes that are chemo-attracted to inflammation sites and undergo degranulation to release histamine and Th2 cytokines and increase expression of toll-like receptors (38). The significant increase in basophil counts in our study indicates a systemic inflammation that might be a result of increased bacterial translocation that was observed in the LCP-fed birds. This is supported by an increase in the expression of inflammatory cytokines and chemokines, including IL-3, IL-4, IL-6, IL-10, CCL-20, and TNF-α in response to the LCP diet. The increase in liver bacterial translocation might be the underlying cause of the observed inflammatory stress. Reducing dietary CP can increase the broiler's intestinal permeability and leakage, which in turn induces bacterial translocation (39–41). Bacterial translocation increases pro-inflammatory cytokine production (42), which adversely impacts growth performance, as nutrients are directed toward immune responses rather than growth (43). This is evidenced in our experimental conditions by lower bodyweight, bodyweight gain, and a 15-point increase in feed conversion ratio in LCP-fed birds compared with their SCP counterparts in unpublished data.
It is worth noting that Kamely et al. (44) reported no differences in inflammatory cytokine TNF-α and IL-1β expression in the abdominal cavity exudate of Ross 708 broilers. The discrepancy between the present study and Kamely et al. (44) suggests that a low protein diet might affect the inflammatory system in a tissue-specific manner [systemic in our experimental conditions vs. local or abdominal cavity in Kamely et al. (44)]. The difference in the experimental approaches (diet formulation, amino acid levels, etc.) might also contribute to the discrepancy mentioned earlier.
Supplementation of Spirulina to LCP diets alleviated these negative effects and reduced inflammation as evidenced by amounts of IL-3, IL-4, IL6, IL-10, CCL-20, C3, and NLRP3 mRNA that were not different from those in the SCP control diet. This was in conjunction with a seven-point increase in feed conversion ratio in algae-LCP fed birds compared with LCP in unpublished data from this trial. Qureshi et al. (19) noted that Spirulina enhances the immune response in chickens fed a standard CP diet, and numerous trials have shown that algae ameliorate the expression of inflammatory molecular signatures in other species (45–47).
Inflammasome-forming NLRP3 and non-inflammasome-forming NLRs (NLRC3, NLRC5, and NLRX1) help mediate inflammatory responses (48), such as the one witnessed in the LCP-fed birds. The NLRs are activated in response to pathogen-associated molecular patterns and damage-associated molecular patterns; however, the exact underlying mechanisms for many NLRs in chicken have yet to be elucidated. In mammals, for instance, it is known that following microbial stimuli, NLR detects molecular patterns in the cytosol and activates the NLRP3 inflammasome, which in turn activates caspase 1, resulting in the production of IL-18 and IL-1β (49). Chen et al. (50) suggested that NLRP3 inflammasome activation occurred via the TLR2-MyD88-nuclear factor-kappa B (NF-κB) signaling pathway when chickens were challenged with Mycoplasma gallisepticum. The upregulation of MyD88 expression in this study supports the involvement of the previously described pathway. MyD88 acts as a downstream signaling adaptor for all TLRs except TLR-3 (51). MyD88 binds to TLRs and IL-1 receptor families to activate mitogen-activated protein kinases, activator protein 1, and NF-κB pathways (52). NF-κB plays an important role in producing pro-inflammatory cytokines after stress induced by gram-negative bacteria infection, reactive oxygen species, oxidized low-density lipoprotein, and multiple other factors (53).
Previous studies in mammals described several additional secondary signals, including plasma membrane disruption for bacterial toxins, lysosome destabilization, and liposome-triggering mitochondrial reactive oxygen species (ROS), leading to inflammasome oligomerization and activation (54–56). Although the mechanisms by which Spirulina reduces bacterial translocation and reverses the pro-inflammatory state induced by LCP are not known at this time point, it is conceivable that Spirulina might enhance gut integrity and merits further in-depth investigations. In addition to enhanced intestinal barrier integrity, Spirulina contains high levels (180 mg/g) of the biliprotein phycocyanin, which has been shown to have antioxidant, antibacterial, and anti-inflammation properties (57–60). Hao et al. (61) recently described a regulatory mechanism by which phycocyanin inhibits NF-κB expression and inflammation in human lung cancer cells through downregulating toll/IL-1 receptor domain-containing adaptor protein. Park et al. (17), on the other hand, showed that Spirulina modulates the antioxidant system in broilers. In our experimental conditions, Spirulina was also shown to modulate the systemic antioxidant defense system by lowering GPx-1 and upregulating GPx-3 and SOD-2, which is quite intriguing. GPx catalyzes the reduction of various hydroperoxides to H2O via oxidation of reduced GSH into its disulfide form (62). SOD2, also known as mitochondrial manganese-dependent SOD, transforms toxic superoxide (a byproduct of the mitochondrial ETC) into hydrogen peroxide and diatomic oxygen (62). The differential expression between GPx-1 and GPx-3 suggests a potential compensatory mechanism; in other words, GPx-3 expression was upregulated by Spirulina to compensate for the lack of GPx-1, which might be depleted due to elevations in ROS production. The upregulation of SOD2 could result from increased xanthophyll content or perhaps suggests enhanced cytoprotection and clearance of mitochondrial ROS by C-phycocyanin derived from Spirulina (63), which might explain at least partly the reduction of inflammation and cytokine expression. Spirulina also has the xanthophyll lutein, which can increase SOD2 irrespective of ROS levels (64). In support of our hypothesis, phycocyanin is considered a principal component responsible for antioxidant activity in algae by scavenging hydroxyl radicals (65). Furthermore, carotenoids such as β-carotene also protect cells from oxidative stress through quenching singlet oxygen damage (66).
In conclusion, this is the first report, to our knowledge, defining potential molecular targets by which Spirulina inclusion reduces systemic inflammatory activity, including cytokines, chemokines, bacterial translocation, and proportions of basophils in broilers fed a low protein diet.
Data Availability Statement
The original contributions presented in the study are included in the article/supplementary material, further inquiries can be directed to the corresponding author/s.
Ethics Statement
The animal study was reviewed and approved by the University of Arkansas Animal Care and Use Committee (#21002).
Author Contributions
GM, MK, WB, and SD: conceptualization. GM, GT-I, MK, and SD: methodology. GM, NE, and GT-I: software. GM, EG, NE, and SD: validation. GM, EG, GT-I, and GE: formal analysis. GM, EG, GT-I, MK, and SD: investigation. WB, MK, GT-I, GE, and SD: resources. GM, NE, and SD: data curation. GM: writing—original draft preparation. NE and SD: writing—review and editing. SD: visualization and supervision. WB, MK, and SD: project administration. WB, SD, and MK: funding acquisition. All authors contributed to the article and approved the submitted version.
Funding
Financial support was received from the United States Department of Agriculture and National Institute of Food and Agriculture, grant number #2019-69012-29905, as part of the Agriculture and Food Research Initiative—Sustainable Agricultural Systems.
Conflict of Interest
The authors declare that the research was conducted in the absence of any commercial or financial relationships that could be construed as a potential conflict of interest.
Acknowledgments
The authors would like to thank Pond Tech Inc. for supplying the Spirulina meal, as well as Kentu Lassiter, Alison Ferver, and Christine Vuong for assistance with sample collection, and the United States Department of Agriculture—Agricultural Research Service group, Center of Excellence for Poultry Science, the University of Arkansas—Fayetteville for access to the Cell-Dyn automated hematology analyzer.
References
2. European Parliament and of the Council - Regulation (EC) No 999/2001. Available online at: https://eur-lex.europa.eu/legal-content/en/TXT/PDF/?uri=CELEX:02001R0999-20130701&from=en (accessed November 5, 2020).
3. Fraps GS. Relation of protein, fat, and energy of the ration to the composition of chickens. Poult Sci. (1943) 22:421–4. doi: 10.3382/ps.0220421
4. Lemme A, Hiller P, Klahsen M, Taube V, Stegemann J, Simon I. Reduction of dietary protein in broiler diets not only reduces n-emissions but is also accompanied by several further benefits. J Appl Poult Res. (2019) 28:867–80. doi: 10.3382/japr/pfz045
5. Belloir P, Méda B, Lambert W, Corrent E, Juin H, Lessire M, et al. Reducing the CP content in broiler feeds: impact on animal performance, meat quality and nitrogen utilization. Animal. (2017) 11:1881–9. doi: 10.1017/S1751731117000660
6. Emmert J, Baker D. Use of the ideal protein concept for precision formulation of amino acid levels in broiler diets' description of problem. J Appl Poult Res. (1997) 6:462–70. doi: 10.1093/japr/6.4.462
7. Kidd MT, Tillman PB, Waldroup PW, Holder W. Feed-grade amino acid use in the United States: the synergetic inclusion history with linear programming. J Appl Poult Res. (2013) 22:583–90. doi: 10.3382/japr.2012-00690
8. Bryan D, Abbott D, Van Kessel A, Classen H. In vivo digestion characteristics of protein sources fed to broilers. Poult Sci. (2019) 98:3313–25. doi: 10.3382/ps/pez067
9. Bingol N, Dede S, Karsli M, Deger Y, Kiliçalp K, Çetin S. Effects of the replacement of soybean meal with pea as dietary protein source on the serum protein fractions of broilers. Braz J Poult Sci. (2016) 18:639–44. doi: 10.1590/1806-9061-2016-0270
10. Kocher A, Choct M, Porter MD, Broz J. The effects of enzyme addition to broiler diets containing high concentrations of canola or sunflower meal. Poult. Sci. (2000) 79:1767–74. doi: 10.1093/ps/79.12.1767
11. Altmann B, Wigger R, Ciulu M, Mörlein D. The effect of insect or microalga alternative protein feeds on broiler meat quality. J Sci Food Agri. (2020) 100:4292–302. doi: 10.1002/jsfa.10473
12. Becker EW. Micro-algae as a source of protein. Biotech Adv. (2007) 25:207–10. doi: 10.1016/j.biotechadv.2006.11.002
13. Toyomizu M, Sato K, Taroda H, Kato T, Akiba Y. Effects of dietary spirulina on meat colour in muscle of broiler chickens. Brit Poult Sci. (2001) 42:197–202. doi: 10.1080/00071660120048447
14. Fathi MA, Namra MM, Ragab MS, Aly MM. Effect of dietary supplementation of algae meal (spirulina platensis) as growth promotor on performance on performance of broiler chickens. Egypt Poult Sci. (2018) 38:375–89.
15. Bonos E, Kasapidou E, Kargopoulos A, Karampampas A, Christaki E, Florou-Paneri P, et al. Spirulina as a functional ingredient in broiler chicken diets. S Afr J Anim Sci. (2016) 4:695–102. doi.org/10.4314/sajas.v46i1.12
16. Gouveia L, Batista AP, Sousa I, Raymundo A, Bandarra NM. Microalgae in novel food products. Food Chem Res Dev. (2008) 2.
17. Park JH, Lee SI, Kim IH. Effect of dietary spirulina (arthrospira) platensis on the growth performance, antioxidant enzyme activity, nutrient digestibility, cecal microflora, excreta noxious gas emission, and breast meat quality of broiler chickens. Poult Sci. (2018) 97:2451–9. doi: 10.3382/ps/pey093
18. Uyisenga JP, Nzayino P, Seneza R, Hishamunda L, Uwantege K, Gasana N, et al. In vitro study of antibacterial and antifungal activity of spirulina platensis. Int J Ecol Dev. (2010) 16:80–8.
19. Qureshi MA, Garlich JD, Kidd MT. Dietary spirulina platensis enhances humoral and cell-mediated immune functions in chickens. Immunopharm Immunotox. (1996) 18:465–76. doi: 10.3109/08923979609052748
20. Shanmugapriya B, Babu SS, Hariharan T, Sivaneswaran S, Anusha MB. Dietary administration of spirulina platensis as probiotics on health and histopathology in broiler chicks. Int J Recent Sci Res. (2015) 6:2650–3.
21. Falquet J. The Nutritional Aspects of Spirulina. Available online at: https://www.antenna.ch/wp-content/uploads/2017/03/AspectNut_UK.pdf (accessed October 13, 2020).
22. Yang Y, Park Y, Cassada DA, Snow DD, Rogers DG, Lee J. In vitro and in vivo safety assessment of edible blue-green algae, Nostoc commune var. Sphaeroides Kutzing and spirulina platensis. Food Chem Toxicol. (2011) 49:1560–4. doi: 10.1016/j.fct.2011.03.052
23. Holman BWB, Malau-Aduli AEO. Spirulina as a livestock supplement and animal feed. J Anim Phys Anim Nut. (2013) 97:615–23. doi: 10.1111/j.1439-0396.2012.01328.x
24. Tellez G, Latorre JD, Kuttappan VA, Hargis BM, Hernandez-Velasco X. Rye affects bacterial translocation, intestinal viscosity, microbiota composition and bone mineralization in turkey poults. PLoS ONE. (2015) 10:e0122390. doi: 10.1371/journal.pone.0122390
25. Schmittgen TD, Livak KJ. Analyzing real-time PCR data by the comparative C (T) method. Nat Protoc. (2008) 3:1101–8. doi: 10.1038/nprot.2008.73
26. Surai PF, Kochish II, Fisinin VI, Kidd MT. Antioxidant defence systems and oxidative stress in poultry biology: An Update. Antiox. (2019) 8:235. doi: 10.3390/antiox8070235
27. Dal Pont GC, Farnell M, Farnell Y, Kogut MH. Dietary factors as triggers of low-grade chronic intestinal inflammation in poultry. Microorg. (2020) 8:139. doi: 10.3390/microorganisms8010139
28. Lu H, Adedokun SA, Adeola L, Ajuwon KM. Anti-inflammatory effects of eon-antibiotic alternatives in coccidia challenged broiler chickens. J Poult Sci. (2014) 51:14–21. doi: 10.2141/jpsa.0120176
29. Perez L. Acute phase protein response to viral infection and vaccination. Arch Biochem. (2019) 671:196–202. doi: 10.1016/j.abb.2019.07.013
30. Baxter MF, Greene ES, Kidd MT, Tellez-Isaias G, Orlowski S, Dridi S. Water amino acid-chelated trace mineral supplementation decreases circulating and intestinal HSP70 and proinflammatory cytokine gene expression in heat-stressed broiler chickens. J Anim Sci. (2020) 98:skaa049. doi: 10.1093/jas/skaa049
31. Ellulu MS, Patimah I, Khaza'ai H, Rahmat A, Abed Y. Obesity and inflammation: the linking mechanism and the complications. Arch Med Sci. (2017) 13:851–63. doi: 10.5114/aoms.2016.58928
32. Franceschi C, Bonafè M, Valensin S, Olivieri F, De Luca M, Ottaviani E, et al. Inflamm-aging. An evolutionary perspective on immunosenescence. Ann N Y Acad Sci. (2000) 908:244–54. doi: 10.1111/j.1749-6632.2000.tb06651.x
33. Panahi Y, Hosseini MS, Khalili N, Naimi E, Simental-Mendía LE, Majeed M, et al. Effects of curcumin on serum cytokine concentrations in subjects with metabolic syndrome: a post-hoc analysis of a randomized controlled trial. Biomed Pharma. (2016) 82:578–82. doi: 10.1016/j.biopha.2016.05.037
34. Drew MD, Syed NA, Goldade BG, Laarveld B, Van Kessel AG. Effects of dietary protein source and level on intestinal populations of clostridium perfringens in broiler chickens. Poult Sci. (2004) 83:414–20. doi: 10.1093/ps/83.3.414
35. Kogut MH, Genovese KJ, Swaggerty CL, He H, Broom L. Inflammatory phenotypes in the intestine of poultry: not all inflammation is created equal. Poult Sci. (2018) 97:2339–46. doi: 10.3382/ps/pey087
36. Khayyatzadeh SS, Kazemi-Bajestani SMR, Bagherniya M, Mehramiz M, Tayefi M, Ebrahimi M, et al. Serum high C reactive protein concentrations are related to the intake of dietary macronutrients and fiber: findings from a large representative Persian population sample. Clin Biochem. (2017) 50:750–5. doi: 10.1016/j.clinbiochem.2017.03.016
37. Maxwell MH, Robertson GW. The avian basophilic leukocyte: a review. World's Poult Sci J. (1995) 51:307–25. doi: 10.1079/WPS19950021
38. Yoshimoto T. The hunt for the source of primary interleukin-4: how we discovered that natural liller T cells and basophils determine T helper type 2 cell differentiation in vivo. Front Immunol. (2018) 9:716. doi: 10.3389/fimmu.2018.00716
39. Barekatain R, Nattrass G, Tilbrook AJ, Chousalkar K, Gilani S. Reduced protein diet and amino acid concentration alter intestinal barrier function and performance of broiler chickens with or without synthetic glucocorticoid. Poult Sci. (2019) 98:3662–75. doi: 10.3382/ps/pey563
40. Fouts DE, Torralba M, Nelson KE, Brenner DA, Schnabl B. Bacterial translocation and changes in the intestinal microbiome in mouse models of liver disease. J Hepatol. (2012) 56:1283–92. doi: 10.1016/j.jhep.2012.01.019
41. Benten D, Wiest R. Gut microbiome and intestinal barrier failure–the “Achilles heel” in hepatology? J Hepatol. (2012) 56:1221–3. doi: 10.1016/j.jhep.2012.03.003
42. Alexopoulou A, Agiasotelli D, Vasilieva LE, Dourakis SP. Bacterial translocation markers in liver cirrhosis. Ann Gastroenterol. (2017) 3:11–2. doi: 10.20524/aog.2017.0178
43. Emami NK, Calik A, White MB, Young M, Dalloul RA. Necrotic enteritis in broiler chickens: the role of tight junctions and mucosal immune responses in alleviating the effect of the disease. Microorg. (2019) 7:231. doi: 10.3390/microorganisms7080231
44. Kamely M, He W, Wakaruk J, Whelan R, Naranjo V, Barreda DR. Impact of reduced dietary crude protein in the starter phase on immune development and response of broilers throughout the growth period. Front Vet Sci. (2020) 7:436. doi: 10.3389/fvets.2020.00436
45. Gemma C, Mesches MH, Sepesi B, Choo K, Holmes DB, Bickford PC. Diets enriched in foods with high antioxidant activity reverse age-induced decreases in cerebellar beta-adrenergic function and increases in pro-inflammatory cytokines. J Neurosci. (2002) 15:6114–20. doi: 10.1523/JNEUROSCI.22-14-06114.2002
46. Chei S, Oh H, Song J, Seo Y, Lee K, Kim K, et al. Spirulina maxima extract prevents activation of the NLRP3 inflammasome by inhibiting ERK signaling. Sci. Rep. (2020) 10:2075. doi: 10.1038/s41598-020-58896-6
47. Yang Y, Du L, Hosokawa M, Miyashita K. Spirulina lipids alleviate oxidative stress and inflammation in mice fed a high-fat and high-sucrose diet. Mar Drugs. (2020) 18:148. doi: 10.3390/md18030148
48. Uchimura T, Oyama Y, Deng M, Guo H, Wilson JE, Rampanelli E, et al. The innate immune sensor NLRC3 acts as a rheostat that fine-tunes T cell responses in infection and autoimmunity. Immunity. (2018) 49:1049–61. doi: 10.1016/j.immuni.2018.10.008
49. Franchi L, Eigenbrod T, Muñoz-Planillo R, Gabriel Nunez. The inflammasome: a caspase-1-activation platform that regulates immune responses and disease pathogenesis. Nat Immunol. (2009) 10:241–7. doi: 10.1038/ni.1703
50. Chen C, Li J, Zhang W, Syed S, IShfaq M. Mycoplasma gallisepticum triggers immune damage in the chicken thymus by activating the TLR-2/MyD88/NF-κB signaling pathway and NLRP3 inflammasome. Vet Res. (2020) 51:52.
51. Akira S, Uematsu S, Takeuchi O. Pathogen recognition and innate immunity. Cell. (2006) 124:783–801. doi: 10.1016/j.cell.2006.02.015
52. Deguine J, Barton GM. MyD88: a central player in innate immune signaling. Prime Rep. (2014) 6:97. doi: 10.12703/P6-97
53. Hewlings SJ, Kalman DS. Curcumin: a review of its effects on human health. Foods. (2017) 6:92. doi: 10.3390/foods6100092
54. Zhong Z, Zhai Y, Liang S, Mori Y, Han R, Sutterwala FS, et al. TRPM2 links oxidative stress to NLRP3 inflammasome activation. Nat Commun. (2013) 4:1611. doi: 10.1038/ncomms2608
55. Pelegrin P, Surprenant A. Pannexin-1 couples to maitotoxin- and nigericin-induced interleukin-1B release through a dye uptake-independent pathway. J Biol Chem. (2007) 282:2386–94. doi: 10.1074/jbc.M610351200
56. Cassel SL, Joly S, Sutterwala FS. The NLRP3 inflammasome: a sensor of immune danger signals. Semin Immunol. (2009) 21:194–8. doi: 10.1016/j.smim.2009.05.002
57. Manconia M, Pendas J, Ledon N, Moreira T, Sinico C, Saso L, et al. Phycocyanin liposomes for topical anito-inflammatory activity: in-vitro in-vivo studies. J Pharm Pharmocol. (2009) 61:423–30. doi: 10.1211/jpp/61.04.0002
58. Sonani RR, Patel S, Bhastana B, Jakharia K, Chaubey MG, Singh NK, et al. Purification and antioxidant activity of phycocyanin from Synechococcus sp. R42DM isolated from industrially polluted site. Bioresour Tech. (2017) 245:325–51. doi: 10.1016/j.biortech.2017.08.129
59. Abd El-Baky HH, EL-Baroty GS. Characterization and bioactivity of phycocyanin isolated from spirulina maxima grown under salt stress. Food Funct. (2012) 3:381–8. doi: 10.1039/c2fo10194g
60. Liao G, Gao B, Gao Y, Yang X, Cheng X, Ou Y. Phycocyanin inhibits tumorigenic potential of pancreatic cancer cells: role of apoptosis and autophagy. Sci Rep. (2016) 6:345–64. doi: 10.1038/srep34564
61. Hao S, Li S, Wang J, Yan Y, Ai X, Zhang J, et al. Phycocyanin exerts anti-proliferative effects through down-regulating TIRAP/NF-κB activity in human non-small cell lung cancer cells. Cells. (2019) 8:588. doi: 10.3390/cells8060588
62. Li S, Yan T, Yang JQ, Oberley TD, Oberley LW. The role of cellular glutathione peroxidase redox regulation in the suppression of tumor cell growth by manganese superoxide dismutase. Cancer Res. (2000) 60:3927–39.
63. Li YJ, Han Z, Ge L, Zhou CJ, Zhao YF, Wang DH, et al. C-phycocyanin protects against low fertility by inhibiting reactive oxygen species in aging mice. Oncotarget. (2016) 7:17393–409. doi: 10.18632/oncotarget.8165
64. Okamoto T, Kawashima H, Osada H, Toda E, Homma K, Nagai N, et al. Dietary spirulina supplementation protects visual function from photostress by suppressing retinal neurodegeneration in mice. Trans Vis Sci Tech. (2019) 8:20. doi: 10.1167/tvst.8.6.20
65. Estrada JP, Besc'os PB, Del Fresno AV. Antioxidant activity of different fractions of Spirulina platensis protean extract. Il Farmaco. (2001) 56:497–500. doi: 10.1016/S0014-827X(01)01084-9
Keywords: broilers, Spirulina, low protein diet, inflammation, cytokines, chemokines, inflammasome
Citation: Mullenix GJ, Greene ES, Emami NK, Tellez-Isaias G, Bottje WG, Erf GF, Kidd MT and Dridi S (2021) Spirulina platensis Inclusion Reverses Circulating Pro-inflammatory (Chemo)cytokine Profiles in Broilers Fed Low-Protein Diets. Front. Vet. Sci. 8:640968. doi: 10.3389/fvets.2021.640968
Received: 12 December 2020; Accepted: 06 March 2021;
Published: 10 May 2021.
Edited by:
Michael Kogut, Agricultural Research Service, United States Department of Agriculture, United StatesReviewed by:
Sergio Luiz Vieira, Federal University of Rio Grande do Sul, BrazilWei Zhai, Mississippi State University, United States
Copyright © 2021 Mullenix, Greene, Emami, Tellez-Isaias, Bottje, Erf, Kidd and Dridi. This is an open-access article distributed under the terms of the Creative Commons Attribution License (CC BY). The use, distribution or reproduction in other forums is permitted, provided the original author(s) and the copyright owner(s) are credited and that the original publication in this journal is cited, in accordance with accepted academic practice. No use, distribution or reproduction is permitted which does not comply with these terms.
*Correspondence: Sami Dridi, dridi@uark.edu