- 1Istituto Zooprofilattico Sperimentale dell'Umbria e delle Marche “Togo Rosati”, Perugia, Italy
- 2Istituto Zooprofilattico Sperimentale del Piemonte Liguria e Valle d'Aosta, Torino, Italy
Paratuberculosis (PTB), also known as Johne's disease, is a chronic proliferative enteritis of ruminants caused by Mycobacterium avium subsp.paratuberculosis (MAP). To date, PTB diagnosis, based on serology, fecal culture, and real-time polymerase chain reaction, has identified animals in advanced stages of infection. To detect MAP infection in animals earlier, the interferon-gamma (IFN-γ) test may be applied. This assay detects cytokines produced by T-lymphocytes of infected subjects after stimulation with purified protein derivatives (PPDs), extracted from Mycobacterium bovis (MB) and from M. avium (MA). The study involved three bovine herds: one PTB-infected herd, one PTB-free herd, and one with an outbreak of bovine tuberculosis. The IFN-γ test was performed on 235 animals, using bovine PPD (PPDB), avian PPD (PPDA), and three experimental PPD Johnins (PPDJs) extracted from a synthetic liquid medium culture of MAP (PPDJ A, B, and C), to assess early MAP detection and avoid false reactions to MB. Furthermore, IFN-γ results were evaluated using 12 interpretative criteria (ICs), based on the differences and ratio between PPD optical density (OD) and IFN-γ basal OD values after lymphocytic stimulation. IC accuracy was expressed as area under the receiver operating characteristic curve. Through a longitudinal study, PPDJs proved to be specific and sensitive in the detection of MAP-infected animals. Among the evaluated ICs, six showed the best performance in terms of accuracy (p < 0.0001), highlighting PTB subclinical infections. In particular, the two best criteria reached sensitivity values of 100% [confidence interval (CI) 95%, 94.1–100%] with a specificity of 91.8% (CI 95%, 81.9–97.3%) and sensitivity levels of 80.6% (CI 95%, 69.1–89.2%) with a specificity of 100% (CI 95%, 94.1–100%). Thus, the IFN-γ assay proved to be a useful diagnostic tool to identify early subclinical MAP-infected animals, in order to manage infected cattle or those exposed to MAP and to monitor younger calves within a herd. Furthermore, the IFN-γ test can be considered an additional test to avoid the introduction of MAP-infected animals, especially in herds where disease has already been eradicated and preservation of the health status is required to maintain the PTB certification level.
Introduction
Mycobacterium avium subsp. paratuberculosis (MAP) is a slow-growing mycobacterium (1) and the causal agent of Johne's disease (JD) or paratuberculosis (PTB), a chronic inflammatory bowel disease seen in farmed ruminants and wildlife species worldwide (2, 3). Infected animals may shed MAP through their feces, and the live bacteria can survive in pastures (4) for a long time, representing a risk to other animals and even humans (5, 6). In fact, MAP may also act as a zoonotic agent in some human diseases (7–10). In particular, for more than a century, it has been associated with Crohn's disease, a chronic inflammatory bowel disease characterized by transmural inflammation and granuloma formation. Recently, other diseases have been associated with MAP, such as sarcoidosis, Blau syndrome, type 1 diabetes, Hashimoto's thyroiditis, and multiple sclerosis (9, 11–16). Regarding the transmission sources, MAP may contaminate food for human consumption, such as dairy and meat products, infant formula (17–19), and water (6, 20); and MAP seems to resist pasteurization treatment of milk at 72°C for 15 s [high-temperature, short-time (HTST) pasteurization] (21–23).
JD causes major economic losses to the global dairy industry due to reduced milk production (24), weight loss, infertility, pre-mature culling, and increased cow replacement costs (25, 26). The prevalence of MAP-infected farms in countries with advanced animal husbandry is growing rapidly and varies worldwide between 7 and 55% (27–29). Control programs to manage PTB in cattle and sheep herds have been adopted and in some cases re-adopted over the past couple of decades, in different countries, such as Australia, the USA, the Netherlands, Japan, and Denmark (28–32). The effectiveness of several recent control programs is yet to be demonstrated, since no countries have yet been able to eradicate the infection and there is no international agreement on PTB eradication plans (29, 30, 33).
A major problem in PTB control is the identification of animals in the early stages of MAP infection. The animals become most frequently infected at a young age, rarely in utero, but more often as newborns (34–36). The fecal–oral route is the main route of transmission, including the ingestion of contaminated feed, fodder, milk, and colostrum (35, 37, 38).
The initial host defense against MAP infection is mediated by the lymphocyte T-helper 1 (Th1) response, characterized by the production of IFN-γ and other pro-inflammatory cytokines (39–41). Nevertheless, MAP can use its evasion mechanisms and can survive by interfering with the host immune response (42). In particular, MAP is captured and processed by macrophages, in which mycobacteria can replicate and avoid phagolysosome maturation (43, 44). This step is followed by the activation of the cell-mediated immune response, which attempts to contain MAP infection (45). Humoral immune response, mediated by lymphocyte Th2, also appears in mycobacterial infection but generally only appears late when the disease is already established (46). This is an oversimplification of what happens in animals during MAP infection, since it has been demonstrated that an overlap between Th1 and Th2 responses could exist (47, 48). In the animals that “lose the battle against infection,” two or more years can pass before the appearance of the first clinical symptoms, and the evolution of the infection is not obvious in all subjects (49). Clinical disease is characterized by intermittent diarrhea, progressive weight loss, inappetence, and death (36, 45). The progression of the infection depends on both the containment action of innate and cell-mediated immunity (CMI) and is related to host genetics (50) and environmental factors. Therefore, PTB can be defined as a conditioned disease (43, 49, 51). The diagnosis of PTB, based on enzyme-linked immunosorbent assay (ELISA) for antibody detection, MAP fecal culture, and real-time quantitative polymerase chain reaction (qPCR) to detect MAP DNA, is particularly difficult (52). In fact, during the initial and subclinical stages, in MAP-infected animals, specific antibodies are absent or present at low levels, while MAP can be excreted intermittently at low concentrations (49, 53–55). When the infection advances, circulating antibodies gradually increase and are easily detectable by ELISA; however, they have no protective effect (46, 56, 57). Generally, animals that are positive for PTB ELISA are in advanced stages of infection; therefore, diagnostic tests based on measuring CMI, such as IFN gamma-release assays (IGRAs), could be more suitable for revealing subclinical stages of infection (48, 49, 57–62). IGRAs, which are used for the diagnosis of mycobacterial infections, consist of the quantification of IFN-γ released after mycobacterial antigen stimulation of peripheral blood lymphocytes. The test is performed using commercial ELISA kits to detect the amount of cytokines produced and secreted by T-cells of infected animals in culture supernatants (63, 64). The IFN-γ assay, developed in Australia in the late 1980s, is an in vitro blood test used as an ancillary test in combination with the skin test for the diagnosis of bovine tuberculosis (bTB) due to Mycobacterium bovis (MB) (63–67). Recently, it has also been used for the early diagnosis of PTB (60). As reported in the literature, the specificity (Sp) of the method varies from 67 to 94%, while the sensitivity (Se) varies from 13 to 85%, depending on the type and quantity of purified protein derivatives (PPDs) and, particularly, on the interpretative criteria (ICs) adopted for the tests (57, 58, 60). The antigens normally used during the stimulation phase of lymphocytes in the IFN-γ test are the traditional bovine and avian tuberculin PPDs extracted from MB AN5 (PPDB) and M. avium D4ER (PPDA), respectively. Johnin (PPDJ), similar to the other tuberculins, is a crude PPD obtained from a MAP culture in a synthetic liquid medium, inactivated by heat treatment, precipitated with trichloroacetic acid, and re-suspended in phenol and glycerin (57, 68).
In the present study, we used three different experimental PPDJs, which are produced in Italy at Istituto Zooprofilattico Sperimentale dell'Umbria e delle Marche “Togo Rosati” (IZSUM) and described by Corneli et al. (69).
The commercial diagnostic ELISA tests available today that are used for bTB have not been validated by the manufacturer for the diagnosis of PTB (57, 58). The interpretation of the values of secreted IFN-γ, expressed as absorbance values in optical density (OD) and relative cutoff points, is crucial for defining the outcome of the IFN-γ test and thus to clarify the state of mycobacterial infection in the animal (58, 70–74). However, there is a substantial difference between the use of the IFN-γ test for bTB diagnosis and the use of the IFN-γ test for PTB diagnosis. In the first case, the aim of the test is to detect the infected animal earlier than the skin test can (63) to facilitate prompt slaughter of the positive animals, according to the “test and cull” strategy. By contrast, in the case of PTB, the IFN-γ test still aims to identify the infected animal, but a different destination can be assigned to this animal depending on the prevalence of the disease in the herd. In a herd where the prevalence is low or PTB is not present, with an advanced health certification for PTB, the animal has to be removed from the herd. Conversely, in a herd with a high prevalence of PTB, animals with a positive reaction to the IFN-γ test for MAP infection diagnosis might be at risk of MAP shedding, and therefore, animals need to be checked more often than others. As reported by several authors (49, 75), these cattle could also include the animals able to contain the evolution of the MAP infection, without ever developing the subclinical and clinical forms of PTB, and therefore, they represent a genetic resource to be preserved and enhanced.
The aim of this longitudinal study was to evaluate the performance of three new experimental PPDJs and their potential application, in association with Italian PPDA and PPDB, in the IFN-γ test for the early detection of animals infected with MAP.
Materials and Methods
Production of Purified Protein Derivative Johnin
For the production of the new Italian Johnin, 20 Italian MAP strains were genotyped by amplification of mini- and microsatellite loci at the Italian National Reference Center for PTB (76). Two field strains, identified as strain A (used for PPDJA) and strain B (used for PPDJB), were selected based on their geographical distribution, growth characteristics, and protein yield. The MAP American Type Culture Collection (ATCC) strain 19698 represented strain C and was used for a third batch of Johnin (PPDJC) only as a production control and for methodical optimization. The three MAP strains were cultured for 4 months at 37°C in Watson–Reid modified broth, and then the bottles were autoclaved at 100°C for 3 h. The cells were removed, and the proteins were extracted by precipitation with trichloroacetic acid. The precipitate was then washed and dissolved in phosphate phenolate buffer and glycerine, with a final protein concentration of 1 mg/ml, as required for PPDB by the European regulation Annex B, Directive 64/432/EEC (77), and European regulation (EC) No. 1226/2002 (78).
Field Trial
Animal Population Characteristics and Ethics Statement
All samples were assessed as per the periodic tests required by the Italian National Health Programs (78–80) and during farmers' voluntary health controls for PTB, provided by the Italian National Guidelines (81).
A total of 235 cattle from farms in central Italy were enrolled in the study and divided into three groups, as follows:
• The first group consisted of 87 dairy cattle from three bTB Officially Free (OF) herds, where clinical cases of PTB had been reported.
• The second group consisted of 61 beef cattle from a bTB OF herd without PTB cases, and the herd had tested negative for serological tests in the last 4 years.
• The third group included 87 beef cattle from a bTB-positive herd with an ongoing outbreak when the study was performed.
Paratuberculosis Status Assessment
Each animal from the bTB OF herds with previous PTB cases or without PTB was assessed in parallel to traditional PTB tests, as follows:
• PTB ELISA test on serum (“ID Screen® paratuberculosis Indirect”—IDVet Innovative Diagnostics, Montpellier, France) in accordance with the manufacturer's instructions;
• MAP isolation on selective solid media following the OIE terrestrial manual (82);
• IS900 qPCR for MAP DNA detection from feces (83, 84) in fast mode (85).
As part of the longitudinal study, cattle were monitored for 4 years to check the evolution of their health status in relation to PTB, and animals were considered positive for PTB if at least one of the three tests (ELISA and/or qPCR and/or MAP culture) yielded a positive result.
Interferon-γ Assay and Interpretative Criteria
Whole Blood in vitro Stimulation and Interferon-γ Detection
Jugular blood samples were collected and delivered to the laboratory within 12 h at room temperature. The heparinized blood samples of each animal were dispensed in aliquots of 1 ml and stimulated, respectively, with phosphate-buffered saline (PBS 0.01 M, pH 7.2), used as a nil control antigen, which represented the IFN-γ basal value in the single animal (PBS); 10 μg of Italian PPDB and 10 μg of Italian PPDA; 20 and 10 μg of the three experimental PPDJs (strains A, B, and C, respectively), with two different dilutions 1:5 and 1:10; Pokeweed Mitogen (PWM; Thermo Fisher Scientific™, Waltham, MA, USA) included at a final concentration of 1 μg/ml, as a positive control of lymphocyte viability. IFN-γ secretion was evaluated using the Bovigam IFN-γ kit (Thermo Fisher Scientific™) in the plasma collected after 24 h of incubation at 37 ± 1°C in 5% CO2. The obtained values were expressed in units of OD measured at 450 nm (OD 450 nm).
Interferon-γ Interpretative Criteria
For the PTB IFN-γ performance evaluation, a comparison among PPDB, PPDA, and PPDJ was carried out, applying differences or ratios among the PPD OD values obtained. For each criterion, different cutoffs were applied to interpret the results. In particular, 12 possible ICs were adopted in the first and second groups to assess the presence or absence of MAP (MAP reactive or MAP negative) and to evaluate the stage of MAP infection. In the third group, to verify the test performance and reliability in the presence of another mycobacterial infection, three of the best criteria, chosen among those evaluated, were adopted. The ICs, shown in Table 1, were applied to all PPDJs (A, B, and C) at two dilutions (1:5 and 1:10) in association with Italian bovine and avian PPDs. Briefly, from the first to fifth criteria, we considered the difference between the PPDs OD values obtained after lymphocyte stimulation and the OD basal value with three different cutoff values (if PPDA or PPDJ – PBS > 0.05; 0.1; 0.2 = MAP infection) and twice the OD basal value at two different cutoff values (if PPDA or PPDJ – 2 * PBS > 0; 0.04 = MAP infection). From the sixth to ninth criteria, we considered the ratio between the PPD OD values obtained (PPDB or PPDA/PPDJ ≤ 0.09 = MAP infection; PPDJ/PPDB or PPDA > 1 = MAP infection). For the 10th criterion, we compared the ratio between PPDs (PPDB/PPDA > PPDB/PPDJ = MAP infection); and finally, for the 11th and 12th criteria, we considered the difference between PPDA and PPDJs and two different cutoff values (PPDJ – PPDA > 0.05; 0.1 = MAP infection).
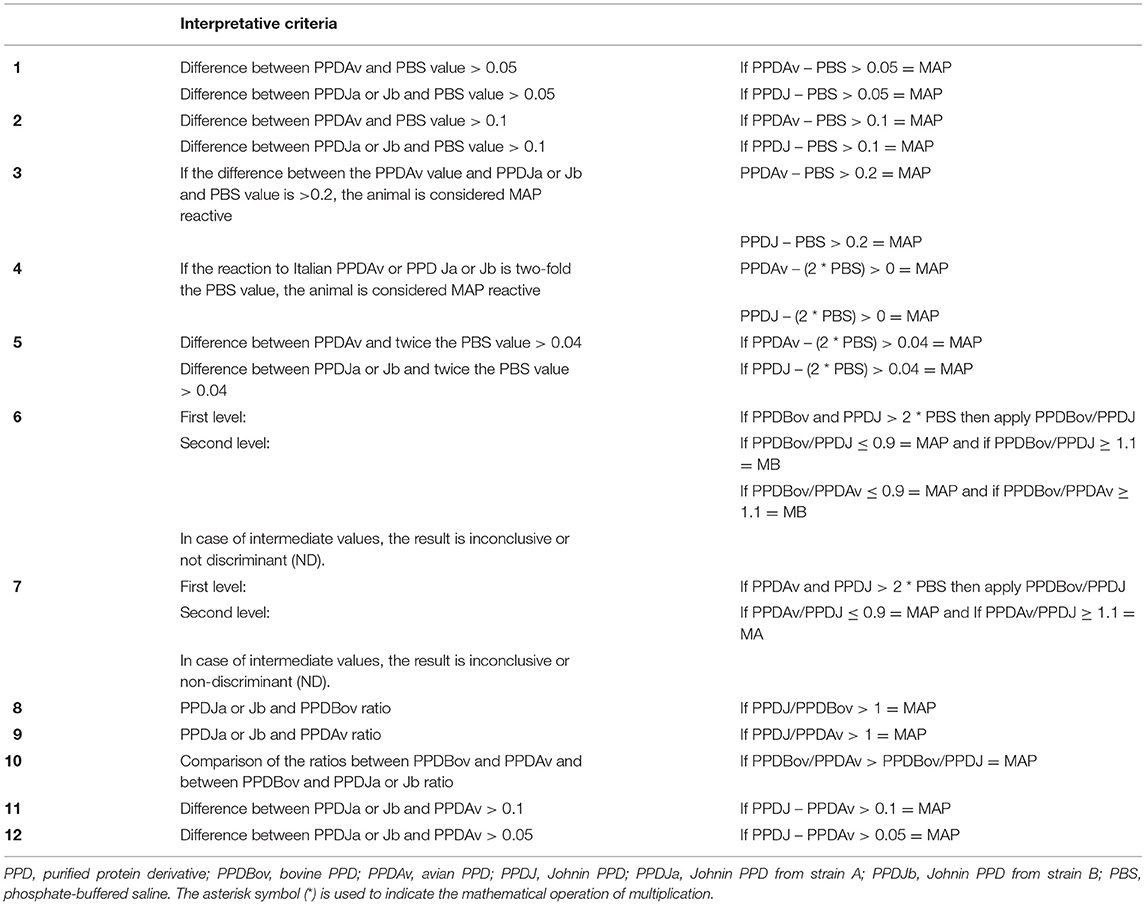
Table 1. Interpretative criteria of the IFN-γ test and cutoff values applied in the study for the diagnosis of Mycobacterium avium subsp. paratuberculosis (MAP) infected cattle.
For every criterion, a maximum threshold of the basal value (PBS ≤ 0.150 OD) was introduced to verify the eligibility of the sample. As reported in the literature (73, 86), this additional quality control is already used in the diagnosis of bTB to exclude animals with high basal values due to pre-existing pathologies or to avoid contaminated blood samples. This quality control (PBS ≤ 0.150 OD) was validated by the Istituto Zooprofilattico Sperimentale del Piemonte, Liguria e Valle d'Aosta Laboratory, with the sixth criterion applied to eradicate bTB in Piedmont from 2004 to 2016, to obtain European official tuberculosis-free status (87), and it is still being used (88–90).
The sixth criterion is currently also adopted in the IZSUM for the official diagnosis of bTB.
Statistical Analysis
The performance of the PPDJs in the 12 IFN-γ test ICs was evaluated on the OD values obtained from a total of 235 cattle, including 87 cattle from PTB affected herds, 61 cattle from a bTB OF herd, without PTB cases in the last 4 years, and another group of 87 cattle from a bTB-positive herd.
To establish the diagnostic accuracy and to compare the diagnostic efficiency of PPDJs, a receiver operating characteristic (ROC) curve analysis was performed. For each criterion, the Se, Sp, accuracy, area under the ROC curve (AUC), and Youden index were calculated. Differences in accuracy, Se, and Sp among the criteria were assessed using a binomial exact test.
The association statistics, the AUC with its standard error, and a confidence interval (CI) for each model were calculated. Differences between the AUC for PPDJA, PPDJB, and PPDA were performed by ROCCONTRAST statements using the non-parametric approach of DeLong (91).
For the purpose of the study, Se was defined as the proportion of samples with positive results from the expected true-positive animals, while Sp was defined as the proportion of samples with negative results to the expected true-negative animals.
The Se of PPDJ (A, B, and C) in the detection of MAP-infected subjects was calculated considering positive animals with a positive outcome in ELISA and/or qPCR and/or fecal culture. The Sp of PPDJ (A, B, and C) was calculated considering negative animals with a negative outcome in ELISA, qPCR, and fecal culture from the herd PTB-free for at least 4 years.
The PPDJ OD distribution was analyzed using histograms, and comparisons between dilutions and strains are shown in box plot graphs.
Two types of analysis of variance were performed using Proc generalized linear models and SAS software v. 9.2 to evaluate the “dilution factor” (1:5 and 1:10) and “strain factor” (A, B, and C) of the PPDJs, in particular if the PPDJ OD concentration values were different among the three strains and between the two dilutions.
Results
Paratuberculosis Status Assessment
Animals belonging to the three groups underwent traditional PTB tests: ELISA PTB, qPCR, and MAP isolation from feces (57). Each subject was considered positive for PTB if at least one of the three tests was positive.
In the first group, among the 87 cattle coming from bTB OF herds with previous PTB cases, 71 were positive for at least one of the traditional tests and 16 were negative to all the PTB tests. In particular, considering only the serological test, 68 subjects were positive, three were doubtful, and 16 were negative. Eighteen cattle were positive for real-time PCR from feces, and 28 were positive for MAP fecal culture. In the next 4 years, among the 16 subjects negative for the three PTB traditional tests at the first examination, five became positive during the follow-up (Supplementary Table 1). Specifically, one subject became positive for the culture test 3 months later, one was positive for ELISA and MAP isolation 10 months later, and two cattle became positive for ELISA (Supplementary Figure 1).
In the second group, among 61 cattle belonging to an OF bTB herd and without cases of PTB in the last 4 years, 100% of the animals were negative using conventional diagnostic tests.
The third group consisted of 87 cattle from a herd in which bTB positivity to the skin test was registered and then confirmed by the isolation of MB. In the first sampling of the study, the bTB outbreak was still present and PPDA reactivity was found in the comparative skin test. For this reason, the IFN-γ test and ELISA PTB test were performed in support of the official bTB diagnosis to avoid false-positive bTB outcomes in eventually PTB-positive animals. The herd was monitored for another 2 years until the bTB-free status was regained and all animals were negative in the two ELISA tests performed annually.
Evaluation of Purified Protein Derivatives Johnin Performance and IFN-γ Innovative Interpretative Criteria in the First and Second Groups
To evaluate the Se and Sp of the PPDJs using the different ICs, 128 animals were enrolled and followed up for 4 years. Among them, 67 from the first group were positive for at least one test for PTB, and 61 from the second group were always negative on the traditional tests for PTB. Table 2 shows the accuracy of the IFN-γ test according to the 12 ICs using PPDJA and PPDJB. Sp and Se values obtained with PPDJC are not shown because ATCC 19698 was used only for production control and method optimization.
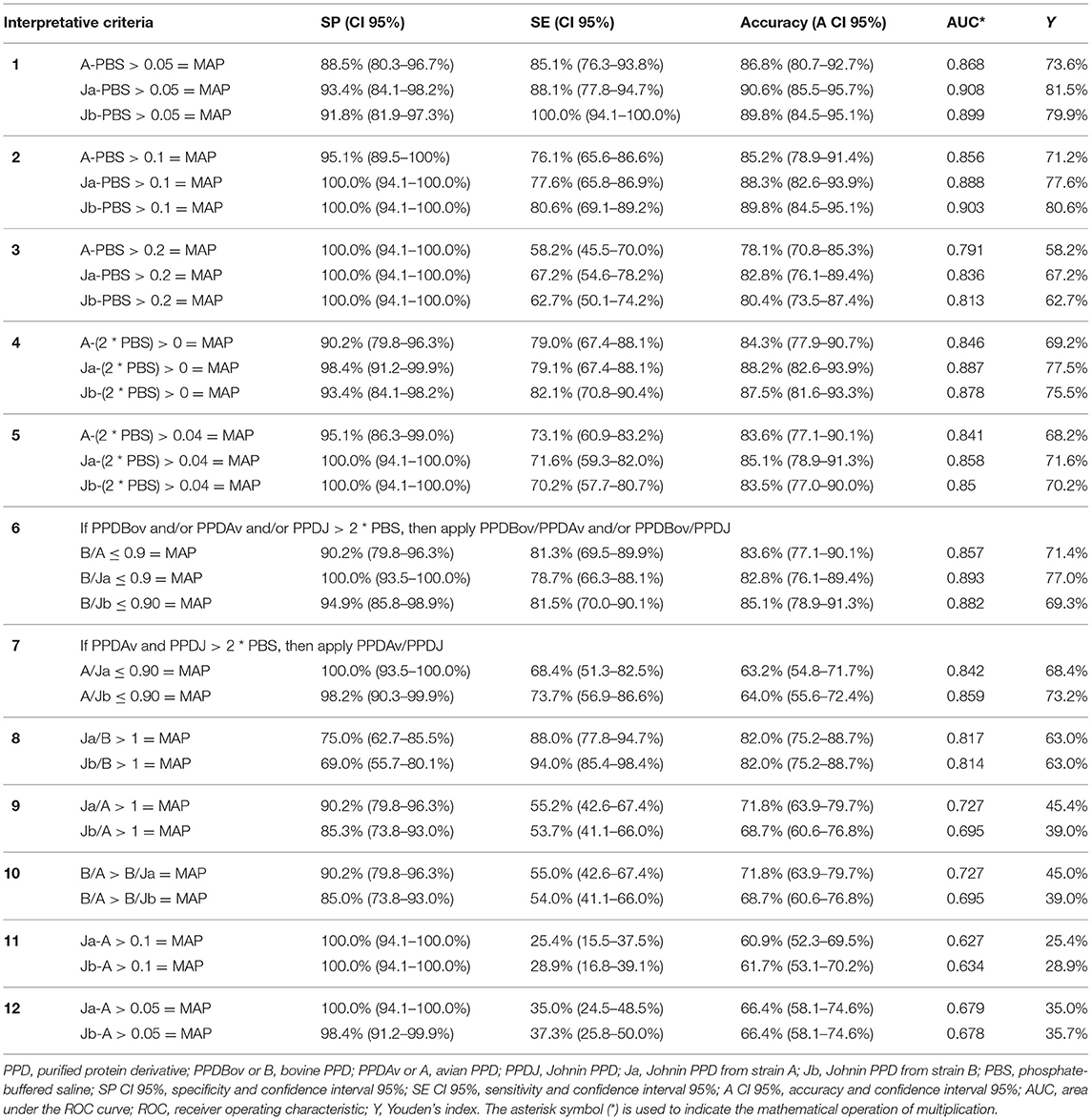
Table 2. Specificity and sensitivity values obtained with 12 interpretative criteria of the IFN-γ test and cutoff values applied in the study for the diagnosis of Mycobacterium avium subsp. paratuberculosis (MAP) infected cattle.
Out of 71 animals that tested positive for traditional PTB tests, four were considered outliers (PBS > 0.150 OD) and were therefore excluded from the performance evaluation.
The amount of IFN-γ produced by the PTB-positive subjects, in response to stimulation with the various PPDs, expressed in OD values, is represented graphically in Figure 1.
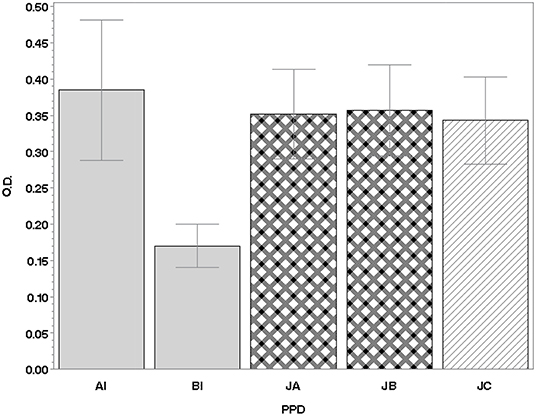
Figure 1. IFN-γ production in lymphocytes of 71 PTB-positive animals. Values are expressed as the mean of the differences between the OD450nm of PPDs and the OD450nm of PBS (±SD). IFN, interferon; PTB, paratuberculosis; PBS, phosphate-buffered saline; PPD, purified protein derivative; SD, standard deviation; OD, optical density; AI, Italian avian PPD; BI, Italian bovine PPD; JA, JB, JC, Johnins produced by the three strains of MAP: A and B (field strains) and C [strain American Type Culture Collection (ATCC) 19698].
Regarding the assessment of PPDJ efficiency, analysis of variance showed no statistically significant differences between the mean OD 450 nm of the PPDJs and the dilution factor (F-test = 1.61; p = 0.2060) (Figure 2A) or for the strain factor (F-test = 0:37; p = 0.6907) (Figure 2B).
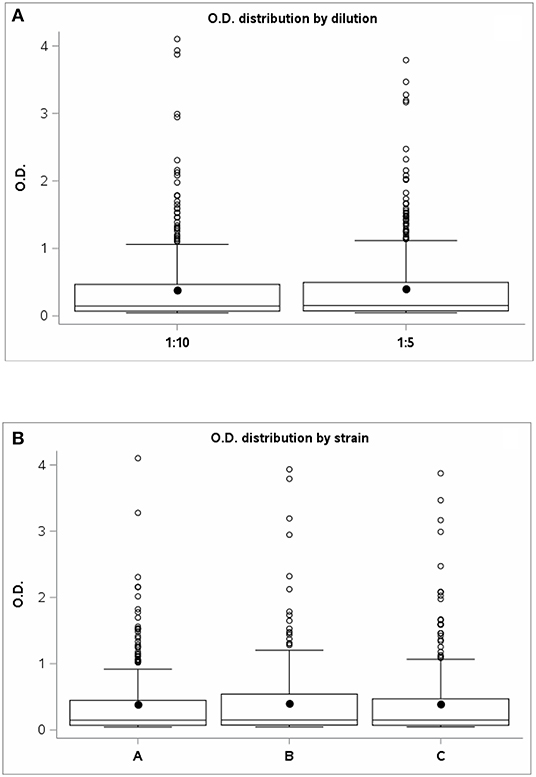
Figure 2. The graph shows the OD values distribution of PPD Johnins (A) for the two dilutions: 1:5 and 1:10 and (B) for strains A, B, and C. OD, optical density; PPD, purified protein derivative.
As shown in Table 2, among the 12 applied ICs, the PPDJs achieved the best performance within the first six ICs, with values of accuracy ranging from 90.6% (CI 95%: 85.5–95.7%) to 80.4% (CI 95%: 73.5–87.4%). The accuracy of the first six criteria was higher than that of the last six criteria, and the difference was statistically significant (binomial exact test, p <0.0001). The first criterion achieved a higher accuracy for criteria 3, 4, 5, and 6; and the difference was statistically significant for each criterion (binomial exact test, p <0.05). No statistically significant differences were observed between the accuracy of the first and second ICs; however, there were differences between the Se and Sp values. In particular, as shown in Table 2, PPDJB with the first criterion achieved better Se values (binomial exact test, p = 0.02; Se 100.0% CI: 94.1–100.0%), while PPDJB with the second criterion achieved the best Sp values (binomial exact test, p <0.0001; Sp 100.0% CI: 94.1–100.0%), but the accuracy was the same (89.8% CI: 84.5–95.1%).
Regarding the comparison of the diagnostic accuracy of PPDJs vs. PPDA, within the same criterion, statistically significant differences were observed in the second criterion (binomial exact test, p = 0.0397). In addition, in the second criterion, the Sp of PPDJs was higher than that of PPDA, and the difference was statistically significant (binomial exact test, p = 0.0381).
Results of ROC analysis obtained with PPDA, PPDJA, and PPDJB according to the first three criteria are shown in Figure 3 and in Supplementary Tables 2, 3. Regarding the comparison between AUC of PPDJs and AUC of PPDA, the differences were statistically significant; in particular, the AUCs of PPDJA and of PPDJB were higher than those of PPDA, with a p < 0.001 and p < 0.05, respectively. No difference was observed between AUC of PPDJA and PPDJB, since DeLong's test for two correlated ROC curves was statistically not significant (p > 0.05).
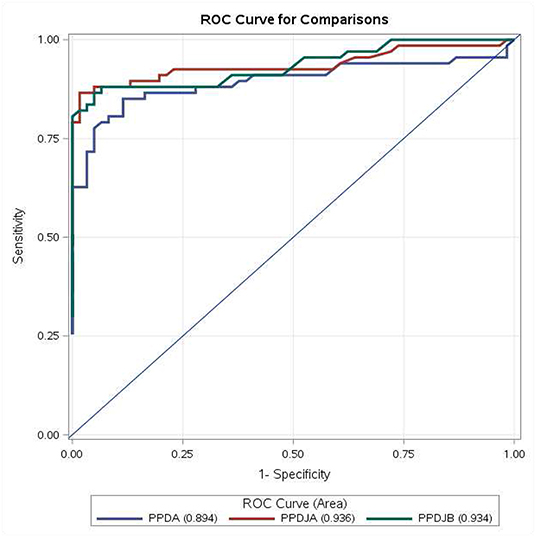
Figure 3. ROC curve for comparisons of PPDA-PBS, PPDJA-PBS, and PPDJB-PBS according to the first three interpretative criteria of the IFN-γ test applied in the study for the diagnosis of Mycobacterium avium subsp. paratuberculosis (MAP)-infected cattle. PPDJA and PPDJB showed higher AUC than PPDA, and the difference is statistically significant (p < 0.05). ROC, receiver operating characteristic; IFN, interferon; PPD, purified protein derivative; PPDA, avian PPD; PPDJA, PPD Johnin strain A; PPDJB, PPD Johnin strain B; AUC, area under the ROC curve.
As shown in Table 2, the IC that showed the best performance in terms of Se and Sp was derived from a comparison between the OD value obtained after PPD stimulation and the OD value of basal IFN-γ of each animal (PBS) (from the first to fifth criteria). All the ICs based on the simple difference or ratio between OD values relative to PPDs (from the eight to 12th criterion), not considering the baseline value of IFN-γ (OD value of PBS), elicited the worst results. The comparison between the criteria that provided difference and ratio showed that the criteria based on PPDs OD ratio, such as the sixth and seventh criteria, yielded numerous inconclusive outcomes (ND) for values in the cutoff range, despite good results in terms of Se and Sp. Furthermore, all the ICs that provided a difference or ratio between the PPDJs and the PPDA showed inefficacy, especially in terms of Se, with values ranging from 25.4% (CI 95%: 15.5–37.5%) to 55.2% (CI 95%: 42.6–67.4%).
Evaluation of Purified Protein Derivatives Johnin Performance and IFN-γ Innovative Interpretative Criteria in the Third Group
In the third group, based on the data obtained from the application of the 12 criteria in the first and second groups on positive and negative PTB animals, respectively, the criteria with the best performance were used. In particular, in a herd with an ongoing bTB outbreak, the criteria that reported Sp values of 100% (second, third, and fifth ICs) were adopted with the aim of verifying the Sp of PPDJs.
Out of 87 animals that were negative to ELISA PTB tests, seven subjects were considered outliers (PBS > 0.150 OD) and therefore excluded from the performance evaluation. As shown in Table 3, for the second criterion (if PPDJ – PBS > 0.1 = MAP), the PPDJA at 1:10 dilution reached 95.0% Sp (CI 95%: 87.69–98.62%) and the PPDJB at 1:10 dilution provided 87.50% Sp (CI 95%: 78.21–93.84%). In the third criterion (if PPDJ – PBS > 0.2 = MAP), PPDJA at 1:10 dilution provided 100.0% Sp (CI 95%: 95.49–100.0%) and 95.0% Sp for PPDJB (CI 95%: 87.69–98.62%). Finally, in the fifth criterion (if PPDJ – 2 × PBS > 0.04 = MAP), the PPDJA at 1:10 dilution reached 96.25% Sp (CI 95%: 89.43–99.22%) and PPDJB reached 91.25% Sp (CI 95%: 82.80–96.41%). With the third criterion, PPDJA reached Sp values higher than those of the other two ICs (binomial exact test, p < 0.0001) and PPDA (binomial exact test, p = 0.0006).
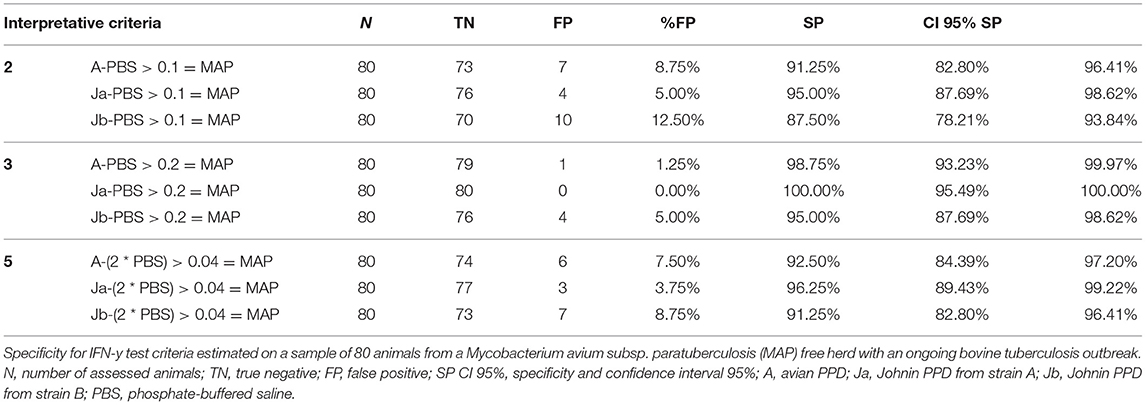
Table 3. Specificity values obtained in the 3rd group of animals, adopting the 2nd, 3rd, and 5th interpretative criteria of the IFN-γ test.
Discussion
The IFN-γ test, in association with the tuberculin skin test, is used in many European countries for bTB diagnosis (63, 64). Both methods show the CMI response of the infected animals following stimulation with mycobacterial antigens, PPDB and PPDA (58, 63, 82). The immunologic evaluation to identify animals infected with MB is often limited by cross-reactions observed in animals exposed to other species of mycobacteria, particularly those belonging to the Mycobacterium avium complex (MAC), mainly MAP (86, 92).
In a single intradermal comparative cervical tuberculin (SICCT) test and in the IFN-γ release assay, a reaction to PPDA can identify animals affected by PTB (58, 82, 93–95). For this reason, in cattle, the IFN-γ test has also been used recently for the early diagnosis of PTB, although the Sp of the method varies from 67 to 94%, depending on the type and amount of PPDs used, and particularly on the IC of the test (57, 60).
With the aim of producing new batches of PPDJ obtained from the field strains of MAPs common in our territory and to develop a more sensitive and specific IFN-γ test for the early diagnosis of MAP infection, three experimental PPDJs were produced at IZSUM, and different IFN-γ ICs were evaluated.
From the first contact with mycobacteria, the immune response is characterized by a complex series of events aimed at controlling the infection before it can compromise homeostasis in the organism (45). In this context, the secretion of pro-inflammatory cytokines, such as IFN-γ, is involved in the containment of infection caused by mycobacteria (48) and can be detected only by the IFN-γ test. However, the humoral immune response, detectable by ELISA, appears only in the late stage when the disease is clinically manifested (60). The animals become infected at a young age, but the clinical PTB form does not occur until 2–3 years, probably because of the control role of innate and CMI, genetic susceptibility of the animal, and environmental factors (45, 47, 48, 50, 75). Therefore, it is strategically advantageous to decide in which categories include animals with a positive reaction to the IFN-γ test and tested negative to traditional tests for MAP infection diagnosis (49, 75). The production of IFN-γ by lymphocytes, after stimulation with PPDs, indicates an “immunological memory” and therefore a previous contact with a mycobacterium, and in the case of the PTB, the presence of a MAP infection. Thus, as an oversimplification, an IFN-γ-positive reactor is a MAP-infected animal that is probably in the stage of infection in which the animal, through CMI, keeps the pathogen under control and avoids its spread, as reported by recent studies (44). At this stage of infection, the animal, even if infected, does not yet eliminate MAP with feces and does not present any antibodies. To date, it is not predictable how long the animal will remain in this stage of infection. Certainly, in a herd with a high PTB prevalence, an IFN-γ-positive reactor could be a possible future MAP shedder and will therefore need to be checked more often than other cattle. However, if this IFN-γ-positive reactor will never test positive in conventional tests, it could be a subject able to contain the MAP infection, which will never develop the disease. It will be an important challenge for future studies to understand whether these animals with these characteristics will be identified as resistant or resilient PTB cattle (49, 75).
As stated earlier, the “infected” animals that do not yet shed MAP and do not yet show clinical signs are the most difficult category to identify with ELISA, fecal culture, and qPCR, due to low antibody production and low or absent MAP shedding. In addition, the “subclinical infected” animals may contaminate the environment, but their detection by serological test and the direct MAP identification has lower probability of success, representing a significant challenge for the control and management of PTB (57). “Affected” animals often present a clinical form and shed large quantities of MAP, representing the main source of infection, and are frequently positive in serological tests and can be easily detected by MAP isolation and biomolecular approaches.
In the present study, through a 4-year follow-up, it was possible to define the different stages of MAP infection in cattle of the first group from three PTB-affected herds, using new Italian Johnins in the IFN-γ assay. The obtained data represent the first assessment of the performance of experimental Italian PPDJs in the IFN-γ test and the first evidence of their ability to detect MAP-infected animals by adopting different ICs.
The critical aspect of this study was the comparison between two different diagnostic approaches, one based on the IFN-γ test and the other based on traditional tests (ELISA, fecal culture, and qPCR). The first approach is able to detect animals in the preliminary stages of MAP infection, while the second is useful when affected subjects are in the advanced stages of the disease or already have the clinical form of PTB. Therefore, the Se and Sp of the IFN-γ assay are related to the tests adopted to define the “positive animal.” These parameters are calculated on the basis of tests applied at the different stages of infection, in animal producing antibodies against MAP and/or in animal shedding MAP, while the IFN-γ test reveals infected animals that generally do not yet produce antibodies and do not yet shed MAP in their feces. This may have a negative effect on the performance of the IFN-γ test.
Simultaneously to the performance evaluation of the new PPDJs, different criteria for the PTB IFN-γ test interpretation were developed and compared, particularly IC based on the difference between OD values and IC based on the OD ratio (Table 1). Moreover, different cutoff values have been applied to each criterion, some of which are often used for the diagnosis of bTB in cattle and buffalo (72, 92, 96).
The PPDJs achieved the best performance within the first six ICs, among the 12 applied ICs (Table 2), with values of accuracy ranging from 90.6% (CI 95%: 85.5–95.7%) to 80.4% (CI 95%: 73.5–87.4%). In particular, the accuracy achieved by the PPDJs adopting the first six criteria resulted in a higher accuracy than that using the last six criteria, and the difference was statistically significant (binomial exact test, p < 0.0001). In addition, both PPDJs with the first criterion achieved a higher accuracy related to values reached adopting IC from the third to sixth, and the difference was statistically significant for each criterion (binomial exact test, p < 0.05).
From the perspective of the field use of PPDJs, we wanted to identify the IC that would maximize their performance, both in terms of Se, as in the case of the first criterion (PPDJB Se 100.0%, CI: 94.1–100.0%), and in terms of Sp, as in the case of the second IC (PPDJs Sp 100.0%, CI: 94.1–100.0%). In fact, even though no statistically significant differences were observed between the first and second ICs, in terms of accuracy, PPDJB with the first criterion achieved better Se values (binomial exact test, p = 0.02), while PPDJB with the second criterion achieved the best Sp values (binomial exact test, p < 0.0001), despite the accuracy being equal. As stated before, the Sp and Se are greatly affected by the tests used to detect the positive animal. On the basis of the six best ICs, in the first group, among 16 subjects with negative outcomes in the three PTB conventional tests, six were IFN-γ positive. The follow-up allowed monitoring of PTB progression, and five animals became PTB positive to traditional tests. In particular, two animals that reacted to both PPDA and PPDJs had PTB-positive bacterial culture 3 months later and also to the ELISA 10 months later. Among the three bovines reactive only to PPDJs, two animals became ELISA-positive 6 months later and a one bovine 18 months later. In the first step, these animals were considered “false positive” by statistical analysis, but the IFN-γ test with the six best ICs detected and unveiled MAP-infected cattle earlier than did the other traditional tests (Supplementary Table 1 and Supplementary Figure 1).
In terms of the mean IFN-γ production following stimulation with PPDJs and PPDA, there were no statistically significant differences (Figure 1) except in the second criterion, where the Sp of PPDJs was higher than the Sp of PPDA, and the difference was statistically significant (binomial exact test p = 0.0381). However, the analysis of the ROC curves of the PPDJA, PPDJB, and PPDA, according to the first three criteria (Figure 3 and Supplementary Tables 2, 3), shows that there were no statistical difference between AUC of PPDJA and of PPDJB, since DeLong's test for two correlated ROC curves was statistically not significant (p > 0.05). Instead, it is important to highlight that AUC of PPDA was different from PPDJA and PPDJB and that the difference was statistically significant (p < 0.05).
This result can be explained since MAP is part of the MAC; therefore, the remarkable similarities between PPD extracted from MAP and PPD extracted from MA do not permit the use of one against the other. However, in the criteria where both PPDJs and PPDA were used (Table 2), the first ones reached better values in terms of accuracy and proved to be more specific, particularly when used with the second criterion. Moreover, the comparison between the two PPDJs did not reveal statistically significant differences, even if the PPDJB seemed to be more sensitive and the PPDJA seemed more specific (Table 2, first and second ICs). These aspects, related to the performance of PPDJs and the robustness of IC validation, could be improved by enrolling more animals in future investigations. In addition, a further evolution of our study could be the use of recombinant antigens, peptides, or proteins from MAP, to increase the Sp of the IFN-γ test (97). These antigens have already been widely used in the IFN-γ test for the diagnosis of bTB in bovines (98, 99) and buffalo (92), and the most widely used antigens are ESAT6/CFP10, or other antigen cocktails, with the aim of increasing the Sp of the IFN-γ test. In the literature, the results obtained with recombinant proteins of MAP have not been completely satisfactory (100). Nevertheless, we are evaluating possible candidates for future inclusion in the lymphocyte stimulation phase of the IFN-γ test, such as the more promising MAP2698C (62) and MAP0586C (101) proteins. However, it should be noted that our experimental PPDJs achieved Sp values of 100%, without compromising Se values and therefore maintaining high accuracy values, when the second, third, and fifth ICs were adopted (Table 2) and when used with the third criterion in a bTB outbreak (Table 3). However, recombinant antigens tend to favor Sp but penalize Se because of their high discriminating power.
Although valid criteria were highlighted in this study, in particular the first and the second, in our opinion, the main aspect is the adoption of different criteria in relation to the PTB status in each farm. In particular, it is advisable to use those criteria with a major Se, such as the first criterion, in herds with a high prevalence of PTB; on the contrary, apply more specific IC, such as the second criterion, in herds with low PTB prevalence, similar to the protocol described by Keck et al. (73) used in France during the bTB eradication plans for cattle from 2003 to 2014.
In this regard, it was of great interest to include in the experiment a particularly problematic herd, the third enrolled group, which consisted of animals from a herd with a bTB outbreak at the time of the survey. In addition, animals have always been brought to pasture in the summer months; hence, they are subject to infections due to atypical mycobacteria, particularly those belonging to the MAC. Therefore, in these animals, non-specific reactions in the PTB IFN-γ test related to possible cross-reactions to MB and MAC were predictable. In these cases, it was useful to assess the Sp of PPDJs to avoid false-positive outcomes for MAP in animals that have always been negative on ELISA tests for PTB. In particular, in this third group, with the aim of verifying the Sp of PPDJs, the second, third, and fifth ICs were adopted because they reached Sp values of 100% (CI 95%: 94.1–100.0%) in the other groups. PPDJA achieved Sp values of 100% (CI 95%: 95.49–100%) when adopted in the third criterion, and the difference with other ICs was statistically significant (binomial exact test, p < 0.0001). Always regarding the third criterion, PPDJA has shown higher Sp than PPDA, and the difference was statistically significant (Binomial exact test, p = 0.0006). Other authors (100) included a group of animals from a bTB outbreak in their assessment of the efficiency of the IFN-γ test for PTB diagnosis and did not find the same performance achieved in the present study. The authors concluded that the IC that they used led to several inconclusive results, and the use of a PPDJ would have resulted in a more precise classification of the animals within the bTB outbreak. Therefore, the use of the PPDJs investigated in the present study and the IC with the best performance could be recommended in herds where bTB and PTB co-infection is suspected, a scenario that could be an additional challenge for us.
In conclusion, the use of PPDJs and the interpretation of the IFN-γ test with the first or second criterion achieved high performance in the identification of MAP-infected cattle. Therefore, it can be assumed that the IFN-γ test could be a useful tool for identifying early subclinical MAP-infected animals, managing cattle infected or exposed to MAP, and monitoring younger calves within a herd.
Conclusions
The IFN-γ test should be used for the early diagnosis of MAP infection, and it can be efficiently used to detect pre-maturely MAP-infected subjects within a herd. Considering that infected cattle may never shed MAP or show clinical signs of PTB, this would allow veterinarians and farmers to decide together about the future of infected animals. The future of the animal, that is, to keep or to cull, must be considered carefully based on PTB prevalence on a farm. In particular, in herds with high PTB prevalence, culling IFN-γ positive reactors could mean eliminating animals that are “controlling” the infection, and paradoxically these animals may be “resistant” to the disease, and as such, should be kept in the herd.
On the contrary, in herds with low PTB prevalence or that are PTB-free, an animal that reacts positively to the IFN-γ test is definitely an animal that has been exposed to MAP or has contracted the infection; therefore, it has to be removed in order to maintain the low PTB or PTB-free status in the herd. Furthermore, the IFN-γ test can be considered as an additional test for animals that may be admitted to the herd, to avoid the introduction of MAP-infected subjects, especially in herds that have already eradicated the disease. Hence, the IFN-γ test will provide an additional diagnostic tool that farmers could adopt voluntarily to reach and preserve health status certification regarding PTB.
Data Availability Statement
The raw data supporting the conclusions of this article will be made available by the authors, without undue reservation.
Author Contributions
PM: conceptualization. SC, ADi, CS, LC, PP, SCo, NV, ADo, and PM: methodology. NV: software. SC, ADi, and PM: validation. NV, LP, CS, MCi, LC, and PM: formal analysis. SC, ADi, CS, MCi, LC, MB, PP, SCo, and PM: investigation. SC, ADi, MB, ADo, and PM: resources. SC, ADi, NV, and PM: data curation. SC, ADi, MT, LP, and PM: writing original draft preparation. SC, ADi, MT, LP, MCa, and PM: writing review and editing. SC, NV, ADi, LP, and PM: visualization. SC, ADi, NV, MT, LP, MCa, ADo, and PM: supervision. All authors contributed to the article and approved the submitted version.
Funding
The present study was supported by the Italian Ministry of Health, RC IZSUM 011/2008, RC IZSUM 04/2011 research projects. LP was funded by a grant from RC IZSUM 06/2019 research project, financed by the Italian Ministry of Health.
Conflict of Interest
The authors declare that the research was conducted in the absence of any commercial or financial relationships that could be construed as a potential conflict of interest.
Acknowledgments
The authors would like to thank the Italian National Reference Center for Paratuberculosis, in particular Dr. Norma Arrigoni and Dr. Matteo Ricchi, for the genotyping of MAP field strains; the veterinary services of the Marche region; and Dr. Marco Ermini, Dr. Luigi Bonifazi, Dr. Martina Sebastianelli, and Dr. Piermario Mangili of IZSUM for their support in the field trials. Finally, a special thanks to Mrs. Angela Caporali for her excellent technical support. We would like to thank the reviewers for the useful observations and comments that enabled us to improve the manuscript quality, contributing to its better understanding.
Supplementary Material
The Supplementary Material for this article can be found online at: https://www.frontiersin.org/articles/10.3389/fvets.2021.638890/full#supplementary-material
References
1. Lambrecht RS, Carriere JF, Collins MT. A model for analyzing growth kinetics of a slowly growing Mycobacterium sp. Appl Environ Microbiol. (1988) 54:910–6. doi: 10.1128/AEM.54.4.910-916.1988
2. Chiodini RJ, Van Kruiningen HJ, Merkal RS. Ruminant paratuberculosis (Johne's disease): the current status and future prospects. Cornell Vet. (1984) 74:218–62.
3. Harris NB, Barletta RG. Mycobacterium avium subsp. paratuberculosis in veterinary medicine. Clin Microbiol Rev. (2001) 14:489–512. doi: 10.1128/CMR.14.3.489-512.2001
4. Fecteau ME, Whitlock RH, Buergelt CD, Sweeney RW. Exposure of young dairy cattle to Mycobacterium avium subsp. paratuberculosis (MAP) through intensive grazing of contaminated pastures in a herd positive for Johne's disease. Can Vet J. (2010) 51:198–200.
5. Garvey M. Mycobacterium avium subspecies paratuberculosis: a possible causative agent in human morbidity and risk to public health safety. Open Vet J. (2018) 8:172–81. doi: 10.4314/ovj.v8i2.10
6. Mazzone P, Corneli S, Di Paolo A, Maresca C, Felici A, Biagetti M, et al. Survival of Mycobacterium avium subsp. paratuberculosis in the intermediate and final digestion products of biogas plants. J Appl Microbiol. (2018) 125:36–44. doi: 10.1111/jam.13762
7. Hermon-Taylor J. Mycobacterium avium subspecies paratuberculosis, Crohn's disease and the Doomsday scenario. Gut Pathog. (2009) 1:15. doi: 10.1186/1757-4749-1-15
8. Wynne JW, Bull TJ, Seemann T, Bulach DM, Wagner J, Kirkwood CD, et al. Exploring the zoonotic potential of Mycobacterium avium subspecies paratuberculosis through comparative genomics. PLoS ONE. (2011) 6:e22171. doi: 10.1371/journal.pone.0022171
9. Sechi LA, Dow CT. Mycobacterium avium ss. paratuberculosis Zoonosis—the hundred year war—beyond Crohn's disease. Front Immunol. (2015) 6:96. doi: 10.3389/fimmu.2015.00096
10. Eslami M, Shafiei M, Ghasemian A, Valizadeh S, Al-Marzoqi AH, Shokouhi Mostafavi SK, et al. Mycobacterium avium paratuberculosis and Mycobacterium avium complex and related subspecies as causative agents of zoonotic and occupational diseases. J Cell Physiol. (2019) 234:12415–21. doi: 10.1002/jcp.28076
11. Scanu AM, Bull TJ, Cannas S, Sanderson JD, Sechi LA, Dettori G, et al. Mycobacterium avium subspecies paratuberculosis infection in cases of irritable bowel syndrome and comparison with Crohn's disease and Johne's disease: common neural and immune pathogenicities. J Clin Microbiol. (2007) 45:3883–90. doi: 10.1128/JCM.01371-07
12. Sisto M, Cucci L, D'Amore M, Dow TC, Mitolo V, Lisi S. Proposing a relationship between Mycobacterium avium subspecies paratuberculosis infection and Hashimoto's thyroiditis. Scand J Infect Dis. (2010) 42:787–90. doi: 10.3109/00365541003762306
13. Dow CT. M. paratuberculosis heat shock protein 65 and human diseases: bridging infection and autoimmunity. Autoimmune Dis. (2012) 2012:150824. doi: 10.1155/2012/150824
14. Frau J, Cossu D, Coghe G, Lorefice L, Fenu G, Melis M, et al. Mycobacterium avium subsp. paratuberculosis and multiple sclerosis in Sardinian patients: epidemiology and clinical features. Mult Scler. (2013) 19:1437–42. doi: 10.1177/1352458513477926
15. Waddell LA, Rajić A, Stärk KD, McEWEN SA. The zoonotic potential of Mycobacterium avium ssp. paratuberculosis: a systematic review and meta-analyses of the evidence. Epidemiol Infect. (2015) 143:3135–57. doi: 10.1017/S095026881500076X
16. Recht J, Schuenemann VJ, Sánchez-Villagra MR. Host diversity and origin of zoonoses: the ancient and the new. Animals (Basel). (2020) 10:1672. doi: 10.3390/ani10091672
17. Grant IR, Ball HJ, Rowe MT. Incidence of Mycobacterium paratuberculosis in bulk raw and commercially pasteurized cows' milk from approved dairy processing establishments in the United Kingdom. Appl Environ Microbiol. (2002) 68:2428–35. doi: 10.1128/AEM.68.5.2428-2435.2002
18. Hruska K, Bartos M, Kralik P, Pavlik I. Mycobacterium avium subsp. paratuberculosis in powdered infant milk: paratuberculosis in cattle-the public health problem to be solved. Vet Med Praha. (2005) 50:327–35. doi: 10.17221/5631-VETMED
19. Eltholth MM, Marsh VR, Van Winden S, Guitian FJ. Contamination of food products with Mycobacterium avium paratuberculosis: a systematic review. J Appl Microbiol. (2009) 107:1061–71. doi: 10.1111/j.1365-2672.2009.04286.x
20. Waddell L, Rajić A, Stärk K, McEwen SA. Mycobacterium avium ssp. paratuberculosis detection in animals, food, water and other sources or vehicles of human exposure: a scoping review of the existing evidence. Prev Vet Med. (2016) 132:32–48. doi: 10.1016/j.prevetmed.2016.08.003
21. Grant IR, Williams AG, Rowe MT, Muir DD. Efficacy of various pasteurization time-temperature conditions in combination with homogenization on inactivation of Mycobacterium avium subsp. paratuberculosis in milk. Appl Environ Microbiol. (2005) 71:2853–61. doi: 10.1128/AEM.71.6.2853-2861.2005
22. Gerrard ZE, Swift BMC, Botsaris G, Davidson RS, Hutchings MR, Huxley JN, et al. Survival of Mycobacterium avium subspecies paratuberculosis in retail pasteurised milk. Food Microbiol. (2018) 74:57–63. doi: 10.1016/j.fm.2018.03.004
23. Fechner K, Dreymann N, Schimkowiak S, Czerny CP, Teitzel J. Efficacy of dairy on-farm high-temperature, short-time pasteurization of milk on the viability of Mycobacterium avium ssp. paratuberculosis. J Dairy Sci. (2019) 102:11280–90. doi: 10.3168/jds.2019-16590
24. Bates A, O'Brien R, Liggett S, Griffin F. The effect of sub-clinical infection with Mycobacterium avium subsp. paratuberculosis on milk production in a New Zealand dairy herd. BMC Vet Res. (2018) 14:93. doi: 10.1186/s12917-018-1421-4
25. Garcia AB, Shalloo L. Invited review: the economic impact and control of paratuberculosis in cattle. J Dairy Sci. (2015) 98:5019–39. doi: 10.3168/jds.2014-9241
26. Barratt AS, Arnoult MH, Ahmadi BV, Rich KM, Gunn GJ, Stott AW. A framework for estimating society's economic welfare following the introduction of an animal disease: the case of Johne's disease. PLoS ONE. (2018) 13:e0198436. doi: 10.1371/journal.pone.0198436
27. Bulletin of the International Dairy. Federation, No. 362/2001, Mycobacterium paratuberculosis, (2001). Available online at: https://fil-idf.org/publications/bulletin/mycobacterium-paratuberculosis/
28. Nielsen SS, Toft N. A review of prevalences of paratuberculosis in farmed animals in Europe. Prev Vet Med. (2009) 88:1–14. doi: 10.1016/j.prevetmed.2008.07.003
29. Whittington R, Donat K, Weber MF, Kelton D, Nielsen SS, Eisenberg S, et al. Control of paratuberculosis: who, why and how. A review of 48 countries. BMC Vet Res. (2019) 15:198. doi: 10.1186/s12917-019-1943-4
30. Benedictus G, Verhoeff J, Schukken YH, Hesselink JW. Dutch paratuberculosis programme history, principles and development. Vet Microbiol. (2000) 77:399–413. doi: 10.1016/S0378-1135(00)00325-4
31. Nielsen SS, Toft N. Assessment of management-related risk factors for paratuberculosis in Danish dairy herds using Bayesian mixture models. Prev Vet Med. (2007) 81:306–17. doi: 10.1016/j.prevetmed.2007.05.001
32. Benedictus A, Mitchell RM, Linde-Widmann M, Sweeney R, Fyock T, Schukken YH, et al. Transmission parameters of Mycobacterium avium subspecies paratuberculosis infections in a dairy herd going through a control program. Prev Vet Med. (2008) 83:215–27. doi: 10.1016/j.prevetmed.2007.07.008
33. Webb Ware JK, Larsen JW, Kluver P. Financial effect of bovine Johne's disease in beef cattle herds in Australia. Aust Vet J. (2012) 90:116–21. doi: 10.1111/j.1751-0813.2012.00896.x
34. Sweeney RW. Pathogenesis of paratuberculosis. Vet Clin North Am Food Anim Pract. (2011) 27:537–46. doi: 10.1016/j.cvfa.2011.07.001
35. Rathnaiah G, Zinniel DK, Bannantine JP, Stabel JR, Gröhn YT, Collins MT, et al. Pathogenesis, molecular genetics, and genomics of Mycobacterium avium subsp. paratuberculosis, the etiologic agent of Johne's disease. Front Vet Sci. (2017) 4:187. doi: 10.3389/fvets.2017.00187
36. Fecteau ME. Paratuberculosis in cattle. Vet Clin North Am Food Anim Pract. (2018) 34:209–22. doi: 10.1016/j.cvfa.2017.10.011
37. Sweeney RW. Transmission of paratuberculosis. Vet Clin North Am Food Anim Pract. (1996) 12:305–12. doi: 10.1016/S0749-0720(15)30408-4
38. Lombard JE. Epidemiology and economics of paratuberculosis. Vet Clin North Am Food Anim Pract. (2011) 27:525–35. doi: 10.1016/j.cvfa.2011.07.012
39. Coussens PM. Model for immune responses to Mycobacterium avium subspecies paratuberculosis in cattle. Infect Immun. (2004) 72:3089–96. doi: 10.1128/IAI.72.6.3089-3096.2004
40. Baquero MM, Plattner BL. Bovine WC1(+) γδ T lymphocytes modify monocyte-derived macrophage responses during early Mycobacterium avium subspecies paratuberculosis infection. Vet Immunol Immunopathol. (2016) 170:65–72. doi: 10.1016/j.vetimm.2015.12.002
41. Baquero MM, Plattner BL. Bovine peripheral blood WC1+ and WC1neg γδ T lymphocytes modulate monocyte-derived macrophage effector functions during in vitro Mycobacterium avium subspecies paratuberculosis infection. Cell Immunol. (2017) 315:34–44. doi: 10.1016/j.cellimm.2017.01.009
42. Arsenault RJ, Maattanen P, Daigle J, Potter A, Griebel P, Napper S. From mouth to macrophage: mechanisms of innate immune subversion by Mycobacterium avium subsp. paratuberculosis. Vet Res. (2014) 45:54. doi: 10.1186/1297-9716-45-54
43. Koets AP, Eda S, Sreevatsan S. The within host dynamics of Mycobacterium avium ssp. paratuberculosis infection in cattle: where time and place matter. Vet Res. (2015) 46:61. doi: 10.1186/s13567-015-0185-0
44. Park HE, Park HT, Jung YH, Yoo HS. Gene expression profiles of immune-regulatory genes in whole blood of cattle with a subclinical infection of Mycobacterium avium subsp. paratuberculosis. PLoS ONE. (2018) 13:e0196502. doi: 10.1371/journal.pone.0196502
45. Stabel JR, Kimura K, Robbe-Austerman S. Augmentation of secreted and intracellular gamma interferon following Johnin purified protein derivative sensitization of cows naturally infected with Mycobacterium avium subsp. paratuberculosis. J Vet Diagn Invest. (2007) 19:43–51. doi: 10.1177/104063870701900107
46. Ganusov VV, Klinkenberg D, Bakker D, Koets AP. Evaluating contribution of the cellular and humoral immune responses to the control of shedding of Mycobacterium avium spp. paratuberculosis in cattle. Vet Res. (2015) 46:62. doi: 10.1186/s13567-015-0204-1
47. Begg DJ, de Silva K, Carter N, Plain KM, Purdie A, Whittington RJ. Does a Th1 over Th2 dominancy really exist in the early stages of Mycobacterium avium subspecies paratuberculosis infections? Immunobiology. (2011) 216:840–6. doi: 10.1016/j.imbio.2010.12.004
48. Stabel JR, Bannantine JP. Divergent antigen-specific cellular immune responses during asymptomatic subclinical and clinical states of disease in cows naturally infected with Mycobacterium avium subsp. paratuberculosis. Infect Immun. (2019) 88:e00650–19. doi: 10.1128/IAI.00650-19
49. Jungersen G, Mikkelsen H, Grell SN. Use of the Johnin PPD interferon-gamma assay in control of bovine paratuberculosis. Vet Immunol Immunopathol. (2012) 148:48–54. doi: 10.1016/j.vetimm.2011.05.010
50. Purdie AC, Plain KM, Begg DJ, de Silva K, Whittington RJ. Candidate gene and genome-wide association studies of Mycobacterium avium subsp. paratuberculosis infection in cattle and sheep: a review. Comp Immunol Microbiol Infect Dis. (2011) 34:197–208. doi: 10.1016/j.cimid.2010.12.003
51. Koets AP, Gröhn YT. Within- and between-host mathematical modeling of Mycobacterium avium subspecies paratuberculosis (MAP) infections as a tool to study the dynamics of host-pathogen interactions in bovine paratuberculosis. Vet Res. (2015) 46:60. doi: 10.1186/s13567-015-0205-0
52. van den Esker MH, Koets AP. Application of transcriptomics to enhance early diagnostics of mycobacterial infections, with an emphasis on Mycobacterium avium ssp. paratuberculosis. Vet Sci. (2019) 6:59. doi: 10.3390/vetsci6030059
53. Sweeney RW, Whitlock RH, Rosenberger AE. Mycobacterium paratuberculosis cultured from milk and supramammary lymph nodes of infected asymptomatic cows. J Clin Microbiol. (1992) 30:166–71. doi: 10.1128/JCM.30.1.166-171.1992
54. Chiodini RJ. Immunology: resistance to paratuberculosis. Vet Clin North Am Food Anim Pract. (1996) 12:313–43. doi: 10.1016/S0749-0720(15)30409-6
55. Arango-Sabogal JC, Paré J, Labrecque O, Côté G, Roy JP, Buczinski S, et al. Incidence of fecal excretion of Mycobacterium avium subsp. paratuberculosis in dairy cows before and after the enrolment in the Québec voluntary program. Prev Vet Med. (2017) 148:94–105. doi: 10.1016/j.prevetmed.2017.10.006
56. Jungersen G, Huda A, Hansen JJ, Lind P. Interpretation of the gamma interferon test for diagnosis of subclinical paratuberculosis in cattle. Clin Diagn Lab Immunol. (2002) 9:453–60. doi: 10.1128/CDLI.9.2.453-460.2002
57. World Organisation for Animal Health OIE. Manual of Diagnostic Tests and Vaccines for Terrestrial Animals; Paratuberculosis (Johne's disease) - Chapter 8.13.1 (NB. Version adopted in 2004). Paris: OIE (2009). Available online: https://www.oie.int/index.php?id=169andL=0andhtmfile=chapitre_paratuberculosis.htm (accessed December 2020).
58. Kalis CH, Collins MT, Hesselink JW, Barkema HW. Specificity of two tests for the early diagnosis of bovine paratuberculosis based on cell-mediated immunity: the Johnin skin test and the gamma interferon assay. Vet Microbiol. (2003) 97:73–86. doi: 10.1016/j.vetmic.2003.07.003
59. Huda A, Jungersen G, Lind P. Longitudinal study of interferon-gamma, serum antibody and milk antibody responses in cattle infected with Mycobacterium avium subsp. paratuberculosis. Vet Microbiol. (2004) 104:43–53. doi: 10.1016/j.vetmic.2004.08.011
60. Nielsen SS, Toft N. Ante mortem diagnosis of paratuberculosis: a review of accuracies of ELISA, interferon-gamma assay and faecal culture techniques. Vet Microbiol. (2008) 129:217–35. doi: 10.1016/j.vetmic.2007.12.011
61. Plain KM, Begg DJ, de Silva K, Purdie AC, Whittington RJ. Enhancement of the interferon gamma assay to detect paratuberculosis using interleukin-7 and interleukin-12 potentiation. Vet Immunol Immunopathol. (2012) 149:28–37. doi: 10.1016/j.vetimm.2012.05.023
62. Gurung RB, Begg DJ, Purdie AC, de Silva K, Bannantine JP, Whittington RJ. Lymphoproliferative and gamma interferon responses to stress-regulated Mycobacterium avium subsp. paratuberculosis recombinant proteins. Clin Vaccine Immunol. (2014) 21:831–7. doi: 10.1128/CVI.00775-13
63. de la Rua-Domenech R, Goodchild AT, Vordermeier HM, Hewinson RG, Christiansen KH, Clifton-Hadley RS. Ante mortem diagnosis of tuberculosis in cattle: a review of the tuberculin tests, gamma-interferon assay and other ancillary diagnostic techniques. Res Vet Sci. (2006) 81:190–210. doi: 10.1016/j.rvsc.2005.11.005
64. Bezos J, Casal C, Romero B, Schroeder B, Hardegger R, Raeber AJ, et al. Current ante-mortem techniques for diagnosis of bovine tuberculosis. Res Vet Sci. (2014) 97:S44–52. doi: 10.1016/j.rvsc.2014.04.002
65. Wood PR, Corner LA, Plackett P. Development of a simple, rapid in vitro cellular assay for bovine tuberculosis based on the production of gamma interferon. Res Vet Sci. (1990) 49:46–9. doi: 10.1016/S0034-5288(18)31044-0
66. Wood PR, Corner LA, Rothel JS, Baldock C, Jones SL, Cousins DB, et al. Field comparison of the interferon-gamma assay and the intradermal tuberculin test for the diagnosis of bovine tuberculosis. Aust Vet J. (1991) 68:286–90. doi: 10.1111/j.1751-0813.1991.tb03254.x
67. Wood PR, Jones SL. BOVIGAM: an in vitro cellular diagnostic test for bovine tuberculosis. Tuberculosis. (2001) 81:147–55. doi: 10.1054/tube.2000.0272
68. Tameni S, Amadori M, Scaccaglia P, Quondam-Giandomenico R, Tagliabue S, Achetti IL, et al. Quality controls and in vitro diagnostic efficiency of bovine PPD tuberculins. Biologicals. (1998) 26:225–35. doi: 10.1006/biol.1998.0147
69. Corneli S, Corte L, Roscini L, Di Paolo A, Colabella C, Petrucci L, et al. Spectroscopic characterization of bovine, avian and Johnin purified protein derivative (PPD) with high-throughput Fourier transform infrared-based method. Pathogens. (2019) 8:136. doi: 10.3390/pathogens8030136
70. Wood PR, Rothel JS. In vitro immunodiagnostic assays for bovine tuberculosis. Vet Microbiol. (1994) 40:125–35. doi: 10.1016/0378-1135(94)90051-5
71. Faye S, Moyen JL, Gares H, Benet JJ, Garin-Bastuji B, Boschiroli ML. Determination of decisional cut-off values for the optimal diagnosis of bovine tuberculosis with a modified IFN gamma assay (Bovigam®) in a low prevalence area in France. Vet Microbiol. (2011) 151:60–7. doi: 10.1016/j.vetmic.2011.02.026
72. EFSA (European Food Safety Authority). EFSA panel on animal health and welfare (AHAW); scientific opinion on the use of a gamma interferon test for the diagnosis of bovine tuberculosis. EFSA J. (2012) 10:2975. doi: 10.2903/j.efsa.2012.2975
73. Keck N, Boschiroli ML, Smyej F, Vogler V, Moyen JL, Desvaux S. Successful application of the gamma-interferon assay in a bovine tuberculosis eradication program: the french bullfighting herd experience. Front Vet Sci. (2018) 5:27. doi: 10.3389/fvets.2018.00027
74. Nuñez-Garcia J, Downs SH, Parry JE, Abernethy DA, Broughan JM, Cameron AR, et al. Meta-analyses of the sensitivity and specificity of ante-mortem and post-mortem diagnostic tests for bovine tuberculosis in the UK and Ireland. Prev Vet Med. (2018) 153:94–107. doi: 10.1016/j.prevetmed.2017.02.017
75. Wright K, Plain K, Purdie A, Saunders BM, de Silva K. Biomarkers for detecting resilience against mycobacterial disease in animals. Infect Immun. (2019) 88:e00401–19. doi: 10.1128/IAI.00401-19
76. Ricchi M, Barbieri G, Taddei R, Belletti GL, Carra E, Cammi G, et al. Effectiveness of combination of Mini-and Microsatellite loci to sub-type Mycobacterium avium subsp. paratuberculosis Italian type C isolates. BMC Vet Res. (2011) 7:54. doi: 10.1186/1746-6148-7-54
77. European Community. Consolidated (English) version of Council Directive 64/432/EEC of 26 June 1964 on animal health problems affecting intra-Community trade in bovine animals and swine. Off J Eur Commu. (1964) Annex B 121:1977–2012.
78. European Community. Commission Regulation (EC)1226/2002 of 8 July 2002 Amending Annex B to Council Directive 64/332/EEC. Available online at: https://eur-lex.europa.eu/legal-content/EN/TXT/HTML/?uri=CELEX: 32002R1226andfrom=EN (accessed August 10, 2020).
79. Italian Ministry of Health Decree No 196 of 22May 1999. Implementation of Q21 Directive 97/12/EC Amending and Updating Directive 64/432/EEC on Animal Q26 Health Problems Affecting Intra-Community Trade in Bovine Animals and Swine. Gazzetta Ufficiale Della Repubblica Italiana-Serie Generale 146 (1999). Available online at: https://www.gazzettaufficiale.it/eli/id/1999/06/24/ 099G0224/sg (accessed August 10, 2020).
80. Italian Ministry of Health. Decree No 592 of 15 December 1995. Regulation Q21 on the National Plan for the Eradication of Tuberculosis in Cattle and Buffalo Q26 Herds-and Subsequent Amendments. Gazzetta Ufficiale Della Repubblica Italiana Serie Generale 125. Available online at: https://www.gazzettaufficiale (accessed December 2020).
81. Agreement. Agreement Between the Government, the Regions and the Autonomous Provinces of Trento and Bolzano on Guidelines for the adoption of control and certification plans for bovine paratuberculosis. (Rep. Acts n. 146/CSR). (13a09123) GU General Seriesn. 271 of 19-11-2013 - Ordinary Suppl. n.79). (2013). Available online at: http://www.gazzettaufficiale.it/eli/gu/2013/11/19/271/so/79/sg/pdf (accessed December 2020).
82. Mazzone P, Agnetti F, Biagetti M, Cagiola M, Mangili PM, Nardini R, et al. Infezione da Mycobacterium avium subsp. Paratuberculosis e gamma-interferon test. Argomenti. (2010) 1:44–7. Available online at: https://sivemp.it/post_rivista/argomenti-nr-1-2010-anno-xiii/
83. Donaghy JA, Rowe MT, Rademaker JL, Hammer P, Herman L, De Jonghe V, et al. An inter-laboratory ring trial for the detection and isolation of Mycobacterium avium subsp. paratuberculosis from raw milk artificially contaminated with naturally infected faeces. Food Microbiol. (2008) 25:128–35. doi: 10.1016/j.fm.2007.06.007
84. Ricchi M, Manini F, Cammi G, Donaghy J, Arrigoni N. Comparison of four different PCR methods for the detection of Mycobacterium avium subsp. paratuberculosis in milk. In: Proceedings of 10 International Colloquium on Paratuberculosis - ICP. Minneapolis Minnesota (2009). p. 56–9.
85. Sebastiani C, Curcio L, Ciullo M, Mazzone P, Pezzotti G, Biagetti M. Development of IS900 and F57 fast real-time PCR assays for the detection of Mycobacterium paratuberculosis. In: Proceedings of XII International Colloquium on Paratuberculosis - ICP. Parma (2014). p. 84.
86. Roupie V, Alonso-Velasco E, Van Der Heyden S, Holberte S, Duytschaevera L, Berthon P, et al. Evaluation of mycobacteria-specific gamma interferon and antibody responses before and after a single intradermal skin test in cattle naturally exposed to M. avium subsp. paratuberculosis and experimentally infected with M. bovis. Vet Immunol Immunopathol. (2018) 196:35–47. doi: 10.1016/j.vetimm.2017.12.007
87. European Commission. Commision Decision of 5 February 2016 Amending Decision 2003/467/EC and Declaring Piedmont Officially free of Bovine Tuberculosis (2016/168/EC). Available online at: https://eur-lex.europa.eu/legal-content/EN/TXT/?uri=celex%3A32016D0168 (accessed August 10, 2020).
88. Dondo A, Goria M, Moda G, Cesano L, Garanzini A, Giammartino M, et al. La prova del g-interferone per la diagnosi della tubercolosi bovina: determinazione della sensibilità e della specificità in prove di campo. Med Vet Prevent. (1996) 13:14–8.
89. Chiavacci L, Dondo A, Goria M, Moda G, Ruocco L, Vignetta P, et al. Tuberculosis eradication in Italy. In: Thoen CO, editor. Zoonotic Tuberculosis: Mycobacteriumbovis and Other Pathogenic Mycobacteria. Hoboken, NJ: Wiley and Sons (2014). p. 357–69.
90. Moda G. Non-technical constraints to eradication: the Italian experience. Vet Microbiol. (2006) 112:253–8. doi: 10.1016/j.vetmic.2005.11.021
91. DeLong ER, DeLong DM, Clarke-Pearson DL. Comparing the areas under two or more correlated receiver operating characteristic curves: a nonparametric approach. Biometrics. (1988) 44:837–45. doi: 10.2307/2531595
92. Martucciello A, Vitale N, Mazzone P, Dondo A, Archetti I, Chiavacci L, et al. Field evaluation of the interferon gamma assay for diagnosis of tuberculosis in water buffalo (Bubalus bubalis) comparing four interpretative criteria. Front Vet Sci. (2020) 7:563792. doi: 10.3389/fvets.2020.563792
93. Dunn JR, Kaneene JB, Grooms DL, Bolin SR, Bolin CA, Bruning-Fann CS. Effects of positive results for Mycobacterium avium subsp. Paratuberculosis as determined by microbial culture of feces or antibody ELISA on results of caudal fold tuberculin test and interferon-g assay for tuberculosis in cattle. J Am Vet Med Assoc. (2005) 226:429–35. doi: 10.2460/javma.2005.226.429
94. Aranaz A, De Juan L, Bezos J, Alvarez J, Romero B, Lozano F, et al. Assessment of diagnostic tools for eradication of bovine tuberculosis in cattle co-infected with Mycobacterium bovis and M. avium subsp. paratuberculosis. Vet Res. (2006) 37:593–606. doi: 10.1051/vetres:2006021
95. Álvarez J, de Juan L, Bezos J, Romero B, Sáez JL. Marqués S, et al. Effect of paratuberculosis on the diagnosis of bovine tuberculosis in a cattle herd with a mixed infection using interferongamma detection assay. Vet Microbiol. (2009) 135:389–93. doi: 10.1016/j.vetmic.2008.09.060
96. Antognoli MC, Remmenga MD, Bengtson SD, Clark HJ, Orloski KA, Gustafson LL, et al. Analysis of the diagnostic accuracy of the gamma interferon assay for detection of bovine tuberculosis in U.S. herds. Prev Vet Med. (2011) 101:35–41. doi: 10.1016/j.prevetmed.2011.05.012
97. Hughes V, McNair J, Strain S, Barry C, McLuckie J, Nath M, et al. Gamma interferon responses to proteome-determined specific recombinant proteins in cattle experimentally- and naturally-infected with paratuberculosis. Res Vet Sci. (2017) 114:244–53. doi: 10.1016/j.rvsc.2017.04.018
98. Aagaard C, Govaerts M, Meikle V, Vallecillo AJ, Gutierrez-Pabello JA, Suarez-Güemes F, et al. Optimizing antigen cocktails for detection of Mycobacterium bovis in herds with different prevalences of bovine tuberculosis: ESAT6-CFP10 mixture shows optimal sensitivity and specificity. J Clin Microbiol. (2006) 44:4326–35. doi: 10.1128/JCM.01184-06
99. Casal C, Bezos J, Díez-Guerrier A, Álvarez J, Romero B, de Juan L, et al. Evaluation of two cocktails containing ESAT-6, CFP-10 and Rv-3615c in the intradermal test and the interferon-γ assay for diagnosis of bovine tuberculosis. Prev Vet Med. (2012) 105:149–54. doi: 10.1016/j.prevetmed.2012.02.007
100. Dernivoix K, Roupie V, Welby S, Roelandt S, Viart S, Letesson, et al. Field performance of six Mycobacterium avium subsp. Paratuberculosis antigens in a 20h interferon gamma release assay in Belgium. Vet Immunol Immunopathol. (2017) 189:17–27. doi: 10.1016/j.vetimm.2017.05.008
Keywords: Mycobacterium avium subsp. paratuberculosis, Johne's disease, bovine paratuberculosis, IFN-γ test, purified protein derivatives, Johnin PPD, interpretative criteria, cattle
Citation: Corneli S, Di Paolo A, Vitale N, Torricelli M, Petrucci L, Sebastiani C, Ciullo M, Curcio L, Biagetti M, Papa P, Costarelli S, Cagiola M, Dondo A and Mazzone P (2021) Early Detection of Mycobacterium avium subsp. paratuberculosis Infected Cattle: Use of Experimental Johnins and Innovative Interferon-Gamma Test Interpretative Criteria. Front. Vet. Sci. 8:638890. doi: 10.3389/fvets.2021.638890
Received: 07 December 2020; Accepted: 30 March 2021;
Published: 14 May 2021.
Edited by:
Kumi de Silva, The University of Sydney, AustraliaReviewed by:
Eduard Otto Roos, Pirbright Institute, United KingdomSanthamani Ramasamy, Public Health Research Institute (PHRI), United States
Copyright © 2021 Corneli, Di Paolo, Vitale, Torricelli, Petrucci, Sebastiani, Ciullo, Curcio, Biagetti, Papa, Costarelli, Cagiola, Dondo and Mazzone. This is an open-access article distributed under the terms of the Creative Commons Attribution License (CC BY). The use, distribution or reproduction in other forums is permitted, provided the original author(s) and the copyright owner(s) are credited and that the original publication in this journal is cited, in accordance with accepted academic practice. No use, distribution or reproduction is permitted which does not comply with these terms.
*Correspondence: Linda Petrucci, bC5wZXRydWNjaUBpenN1bS5pdA==
†These authors have contributed equally to this work and share first authorship