- 1School of Health and Biomedical Sciences, RMIT University, Bundoora, VIC, Australia
- 2Department of Pathology, Sohar Hospital, Sohar, Oman
- 3School of Science, RMIT University, Bundoora, VIC, Australia
- 4The University of Melbourne, Department of Medicine, Austin Health, Heidelberg, VIC, Australia
Osteosarcoma (OS) is the most common primary bone tumor and originates from bone forming mesenchymal cells and primarily affects children and adolescents. The 5-year survival rate for OS is 60 to 65%, with little improvement in prognosis during the last four decades. Studies have demonstrated the evolving roles of parathyroid hormone-related protein (PTHrP) and its receptor (PTHR1) in bone formation, bone remodeling, regulation of calcium transport from blood to milk, regulation of maternal calcium transport to the fetus and reabsorption of calcium in kidneys. These two molecules also play critical roles in the development, progression and metastasis of several tumors such as breast cancer, lung carcinoma, chondrosarcoma, squamous cell carcinoma, melanoma and OS. The protein expression of both PTHrP and PTHR1 have been demonstrated in OS, and their functions and proposed signaling pathways have been investigated yet their roles in OS have not been fully elucidated. This review aims to discuss the latest research with PTHrP and PTHR1 in OS tumorigenesis and possible mechanistic pathways.
This review is dedicated to Professor Michael Day who died in May 2020 and was a very generous collaborator.
Introduction
Osteosarcoma (OS) or osteogenic sarcoma is defined as the malignancy that originates from bone-forming mesenchymal cells (1–5). This tumor is also known as the “growing bone tumor” (6). OS is the primary malignant tumor of the skeleton in which tumor cells directly form immature bone or osteoid (7). OS is the most prevalent type of primary bone cancer in both humans and dogs (8–11). OS occurs more frequently in children, adolescents, taller humans, and large breeds of dogs (9, 12). In both species, OS mostly affects the ends of long bones near the metaphyseal regions (9, 13). The femur, tibia and humerus are the locations that are most often affected by OS in humans (14).
OS is not a modern disease. A recent study revealed that dinosaurs also were affected by OS (15). Ekhtiari et al. confirmed this grossly, radiographically, and histologically in a fibula from a Centrosaurus in Canada. The dinosaur dates from around 77 to 75.5 million years ago (15). Previously, paleontologists found periosteal OS using micro-computerized tomography (CT) in the hindleg of a fossilized turtle (16). This was the oldest OS to be found in an amniote indicating that OS was present in this fossil that has been dated to 240 million years old.
There has been little improvement in the treatment of OS and its prognosis in the last 40 years, especially for those patients with metastatic OS (17–21). The reason behind this could be the unavailability of novel biomarkers. Perhaps if there were confirmed prognostic tumor markers, this might assist in categorizing patients for risk-based treatment. Furthermore, the complexity of OS is such that no two tumors look alike (22).
The current treatment strategy for human OS involves neoadjuvant chemotherapy followed by surgical removal of the tumor and adjuvant chemotherapy (23). Standard chemotherapy uses a combination of doxorubicin and cisplatin with a high dose of methotrexate in the neoadjuvant and adjuvant regimens (24). This treatment procedure can improve the five-year survival rate by 60-65% (23, 25). However, early surgical removal of the tumor is the most successful treatment method (26, 27).
Canine and human OS share several key features such as presence of micrometastatic disease at diagnosis, p53 mutations, abnormal expression of several proteins (e.g., activator of transcription 3, tensin homolog, Met, phosphatase, signal transducer and ezrin), affected site and development of chemotherapy-resistance (28). Furthermore, OS in dogs and humans share similar DNA copy number aberrations and show overlapping transcriptional profiles, suggesting that these two diseases are similar at the molecular level. In addition, the metastatic rate of OS without chemotherapy is 90% for dogs and 85–90% for humans and occur mostly in lung, bone and soft tissues, in both species (28).
The high metastasis rate of OS results from the primary bone tumor spread via hematogenous path to other secondary locations (28). The most common cause of death in OS patients is the development of pulmonary metastasis (28). Metastasis occurs most frequently in lungs but rarely occurs in the surrounding pleura. There is one case report where this happened and the authors suggested it was due to the direct contact of pleura with the lungs (29).
Even though <15% of OS metastases in canine and human patients are detected at diagnosis radiologically, 85 to 90% of patients develop gross metastases regardless of effective management of the primary bone tumor (28). This shows that microscopic metastases arise in the early stages of the disease (30). The overall 5-year survival rate for OS in humans is around 60 to 70% in patients with no metastases and 10 to 30% in patients with metastases at diagnosis (24, 31–33). On the other hand, long-term survival rates for OS in dogs is only 10 to 15% (34), supporting the idea that the canine OS may be more aggressive compared to human OS (28).
One study found that overexpression of membrane-cytoskeleton linker ezrin is involved with early development of OS metastases in dogs (35). In line with canine OS data, it has been found that increased expression of ezrin is significantly associated with poor prognosis in OS cases in children (28). Using canine OS cell lines, Hong et al. found that there is an association between PKC and ezrin-radixin-moesin (36). They showed that PKC inhibitor stops ezrin phosphorylation and tumor cell migration (36). Jaroensong et al. reported that overexpression of p-ezrin-radixin-moesin occurred early in the development of pulmonary micrometastases of OS using orthotopic xenograft mouse model of canine OS (37). This expression decreased at later stages suggesting that ezrin is involved in roles related to the survival of cancer cells after their arrival at secondary metastatic sites (37).
Development of metastatic OS is the major cause of death in dogs and humans. So, the identification of new and significant treatments are crucial for the prevention of tumor metastasis which would lead to the reduction of the number of deaths in both dogs and humans (28).
The only basic prognostic indicators of human OS are the patient's response to chemotherapy, the presence of metastases and satisfactory surgical margins (38). Other prognostic indicators such as histological subtype, age, high concentration of serum lactate dehydrogenase or alkaline phosphatase (ALP), tumor size and site are still contentious (38). Recently, it has been shown that the expression of parathyroid hormone receptor 1 (PTHR1) is a prognostic indicator in canine OS (39). Although several studies have been carried out to elucidate the molecular pathogenesis and related signaling pathways of OS using human tissue, murine, canine models and cell lines, the disease remains an unsolved puzzle.
Parathyroid hormone-related protein (PTHrP) was first discovered as a causative factor of humoral hypercalcemia of malignancy syndrome (40, 41). This syndrome occurs because of increased secretion of PTHrP from tumor cells resulting in elevated levels of calcium in serum and increasing cyclic adenosine 3′,5′-monophosphate (cAMP) excretion in urine (42, 43). In humans, PTHrP is synthesized as a protein with either 139, 141, or 173 amino acids due to differences in mRNA splicing (44). PTHrP shares homology of its N-terminal amino acid sequence (1–34) with parathyroid hormone (PTH) (41). This allows both hormones to act through a common receptor (PTH/PTHrP receptor or PTHR1) (45).
PTHR1 is a seven-transmembrane class B G-protein-coupled receptor (GPCR) (46). Examples of receptors included in this family are the receptors for secretin, glucagon, pituitary adenylate cyclase-activating peptide, growth hormone-releasing hormone, vasoactive intestinal peptide, corticotrophin-releasing factor, glucagon-like peptide, calcitonin, and gastric inhibitory peptide (47). Structurally, PTHR1 contains N-terminal extracellular domain (ECD) of ~100–160 amino acid residues, a transmembrane domain (TMD) containing the seven membrane-spanning α-helices and a C-terminal tail (48).
PTHR1 is activated by the binding of the N-terminal (1–34) amino acids of PTH or PTHrP (47). The NH2-terminal part of PTH/PTHrP binds to the extracellular connecting loops and the TMD α-helices of PTHR1 (49, 50). This interaction induces conformational changes in PTHR1, which initiates intracellular signaling (51, 52). However, the COOH-terminal part of PTH/PTHrP binds to the N-terminal ECD of PTHR1 (53, 54).
Activation of PTHR1 initiates events of intracellular processes by signaling through the stimulatory G-protein α-subunit (Gsα) (55). Subsequently, the synthesis of cAMP is stimulated and PKA is triggered (56). However, PTHR1 can be activated by another signaling pathway through the Gq class of G-protein α-subunits (Gqα) (57). This activation results in triggering phospholipase C (57) which in turn activates PKC and raises inositol triphosphate and intracellular calcium in tissues (56, 58).
Numerous studies have established the roles of PTHrP and PTHR1 in bone formation, remodeling (Figures 1, 2) and regulation of calcium transport (60–64). In addition, these molecules play a role in the progression and metastasis of many human tumor types such as lung and breast cancers (65, 66). The aim of this review is to highlight the latest findings about functions of PTHrP and PTHR1 in normal and neoplastic tissues by focusing on their roles in the progression of OS and discuss the possible related pathways.
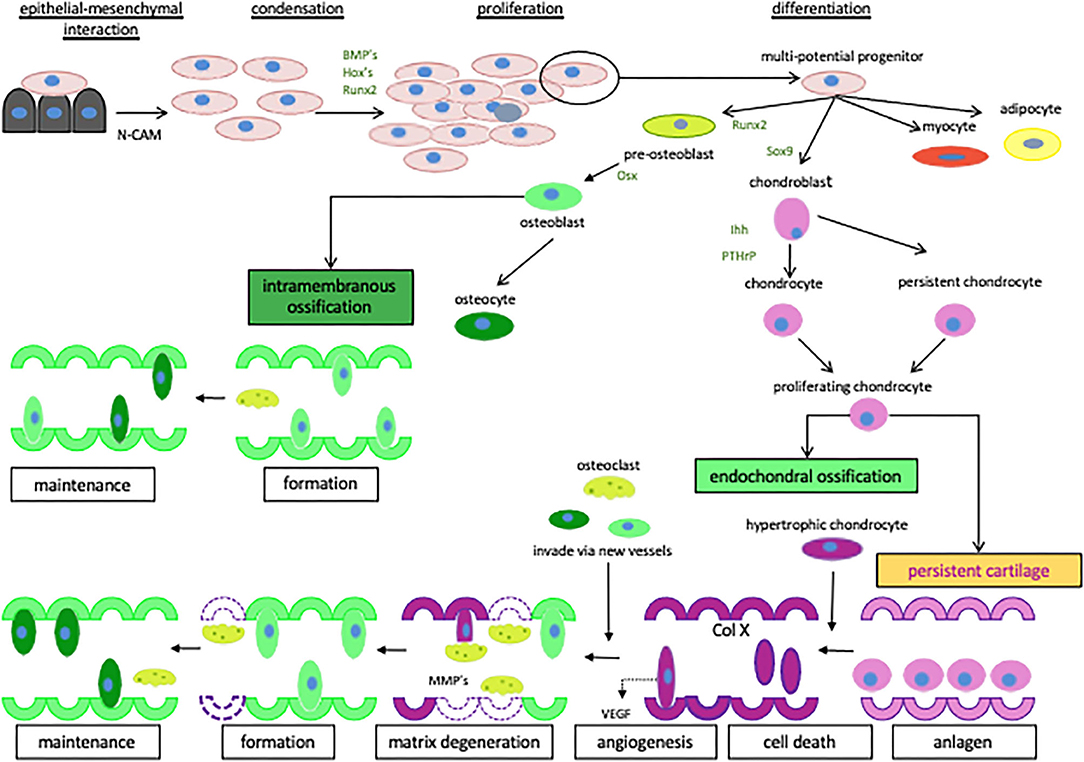
Figure 1. The regulation of cartilage and bone formation. Diagrammatic representation of the network of signaling factors involved in cartilage and bone formation. Starting with the creation of mesenchymal condensations and their subsequent transition to differentiated cartilage and bone. The cells are represented as osteoblasts , pre-osteoblasts
, chondroblasts
, chondrocytes
, osteoclasts
, hypertrophic chondrocytes
, adipocytes
and myocytes
. Reproduced with permission from reference (59).
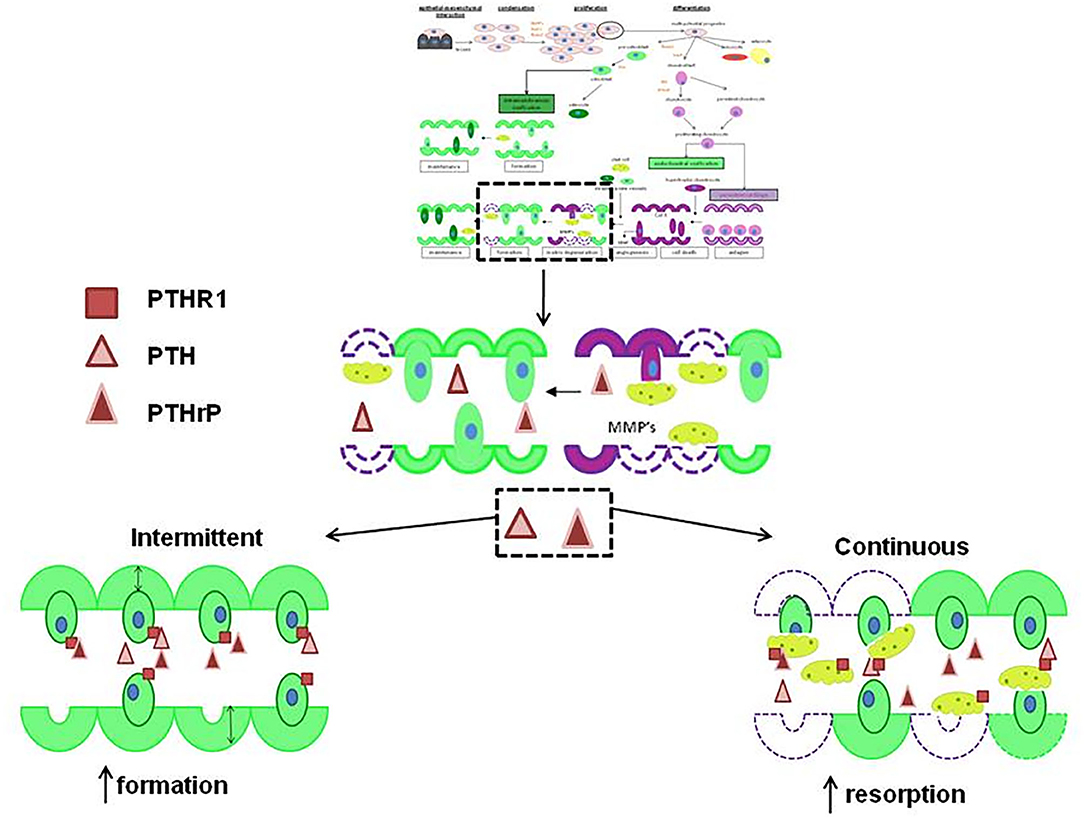
Figure 2. The actions of PTH and PTHrP on new bone formation. If PTHrP and PTH
are given intermittently to patients, they increase the formation of new bone
but if either are given continuously, they increase resorption by stimulating osteoclasts to remodel bone
. Both act via PTHR1
. Reproduced with permission from reference (59).
Roles of PTHrP in Normal and Tumor Tissues
PTHrP acts as an autocrine or paracrine factor and has a role in a number of significant physiological processes in bone, such as the regulation of chondrocyte and osteoblast differentiation and the proliferation (Figure 1) in the growth plates of developing long bones (60, 61). In bone tissue, PTHrP maintains the columnar organization of the chondrocytes and slows down their differentiation (61). Garcia-Martin et al. (67) suggested that PTHrP promotes proliferation of osteoblasts and matrix mineralization via three partially redundant mechanisms. These mechanisms are an intracrine nuclear localization signal-dependent mechanism, an autocrine/paracrine signal-peptide/PTHR1-dependent mechanism, and mixed mechanism (67). Thus, secretion of PTHrP and subsequent activation of PTHR1 would induce proliferation and mineralization of osteoblastic cells (67) (Figure 1).
In addition, PTHrP is involved in significant processes in other tissues including breast (62, 68) and placenta (63, 64). In the breast, PTHrP is abundant in milk, produced via the lactating breast and has an important role in branching morphogenesis of the mammary glands (62, 68). The concentration of PTHrP in plasma is increased during lactation resulting in the regulation of calcium transport from blood into the milk (62) and stimulation of calcium mobilization from bone (68). In the placenta, PTHrP has a role in regulating the direct transport of maternal calcium to the fetus across the placental membrane (63, 64).
Over and above its normal roles, increasing evidence has indicated that PTHrP plays critical roles in tumorigenesis (69–72). It has been found that PTHrP has a role in the activation of protein kinase A (PKA) and C (PKC) pathways (73), regulation of primary tumor growth and in metastasis (72). Luparello et al. (69) found that PTHrP stimulates cell invasion using the 8701-BC human primary breast ductal infiltrating carcinoma cell line. Further data obtained from immortalized human mammary epithelial cell lines (S1T3, S2T2, and NS2T2A1) indicated that PTHrP stimulates proliferation of tumor cells (70). In addition, it has been found that knockdown of PTHrP reduced tumor growth, induced apoptosis of osteoblasts and stimulated the formation of autophagosomes using human MDA-MB-231 breast cancer cell line (74). The authors suggested that blocking of PTHrP in the tumor cells might be a possible targeted therapy for breast cancers, particularly those with skeletal metastases (74). Similarly, Li et al. showed that PTHrP promotes breast tumor initiation, progression and metastasis in mice and it could be a novel therapy target (75). Together, these studies revealed that PTHrP plays a critical role in the initiation of breast cancer (74, 75).
A retrospective study found that increased circulating PTHrP levels might be prognostic with shorter survival time and bone metastases in patients with lung carcinoma (71). Recently, Hastings et al. (65) also examined whether N-terminus or C-terminus of PTHrP correlated with different lung carcinoma type and prognosis. They established that C-terminus of PTHrP may reduce the effect of N-terminus PTHrP on tumor growth and progression (65). Iguchi et al. (76) established the role of PTHrP in bone metastasis in mice models using human lung squamous cell carcinoma-derived cells. Breast and lung cancers usually cause osteolytic metastases in bone (77). This osteolytic process depends on osteoclast-mediated bone resorption via up-regulated osteoclastogenesis (77). Osteoclast differentiation factors, which play a significant role in this process are receptor activator of nuclear factor-jB (RANK), its ligand (RANKL) and the decoy receptor, osteoprotegerin (OPG) (77). In humans, positive PTHrP staining was seen in 60% of primary breast tumors (78) and 92% of bone metastases (79). Recently Kim et al. (66) showed that activation of the calcium-sensing receptor (CaSR), a GPCR, up-regulated the production of PTHrP in breast cancer in vitro. As a result, this enhanced proliferation of breast cancer cells and reduced apoptosis (66). It was observed that reducing the expression of CaSR in vivo and in vitro inhibited the production of PTHrP and reduced the growth of the breast cancer (66).
In addition to breast and lung cancers, PTHrP has been found to stimulate tumor cell survival and proliferation in other cancers including chondrosarcoma (80), anaplastic thyroid cancer (81), medulloblastoma (82), adrenocortical cancer (83), oral squamous cancer (84), colon cancer (85), prostate cancer (86) and renal cancer (87). It has also been found that PTHrP is an essential growth factor for human clear cell renal carcinoma (CCRC) and acts as a novel target for the von Hippel-Lindau tumor suppressor protein in vitro (88). Talon et al. (87) demonstrated that apoptosis could be induced in the human CCRC cell line via the induction of PTHrP-neutralizing antibodies followed by the inhibition of PTHR1. Furthermore, Danilin et al. (89) showed that the mRNA-binding protein HuR is involved in increased expression of PTHrP and in mRNA stabilization in CCRC. A number of case studies reported a strong expression of PTHrP in pancreatic adenocarcinoma (90), intrahepatic cholangiocarcinoma (91), pancreatic neuroendocrine cancer and that PTHrP levels were elevated in the patient serum (92).
In addition to its role in tumorigenesis, Kir et al. (93) showed that PTHrP is involved in cancer cachexia, a wasting disorder of adipose and skeletal muscle tissues that leads to intensive weight loss resulting in reduced survival time in patients with cancer. PTHrP drives the expression of genes that are involved in thermogenesis in adipose tissue (93). It was demonstrated that the genes responsible for fat and muscle tissue loss were neutralized by anti-PTHrP antiserum (93). In summary, PTHrP is appearing to be a crucial factor in the pathogenesis of a large range of epithelial and non-epithelial tumors.
Roles of PTHrP in OS
The first attempt to understand the role of PTH in OS was by Martin et al. (94) by inducing OS in rats using radioactive phosphorous isotopes. Several later studies found that PTHrP also plays a role in pathogenesis of OS (Table 1, Figure 3) (96, 101–104). Suda et al. (102) demonstrated the expression of PTHrP mRNA in all investigated rat UMR 106-01 and UMR 106-06 OS cell lines. Ho et al. (99) revealed that PTHrP is also expressed by murine OS cells. Recently, PTHrP was detected in all primary canine OS tissues (n = 50) using immunohistochemistry staining (39). The findings showed that 50% of these canine OS tissues had weak staining intensity and 50% strong staining intensity. The study also found that there was not significant correlation between the staining intensity and the prognosis of OS in dogs (39).
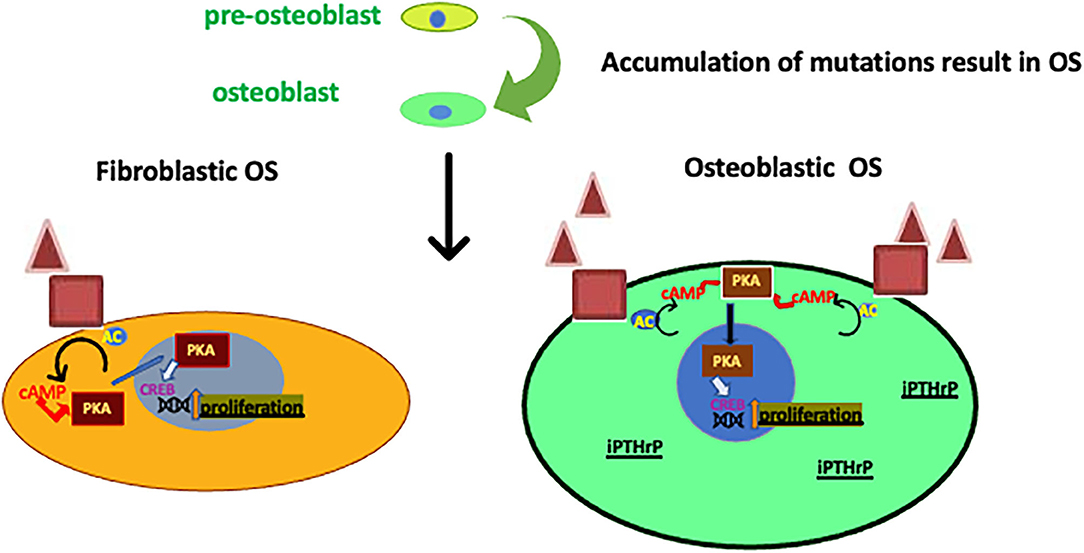
Figure 3. Roles of PTHrP on two of the OS subtypes. Subtypes of OS arise from pre-osteoblasts that accrue mutations (possibly in Rb or p53). PTHrP, PTHR1 and CREB activity are increased in osteoblastic OS influencing proliferation (99, 100) when compared to fibroblastic OS. Also, the intracrine PTHrP (iPTHrP) may contribute to this process.
In fact, the immunohistochemical (IHC) staining of PTHrP demonstrated the presence of the protein in the OS at the time of staining, but it does not tell us how much PTHrP is produced and secreted over the time (39). This association between the presence of PTHrP protein and prognosis has not yet been investigated in humans
In contrast, PTHrP mRNA was not detected in aggressive human OS xenografts (105). It has also been found that increased expression of the PTHrP gene is associated with reduced tumor growth and cell proliferation (Table 1) using a murine OS cell line (96) and a rat OS cell line (106). Previous findings discussed above showed that over-expression of PTHrP could be correlated with a better prognosis for OS (Figure 4).
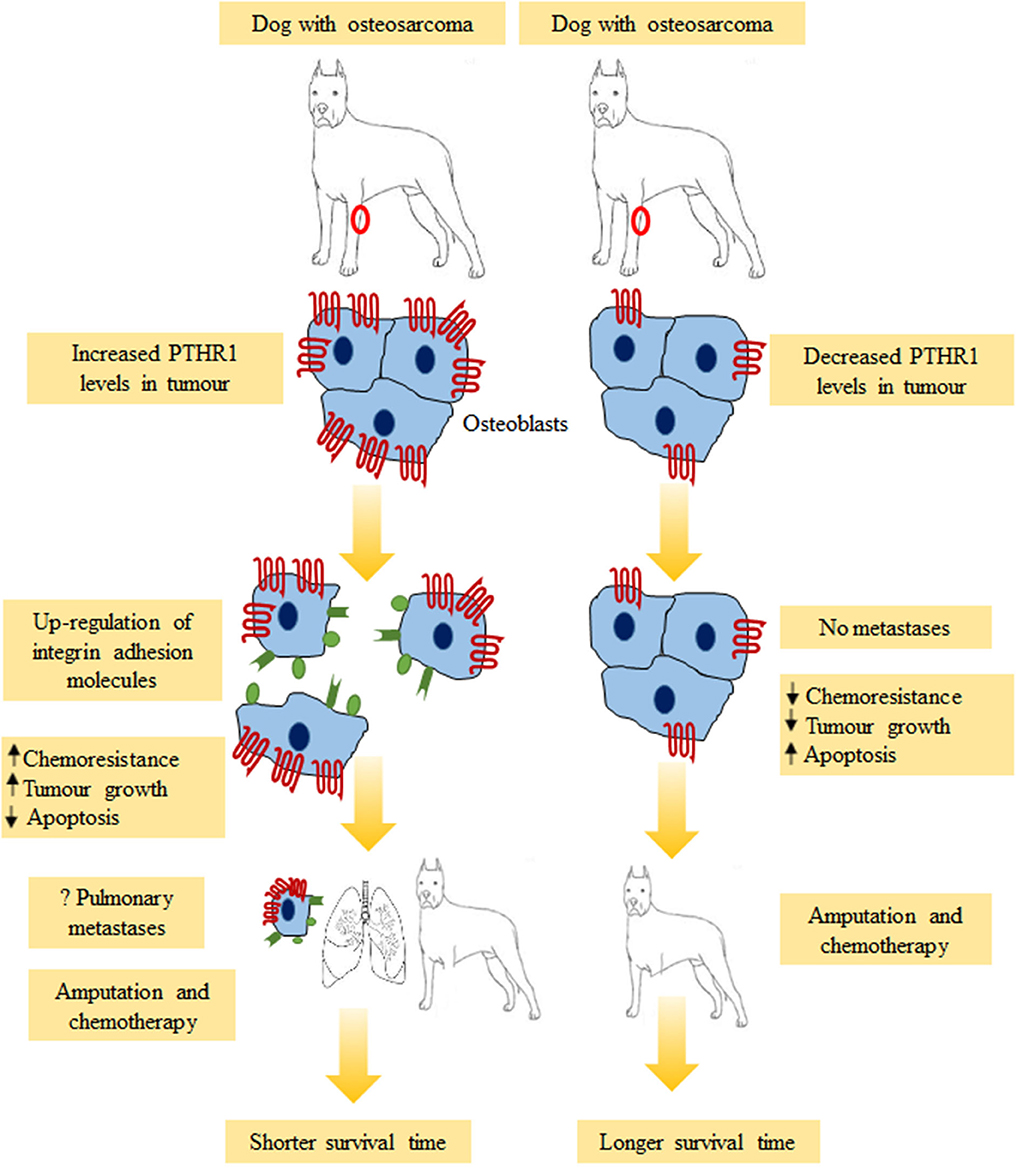
Figure 4. Possible outcomes for dogs with osteosarcoma. Dogs with strong PTHR1 immunostaining tumors had shorter overall survival times compared to those with weak immunostaining. Overabundance of PTHR1 could activate neoplastic osteoblasts to detach via up-regulation of integrin adhesion molecules (αvβ3, β1, α2β1, α5β1, α6β1), resulting in pulmonary metastases. Other possible mechanisms which could explain the effects of PTHR1 expression including increased chemoresistance, increased tumor growth and decreased apoptosis. This might result in shorter survival time.
However, Gagiannis et al. (97) noted that PTHrP caused tumor cells of SaOS2 human OS cell line to be chemoresistant (Table 1). This was observed after inhibiting major apoptosis signaling pathways via blocking the death receptor and mitochondria-mediated apoptosis signaling (97). It has also been found that PTHrP stimulates migration of SaOS-2 and MG-63 human OS cell lines (98). These two studies suggest that overexpression of PTHrP could be correlated with a poorer prognosis of OS (97, 98). These conflicting data may be related to the use of different portion of PTHrP sequences in these different studies (67, 107). If PTHrP influences chemoresistance then it would be a good therapeutic target. Blocking this action could improve patient survival with current treatments.
Ho et al. (99) found that the three major OS subtypes (osteoblastic, chondroblastic and fibroblastic OS) produce PTHrP, which act through the PTHR1 to activate adenylyl cyclase, PKA, and the transcription factor cAMP responsive element binding protein 1 (CREB1) (Figure 3) (99). The osteoblastic subtype had an increased level of PTHR1 compared with the fibroblastic subtype but the PTHrP levels were no different (99). The knockdown of PTHrP in OS reduced cell growth and invasion in vitro and increased apoptosis and growth inhibition in vivo, while the knockdown of CREB1 had much greater growth inhibition and apoptosis (99). Moreover, Walia et al. (108), found that PTHrP is a key factor for initiation of OS in p53-deficient osteoblasts. The production of cAMP is stimulated by PTHrP (108). This stimulation is followed by PTHR1 activation, then, phosphorylation and transcription of CREB1 is activated in p53-deficient OS (Figure 3) (108). It was suggested that PTHrP-cAMP-CREB1-axis is essential for the initiation and progression of OS in p53-deficient osteoblasts (108). These findings are significant because P53 deficiency is a common event in OS and understanding of this pathway could lead to a better elucidation of this disease (108).
All of the above data showed that PTHrP is crucial for tumorigenesis of OS and increased expression could be linked with poor prognosis in mice (Table 1). However, further in vivo studies are necessary to clarify the exact roles of PTHrP in the progression of OS, possibly to be undertaken in dogs.
Roles of Human Parathyroid Hormone in OS
The active portion of human parathyroid hormone is a 34-amino acid peptide (109). Studies demonstrated that PTH (1–34) and the native 84-amino acid hormone have identical spectrum of biological responses in bone (110, 111). It has been shown that single-daily subcutaneous administration of PTH (1–34) accelerates the production of new bone matrix on the endocortical, trabecular and periosteal surfaces via the stimulated osteoblasts (Figure 2) (110). This leads to significant elevation of bone mineral density, bone mass and strength of the bones (112, 113). Because of this, PTH (1–34) or teriparatide has been used in the management of adult patients with osteoporosis to increase bone mass and prevent bone fracture (114–117).
The Food and Drug Administration (FDA) approved teriparatide Eli Lilly & Co. (Indianapolis, IN, USA) as a treatment for osteoporosis under the name “Forteo” in November 2002 (118). The approval of this drug came after preclinical and clinical trials produced some conflicting results. Data from preclinical trials revealed that a high number of rodents developed OS after the treatment with very large doses of teriparatide for most of their lifespan. For this reason, the FDA was required to balance the possible side effects with the vital benefits of this distinctive product (118). In addition, teriparatide is not used to treat patients affected by primary malignant and metastatic bone tumors (119), Paget's disease (120) or who have had radiotherapy (121). All these conditions may increase the probability of OS development in patient treated with teriparatide (122).
Watanabe et al. showed that the induction of OS in rats treated with teriparatide depends on the duration and dose of treatment (123). In 2004, Vahle et al. described a safe regime of teriparatide for rats (124), starting with 5 μg/kg at 6 months of age and continued for either six or 20 months (up to 70% of life span) resulted in significant increase in bone mass with no development of neoplasms (124).
In humans, two cases of OS after treatment with teriparatide have been reported in the USA (122). Nevertheless, in the first case, the connection between teriparatide and the OS was not clear (121). In the second case, the patient was treated with radiotherapy before treated with teriparatide; thus, it is uncertain whether the teriparatide treatment or radiotherapy was associated with development of OS (119). Recently, another patient developed OS after administration of teriparatide (122). This patient had no history of Paget's disease and had never received any radiotherapy. According to Ogawa et al. (122), this case was the first case with definite correlation between teriparatide and acceleration of growth of a pre-existing malignant tumor in humans.
Hyaluronan (HA) is a glycosaminoglycan component of the extracellular matrix. It is involved is regulation of cancer cell function (125, 126). It has been found that PTH increases the production of HA in osteoblast-like OS cell line (UMR 106-01 BSP) (127). Furthermore, as a response to PTH, endosteal and periosteal osteoblastic cells exhibited metabolic variances in their HA synthesis (128). It is suggested that PTH (1–34) has a role in an administration mode-dependent manner, on HA metabolism that is vital for migration of OS cell (98). This role is correlated with OS cell differentiation and behavior (98). Treatment of aggressive and poorly differentiated MG-63 cells with intermittent PTH (1–34) was found to increase expression of their HA-synthase-2, which lead to enhanced high-molecular size HA deposition in the pericellular matrix and increased migration of these cells. Continuous treatment of well-differentiated Saos2 cell with PTH (1–34) also increased the production of HA and modestly stimulated their migration (98). Another study showed that the anabolic effect of PTH (1–34) on bone metabolism was associated with changes in fibroblast growth factor-2 (FGF-2) expression (129). These FGF variations could modify the nuclear accumulation and subsequent action of runt-related transcription factor 2 (Runx-2) and CREB transcription factors which are important in the regulation of osteoblast differentiation and growth (129).
Although the mechanism responsible for the rodent bone neoplasms is still a puzzle, it was suggested that the incidence of bone tumors is increased as a result of the prolonged treatment period in these rats in conjunction with an extreme response of the skeleton to the elevated bone formation effect of daily administration of teriparatide (110). Moreover, as mentioned previously, PTHR1 is activated by the binding of the N-terminal (1–34) amino acids of PTH or PTHrP (47). The abundant production of PTHrP which can bind to PTHR1 and promote the formation of cAMP could result in induction of OS as it will be discussed in the section “Roles of PTHR1 in OS” (130). Hypothetically, treatment with teriparatide and blocking of PTHR1 at the same time could reduce the possibility of OS induction. More studies are warranted to clarify the correlation between PTH, PTHR1, and OS.
Roles of PTHR1 in Normal and Tumor Tissues
PTHR1 is found mainly in bones and kidneys (131), and is involved in mineral ion homeostasis, bone turnover and skeletal development (132). In bone, PTHR1 regulates function, differentiation and proliferation of chondrocytes and osteoblasts (Figure 1) (133–135). It also controls calcium release from the matrix (136, 137).
In the kidney, PTHR1 has a role in the reabsorption of calcium in the distal convoluted tubule (46, 138) and in the maintenance of blood phosphate levels via inhibiting phosphate reabsorption in the distal and proximal tubules (139, 140). It also increases the activity of 1α-hydroxylase, resulting in increased calcium absorption from the intestine through increasing levels of 1,25-dihydroxycholecalciferol (46, 138).
Expression of PTHR1 protein has been detected in human primary tumors, including melanoma (100%), prostate adenocarcinoma (100%), colorectal carcinoma (100%), OS (50%), renal cell carcinoma (23%), and breast carcinoma (17%) (141). Studies showed that expression of PTHR1 was also detected in several human breast cancer cell lines (70, 142). Previously, Linforth et al. (143) found that expression of PTHR1 is correlated with poor prognosis in patients with primary breast cancer whilst Hoey et al. (144) reported that PTHR1 was highly expressed in human breast cancer bone metastases samples compared to primary breast cancer. The overexpression of PTHR1 in MCF-7 cells stimulated tumor cell proliferation through autocrine signals, which are mediated by cAMP and extracellular signal-regulated kinase (ERK) pathways (144).
In addition to PTHR1, recent studies have shown that overexpression of other GPCRs were associated with poor prognosis in pancreatic, breast and prostate cancers (145–147). Li et al. (146) found that increased expression of purinergic receptor P2Y2, a class A GPCR, correlated with a poor prognosis in prostate cancer. Moreover, protease-activated receptor 1 (PAR1), a second-class A GPCR, was reported to be highly expressed in aggressive breast tumors (146). Wang et al. (147) found that overexpression of GPR87, another class A GPCR, was linked with reduced survival for patients with pancreatic cancer. Furthermore, GPR87 was reported to promote aggressiveness in primary cell lines derived from the above patients' tumors (147). These data might support the carcinogenicity of PTHR1 and other GPCRs.
PTHR1 was not well-studied in cancers other than breast and OS. The next section highlights the critical roles of PTHR1 in OS.
Roles of PTHR1 in OS
Numerous studies using human cell lines (105), murine (99), human (141) and canine (39) tissues have reported the association between overexpression of PTHR1 and OS progression (Table 2). Mutsaers et al. (100) detected PTHR1 in primary and metastatic OS of osteoblastic and fibroblastic subtypes in vivo from two different types of transgenic mice. It has been suggested that increased expression of PTHR1 in OS could stimulate progression by formation of a more aggressive subtype (105).
PTHR1 mRNA is highly expressed in metastatic human OS compared with primary tumors (105). Overexpression of PTHR1 was linked with increased invasion and proliferation in 143B, U2OS, SaOD-2 and HOS cell lines (105). In addition, Ho et al. (99) reported that knockdown of PTHR1 in murine OS cells stimulated tumor differentiation and decreased invasion and growth. It has been found that reduced expression of PTHR1 in vivo enhanced mineralization and differentiation in OS (99).
Recently, immunostaining for PTHR1 was detected in all canine OS tissues (n = 50) (39). The findings showed that dogs with PTHR1 strongly staining OS tumors had significant shorter survival time compared to those with weakly staining tumors (39). According to this study, dogs with appendicular OS showing PTHR1 strong immunostaining lived for 212 days compared to those with weak immunostaining who lived for more than double the time (459 days). The conclusion was that expression of PTHR1 could be a significant prognostic indicator in canine OS (39). As was mentioned previously, the relationship between the expression of PTHR1 and survival time of OS patient has not yet studied in humans. However, recent experiments by the group at Liaoning Cancer Hospital showed that treatment of human Saos-2 and U2OS cell lines with mangiferin, a xanthonoid, decreased mRNA expression of PTHR1 in vitro (149). This study suggested that the inhibition of proliferation, migration and invasion of OS cells that resulted from this treatment are correlated with inhibition of PTHR1 (149). Moreover, a recent evidence revealed that blocking of PTHR1 in human Saos-2 and U2OS cell lines by using of Quercetin, a flavonoid found in vegetables, fruits, and grains, reduced metastatic cell invasion, proliferation, migration, and adhesion (148). These findings suggest that PTHR1 could be a novel and promising therapeutic target for OS.
The pathway of PTHR1 in tumorigenesis of OS was suggested by Walkley et al. (130). Under normal conditions, PTHrP binds and activates PTHR1which is located on the surface of osteoblasts. Activation of PTHR1 leads to the synthesis of cAMP from ATP via adenylyl cyclase. Consequently, cAMP induces the detachment of cAMP-dependent PKA from its α regulatory subunit of PKA type 1 (PRKAR1A) (130). Activated PKA translocates into the nucleus to phosphorylate and activates CREB. As a result, target genes downstream of PTHR1 signaling are activated (130). In OS, several abnormalities in the PTHrP-PTHR1-PKA pathway increased the activity of PKA pathway. This includes an elevated number of PTHR1 on the cell surface and increased expression of the Prkaca gene that encodes the catalytic component of PKA (130). Other abnormalities are increased production of PTHrP, which can bind to PTHR1 and promote the formation of cAMP and mutations in PRKAR1A gene, which result in an increase in the PKA activity (130).
A recent study carried by Li et al. (150) proposed that the effects of PTHR1 could be mediated by angiogenesis, inflammation and the Wnt pathway through altering the expression of the crucial enriched genes (Dkk1, Lef1, Agt-CCR3, and Agt-CCL9) using mouse OS cells.
Previous studies have reported that integrin adhesion molecules are involved in the migration of OS cells (151–153). Up-regulation of integrins including α5β1, α2β1, α6β1 (151), β1 (152) and αvβ3 (153) was associated with aggressive metastastic OS. PTHR1 could have a role in down-regulation or up-regulation of cell-cell or cell-extracellular matrix adhesion molecules. Integrins might be upregulated by PTHR1 in aggressive OS (Figure 4). To validate the current hypothesis and to further understand OS, future studies should investigate the correlation between PTHR1 and integrins in OS.
The results from all these studies taken together, show that detection of PTHR1 in OS could predict prognosis and therefore may be a potential therapeutic target.
The obvious question that may arise from this review is, why increased immunostaining of PTHR1 is correlated with reduced survival time, although dogs studied by Al-Khan et al. (39). had no clear evidence of metastasis at presentation in the smaller group (n = 20 dogs). This suggests that increased amounts of PTHR1 may activate tumor cells later to detach and metastasize to the lung, which leads to a reduced survival time (see Figure 4). The increase in PTHR1 in OS could be correlated with increasing the capability of tumor cells to metastasize and this was supported by a recent study (99). Knockdown of PTHR1 in OS reduced invasion of tumor cells in vitro (99). In addition, Yang et al. (105) revealed that overexpression of PTHR1 increased invasion and showed that metastatic OS had increased expression of PTHR1 mRNA compared to the primary tumor.
Cytoplasmic and Nuclear Localization of PTHrP and PTHR1 in OS
It has been found that full length PTHrP has a nuclear localization signal (NLS) that allows transport into the nucleus after binding to the transport regulatory protein, importin β in the cytoplasm (154). PTHR1 binds to both importin α1 and importin β (155). PTHR1 overexpression has been found in the nucleus during early interphase stage (G0/G1, S, and G2 phases) of the cell cycle in the following cell lines; SaOS-2 human OS, MC3T3-E1 mouse non-transformed osteoblasts and ROS 17/2.8 rat OS (155). At G0/G1, S, and G2 phases, DNA is more open to transcriptional activity compared to the later phases where DNA is compact, transcriptional activities are reduced and the immunofluorescent staining of PTHR1 was weaker (155).
The localization of PTHrP was observed in the cytoplasm of canine primary OS cells in 66% cases and in the nucleus plus the cytoplasm in 34% cases (n = 50 dogs) (39). Similarly, PTHrP was detected in the cytoplasm and nucleus using murine OS tissue (99) and human metastatic bone lesions in patients with prostate carcinoma (156). In contrast, PTHR1 was localized to the cytoplasmic plus nucleus of canine OS cells in 100% cases. Another study detected PTHR1 in the cytoplasm of murine OS cell (99), while it was detected also in the nucleus and cytoplasm of normal rat liver cells (157). The study of Al-Khan found that there was no significant correlation between the localization of PTHrP and PTHR1 and prognosis of OS in dogs (39). According to this study, the increased nuclear localization of PTHR1 in OS cells could be linked to the high rate of mitosis. Moreover, most of these cells are at stage G0 and G1.
On the other hand, it has been found that nuclear localization of PTHrP is correlated with inhibition of apoptosis using nine human and rat prostate cancer cell lines [PC-3, PC-3 MB, LNCaP, DU-145, AT-2.1, MLL, AT-3.1, MAT-Lu (ML), and GP9F3] (156). It is suggested that PTHrP has a vital role in the promotion of prostate tumor growth and/or progression (123). Another study revealed that nuclear localization of PTHrP promotes survival of chondrocytes under conditions that stimulate cell death using COS-7 cell line (158).
The only study that investigated the immuno-localization of PTHR1 in human OS cells did not mention the pattern of the immunostaining and they used only four cases of OS (141). The study of Al-Khan et al. (39) is the only immunohistochemical study that investigated the localization of PTHR1 in canine OS. More studies are warranted to confirm the present findings.
Conclusion
In conclusion, this review has shown that canine OS is a good model for the human disease and highlighted the roles of PTHrP and PTHR1 in normal tissue and in OS. Both PTHrP and PTHR1 are crucial factors for induction of OS. Increased expression of these two proteins in OS is correlated with a poor prognosis. PTHrP and PTHR1 play critical roles in pulmonary metastasis, chemoresistance, tumor growth and decreased apoptosis in OS patients. Although the function of these two proteins in bone, breast, placenta, and kidney has been described, their evolving roles in the pathogenesis of OS requires further investigation. This review supported the proposition that PTHR1 could be a novel and significant prognostic indicator in OS and both PTHrP and PTHR1 could be targets for novel therapeutics for OS. Also, future studies on the correlation between increased expression of PTHR1 and integrins may improve our understanding of OS progression via the discovery of novel signaling pathways that could be manipulated to improve patient outcomes.
Author Contributions
AA-K reviewed the literature and wrote the manuscript. JD, SR, and NA wrote and edited the manuscript. All authors read and approved the final version of the manuscript.
Funding
This review was based on research carried out during an Australian National Health and Medical Research Council Project Grant 620203. We acknowledge the scholarship support given by The Ministry of Higher Education in Oman, The Consulate General of the Sultanate of Oman in Melbourne to AA-K and the School of Health and Biomedical Sciences at RMIT University.
Conflict of Interest
The authors declare that the research was conducted in the absence of any commercial or financial relationships that could be construed as a potential conflict of interest.
References
1. Dahlin DC. Pathology of osteosarcoma. Clin Orthop Relat Res. (1975) 111:23–32. doi: 10.1097/00003086-197509000-00004
2. Lewis RJ, Lotz MJ. Proceedings: medullary extension of osteosarcoma. Implications for rational therapy. Cancer. (1974) 33:371–5. doi: 10.1002/1097-0142(197402)33:2<371::AID-CNCR2820330211>3.0.CO;2-8
3. Bielack S, Carrle D, Casali PG. Osteosarcoma: ESMO clinical recommendations for diagnosis, treatment and follow-up. Ann Oncol. (2009) 20:137–9. doi: 10.1093/annonc/mdp154
4. Mohseny AB, Szuhai K, Romeo S, Buddingh EP, Briaire-de Bruijn I, de Jong D, et al. Osteosarcoma originates from mesenchymal stem cells in consequence of aneuploidization and genomic loss of Cdkn2. J Pathol. (2009) 219:294–305. doi: 10.1002/path.2603
5. Xiao W, Mohseny AB, Hogendoorn PC, Cleton-Jansen AM. Mesenchymal stem cell transformation and sarcoma genesis. Clin Sarcoma Res. (2013) 3:10. doi: 10.1186/2045-3329-3-10
6. Lee V. Bone tumours in childhood and adolescence. Paediatrics Child Health. (2014) 24:143–7. doi: 10.1016/j.paed.2014.02.008
7. Raymond AK, Ayala AG, Knuutila S. Conventional osteosarcoma. In: Fletcher CDM, Unni KK, Mertens F, editors. Pathology and Genetics of Tumours of Soft Tissue and Bone. 1st ed. Lyon: IARC Press. (2002). p. 264–70.
8. Brodey RS, Riser WH. Canine osteosarcoma: a clinicopathologic study of 194 cases. Clin Orthop Relat Res. (1969) 62:54–64. doi: 10.1097/00003086-196901000-00008
9. Mirabello L, Pfeiffer R, Murphy G, Daw NC, Patino-Garcia A, Troisi RJ, et al. Height at diagnosis and birth-weight as risk factors for osteosarcoma. Cancer Causes Control. (2011) 22:899–908. doi: 10.1007/s10552-011-9763-2
10. Kansara M, Teng MW, Smyth MJ, Thomas DM. Translational biology of osteosarcoma. Nat Rev Cancer. (2014) 14:722–35. doi: 10.1038/nrc3838
11. Yang Y, Han L, He Z, Li X, Yang S, Yang J, et al. Advances in limb salvage treatment of osteosarcoma. J Bone Oncol. (2018) 10:36–40. doi: 10.1016/j.jbo.2017.11.005
12. Sapierzynski R, Czopowicz M. The animal-dependent risk factors in canine osteosarcomas. Pol J Vet Sci. (2017) 20:293–8. doi: 10.1515/pjvs-2017-0035
13. Mirabello L, Troisi RJ, Savage SA. Osteosarcoma incidence and survival rates from 1973 to 2004: data from the surveillance, epidemiology, and end results program. Cancer. (2009) 115:1531–43. doi: 10.1002/cncr.24121
14. Dorfman H, Vanel D, Czerniak B, Park Y, Kotz R, Unni K. WHO classification of bone tumours. In: Fletcher CD, Unni K, Mertens F, editors. Pathology and Genetics of Tumours of Soft Tissue and Bone. Lyon: IARC Press. (2002). p. 225-32.
15. Ekhtiari S, Chiba K, Popovic S, Crowther R, Wohl G, Wong A, et al. First case of osteosarcoma in a dinosaur: a multimodal diagnosis. Lancet. (2020) 21:1021–2. doi: 10.1016/S1470-2045(20)30171-6
16. Haridy Y, Witzmann F, Asbach P, Schoch RR, Forbisch N, Rotheschild BM. Triassic cancer-osteosracome in a 240-million-year-old stem turtle. JAMA Oncol. (2019) 5:425–6. doi: 10.1001/jamaoncol.2018.6766
17. Chou AJ, Kleinerman ES, Krailo MD, Chen Z, Betcher DL, Healey JH, et al. Addition of muramyl tripeptide to chemotherapy for patients with newly diagnosed metastatic osteosarcoma: a report from the Children's Oncology Group. Cancer. (2009) 115:5339–48. doi: 10.1002/cncr.24566
18. Lewis VO, Devarajan E, Cardo-Vila M, Thomas DG, Kleinerman ES, Marchio S, et al. BMTP-11 is active in preclinical models of human osteosarcoma and a candidate targeted drug for clinical translation. Proc Natl Acad Sci USA. (2017) 114:8065–70. doi: 10.1073/pnas.1704173114
19. Bishop MW, Janeway KA, Gorlick R. Future directions in the treatment of osteosarcoma. Curr Opin Pediatr. (2016) 28:26–33. doi: 10.1097/MOP.0000000000000298
20. Lindsey BA, Markel JE, Kleinerman ES. Osteosarcoma overview. Rheumatol Ther. (2017) 4:25–43. doi: 10.1007/s40744-016-0050-2
21. Varshney J, Scott MC, Largaespada DA, Subramanian S. Understanding the osteosarcoma pathobiology: a comparative oncology approach. Vet Sci. (2016) 3:3. doi: 10.3390/vetsci3010003
22. Lau CC. Molecular classification of osteosarcoma. Cancer Treat Res. (2009) 152:459–65. doi: 10.1007/978-1-4419-0284-9_26
23. Morello E, Martano M, Buracco P. Biology, diagnosis and treatment of canine appendicular osteosarcoma: similarities and differences with human osteosarcoma. Vet J. (2011) 189:268–77. doi: 10.1016/j.tvjl.2010.08.014
24. Bielack SS, Kempf-Bielack B, Delling G, Exner GU, Flege S, Helmke K, et al. Prognostic factors in high-grade osteosarcoma of the extremities or trunk: an analysis of 1,702 patients treated on neoadjuvant cooperative osteosarcoma study group protocols. J Clin Oncol. (2002) 20:776–90. doi: 10.1200/JCO.2002.20.3.776
25. Serra M, Hattinger CM. The pharmacogenomics of osteosarcoma. Pharmacogenomics J. (2017) 17:11–20. doi: 10.1038/tpj.2016.45
26. Coventry MB, Dahlin DC. Osteogenic sarcoma: a critical analysis of 430 cases. J Bone Joint Surg Am. (1957) 39:741–58. doi: 10.2106/00004623-195739040-00002
27. Bacci G, Longhi A, Versari M, Mercuri M, Briccoli A, Picci P. Prognostic factors for osteosarcoma of the extremity treated with neoadjuvant chemotherapy: 15-year experience in 789 patients treated at a single institution. Cancer. (2006) 106:1154–61. doi: 10.1002/cncr.21724
28. Fenger JM, London CA, Kisseberth WC. Canine osteosarcoma: a naturally occurring disease to inform pediatric oncology. Ilar j. (2014) 55:69–85. doi: 10.1093/ilar/ilu009
29. Saha D, Saha K, Banerjee A, Jash D. Osteosarcoma relapse as pleural metastasis. South Asian J Cancer. (2013) 2:56. doi: 10.4103/2278-330X.110483
31. Allison DC, Carney SC, Ahlmann ER, Hendifar A, Chawla S, Fedenko A, et al. A meta-analysis of osteosarcoma outcomes in the modern medical era. Sarcoma. (2012) 2012:704872. doi: 10.1155/2012/704872
32. Harris MB, Gieser P, Goorin AM, Ayala A, Shochat SJ, Ferguson WS, et al. Treatment of metastatic osteosarcoma at diagnosis: a Pediatric Oncology Group Study. J Clin Oncol. (1998) 16:3641–8. doi: 10.1200/JCO.1998.16.11.3641
33. Meyers PA, Schwartz CL, Krailo M, Kleinerman ES, Betcher D, Bernstein ML, et al. Osteosarcoma: a randomized, prospective trial of the addition of ifosfamide and/or muramyl tripeptide to cisplatin, doxorubicin, and high-dose methotrexate. J Clin Oncol. (2005) 23:2004–11. doi: 10.1200/JCO.2005.06.031
34. Withrow SJ, Powers BE, Straw RC, Wilkins RM. Comparative aspects of osteosarcoma. Dog vs. man. Clin Orthop Relat Res. (1991) 1991:159–68. doi: 10.1097/00003086-199109000-00023
35. Khanna C, Wan X, Bose S, Cassaday R, Olomu O, Mendoza A, et al. The membrane-cytoskeleton linker ezrin is necessary for osteosarcoma metastasis. Nat Med. (2004) 10:182–6. doi: 10.1038/nm982
36. Hong SH, Osborne T, Ren L, Briggs J, Mazcko C, Burkett SS, et al. Protein kinase C regulates ezrin-radixin-moesin phosphorylation in canine osteosarcoma cells. Vet Comp Oncol. (2011) 9:207–18. doi: 10.1111/j.1476-5829.2010.00249.x
37. Jaroensong T, Endo Y, Lee SJ, Kamida A, Mochizuki M, Nishimura R, et al. Effects of transplantation sites on tumour growth, pulmonary metastasis and ezrin expression of canine osteosarcoma cell lines in nude mice. Vet Comp Oncol. (2012) 10:274–82. doi: 10.1111/j.1476-5829.2011.00294.x
38. Hagleitner MM, Hoogerbrugge PM, van der Graaf WT, Flucke U, Schreuder HW, te Loo DM. Age as prognostic factor in patients with osteosarcoma. Bone. (2011) 49:1173–7. doi: 10.1016/j.bone.2011.08.014
39. Al-Khan AA, Nimmo JS, Tayebi M, Ryan SD, Simcock JO, Tarzi R, et al. Parathyroid hormone receptor 1 (PTHR1) is a prognostic indicator in canine osteosarcoma. Sci Rep. (2020) 10:1564. doi: 10.1038/s41598-020-58524-3
40. Strewler GJ, Stern PH, Jacobs JW, Eveloff J, Klein RF, Leung SC, et al. Parathyroid hormonelike protein from human renal carcinoma cells. Structural and functional homology with parathyroid hormone. J Clin Investigation. (1987) 80:1803–7. doi: 10.1172/JCI113275
41. Suva LJ, Winslow GA, Wettenhall RE, Hammonds RG, Moseley JM, Diefenbach-Jagger H, et al. A parathyroid hormone-related protein implicated in malignant hypercalcemia: cloning and expression. Science. (1987) 237:893–6. doi: 10.1126/science.3616618
42. Stewart AF, Horst R, Deftos LJ, Cadman EC, Lang R, Broadus AE. Biochemical evaluation of patients with cancer-associated hypercalcemia: evidence for humoral and nonhumoral groups. N Engl J Med. (1980) 303:1377–83. doi: 10.1056/NEJM198012113032401
43. Moseley JM, Kubota M, Diefenbach-Jagger H, Wettenhall RE, Kemp BE, Suva LJ, et al. Parathyroid hormone-related protein purified from a human lung cancer cell line. Proc Natl Acad Sci USA. (1987) 84:5048–52. doi: 10.1073/pnas.84.14.5048
44. Brandt DW, Wachsman W, Deftos LJ. Parathyroid hormone-like protein: alternative messenger RNA splicing pathways in human cancer cell lines. Cancer Res. (1994) 54:850–3.
45. Juppner H, Abou-Samra AB, Freeman M, Kong XF, Schipani E, Richards J, et al. A G protein-linked receptor for parathyroid hormone and parathyroid hormone-related peptide. Science. (1991) 254:1024–6. doi: 10.1126/science.1658941
46. Gensure RC, Gardella TJ, Juppner H. Parathyroid hormone and parathyroid hormone-related peptide, and their receptors. Biochem Biophys Res Commun. (2005) 328:666–78. doi: 10.1016/j.bbrc.2004.11.069
48. Hoare SR. Mechanisms of peptide and nonpeptide ligand binding to Class B G-protein-coupled receptors. Drug Discov Today. (2005) 10:417–27. doi: 10.1016/S1359-6446(05)03370-2
49. Gardella TJ, Juppner H, Wilson AK, Keutmann HT, Abou-Samra AB, Segre GV, et al. Determinants of (Arg2)PTH-(1-34) binding and signaling in the transmembrane region of the parathyroid hormone receptor. Endocrinology. (1994) 135:1186–94. doi: 10.1210/endo.135.3.8070362
50. Luck MD, Carter PH, Gardella TJ. The (1-14) fragment of parathyroid hormone (PTH) activates intact and amino-terminally truncated PTH-1 receptors. Mol Endocrinol. (1999) 13:670–80. doi: 10.1210/mend.13.5.0277
51. Shimizu M, Carter PH, Gardella TJ. Autoactivation of type-1 parathyroid hormone receptors containing a tethered ligand. J Biol Chem. (2000) 275:19456–60. doi: 10.1074/jbc.M001596200
52. Castro M, Nikolaev VO, Palm D, Lohse MJ, Vilardaga JP. Turn-on switch in parathyroid hormone receptor by a two-step parathyroid hormone binding mechanism. Proc Natl Acad Sci USA. (2005) 102:16084–9. doi: 10.1073/pnas.0503942102
53. Caulfield MP, McKee RL, Goldman ME, Duong LT, Fisher JE, Gay CT, et al. The bovine renal parathyroid hormone (PTH) receptor has equal affinity for two different amino acid sequences: the receptor binding domains of PTH and PTH-related protein are located within the 14-34 region. Endocrinology. (1990) 127:83–7. doi: 10.1210/endo-127-1-83
54. Juppner H, Schipani E, Bringhurst FR, McClure I, Keutmann HT, Potts JT, et al. The extracellular amino-terminal region of the parathyroid hormone (PTH)/PTH-related peptide receptor determines the binding affinity for carboxyl-terminal fragments of PTH-(1-34). Endocrinology. (1994) 134:879–84. doi: 10.1210/endo.134.2.8299582
55. Schwindinger WF, Fredericks J, Watkins L, Robinson H, Bathon JM, Pines M, et al. Coupling of the PTH/PTHrP receptor to multiple G-proteins: direct demonstration of receptor activation of Gs, Gq/11, and Gi(1) by (alpha-32P)GTP-gamma-azidoanilide photoaffinity labeling. Endocrine. (1998) 8:201–9. doi: 10.1385/ENDO:8:2:201
56. Abou-Samra AB, Juppner H, Force T, Freeman MW, Kong XF, Schipani E, et al. Expression cloning of a common receptor for parathyroid hormone and parathyroid hormone-related peptide from rat osteoblast-like cells: a single receptor stimulates intracellular accumulation of both cAMP and inositol trisphosphates and increases intracellular free calcium. Proc Natl Acad Sci USA. (1992) 89:2732–6. doi: 10.1073/pnas.89.7.2732
57. Offermanns S, Iida-Klein A, Segre GV, Simon MI. G alpha q family members couple parathyroid hormone (PTH)/PTH-related peptide and calcitonin receptors to phospholipase C in COS-7 cells. Mol Endocrinol. (1996) 10:566–74. doi: 10.1210/me.10.5.566
58. Mannstadt M, Juppner H, Gardella TJ. Receptors for PTH and PTHrP: their biological importance and functional properties. Am J Physiol. (1999) 277(5 Pt 2):665–75. doi: 10.1152/ajprenal.1999.277.5.F665
59. Danks JA, D'Souza DG, Gunn HJ, Milley KM, Richardson SJ. Evolution of the parathyroid hormone family and skeletal formation pathways. Gen Comp Endocrinol. (2011) 170:79–91. doi: 10.1016/j.ygcen.2010.10.023
60. Kano J, Sugimoto T, Fukase M, Chihara K. The direct involvement of cAMP-dependent protein kinase in the regulation of collagen synthesis by parathyroid hormone (PTH) and PTH-related peptide in osteoblast-like osteosarcoma cells (UMR-106). Biochem Biophys Res Commun. (1992) 184:525–9. doi: 10.1016/0006-291X(92)91226-G
61. Kronenberg HM. Developmental regulation of the growth plate. Nature. (2003) 423:332–6. doi: 10.1038/nature01657
62. VanHouten J, Dann P, McGeoch G, Brown EM, Krapcho K, Neville M, et al. The calcium-sensing receptor regulates mammary gland parathyroid hormone-related protein production and calcium transport. J Clin Investigation. (2004) 113:598–608. doi: 10.1172/JCI200418776
63. Rodda CP, Kubota M, Heath JA, Ebeling PR, Moseley JM, Care AD, et al. Evidence for a novel parathyroid hormone-related protein in fetal lamb parathyroid glands and sheep placenta: comparisons with a similar protein implicated in humoral hypercalcaemia of malignancy. J Endocrinol. (1988) 117:261–71. doi: 10.1677/joe.0.1170261
64. Kovacs CS, Lanske B, Hunzelman JL, Guo J, Karaplis AC, Kronenberg HM. Parathyroid hormone-related peptide (PTHrP) regulates fetal-placental calcium transport through a receptor distinct from the PTH/PTHrP receptor. Proc Natl Acad Sci USA. (1996) 93:15233–8. doi: 10.1073/pnas.93.26.15233
65. Hastings RH, Montgrain PR, Quintana RA, Chobrutskiy B, Davani A, Miyanohara A, et al. Lung carcinoma progression and survival vs. amino- and carboxyl-parathyroid hormone-related protein expression. J Cancer Res Clin Oncol. (2017) 143:1395–407. doi: 10.1007/s00432-017-2396-4
66. Kim W, Takyar FM, Swan K, Jeong J, VanHouten J, Sullivan C, et al. Calcium-sensing receptor promotes breast cancer by stimulating intracrine actions of parathyroid hormone-related protein. Cancer Res. (2016) 76:5348–60. doi: 10.1158/0008-5472.CAN-15-2614
67. Garcia-Martin A, Ardura JA, Maycas M, Lozano D, Lopez-Herradon A, Portal-Nunez S, et al. Functional roles of the nuclear localization signal of parathyroid hormone-related protein (PTHrP) in osteoblastic cells. Mol Endocrinol. (2014) 28:925–34. doi: 10.1210/me.2013-1225
68. Neville MC, McFadden TB, Forsyth I. Hormonal regulation of mammary differentiation and milk secretion. J Mammary Gland Biol Neoplasia. (2002) 7:49–66. doi: 10.1023/A:1015770423167
69. Luparello C, Burtis WJ, Raue F, Birch MA, Gallagher JA. Parathyroid hormone-related peptide and 8701-BC breast cancer cell growth and invasion in vitro: evidence for growth-inhibiting and invasion-promoting effects. Mol Cell Endocrinol. (1995) 111:225–32. doi: 10.1016/0303-7207(95)03577-T
70. Cataisson C, Lieberherr M, Cros M, Gauville C, Graulet AM, Cotton J, et al. Parathyroid hormone-related peptide stimulates proliferation of highly tumorigenic human SV40-immortalized breast epithelial cells. J Bone Mineral Res. (2000) 15:2129–39. doi: 10.1359/jbmr.2000.15.11.2129
71. Hiraki A, Ueoka H, Bessho A, Segawa Y, Takigawa N, Kiura K, et al. Parathyroid hormone-related protein measured at the time of first visit is an indicator of bone metastases and survival in lung carcinoma patients with hypercalcemia. Cancer. (2002) 95:1706–13. doi: 10.1002/cncr.10828
72. Rabbani SA, Khalili P, Arakelian A, Pizzi H, Chen G, Goltzman D. Regulation of parathyroid hormone-related peptide by estradiol: effect on tumor growth and metastasis in vitro and in vivo. Endocrinology. (2005) 146:2885–94. doi: 10.1210/en.2005-0062
73. Maioli E, Fortino V, Torricelli C. PTHrP/PTH1R: a complex crosstalk among diffrent signalling pathways. In: Luparello C, editor. Novel Aspects of PTHrP Physiopathology. 1st ed. New York, NY: Nova Science Publishers (2007). p. 143–64.
74. Zheng L, Zhu K, Jiao H, Zhao Z, Zhang L, Liu M, et al. PTHrP expression in human MDA-MB-231 breast cancer cells is critical for tumor growth and survival and osteoblast inhibition. Int J Biol Sci. (2013) 9:830–41. doi: 10.7150/ijbs.7039
75. Li J, Karaplis AC, Huang DC, Siegel PM, Camirand A, Yang XF, et al. PTHrP drives breast tumor initiation, progression, and metastasis in mice and is a potential therapy target. J Clin Investigation. (2011) 121:4655–69. doi: 10.1172/JCI46134
76. Iguchi H, Tanaka S, Ozawa Y, Kashiwakuma T, Kimura T, Hiraga T, et al. An experimental model of bone metastasis by human lung cancer cells: the role of parathyroid hormone-related protein in bone metastasis. Cancer Res. (1996) 56:4040–3.
77. Hofbauer LC, Schoppet M. Clinical implications of the osteoprotegerin/RANKL/RANK system for bone and vascular diseases. JAMA. (2004) 292:490–5. doi: 10.1001/jama.292.4.490
78. Southby J, Kissin MW, Danks JA, Hayman JA, Moseley JM, Henderson MA, et al. Immunohistochemical localization of parathyroid hormone-related protein in human breast cancer. Cancer Res. (1990) 50:7710–6. doi: 10.1016/S0031-3025(16)36341-3
79. Powell GJ, Southby J, Danks JA, Stillwell RG, Hayman JA, Henderson MA, et al. Localization of parathyroid hormone-related protein in breast cancer metastases: increased incidence in bone compared with other sites. Cancer Res. (1991) 51:3059–61.
80. Miyaji T, Nakase T, Onuma E, Sato K, Myoui A, Tomita T, et al. Monoclonal antibody to parathyroid hormone-related protein induces differentiation and apoptosis of chondrosarcoma cells. Cancer Lett. (2003) 199:147–55. doi: 10.1016/S0304-3835(03)00347-1
81. Dackiw A, Pan J, Xu G, Yeung SC. Modulation of parathyroid hormone-related protein levels (PTHrP) in anaplastic thyroid cancer. Surgery. (2005) 138:456–63. doi: 10.1016/j.surg.2005.06.033
82. Gessi M, Monego G, Calviello G, Lanza P, Giangaspero F, Silvestrini A, et al. Human parathyroid hormone-related protein and human parathyroid hormone receptor type 1 are expressed in human medulloblastomas and regulate cell proliferation and apoptosis in medulloblastoma-derived cell lines. Acta Neuropathol. (2007) 114:135–45. doi: 10.1007/s00401-007-0212-y
83. Rizk-Rabin M, Assie G, Rene-Corail F, Perlemoine K, Hamzaoui H, Tissier F, et al. Differential expression of parathyroid hormone-related protein in adrenocortical tumors: autocrine/paracrine effects on the growth and signaling pathways in H295R cells. Cancer Epidemiol Biomarkers Prev. (2008) 17:2275–85. doi: 10.1158/1055-9965.EPI-07-2924
84. Yamada T, Tsuda M, Ohba Y, Kawaguchi H, Totsuka Y, Shindoh M. PTHrP promotes malignancy of human oral cancer cell downstream of the EGFR signaling. Biochem Biophys Res Commun. (2008) 368:575–81. doi: 10.1016/j.bbrc.2008.01.121
85. Parri M, Chiarugi P. Rac and Rho GTPases in cancer cell motility control. Cell Commun Signal. (2010) 8:23. doi: 10.1186/1478-811X-8-23
86. Bhatia V, Mula RV, Weigel NL, Falzon M. Parathyroid hormone-related protein regulates cell survival pathways via integrin alpha6beta4-mediated activation of phosphatidylinositol 3-kinase/Akt signaling. Mol Cancer Res. (2009) 7:1119–31. doi: 10.1158/1541-7786.MCR-08-0568
87. Talon I, Lindner V, Sourbier C, Schordan E, Rothhut S, Barthelmebs M, et al. Antitumor effect of parathyroid hormone-related protein neutralizing antibody in human renal cell carcinoma in vitro and in vivo. Carcinogenesis. (2006) 27:73–83. doi: 10.1093/carcin/bgi203
88. Massfelder T, Lang H, Schordan E, Lindner V, Rothhut S, Welsch S, et al. Parathyroid hormone-related protein is an essential growth factor for human clear cell renal carcinoma and a target for the von Hippel-Lindau tumor suppressor gene. Cancer Res. (2004) 64:180–8. doi: 10.1158/0008-5472.CAN-03-1968
89. Danilin S, Sourbier C, Thomas L, Rothhut S, Lindner V, Helwig JJ, et al. von Hippel-Lindau tumor suppressor gene-dependent mRNA stabilization of the survival factor parathyroid hormone-related protein in human renal cell carcinoma by the RNA-binding protein HuR. Carcinogenesis. (2009) 30:387–96. doi: 10.1093/carcin/bgn275
90. Yamada R, Tanaka K, Inoue H, Sakuno T, Harada T, Yoshizawa N, et al. Pancreatic adenocarcinoma producing parathyroid hormone-related protein. Case Rep Oncol Med. (2017) 2017:5656130. doi: 10.1155/2017/5656130
91. Takeda K, Kimura R, Nishigaki N, Sato S, Okamoto A, Watanabe K, et al. Humoral hypercalcemia of malignancy with a parathyroid hormone-related peptide-secreting intrahepatic cholangiocarcinoma accompanied by a gastric cancer. Case Rep Endocrinol. (2017) 2017:7012520. doi: 10.1155/2017/7012520
92. Symington M, Davies L, Kaltsas G, Weickert MO. Malignant hypercalcaemia related to parathyroid hormone-related peptide (PTHrP) secretion from a metastatic pancreatic neuroendocrine tumour (NET). BMJ Case Rep. (2017) 2017:bcr2017219692. doi: 10.1136/bcr-2017-219692
93. Kir S, White JP, Kleiner S, Kazak L, Cohen P, Baracos VE, et al. Tumour-derived PTH-related protein triggers adipose tissue browning and cancer cachexia. Nature. (2014) 513:100–4. doi: 10.1038/nature13528
94. Martin TJ, Ingleton PM, Underwood JC, Michelangeli VP, Hunt NH, Melick RA. Parathyroid hormone-responsive adenylate cyclase in induced transplantable osteogenic rat sarcoma. Nature. (1976) 260:436–8. doi: 10.1038/260436a0
95. Al-Khan A, Gunn H, Day M, Tayebi M, Ryan S, Kuntz C, et al. Immunohistochemical validation of spontaneously arising canine osteosarcoma as a model for human osteosarcoma. J Comparative Pathol. (2017) 157:256–65. doi: 10.1016/j.jcpa.2017.07.005
96. Pasquini GM, Davey RA, Ho PW, Michelangeli VP, Grill V, Kaczmarczyk SJ, et al. Local secretion of parathyroid hormone-related protein by an osteoblastic osteosarcoma (UMR 106-01) cell line results in growth inhibition. Bone. (2002) 31:598–605. doi: 10.1016/S8756-3282(02)00872-4
97. Gagiannis S, Muller M, Uhlemann S, Koch A, Melino G, Krammer PH, et al. Parathyroid hormone-related protein confers chemoresistance by blocking apoptosis signaling via death receptors and mitochondria. Int J Cancer. (2009) 125:1551–7. doi: 10.1002/ijc.24471
98. Berdiaki A, Datsis GA, Nikitovic D, Tsatsakis A, Katonis P, Karamanos NK, et al. Parathyroid hormone (PTH) peptides through the regulation of hyaluronan metabolism affect osteosarcoma cell migration. IUBMB life. (2010) 62:377–86. doi: 10.1002/iub.320
99. Ho PW, Goradia A, Russell MR, Chalk AM, Milley KM, Baker EK, et al. Knockdown of PTHR1 in osteosarcoma cells decreases invasion and growth and increases tumor differentiation in vivo. Oncogene. (2015) 34:2922–33. doi: 10.1038/onc.2014.217
100. Mutsaers AJ, Ng AJ, Baker EK, Russell MR, Chalk AM, Wall M, et al. Modeling distinct osteosarcoma subtypes in vivo using Cre:lox and lineage-restricted transgenic shRNA. Bone. (2013) 55:166–78. doi: 10.1016/j.bone.2013.02.016
101. Rodan SB, Wesolowski G, Ianacone J, Thiede MA, Rodan GA. Production of parathyroid hormone-like peptide in a human osteosarcoma cell line: stimulation by phorbol esters and epidermal growth factor. J Endocrinol. (1989) 122:219–27. doi: 10.1677/joe.0.1220219
102. Suda N, Gillespie MT, Traianedes K, Zhou H, Ho PW, Hards DK, et al. Expression of parathyroid hormone-related protein in cells of osteoblast lineage. J Cell Physiol. (1996) 166:94–104. doi: 10.1002/(SICI)1097-4652(199601)166:1<94::AID-JCP11>3.0.CO;2-P
103. Walsh CA, Bowler WB, Bilbe G, Fraser WD, Gallagher JA. Effects of PTH on PTHrP gene expression in human osteoblasts: up-regulation with the kinetics of an immediate early gene. Biochem Biophys Res Commun. (1997) 239:155–9. doi: 10.1006/bbrc.1997.7351
104. Jemtland R, Rian E, Olstad OK, Haug E, Bruland OS, Bucht E, et al. Two human osteoblast-like osteosarcoma cell lines show distinct expression and differential regulation of parathyroid hormone-related protein. J Bone Mineral Res. (1999) 14:904–14. doi: 10.1359/jbmr.1999.14.6.904
105. Yang R, Hoang BH, Kubo T, Kawano H, Chou A, Sowers R, et al. Over-expression of parathyroid hormone Type 1 receptor confers an aggressive phenotype in osteosarcoma. Int J Cancer. (2007) 121:943–54. doi: 10.1002/ijc.22749
106. Motomura K, Ohtsuru A, Enomoto H, Tsukazaki T, Namba H, Tsuji Y, et al. Osteogenic action of parathyroid hormone-related peptide (1-141) in rat ROS cells. Endocr J. (1996) 43:527–35. doi: 10.1507/endocrj.43.527
107. Martin TJ. Parathyroid hormone-related protein, its regulation of cartilage and bone development, and role in treating bone diseases. Physiol Rev. (2016) 96:831–71. doi: 10.1152/physrev.00031.2015
108. Walia MK, Ho PM, Taylor S, Ng AJ, Gupte A, Chalk AM, et al. Activation of PTHrP-cAMP-CREB1 signaling following p53 loss is essential for osteosarcoma initiation and maintenance. Elife. (2016) 5:e13446. doi: 10.7554/eLife.13446
109. O'Riordan JL, Woodhead JS, Robinson CJ, Parsons JA, Keutmann H, Niall H, et al. Structure-function studies in parathyroid hormone. Proc Royal Soc Med. (1971) 64:1263–5. doi: 10.1177/003591577106401243
110. Vahle JL, Sato M, Long GG, Young JK, Francis PC, Engelhardt JA, et al. Skeletal changes in rats given daily subcutaneous injections of recombinant human parathyroid hormone (1-34) for 2 years and relevance to human safety. Toxicol Pathol. (2002) 30:312–21. doi: 10.1080/01926230252929882
111. McCauley LK, Martin TJ. Twenty-five years of PTHrP progress: from cancer hormone to multifunctional cytokine. J Bone Mineral Res. (2012) 27:1231–9. doi: 10.1002/jbmr.1617
112. Dempster DW, Cosman F, Parisien M, Shen V, Lindsay R. Anabolic actions of parathyroid hormone on bone. Endocr Rev. (1993) 14:690–709. doi: 10.1210/edrv-14-6-690
113. Sato M, Zeng GQ, Turner CH. Biosynthetic human parathyroid hormone (1-34) effects on bone quality in aged ovariectomized rats. Endocrinology. (1997) 138:4330–7. doi: 10.1210/endo.138.10.5440
114. Alkhiary YM, Gerstenfeld LC, Krall E, Westmore M, Sato M, Mitlak BH, et al. Enhancement of experimental fracture-healing by systemic administration of recombinant human parathyroid hormone (PTH 1-34). J Bone Joint Surg Am. (2005) 87:731–41. doi: 10.2106/00004623-200504000-00006
115. Aspenberg P, Johansson T. Teriparatide improves early callus formation in distal radial fractures. Acta Orthop. (2010) 81:234–6. doi: 10.3109/17453671003761946
116. Della Rocca GJ, Crist BD, Murtha YM. Parathyroid hormone: is there a role in fracture healing? J Orthop Trauma. (2010) 24(Suppl. 1):S31–5. doi: 10.1097/BOT.0b013e3181cde5d1
117. Im GI, Lee SH. Effect of teriparatide on healing of atypical femoral fractures: a systemic review. J Bone Metab. (2015) 22:183–9. doi: 10.11005/jbm.2015.22.4.183
118. Tashjian AH Jr, Gagel RF. Teriparatide [human PTH(1-34)]: 2.5 years of experience on the use and safety of the drug for the treatment of osteoporosis. J Bone Mineral Res. (2006) 21:354–65. doi: 10.1359/JBMR.051023
119. Harper KD, Krege JH, Marcus R, Mitlak BH. Osteosarcoma and teriparatide? J Bone Mineral Res. (2007) 22:334. doi: 10.1359/jbmr.061111
120. Andrews EB, Gilsenan AW, Midkiff K, Sherrill B, Wu Y, Mann BH, et al. The US postmarketing surveillance study of adult osteosarcoma and teriparatide: study design and findings from the first 7 years. J Bone Mineral Res. (2012) 27:2429–37. doi: 10.1002/jbmr.1768
121. Subbiah V, Madsen VS, Raymond AK, Benjamin RS, Ludwig JA. Of mice and men: divergent risks of teriparatide-induced osteosarcoma. Osteoporos Int. (2010) 21:1041–5. doi: 10.1007/s00198-009-1004-0
122. Ogawa T, Ohshika S, Yanagisawa M, Kurose A, Ishibashi Y. Teriparatide may accelerate the growth of a pre-existing malignant tumor in an elderly patient with osteoporosis: a case report. Mol Clin Oncol. (2020) 12:144–7. doi: 10.3892/mco.2019.1966
123. Watanabe A, Yoneyama S, Nakajima M, Sato N, Takao-Kawabata R, Isogai Y, et al. Osteosarcoma in Sprague-Dawley rats after long-term treatment with teriparatide (human parathyroid hormone (1-34)). J Toxicol Sci. (2012) 37:617–29. doi: 10.2131/jts.37.617
124. Vahle JL, Long GG, Sandusky G, Westmore M, Ma YL, Sato M. Bone neoplasms in F344 rats given teriparatide [rhPTH(1-34)] are dependent on duration of treatment and dose. Toxicol Pathol. (2004) 32:426–38. doi: 10.1080/01926230490462138
125. Kouvidi K, Nikitovic D, Berdiaki A, Tzanakakis GN. Hyaluronan/RHAMM interactions in mesenchymal tumor pathogenesis: role of growth factors. Adv Cancer Res. (2014) 123:319–49. doi: 10.1016/B978-0-12-800092-2.00012-5
126. Nikitovic D, Kouvidi K, Kavasi RM, Berdiaki A, Tzanakakis GN. Hyaluronan/Hyaladherins - a Promising Axis for Targeted Drug Delivery in Cancer. Curr Drug Deliv. (2016) 13:500–11. doi: 10.2174/1567201813666151109103013
127. Midura RJ, Evanko SP, Hascall VC. Parathyroid hormone stimulates hyaluronan synthesis in an osteoblast-like cell line. J Biol Chem. (1994) 269:13200–6. doi: 10.1016/S0021-9258(17)36819-9
128. Midura RJ, Su X, Morcuende JA, Tammi M, Tammi R. Parathyroid hormone rapidly stimulates hyaluronan synthesis by periosteal osteoblasts in the tibial diaphysis of the growing rat. J Biol Chem. (2003) 278:51462–8. doi: 10.1074/jbc.M307567200
129. Sabbieti MG, Agas D, Xiao L, Marchetti L, Coffin JD, Doetschman T, et al. Endogenous FGF-2 is critically important in PTH anabolic effects on bone. J Cell Physiol. (2009) 219:143–51. doi: 10.1002/jcp.21661
130. Walkley CR, Walia MK, Ho PW, Martin TJ. PTHrP, its receptor, and protein kinase A activation in osteosarcoma. Mol Cell Oncol. (2014) 1:e965624. doi: 10.4161/23723548.2014.965624
131. Liao J, McCauley LK. Skeletal metastasis: established and emerging roles of parathyroid hormone related protein (PTHrP). Cancer Metastasis Rev. (2006) 25:559–71. doi: 10.1007/s10555-006-9033-z
132. Cheloha RW, Gellman SH, Vilardaga JP, Gardella TJ. PTH receptor-1 signalling-mechanistic insights and therapeutic prospects. Nat Rev Endocrinol. (2015) 11:712–24. doi: 10.1038/nrendo.2015.139
133. Bos MP, van der Meer JM, Feyen JH, Herrmann-Erlee MP. Expression of the parathyroid hormone receptor and correlation with other osteoblastic parameters in fetal rat osteoblasts. Calcif Tissue Int. (1996) 58:95–100. doi: 10.1007/BF02529730
134. Amizuka N, Kwan MY, Goltzman D, Ozawa H, White JH. Vitamin D3 differentially regulates parathyroid hormone/parathyroid hormone-related peptide receptor expression in bone and cartilage. J Clin Investigation. (1999) 103:373–81. doi: 10.1172/JCI3265
135. Langub MC, Monier-Faugere MC, Qi Q, Geng Z, Koszewski NJ, Malluche HH. Parathyroid hormone/parathyroid hormone-related peptide type 1 receptor in human bone. J Bone Mineral Res. (2001) 16:448–56. doi: 10.1359/jbmr.2001.16.3.448
136. Yasuda H, Shima N, Nakagawa N, Yamaguchi K, Kinosaki M, Mochizuki S, et al. Osteoclast differentiation factor is a ligand for osteoprotegerin/osteoclastogenesis-inhibitory factor and is identical to TRANCE/RANKL. Proc Natl Acad Sci USA. (1998) 95:3597–602. doi: 10.1073/pnas.95.7.3597
137. Quinn JM, Elliott J, Gillespie MT, Martin TJ. A combination of osteoclast differentiation factor and macrophage-colony stimulating factor is sufficient for both human and mouse osteoclast formation in vitro. Endocrinology. (1998) 139:4424–7. doi: 10.1210/endo.139.10.6331
138. Bacic D, Lehir M, Biber J, Kaissling B, Murer H, Wagner CA. The renal Na+/phosphate cotransporter NaPi-IIa is internalized via the receptor-mediated endocytic route in response to parathyroid hormone. Kidney Int. (2006) 69:495–503. doi: 10.1038/sj.ki.5000148
139. Cheng L, Sacktor B. Sodium gradient-dependent phosphate transport in renal brush border membrane vesicles. J Biol Chem. (1981) 256:1556–64. doi: 10.1016/S0021-9258(19)69840-6
140. Bringhurst FR. Calcium and phosphate distribution, turnover, and metabolic actions. In: DeGroot LJ, editor. Endocrinology. Philadelphia, PA: Saunders. (1989). p. 805–43.
141. Lupp A, Klenk C, Rocken C, Evert M, Mawrin C, Schulz S. Immunohistochemical identification of the PTHR1 parathyroid hormone receptor in normal and neoplastic human tissues. Eur J Endocrinol. (2010) 162:979–86. doi: 10.1530/EJE-09-0821
142. Birch MA, Carron JA, Scott M, Fraser WD, Gallagher JA. Parathyroid hormone (PTH)/PTH-related protein (PTHrP) receptor expression and mitogenic responses in human breast cancer cell lines. Br J Cancer. (1995) 72:90–5. doi: 10.1038/bjc.1995.282
143. Linforth R, Anderson N, Hoey R, Nolan T, Downey S, Brady G, et al. Coexpression of parathyroid hormone related protein and its receptor in early breast cancer predicts poor patient survival. Clinical Cancer Res. (2002) 8:3172–7.
144. Hoey RP, Sanderson C, Iddon J, Brady G, Bundred NJ, Anderson NG. The parathyroid hormone-related protein receptor is expressed in breast cancer bone metastases and promotes autocrine proliferation in breast carcinoma cells. Br J Cancer. (2003) 88:567–73. doi: 10.1038/sj.bjc.6600757
145. Li WH, Qiu Y, Zhang HQ, Liu Y, You JF, Tian XX, et al. P2Y2 receptor promotes cell invasion and metastasis in prostate cancer cells. Br J Cancer. (2013) 109:1666–75. doi: 10.1038/bjc.2013.484
146. Yang E, Cisowski J, Nguyen N, O'Callaghan K, Xu J, Agarwal A, et al. Dysregulated protease activated receptor 1 (PAR1) promotes metastatic phenotype in breast cancer through HMGA2. Oncogene. (2016) 35:1529–40. doi: 10.1038/onc.2015.217
147. Wang L, Zhou W, Zhong Y, Huo Y, Fan P, Zhan S, et al. Overexpression of G protein-coupled receptor GPR87 promotes pancreatic cancer aggressiveness and activates NF-kappaB signaling pathway. Mol Cancer. (2017) 16:61. doi: 10.1186/s12943-017-0627-6
148. Li S, Pei Y, Wang W, Liu F, Zheng K, Zhang X. Quercetin suppresses the proliferation and metastasis of metastatic osteosarcoma cells by inhibiting parathyroid hormone receptor 1. Biomed Pharmacotherapy. (2019) 114:108839. doi: 10.1016/j.biopha.2019.108839
149. Wen J, Qin Y, Li C, Dai X, Wu T, Yin W. Mangiferin suppresses human metastatic osteosarcoma cell growth by down-regulating the expression of metalloproteinases-1/2 and parathyroid hormone receptor 1. AMB Express. (2020) 10:13. doi: 10.1186/s13568-020-0949-4
150. Li S, Dong Y, Wang K, Wang Z, Zhang X. Transcriptomic analyses reveal the underlying pro-malignant functions of PTHR1 for osteosarcoma via activation of Wnt and angiogenesis pathways. J Orthopaedic Surg Res. (2017) 12:168. doi: 10.1186/s13018-017-0664-2
151. Scotlandi K, Serra M, Manara M, Nanni P, Nicoletti G, Landuzzi L, et al. Human osteosarcoma cells, tumorigenic in nude-mice, express beta(1)-integrins and low-levels of alkaline-phosphatase activity. Int J Oncol. (1993) 3:963–9. doi: 10.3892/ijo.3.5.963
152. Miura K, Uniyal S, Leabu M, Oravecz T, Chakrabarti S, Morris VL, et al. Chemokine receptor CXCR4-beta1 integrin axis mediates tumorigenesis of osteosarcoma HOS cells. Biochem Cell Biol. (2005) 83:36–48. doi: 10.1139/o04-106
153. Tome Y, Kimura H, Maehara H, Sugimoto N, Bouvet M, Tsuchiya H, et al. High lung-metastatic variant of human osteosarcoma cells, selected by passage of lung metastasis in nude mice, is associated with increased expression of alpha(v)beta(3) integrin. Anticancer Res. (2013) 33:3623–7.
154. Lam MH, House CM, Tiganis T, Mitchelhill KI, Sarcevic B, Cures A, et al. Phosphorylation at the cyclin-dependent kinases site (Thr85) of parathyroid hormone-related protein negatively regulates its nuclear localization. J Biol Chem. (1999) 274:18559–66. doi: 10.1074/jbc.274.26.18559
155. Pickard BW, Hodsman AB, Fraher LJ, Watson PH. Type 1 parathyroid hormone receptor (PTH1R) nuclear trafficking: association of PTH1R with importin alpha1 and beta. Endocrinology. (2006) 147:3326–32. doi: 10.1210/en.2005-1408
156. Dougherty KM, Blomme EA, Koh AJ, Henderson JE, Pienta KJ, Rosol TJ, et al. Parathyroid hormone-related protein as a growth regulator of prostate carcinoma. Cancer Res. (1999) 59:6015–22.
157. Watson PH, Fraher LJ, Hendy GN, Chung UI, Kisiel M, Natale BV, et al. Nuclear localization of the type 1 PTH/PTHrP receptor in rat tissues. J Bone Mineral Res. (2000) 15:1033–44. doi: 10.1359/jbmr.2000.15.6.1033
Keywords: canine, osteosarcoma, parathyroid hormone, parathyroid hormone related protein, prognostic factor
Citation: Al-Khan AA, Al Balushi NR, Richardson SJ and Danks JA (2021) Roles of Parathyroid Hormone-Related Protein (PTHrP) and Its Receptor (PTHR1) in Normal and Tumor Tissues: Focus on Their Roles in Osteosarcoma. Front. Vet. Sci. 8:637614. doi: 10.3389/fvets.2021.637614
Received: 03 December 2020; Accepted: 17 February 2021;
Published: 16 March 2021.
Edited by:
Mariarita Romanucci, University of Teramo, ItalyReviewed by:
Thomas Rosol, Ohio University, United StatesDragana Nikitovic, University of Crete, Greece
Copyright © 2021 Al-Khan, Al Balushi, Richardson and Danks. This is an open-access article distributed under the terms of the Creative Commons Attribution License (CC BY). The use, distribution or reproduction in other forums is permitted, provided the original author(s) and the copyright owner(s) are credited and that the original publication in this journal is cited, in accordance with accepted academic practice. No use, distribution or reproduction is permitted which does not comply with these terms.
*Correspondence: Janine A. Danks, amFuaW5lLmRhbmtzJiN4MDAwNDA7cm1pdC5lZHUuYXU=