- 1Division of Small Animal Emergency and Critical Care, Department of Clinical Veterinary Science, Vetsuisse Faculty, University of Bern, Bern, Switzerland
- 2School of Veterinary Science, Massey University, Palmerston North, New Zealand
Colloid solutions, both natural and synthetic, had been widely accepted as having superior volume expanding effects than crystalloids. Synthetic colloid solutions were previously considered at least as effective as natural colloids, as well as being cheaper and easily available. As a result, synthetic colloids (and HES in particular) were the preferred resuscitation fluid in many countries. In the past decade, several cascading events have called into question their efficacy and revealed their harmful effects. In 2013, the medicines authorities placed substantial restrictions on HES administration in people which has resulted in an overall decrease in their use. Whether natural colloids (such as albumin-containing solutions) should replace synthetic colloids remains inconclusive based on the current evidence. Albumin seems to be safer than synthetic colloids in people, but clear evidence of a positive effect on survival is still lacking. Furthermore, species-specific albumin is not widely available, while xenotransfusions with human serum albumin have known side effects. Veterinary data on the safety and efficacy of synthetic and natural colloids is limited to mostly retrospective evaluations or experimental studies with small numbers of patients (mainly dogs). Large, prospective, randomized, long-term outcome-oriented studies are lacking. This review focuses on advantages and disadvantages of synthetic and natural colloids in veterinary medicine. Adopting human guidelines is weighed against the particularities of our specific patient populations, including the risk–benefit ratio and lack of alternatives available in human medicine.
Background
A colloid is the collective term for electrolyte solutions containing macromolecules, a portion of which cannot pass freely out of the healthy intravascular space. Hence, they exert a colloid osmotic pressure (COP) and thereby retain or attract water commensurate with the number rather than the size of the colloid molecules on each side of the microvascular barrier (1). Natural colloids include blood products and albumin solutions, such as human serum albumin (HSA) and canine serum albumin (CSA). Synthetic colloids include hydroxyethyl starches (HES), gelatins, and dextrans (2) (Table 1). Synthetic colloids and particularly HES solutions have been preferred as volume expanders over isotonic crystalloids for decades (3). This was based on their presumed “volume-sparing effect.” Indeed, it was accepted that less colloid than isotonic crystalloid is necessary to reach adequate volume expansion in various populations of patients. Furthermore, since synthetic colloids do not readily cross the vascular barrier and produce COP, they were believed to contribute to prevention and reduction of edema formation (2, 4). However, the advantages of synthetic colloids have been questioned in recent years for two main reasons. First, regardless of their proposed short-term benefits, synthetic colloids are now known to be associated with serious long-term adverse effects. Second, the microanatomic and physiological basis of transvascular fluid flux has been revised in light of the more widespread appreciation of the role played by the endothelial surface layer (ESL) (see below). This newer understanding has raised doubts regarding the fundamental validity of the physiological basis for colloid therapy. Nevertheless, according to an international survey from 2016, synthetic colloids and particularly HES were extensively used in small animals (5). Since 2013 pharmacovigilance authorities worldwide have restricted the use of HES in people and colloid usage has therefore shifted to other products (namely, albumin and gelatins) (6). As a result, veterinarians are facing difficulties in procurement and overall reduction in availability of HES. Whether a similar shift toward alternative colloids is possible and safe for veterinary patients is unclear. This review will describe the characteristics and adverse effects of various synthetic and natural colloids, with the aim to provide risk-assessment and evidence-based recommendations for their use.
Physiology of Colloid Solutions
Classical vs. Revised Starling Principle
According to the traditional Starling principle, the opposing hydrostatic and colloid osmotic pressures of the intravascular space and the interstitium dictate transvascular fluid fluxes. Thus, administering a fluid that is preferentially retained in the intravascular space and with a COP higher than that of a crystalloid will expand intravascular volume to a greater extent per unit volume administered (1). However, the Starling principle has been revised in recent decades.
The newer “revised” or “extended” Starling principle considers the role of the endothelial glycocalyx (EG) within the ESL as an additional barrier to fluid movement across the vascular wall (7). The EG is a meshwork of membrane-bound complex sugars lining the luminal surface of the endothelium of all mammalian vessels (7). As part of the ESL, it acts as a selective barrier to movement of molecules across the vascular wall, due to its tight entanglement and predominantly negatively charged structure (8). The EG retains albumin and other proteins within the intravascular space. In that way, it is a major determinant of vascular permeability to fluid (9). The EG maintains a relatively low rate of filtration throughout the entire capillary length. At normal hydrostatic pressures, no reabsorption from the interstitium into the intravascular space occurs in non-fenestrated capillaries (10). Contrary to the “classical” Starling principle, the revised Starling principle proposes that the opposing pressures determining fluid extravasation or retention are the capillary hydrostatic pressure, the capillary COP (including that of the EG), and the hydrostatic and colloid osmotic pressure of the sub-glycocalyx area, which is virtually protein free (10). Therefore, in scenarios where the EG is intact, the major determinant of fluid filtration is the capillary hydrostatic pressure. However, in situations where the EG becomes damaged, such as systemic inflammatory states, it can no longer maintain its barrier function and extravasation of both plasma proteins and electrolytes, or administered colloids and crystalloids, is equally likely (11). Furthermore, extravasated colloid solutions increase the interstitial COP, thus retaining more fluid and aggravating interstitial edema (12). Therefore, theoretically, the change in intravascular volume that is achieved from a given dose of crystalloid or colloid depends on the integrity of the individual's EG. It is noteworthy that most of the statements supporting the revised Starling principle are derived from the experimental setting, and the clinical importance of changes in the EG requires determination. Indeed, the clinical significance of some aspects of the revised Starling principle has been called into question in a recent review (13). The interested reader is referred to the article “Advances in the Starling principle and microvascular fluid exchange; consequences and implications for fluid therapy” of this special issue for a more in-depth review on the EG.
Volume Effects of Colloids
Classically, colloids were believed to exert three to four times the volume effects of crystalloids (14, 15). This has been demonstrated in patients without systemic disease (16), in older studies of people in shock (17, 18), and in experimental hemorrhagic shock models (19–23). Several recent clinical studies, however, failed to corroborate these findings in systemically ill people, suggesting colloid-to-crystalloid relative volume expansion ratios of 1:1–1:1.5 (24). The proposed cause for this discrepancy is the damage to the EG resulting in fluid extravasation of both crystalloids and colloids, and therefore similar volume effects (2). In states of volume depletion, however, colloids seemingly exert a superior volume expanding effect to crystalloids. In people undergoing planned normovolemic hemodilution to a hematocrit of 21%, replacement of the removed blood with 115% volume of 6% HES 130/0.4 restored intravascular volume to 105 ± 4% (25). In a similar setting, replacement of four times the removed blood volume with Ringer's lactate solution led to a volume effect of only 17 ± 10%. In contrast, subsequent infusion of a 20% albumin solution to correct a volume deficit led to a volume effect of 184 ± 63% (16). Several experimental hyper- and normovolemic hemodilution models have shown similar findings, whether by evaluating volume expansion or using resuscitation endpoints (26–28).
Interestingly, these findings contrast with the revised Starling principle, whereby in the transient state of decreased hydrostatic pressure, both crystalloids and colloids should be able to equally restore blood volume (10). The cause for these discrepant findings is probably multifactorial. For instance, they might simply be the result of incomparable study designs or imprecise or different methodology. Indeed, the current techniques for estimating blood volume (such as dilution tracer techniques and the more common measurement of hemodilution) are challenging to perform and may carry inherent errors (12, 13, 29, 30). As a result, comparison between studies is difficult. This further hinders the ability of scientific evidence to translate into meaningful clinical guidelines.
In addition to “superior” volume expansion effects, colloids are proposed to stay “longer in the vessel” than crystalloids. While crystalloids are expected to redistribute over 20–40 min, studies have shown that the colloids persist in the intravascular space for 2–3 h in healthy euvolemic volunteers (31–33). Systemic inflammatory and otherwise “leaky” states are expected to shorten the duration of this effect (34, 35). However, extensive studies on redistribution of colloids are lacking (12). Further, colloids (and especially HES) have been specifically indicated in states of increased capillary permeability to “plug the holes in the endothelium.” The ability of the macromolecules contained in colloids to wedge into endothelial intercellular orifices, a product of systemic inflammation and endothelial dysfunction, has been proclaimed in textbook chapters and review articles (4, 14). This has been extrapolated mostly from experimental models using entire animals (36–42), isolated tissues (43), or endothelial cell lines (44). However, this ability remains to be tested in the clinical setting. Whether colloids reduce or exacerbate fluid extravasation in systemic inflammatory states remains controversial with studies reporting opposing results (34, 45–47). The reader is reminded that evaluating extravasation is challenging in the clinical setting, which might be a plausible explanation for these discrepancies. Another explanation for the “plugging potential” of colloids may lie in their interaction with the EG. Indeed, experimental studies have demonstrated that albumin might exert protective effects on the EG (48–52). In the same series of experiments, HES showed a similar, albeit weaker, protective effect (53). This is proposed to be the result of colloids binding to the glycosaminoglycan side chains of proteoglycans and glycoproteins and stabilizing the scaffolding structure of the EG (54, 55). However, in the majority of those studies, the study fluid was infused before an insult on the EG. Therefore, infusing colloids once the EG damage has occurred might not carry the same protective effect.
Colloid Osmotic Pressure Optimization
Synthetic colloids have been used as a substitute for albumin in patients with hypoalbuminemia with the rationale that they are capable of restoring plasma COP. Indeed, several veterinary studies in hypoalbuminemic patients have demonstrated that HES stabilizes (56) or increases plasma COP (57–59), with one study demonstrating a subjective decrease in peripheral edema when using high molecular weight HES (hetastarch) (60). However, the decrease in edema was not correlated with the dose administered and a drop of COP was noted after subsequent HES administrations. In another study where COP increased after administration of HES, it returned to baseline within 12 h of administration (58).
Administering synthetic colloids as a constant rate infusion (CRI) seems to be unique to veterinary medicine. Veterinarians often use synthetic colloids as CRIs for patients with hypoalbuminemia and edema (5). Non–peer-reviewed conference proceedings (61, 62) and review articles (2) recommend doses of 1–2 ml/kg/h or the maximal recommended daily dose divided by 24 h to support patients with low albumin or COP, respectively. In a recent study, HES 130/0.4 was able to maintain COP levels but did not significantly increase COP when administered as a CRI over 24 h to a small group of dogs with hypoalbuminemia (56). To the authors' knowledge, there are no other publications reporting the efficacy of administering colloids as a CRI rather than as a bolus. Ultimately, whether normalizing and maintaining COP should be a critical point in patient care is unclear as extravasation is at least as dependent on the integrity of the EG as it is on intravascular COP. Furthermore, synthetic colloids are not capable of carrying out all the other roles of natural colloids and specifically albumin. Therefore, they cannot be used interchangeably in the hypoalbuminemic patient.
Summary on Physiology of Colloid Solutions
In conclusion, natural and synthetic colloids may exert greater intravascular volume expanding effect per unit administered than crystalloids in healthy patients or patients with hypovolemia. However, caution is required when administered in patients with EG damage or other causes of increased vascular permeability. Not only does increased vascular permeability reduce the expansion of volume that colloids can lead to but extravasation of the macromolecules might exacerbate edema and subsequently impair tissue oxygenation. Previous indications for use of colloids include volume expansion (including perioperative hypotension) and COP optimization. These indications should be revised considering specific conditions (e.g., intact or damaged EG), alternative therapies (e.g., vasopressors rather than fluids for drug-induced hypotension), potential harmful effects (e.g., HSA leads to anaphylactic reactions in dogs), and overall availability (e.g., canine albumin found only in limited numbers of countries).
Hydroxyethyl Starch
Characteristics of HES Solutions
Hydroxyethyl starch is a potato or waxy maize–derived amylopectin, modified by the substitution of hydroxyl groups with hydroxyethyl residues on the glucose subunits to increase resistance to degradation in the blood. Recent reviews described pharmacokinetics, pharmacodynamics, effects, and adverse effects of HES (2, 4, 63, 64). Preparations are characterized by the mean molecular weight (molecular size, indicated in kilodalton, kDa), molar substitution (mole of hydroxyethyl residues per mole of glucose subunit), C2:C6 ratio (locations of hydroxyethyl residues on the carbon atom of the glucose subunits) and the concentration [e.g., 6% (60 g/L) vs. 10% (100 g/L)], the carrier solution (normal saline vs. polyionic, buffered, and balanced), and the starch source [potato (e.g., HES 130/0.42) vs. maize starch (e.g., HES 130/0.4)].
Since its introduction on the market in the 1970s, HES was one of the most commonly used resuscitation fluids in people worldwide (2, 3).
Mechanisms of Adverse Effects of HES
HES-associated adverse effects, such as nephrotoxicity, coagulopathy, tissue storage, and therapy-resistant pruritus, were reported early after its introduction on the market (2). After intravenous administration, large HES molecules (>60 kDa) are degraded by plasma α-amylase and by the reticuloendothelial system, before they undergo glomerular filtration and urinary excretion (2). However, HES is also rapidly (within hours) taken up by a wide variety of tissues in the body, with skin and kidney being the most affected organs (65). Intracellular HES degradation is slow and incomplete, and HES deposits persist up to 10 years in the human kidney (65). A key mechanism responsible for HES-induced acute kidney injury (AKI) is osmotic nephrosis after HES uptake in renal proximal tubular epithelial cells by pinocytosis. Osmotic nephrosis is characterized histologically by a vacuolization (i.e., storage in lysosomes) and swelling of the renal tubular epithelial cells, which compromises and occludes the tubular lumen, with subsequent stasis of urine flow (66). Another mechanism is hyperviscosity-mediated injury, in which hyperoncotic tubular fluid leads to stasis of flow and obstruction of the tubular lumen (Figure 1) which has been described with 10% HES solutions (ovine endotoxemic shock model) (67). Further, renal interstitial cell proliferation, macrophage infiltration, and tubular damage are documented pathological mechanisms of HES-induced adverse effects on renal function (68, 69). In an isolated renal perfusion model, 10% HES 200/0.5 caused more tubular damage compared with 6% HES 130/0.42 and Ringer's lactate (68).
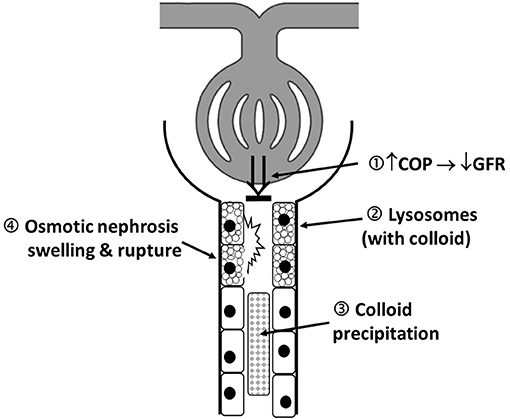
Figure 1. Schematic illustration of proposed mechanism of colloid-induced AKI: 1. Colloid induced increase in plasma COP that decreases filtration pressure and consequently GFR (“hyperoncotic AKI”). 2. Accumulation of colloid containing proximal tubular lysosomes, leading cellular dysfunction. 3. Local hyperviscosity and colloid precipitation, forming of occluding casts. 4. Osmotic nephrosis represents vacuolization and swelling of the renal proximal tubular cells; It can be reversible, and function restored but may also be a first step in the development of irreversible cell lesions. The above mechanisms are not mutually exclusive and may occur in combination. AKI, acute kidney injury; COP, colloid osmotic pressure; GFR, glomerular filtration rate.
Mechanisms for HES-induced coagulopathy include dilution of clotting factors, impaired platelet function, decreased concentrations of circulating von Willebrand factor and factor VIII, impaired factor XIII–fibrin cross-linking, and enhanced fibrinolysis, with the subsequent risk of bleeding complications (2, 4, 70, 71). The pathophysiologic consequence is an impairment of different hemostatic variables (i.e., activated partial thromboplastin time) (71), which may also complicate the interpretation of coagulation abnormalities in critically ill human and animal patients (e.g., HES induced vs. disseminated intravascular coagulation-induced prolongation of clotting times).
Adverse Effects of HES in Humans
Acute Kidney Injury and Mortality
The presumed beneficial effects of HES and the development of modern and putatively safer HES preparations seemed to outweigh the described adverse effects for a long time. This changed after the results of large multicenter randomized controlled trials (RCTs) in people, which compared HES and crystalloids for resuscitation of critically ill patients. Indeed, three large influential RCTs in nearly 600 patients with severe sepsis (“VISEP” in 2009), in 800 patients with severe sepsis with high illness severity (“6S” in 2012), and in 7,000 less sick intensive care patients (“CHEST” in 2012) found an increased risk of AKI and renal replacement therapy (RRT) (in all three trials), and mortality (only in “6S”) in patients receiving HES (72–74) (Supplementary Table 1). These findings challenged the risk:benefit ratio of HES. Proponents criticized these landmark trials for their “flawed” design, i.e., late use of HES in patients who were already volume resuscitated (in VISEP study patients received HES for up to 21 days) and including patients who received HES even though they were in renal failure (75). Around the same time, two smaller RCTs in 115 patients with penetrating and blunt trauma (“FIRST” in 2011) and in 156 patients with severe sepsis (“CRYSTMAS” in 2012) reported no adverse effects (76, 77). In addition, a large open-label randomized comparison between crystalloids and various colloids for the treatment of acute hypovolemia in nearly 2,900 patients with hypovolemic shock (“CRISTAL” in 2013) showed no significant difference in 28-days mortality, but a significant reduction in mortality at 90 days [34.2% (crystalloids) vs. 30.7% (colloids), p = 0.03] in the colloid group (70% of colloids were HES), and more vasopressor-free and ventilator-free days by day 28. The study also found no evidence that colloids increased the risk of AKI or any other serious adverse event (Supplementary Table 1) (78). Independently of these controversial RCT results, almost 90 HES-related scientific articles authored by a prominent German anesthetist and prolific defender of HES were retracted 2012 for data fabrication and lack of ethics approval (79, 80). The retraction of such a large body of work has had far-reaching effects on clinical practice and research oversight (81). Because of the RCTs demonstrating harm in critically ill patients receiving HES and the retraction of the HES-supporting scientific articles, several systematic reviews then highlighted the adverse effects of HES (82–85). As a consequence, two pharmacovigilance safety reviews of HES worldwide in 2012/2013 and in the European Union (EU) in 2017/2018 led to progressive restrictions of HES use in people (86). Since then, experts worldwide have considered HES solutions to be contraindicated in patients with sepsis or other critical illnesses, additional warnings on packaging are required in the EU, and supply has been limited to accredited hospitals after training of healthcare professionals (86). These restrictions are still a matter of debate between HES proponents and opponents. Nevertheless, between 2007 and 2014, the proportion of patients receiving colloids in human medicine decreased significantly, which was primarily due to a decrease in the use of HES, despite an overall increase in the use of human albumin (6). As most of the negative effects of HES reported in the RCTs involved patients with sepsis, in 2013 European medicines authorities requested further studies evaluating the safety and efficacy of HES in perioperative non-septic patients (86). Several smaller studies and RCTs in non-septic patients in the perioperative setting found no evidence of a nephrotoxic effect of HES (87, 88). Likewise, two recent larger, multicenter trials in 4,545 (“RaFTinG” in 2018) and 775 (“FLASH” in 2020) surgical patients also found no significant difference in mortality between patients who received HES or crystalloids and AKI in one (89), and according to the authors a reduced risk of RRT and AKI in another (90) (Supplementary Table 1). Notably, “RaFTinG” was criticized for statistical over-adjustment and “FLASH” for misrepresenting results (91, 92). In summary (and regardless of the exhaustive criticism all RCTs have been subjected to), critically ill and specifically septic patients seem to be particularly burdened by the nephrotoxicity of HES. This is exacerbated by long-term HES administration to otherwise hemodynamically stable patients. Such effects seem to be lost in less severely ill patients and specifically those presented with acute hypovolemia or those requiring perioperative fluid therapy.
HES Coagulopathy
In addition to the results regarding kidney failure in the aforementioned studies, HES-induced coagulopathy and hemorrhage is also a well-known adverse effect (73, 74). Despite improved physicochemical properties of newer HES products (e.g., tetrastarch), a systematic review from 2011 found HES 130/0.4 led to hypocoagulability in a dose-dependent fashion (70). This was supported in further clinical studies, where patients who received HES lost more blood and had higher need for packed red blood cell (pRBC) transfusions than patients receiving only crystalloids (74, 88). In 6S, more patients in the HES group than in the Ringer's acetate group received blood products, including packed red cells (relative risk, 1.28; 95% CI 1.12–1.47; p < 0.001) (74). In two recently published RCTs, HES therapy was associated with a blood loss more than twice as high as with crystalloid alone (87, 93). In contrast, a meta-analysis including 49 studies in a total of nearly 3,439 patients undergoing cardiac surgery (i.e., colloids are used as priming solution and volume replacement) could not identify evidence for a higher risk of bleeding, blood transfusion, or reoperation associated with tetrastarch compared with pentastarch, albumin, gelatin, and crystalloids. Tetrastarch was superior to human albumin in terms of blood loss and transfusion requirements (blood loss after tetrastarch vs. albumin: standardized mean difference, −0.34; 95% CI, −0.63 to −0.05; p = 0.02) (94).
Safety Recommendation in People
According to the latest recommendation from the European Medicines Agency from 2018, valid for the EU countries, HES use should be limited to initial volume resuscitation with a dose not exceeding 30 ml/kg over a period of administration not exceeding 24 h, and that kidney function should be monitored for at least 90 days thereafter. In addition, HES is contraindicated in sepsis, critically illness, burns, severe preexisting coagulopathy, and bleeding in people, among others (86).
Adverse Effects of HES in Small Animals
HES is currently the most used and studied synthetic colloid in veterinary medicine. Guidelines or absolute contraindications on the use of HES do not yet exist for small animals. Longstanding recommendations for the use of HES in animals have been mostly extrapolated from human medicine so the recent changes in human guidelines have led to more veterinary research.
HES-Induced Acute Kidney Injury in Small Animals
Most of the studies of the renal effects of HES in dogs and cats have been retrospective or experimental and have yielded conflicting results (95–102) (Table 2). In one retrospective study, treatment with 10% HES 200/0.5 was a risk factor for adverse outcome, including in-hospital death or AKI, and the risk of AKI increased with increasing HES dose (97). In four retrospective studies in critically ill dogs and cats, 6% HES 130/0.4 was not associated with an increased risk for AKI (98–101), although the number of HES days was significantly associated with an increase in AKI grade within 10 days post-HES (98). Two of these studies specifically evaluated a subgroup of cats and dogs with sepsis and found no HES-associated acute kidney injury (100, 101). Interpretation of these studies is hampered by the fact that different HES types are used, the definition of AKI is not standardized, study populations are comparatively small, and the studies are retrospective in nature, indicating associations rather than causality. Further, creatinine has a poor sensitivity to detect early stages of AKI. A conclusion regarding the renal safety of tetrastarch based on these four studies is therefore limited.
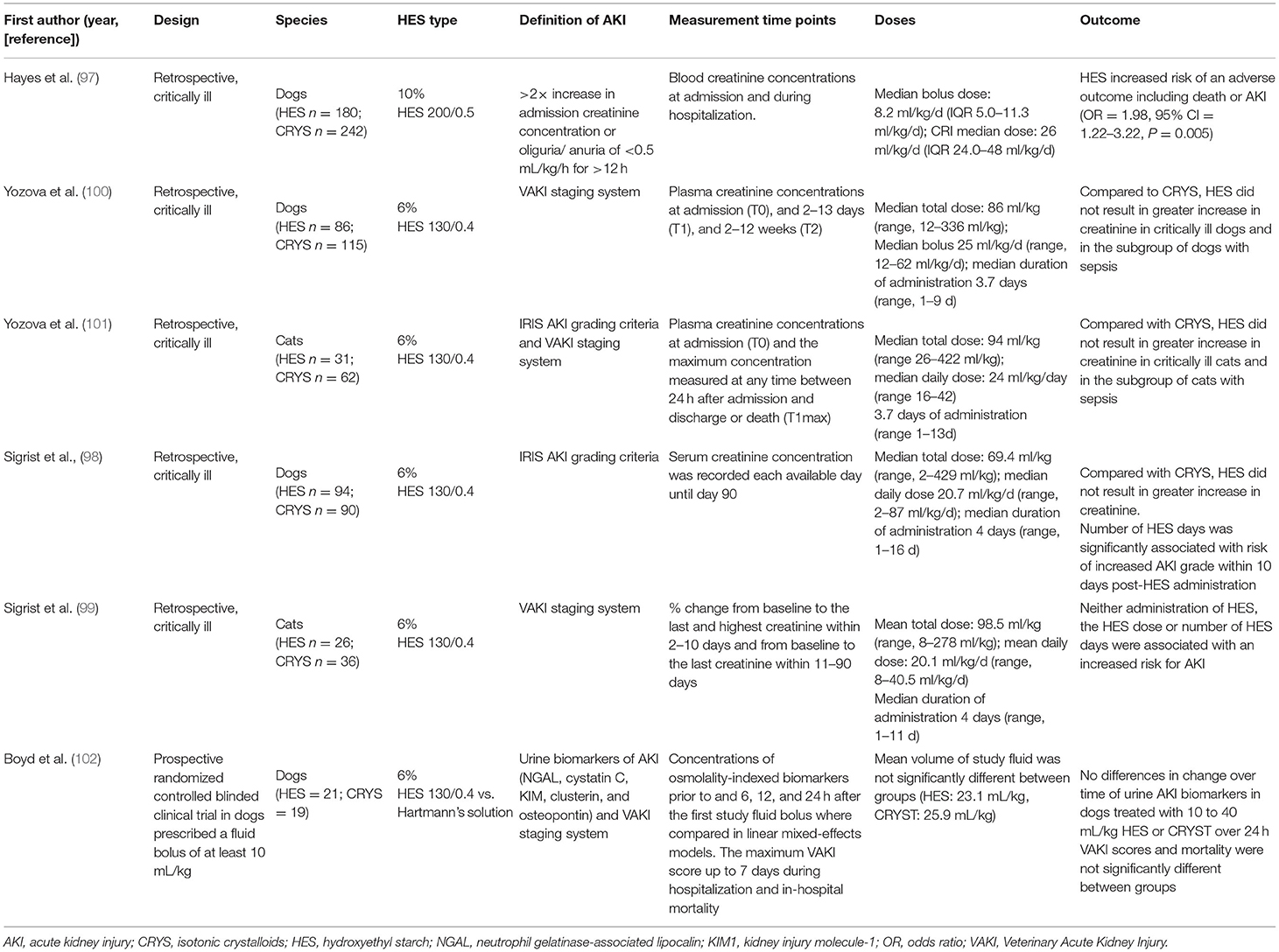
Table 2. Clinical studies evaluating kidney injury after HES administration in dogs and cats (sorted by publication year).
Two experimental studies compared the effect of 6% HES 130/0.4 (bolus of ~20 ml/kg once), of other colloids, and of crystalloids on kidney injury in canine hemorrhagic shock models (95, 96). Concentrations of several plasmatic and urinary renal biomarkers were analyzed, e.g., neutrophil gelatinase-associated lipocalin, a protein expressed after renal proximal tubular damage and secreted in blood and urine. Both studies found no association between HES and biomarkers of AKI within 3 or 72 h after HES bolus, respectively (95, 96). It is unclear to what extent these results can be extrapolated to critically ill or septic dogs and cats, as acute hemorrhagic shock was induced in previous healthy dogs, and the studies were conducted over a relatively short period of time in a small group of dogs and in a defined disease model.
Prospective RCTs are needed and are currently underway. Recently, results from a randomized, blinded, clinical trial to compare different urine biomarkers (e.g., neutrophil gelatinase-associated lipocalin) of AKI in dogs receiving 6% HES 130/0.4 vs. Hartmann's solution for shock resuscitation were published (102). No differences in urinary AKI biomarkers between the HES group (21 dogs) and the crystalloid group (19 dogs) were found up to 24 h after the first fluid bolus. In addition, in the five dogs which developed AKI (i.e., three in the HES group, two in the crystalloid group), no significant difference in maximum Veterinary Acute Kidney Injury scores was found (102).
Intrarenal HES accumulation has also been evaluated histologically in dogs (95, 103). Tubular injury scores (based on damaged epithelial cells and presence of sloughed cells within tubular lumen) and the degree of renal tubular microvesiculation (vesicles within renal epithelial cells) were not significantly different between dogs receiving 6% HES 130/0.4 compared with dogs receiving whole blood or isotonic crystalloids in an experimental hemorrhagic shock model (95). In contrast, retrospective histopathological examination of kidney tissue from critically ill dogs revealed that the cumulative dose of 6% HES 670/0.75 was positively associated with the severity of renal tubular vacuolization (103). It is worth mentioning that osmotic nephrosis is not specific to HES administration but can be due to other drugs (e.g., mannitol, glucose, intravenous immunoglobulins), primary renal diseases, and postmortem autolysis (66). Immunohistochemical evaluation with specific HES antibodies would be ideal to provide a reliable diagnosis of HES-induced osmotic nephrosis.
HES-Induced Coagulopathy in Small Animals
Several in vitro and in vivo studies in healthy dogs and cats (104–108) have shown a dose-dependent and transient coagulation impairment after in vitro dilution of blood or IV administration of HES. Hemostasis assessment was performed predominately by whole blood platelet function analyses and viscoelastometric coagulation analyses (e.g., rotational thromboelastometry). Platelet function, speed of clot formation, and clot firmness were more impaired after HES than after crystalloids using similar dilutions (104–108). However, when clinical doses of HES and isotonic crystalloid (i.e., 10 ml/kg HES vs. the 3- to 4-fold volume of crystalloid) were compared in vitro (blood from healthy dogs) (107) or in vivo (experimentally induced canine hemorrhagic shock model), no difference was found (109, 110). Different results were obtained in two other studies which used thromboelastometry. In one hemorrhagic shock model, the administration of 20 ml/kg of 6% HES 130/0.4 was associated with hypocoagulability beyond effects of hemodilution (i.e., 80 ml/kg crystalloids) (111). Likewise, in critically ill dogs with spontaneous hemoperitoneum, more impaired coagulation was found after 10 ml/kg of 6% HES 130/0.4 compared with a 3-fold volume of crystalloid (112). However, in this study, parameters for clot firmness remained within reference ranges and no statistically significant differences in standard coagulation tests were found between the HES and crystalloid group (112). The clinical relevance of the aforementioned changes has not yet been conclusively determined because actual bleeding tendencies have been evaluated in only one study in dogs. In this study, no clinical bleeding was detected after 10 ml/kg of 6% HES 600/0.75 administered to healthy anesthetized dogs. HES coagulopathy appears to last <3 h [15 ml/kg of 6% tetrastarch (106)] and <24 h [20 ml/kg of 6% hetastarch (113)], respectively, after a single bolus in healthy dogs.
Additional findings in several in vivo studies were a significant decrease in hematocrit and platelet count after a HES bolus (106, 112) which was disproportionate to the administered volume of HES (i.e., greater decrease in hematocrit after HES than after crystalloid). The reason for this disproportionate decrease in hematocrit has been proposed to be mobilization of plasma volume previously retained within the endothelial glycocalyx (25).
Recommendations for HES Use in Small Animals
In general, HES should be avoided if the animal responds already to crystalloid or other therapy. HES is not a replacement for albumin and in patients with severe hypoalbuminemia, natural colloids (such as plasma products or albumin) should be considered. In complex cases (such as sepsis or septic shock), therapy is multimodal, and potentially natural colloids and/or vasopressors are necessary. The current evidence favors a very limited risk for HES-associated AKI in dogs and cats when administered in small doses (<20 ml/kg) for short periods of time. Previously healthy animals with acute hypovolemia (e.g., trauma-induced hemorrhage) could inconsequently tolerate administration of HES in the initial resuscitation phase, and HES can be considered if substantial amounts of crystalloids are required. The necessity for colloids is questionable as isotonic or hypertonic crystalloids usually provide adequate resuscitation endpoints in such patients. Administering HES to hemodynamically stable patients and patients with pre-existing azotemia is not recommended.
Based on extrapolated data from people, resuscitating critically ill and specifically septic patients with HES might be detrimental especially in high doses and for long periods of time. Furthermore, as mentioned previously, it might also be futile because damage to their EG exacerbates extravasation of any fluid. Currently, there is no evidence that HES has any disadvantages in veterinary patients with sepsis; however, there are no proven benefits to date either.
The effects of HES on different coagulation tests seem to be mild in dogs without preexisting coagulopathy, and mild to moderate in those with preexisting coagulopathy when using doses <20 ml/kg. Given the evidence in human medicine (definite increased risk of bleeding) and the unclear association between standard laboratory tests and actual clinical bleeding risk in dogs, monitoring of coagulation parameters and clinical bleeding as well as hematocrit and platelet count should be considered if the HES dose is ≥10 ml/kg in patients with potential preexisting coagulopathy, anemia, or thrombocytopenia (112).
In contrast to human medicine and specifically high-income countries, veterinarians need to incorporate financial considerations in their decision-making. Furthermore, prompt expensive therapy might be hindered by owner indecisiveness (whether on financial or personal grounds) or availability. In such instances, HES could be considered as a short-term solution in patients where more costly therapies (i.e., allogenic albumin and plasma products) are unaffordable or while decisions are made. Hydroxyethyl starch should be used as a bolus at the lowest possible dose. Under consideration of the existing literature, the authors recommend a dose for 6% tetrastarch of 5–10 ml/kg for dogs and 3–5 ml/kg in cats over 10–15 min in states of hypovolemia. This can be repeated if needed up to a total dose of 20 ml/kg. The authors do recommend against the use of HES as a CRI, as the effects on COP is negligible (56) and a significant association was found between days of administration of HES and AKI grade (98). Kidney function should be monitored after HES exposure—according to human studies up to 90 days after exposure. Veterinary clinicians should consider discussing the potential adverse effects of HES with owners of septic patients.
Gelatin
Characteristics of Gelatin Solutions
Gelatin solutions contain bovine gelatin hydrolysate, chemically modified to increase solubility. Gelatin preparations have a mean molecular weight of ~30 kDa. As this is below the renal threshold for glomerular filtration, most of the gelatin is excreted into the urine within minutes of infusion. Thus, the volume effect (70–80%) and the duration of volume expansion (2–3 h) are expected to be more limited compared with HES (114). Gelatin is available as a 4% succinylated gelatin solution in normal saline or in a buffered, polyionic isotonic carrier solution (Table 1). Gelatin was withdrawn from the US market in 1978 due to concerns of increased blood viscosity and coagulation impairment (115). In addition, animal experiments suggest harmful effects on kidney structure and function similar to the effects seen with HES (116, 117) (Figure 1). In countries where gelatin is still licensed (e.g., Germany, Switzerland), it is contraindicated in severe renal failure. However, there is no clear dose or maximum daily limit, and the recommendation on the package insert states that the “maximum daily dose is determined by the degree of hemodilution.” Due to the restrictions in HES use, gelatin could potentially become an alternative synthetic colloid in many European countries.
Adverse Effects of Gelatin in People
Significantly fewer clinical studies on gelatin exist in human medicine as compared with HES. Despite the lack of RCTs evaluating the risks and benefits of intravenous gelatin in people, clinicians in human medicine seem to have increased their use of gelatin following the HES restrictions from 2013 and 2018 (118, 119). There is nonetheless evidence that gelatin might be at least as detrimental to kidney function as HES with a more limited understanding of the underlying mechanism. In a prospective study in people with severe sepsis, AKI occurred in 70% of patients receiving HES and in 68% of patients receiving gelatin, vs. 47% of patients receiving crystalloids (120). In a systematic review and meta-analysis of gelatin-containing plasma expanders vs. crystalloids and albumin in people, the authors concluded that gelatin solutions increase the risk of anaphylaxis, and may increase bleeding, renal failure, and mortality. The authors recommended against the use of gelatins (119). A prospective currently ongoing study, Gelatin in ICU and Sepsis (GENIUS), is a double-blind RCT investigating the efficacy and safety of gelatin as opposed to crystalloid administration in two parallel groups of patients with severe sepsis/septic shock (NCT02715466). Results from that study are expected end of 2021.
Adverse Effects of Gelatin in Small Animals
Gelatin seems to be rarely used in small animals. According to an international survey about the use of colloids in small animals from 2016, gelatin was used by only 4% of the respondents (in contrast to 85% who used HES), although it was the main synthetic colloid used by 24% of respondents from the UK (5). This finding might be the result of reduced availability of HES products in specific countries (e.g., the UK recalled all HES products from the market in 2013 after the EMA review was initiated). Therefore, veterinarians were forced to use alternative licensed options such as gelatins. Only a few reports of the effects on coagulation and renal injury in dogs, and no reports in cats have been published (95, 111, 121–123).
In regard to its effects on coagulation, experimental studies have shown that 20 ml/kg of 4% gelatin leads to impaired platelet function (111), but that 10 to 20 ml/kg of 4% gelatin had negligible effects on thromboelastography and plasma coagulation (111, 123).
Regarding its renal effects, gelatin led to a marked increase in urine and plasma renal biomarkers, e.g., a 33-fold increase in urinary neutrophil gelatinase-associated lipocalin and a 5.9-fold increase in the urinary concentration of clusterin (a renal glycoprotein induced in response to a variety of injuries including ischemia/reperfusion) (95). Notably, this increase was significantly more than after the same dose of 6% tetrastarch. The same study found prominent renal epithelial cell microvesiculation in all dogs receiving 4% gelatin, although these were not sufficiently severe to meet the definition of “osmotic nephrosis” (95). As mentioned earlier, these data cannot be directly extrapolated to critically ill dogs and cats. However, preexisting renal damage in critically ill and septic patients favors the pathogenesis of osmotic nephrosis-related AKI, and certainly predisposes patients even more to gelatin-induced AKI.
Recommendations for Gelatin Use in Small Animals
According to the results of a small number of studies, the administration of gelatin significantly impairs platelet function and is associated with a high risk of renal injury in dogs. Because the volume-expanding effects of gelatin are inferior to HES, yet the risk of renal injury is significant, it is not appropriate to use gelatin as an alternative to HES.
Dextrans
Characteristics of Dextran Solutions
Dextrans are neutral, high-molecular-weight glucose polysaccharides synthesized from sucrose by the bacteria Leuconostoc mesenteroides or dextranicum (114). Commercially available dextran solutions have an average molecular weight of either 70 kDa (dextran-70) or 40 kDa (dextran-40). Concentrations used are 3, 6, and 10%, and they are mixed in either isotonic or hypertonic electrolyte solutions (Table 1) (1, 124). Dextran is either excreted by the kidneys or metabolized by hepatic dextranase and the glucose monomers are ultimately oxidized to carbon dioxide and water (1). Dextran-40, consisting of smaller molecules, has more particles per unit weight than dextran-70, and hence it is more osmotically active. However, its smaller molecular fractions pass faster through semipermeable membranes of the capillaries into the interstitial space and through glomeruli into the urine. Thus, the volume expansion effect of 10% dextran-40 is 150% with a duration of action of 3–5 h, while the volume effect of 6% dextran-70 is 100% with a duration of action of 6–8 h (up to 20 h), due to its larger molecular fractions and prolonged excretion (125). Hypertonic 10% dextran-40 leads to an initial volume expansion of up to 200% (114).
Major adverse effects in people include anaphylactoid reactions, AKI, and impaired coagulation. Several theories about the mechanism for dextran-induced AKI have been proposed, with some of these mechanisms shared with other colloids. These include an increase in plasma COP with subsequent decrease in glomerular filtration pressure and reduced GFR; precipitation of dextran within the renal tubules forming occluding casts and osmotic nephrosis (66, 126, 127) (Figure 1). Dextran is also known to impair coagulation, with a subsequent increased risk of bleeding (128). The underlying mechanisms are not well-understood, but bare similarities with other synthetic colloids. These include inhibition of platelet aggregation by reducing the activity of factor VIII, von Willebrand factor, and the glycoprotein IIb/IIIa receptor, affecting fibrinogen polymerization by co-binding, and enhancing fibrinolysis by increasing the circulating levels of tissue type plasminogen activator, and decreasing the levels of plasminogen activator inhibitor-1 (128–130).
According to a 2010 international study (3), the use of dextran in people was widespread in Scandinavia. However, it remained the least used colloid, accounting for only 3% of the total international responders. Dextran has been withdrawn from the human market in several countries (i.e., Germany, Switzerland) but seems to replace HES in others (e.g., Sweden) (131).
Adverse Effects of Dextrans in People
Anaphylactoid reactions after dextran are the result of preformed endogenous anti-polysaccharide antibodies, which cross-react with dextran molecules, causing an immune reaction (114, 132). These severe reactions are prevented by “hapten inhibition” using dextran 1, which binds dextran-reactive antibodies and forms inactive complexes. The dextran 1, which has been used for hapten inhibition since the 1980s, can be administered a few minutes before the infusion of the main solution of dextran. A 35-fold reduction in anaphylactoid reactions after introduction of the prophylactic use of hapten inhibition was reported in a study from Sweden from a decade after its introduction (133).
Numerous cases of AKI after administration of 10% dextran-40 have been published (69, 134–137). While these older studies have shown an increased risk of AKI with the use of dextran-40, studies of dextran-70 are few, probably because of its limited use worldwide. However, existing data suggest that dextran-70 is also a potential risk factor for the development of AKI in patients with septic shock (138, 139).
Impairment of in vitro coagulation and increased bleeding after dextran-70 has also been found in different patient groups (131, 138, 139). Due to this particular side effect, dextran was used as a prophylactic agent against postoperative thrombo-embolism in the 1980 (140).
Adverse Effects of Dextrans in Small Animals
In the 1990s, hypertonic saline with 6% dextran-70 (HSD) preparations were studied in different canine shock scenarios, such as septic, endotoxic, hemorrhagic, and traumatic shock, and gastric dilatation volvulus. As part of developing “small volume resuscitation” strategies, the volume effects of dextran were compared with isotonic crystalloids (141–146). In all mentioned studies, bolus therapy with 4–5 ml/kg of HSD solution was found to be a more effective resuscitation solution compared with an isotonic crystalloid, and 8–10 times less volume was necessary to reach similar cardiovascular endpoints. To the authors' knowledge, there are no reports about dextran-induced anaphylactoid reactions or AKI in dogs. Two studies evaluated hemostasis after dextran-70 in dogs and found a dose-dependent impairment in different hemostatic variables (such as plasma coagulation assays, platelet numbers, factor VIII coagulant activity, von Willebrand factor antigen concentration, and platelet function and buccal mucosal bleeding time) (122, 147). It is likely that as the preference of synthetic colloids shifted toward HES solutions, clinical studies of dextrans in dogs or cats were no longer performed. This may change in the near future. In a recent experimental study in dogs, resuscitation after hemorrhagic shock with HSD showed a similar hemodynamic response compared with Ringer's lactate at 10 times the volume of HSD, but HSD showed superior efficacy in organ protection (kidneys, lungs, and liver) (148).
Recommendations for Dextran Use in Small Animals
Dextran-70 seems to be efficient for volume resuscitation in dogs, especially when combined with hypertonic saline. Other than coagulation impairing effects, adverse effects are largely unknown, and as for other colloids, nephrotoxic effects are likely. There are insufficient data in dogs, and no data in cats, to make a recommendation for dextran use.
Albumin
Characteristics of Albumin Solutions
Solutions of human serum albumin are prepared from pooled human serum, with sterile water as the carrier. The solutions available for intravenous use contain no preservative, and pathogen inactivation is performed by pasteurization at >60°C for 10 h (149, 150). It is usually available as 5, 20, and 25% solutions, with availability varying geographically. Albumin accounts for ~50% of the plasma protein content and is responsible for about 80% of the plasma COP (151, 152). A near exponential relationship exists between albumin concentrations and in vitro COP, which is explained by the Gibbs–Donnan effect (153). The negative charges on amino acids within albumin attract cations (which have and osmotic effect), leading to a disproportional increase in COP (153). Thus, a 5, 12.5, and 25% HSA solution exerts a COP of ~20, ~95, and >200 mmHg, respectively (153).
Albumin is incorporated into the EG, where it contributes to vascular integrity, and normal capillary permeability. In addition, albumin has antithrombotic, antioxidant, and anti-inflammatory properties (55, 154). Hypoalbuminemia occurs as a result of many critical diseases such as systemic inflammation, sepsis, burns, liver failure, protein-losing enteropathy/ nephropathy, and severe prolonged malnutrition. Severe hypoalbuminemia can lead to gastrointestinal complications (i.e., gastric and intestinal edema, gastrointestinal ileus), effects on coagulation (i.e., hypercoagulability, increased platelet aggregation), delayed wound healing, and consequences secondary to the decreased COP (i.e., tissue edema, body cavity effusions) (152). Furthermore, hypoalbuminemia has been associated with high morbidity and mortality rates in human and veterinary patients (100, 155, 156). A meta-analysis in critically ill hypoalbuminemic human patients found that each 10 g/L decrease in serum albumin concentration increased the odds of mortality by 1.37 (156). Nevertheless, it remains unclear whether these effects are a direct result of the albumin deficit, in which case albumin replacement would be beneficial, or if it is merely a marker for the disease severity, and albumin optimization would not lead to improved patient outcomes.
Use of Albumin in People
Albumin is commonly used for fluid resuscitation in people (157–159). Usually, 5% HSA (and more rarely 20% HSA) is administered as a second-line treatment after crystalloids (24, 159, 160). Another indication is correction of significant hypoalbuminemia. Albumin is not only used in conditions requiring COP support. In severe liver diseases such as cirrhosis albumin is recommended for its non-oncotic properties such as antioxidant, scavenging, immune-modulating, and endothelial protective functions (161). Albumin solutions are used in various settings and conditions including priming cardiopulmonary bypass circuits, therapeutic plasma exchange, nephrotic syndrome, and pancreatitis (159). They are not indicated as a nutritional source to raise serum albumin per se without hypovolemia (159). Anaphylactoid reactions have been reported in <0.1% of recipients (162).
The use of albumin in critical care has generated controversy for decades. In 1998, a Cochrane systematic review of 30 small RCTs suggested harmful effects (i.e., increased mortality) of albumin as compared with crystalloids for volume replacement in critically ill patients (163). The impact of this meta-analysis was dramatic and led to a substantial reduction in the use of albumin in some countries. In 2004, the “SAFE” study was published, a RCT which compared mortality in 6,997 critically ill patients with hypovolemia who had received 4% albumin or saline for intravascular-fluid resuscitation (164). A small volume sparing effect was observed during the first 4 days in the albumin group (ratio of albumin to saline was ~1:1.4), but no significant difference was found in 28-days mortality, length of hospitalization, or organ dysfunction. Consequently, albumin was considered to be “safe,” but that it did not offer any advantage over saline. Subgroup analyses revealed that patients with severe sepsis treated with albumin tended to show a better survival, although the difference did not reach statistical significance (p = 0.09) (164). In 2014, the “ALBIOS” trial was conducted in 1,818 patients (158). This RCT aimed to answer the question of whether in patients with severe sepsis or septic shock, administration of 20% HSA to maintain a serum albumin level ≥3 g/dl (≥30 g/L) reduces all-cause mortality at 28 days compared with no albumin. Similarly to the SAFE study (164), there was no significant difference in either the 28- or 90-days mortality or total organ failure scores. Patients who received albumin had a lower daily positive fluid balance over the first 7 days (158).
Despite the theoretical benefits of albumin and efforts to translate this into positive patient outcomes (158, 164), no RCT has yet demonstrated a significant advantage of albumin over other types of fluid, including crystalloids (165). Nevertheless, an increase in albumin use in adult intensive care patients was found recently (6). Albumin appears to have replaced HES as a resuscitation solution (6, 166). This is potentially associated with the Surviving Sepsis Campaign from 2016 which recommend volume replacement with albumin (instead of a synthetic colloid) when patients require “substantial” amounts of crystalloid during hemodynamic stabilization (167).
Adverse Effects of Albumin in Small Animals
In small animals, intravenous albumin is mostly used to correct hypoalbuminemia and COP. Sources of albumin in dogs and cats include CSA, allogenic plasma products, and HSA. No feline-specific albumin products are available (Table 1). Canine albumin (e.g., lyophilized canine albumin) manufactured in the USA is currently not ubiquitously available. Consequently, some veterinarians are forced to use HSA products if they wish to administer concentrated albumin to dogs and cats. Human albumin shares ~80% structural homology with canine albumin (168); therefore, allergic reactions are possible. Anaphylactic and other immune reactions, and life-threatening complications have been reported in dogs after administration of 5, 10, and 25% HSA (169–173). Relevant study results are summarized in Table 3 and are explained in more detail later.
In 2007, two research groups observed profound adverse reactions after 25% HSA was administered to healthy, non-hypoalbuminemic dogs (169, 170). Cohn et al. (169) administered 25% HSA twice, 5 weeks apart, to nine healthy dogs. Anaphylactic shock (collapse, hypotension, hypothermia, tachypnea, vomiting, and diarrhea) occurred within minutes in one out of nine dogs after the first dose of HSA, and in two out of two dogs receiving a second dose, while the second HSA infusion for the remaining seven dogs was abandoned. Furthermore, two out of nine dogs developed urticaria and edema 1 week after administration, and all developed anti-HSA antibodies. Similarly, Francis et al. (170) administered 25% HSA to six healthy dogs. One out of the six developed anaphylactic shock, and all dogs developed a delayed type III hypersensitivity including lameness, edema, vasculitis, and vomiting. Two of the dogs died at 21 and 28 days after HSA administration, despite intensive care treatment. These two dogs developed disseminated intravascular coagulation, AKI, and pulmonary edema. All six dogs were found to have anti-HSA antibodies (170).
Eight publications have reported the effects (including adverse effects) of administration of HSA in critically ill hypoalbuminemic dogs and cats in a clinical setting (171–175, 177–179). Human serum albumin resulted in increased serum albumin concentrations and COP (173, 174, 176, 178) and increased systemic blood pressure (174). Two studies reported higher serum albumin concentrations in survivors after HSA administration (173, 176) and in one study the magnitude of serum albumin increase was positively associated with survival (173). All but one of the aforementioned studies (171–175, 177, 179) reported adverse reactions in some patients, of which a few were severe or fatal. One prospective study in 40 cats reported no adverse effects after administration of 5% HSA (178). Most studies were retrospective in nature and adverse effects of HSA may have remained undetected or unrecorded. Furthermore, the definition and recognition of adverse reactions is not uniform between studies.
The available literature suggests that administration of HSA induces severe adverse reactions predominantly in healthy, normoalbuminemic dogs and may induce only mild or no adverse reactions in critically ill patients. Several possible explanations for this discordant response have been proposed. Supraphysiologic concentrations of albumin might be partly responsible for acute adverse reactions in healthy normoalbuminemic dogs. Therefore, administration of HSA over a longer period (e.g., 12–24 h) or administration of diluted albumin solutions may result in fewer immunologically mediated adverse effects (170, 175, 178). Further, it is possible that deterioration of the clinical condition or death in critically ill patients have been incorrectly attributed to the underlying disease, rather than to an adverse reaction (170). It is also possible that some hypoalbuminemic dogs given HSA may not have survived long enough to develop a type III hypersensitivity reaction (170). Lastly, it is speculated that critically ill dogs may be immunosuppressed (due to the severe underlying disease) and therefore not respond as strongly to HSA as healthy immunocompetent dogs (169, 170). This was challenged by a recent case series of two critically ill hypoalbuminemic dogs with septic peritonitis receiving 25% HSA (171). These dogs developed a delayed type III hypersensitivity reaction with AKI, hypoalbuminemia, proteinuria as well as immune complex deposition and vasculitis. As the use of HSA increases, more reports of allergic reactions might be expected to emerge thus proving a fuller picture on HSA safety in animals.
Both healthy (169, 170, 179) and critically ill dogs (179) developed anti-human albumin antibodies within 7 days after administration of 25% HSA. Antibody concentrations were highest 4–6 weeks after HSA administration in the critically ill dogs. Interestingly, one study reported that 7% of dogs had anti-HSA antibodies without previous HSA infusion (179). The latter could be attributed to cross-reaction with anti-bovine albumin antibodies that developed when dogs were exposed to bovine albumin through vaccination or ingestion (180). This not only shows that HSA albumin should not be administered over longer periods of time (several days), but also some patients might be previously sensitized to the molecule and show reactions on initial administration.
Canine serum albumin was evaluated in two prospective, small-scale, clinical studies. One study evaluated 14 dogs with septic peritonitis and found increased serum albumin concentrations, COP, and Doppler blood pressure 2 h after CSA administration. No acute or delayed adverse events were found (181). In a second study (presented in an abstract form to date), six healthy Beagles received CSA at a dose of 1 g/kg at three different time points (on days 1, 2, and 14). Dogs were monitored for evidence of transfusion-associated complications during the infusions and at 1, 2, 12, and 24 h after each infusion. The follow-up period was 28 days. The authors concluded that repeated infusions appeared safe, with no adverse changes to physical examination, hematologic, or biochemical parameters (182).
Recommendations for Albumin Use in Small Animals
In contrast to people, albumin solutions in small animals have been mainly used to increase and maintain plasma albumin concentrations (CRI over hours and days) in patients that may or may not be cardiovascularly unstable. Only one study to date has shown an association of albumin administration and increased patient survival (173). Immediate and delayed adverse reactions in some critically ill dogs have been observed in several studies, with some of them proving fatal (169–172, 174, 177, 179). Critical illness does not seem to be protective against adverse immune reactions to HSA solutions. Cats seem to be less sensitive to HSA transfusions compared with dogs; however, this is based on only three studies with a total of 211 cats (174, 175, 178). Due to the potential adverse effects, HSA should be considered only for severely hypoalbuminemic patients in which the use of isotonic crystalloids alone carries a high risk of edema and/or in which hypoalbuminemia itself is a greater risk factor than the potential adverse effects of HSA. Autologous albumin products should be used when possible. Intravenous HSA or CSA is not a substitute for a proper nutritional management in critically ill patients.
Depending on the hydration status of the patient, albumin administration may be considered at plasma albumin concentrations below 10–15 g/L (1.0–1.5 g/dl) (173–176, 178). Albumin supplementation should discontinue once the serum concentration has reached 20–25 g/L (2.0–2.5 g/dl) (152). The recommended formula for calculation of the albumin dose is dose albumin (g) = (desired albumin g/L – patient albumin g/L) × 0.3 × kg body weight (171) over 12–24 h. In the authors' experience, this formula overestimates albumin requirements, so we recommend re-evaluating plasma albumin concentration after 50% of the calculated dose and adjusting requirements accordingly. Alternatively, a dose of 2 g/kg over 10 h is recommended (183). Some authors prefer to administer diluted HSA (5 or 10%) which may be better tolerated due to a more physiological COP (173, 175, 178), but there is no conclusive proof for fewer adverse effects compared with the more concentrated form. The described length of administration varies between authors from 4–6 h (184) to 12 h (173) to several days (174, 175). Based on current literature, it is not clear whether the length of administration, the total dose, or both have an influence on delayed adverse reactions. Supraphysiological concentrations are not desired as it might lead to suppression of hepatic albumin synthesis (152).
Allogenic Plasma Products
Use of Allogenic Plasma Products in People
The two major indications for administration of fresh frozen plasma (FFP) in people are to prevent hemorrhage and to stop active bleeding (185). Common clinical scenarios involving the use of FFP include liver failure, reversal of anticoagulant toxicity, temporary treatment of coagulation factor deficiencies, cardiopulmonary bypass surgery, disseminated intravascular coagulation, and thrombotic thrombocytopenic purpura (185). Its use has also been reported in patients with acute pancreatitis, uremic syndrome, and severe burns (185). Furthermore, FFP is a component of “massive transfusion” protocols and used in patients with traumatic coagulopathy. Substantial controversy surrounds the indications and efficacy of administration of FFP in people (186–195). While the evidence of its efficacy in preventing or stopping bleeding is somewhat robust, other less common indications are not evidence based.
Use of Allogenic Plasma Products in Small Animals
In veterinary medicine, FFP is most commonly used to prevent or stop bleeding (196). However, its indications extend beyond treating or preventing coagulopathies. Fresh frozen plasma is administered as a means of albumin supplementation as well as a volume expander (197, 198). It is specifically advocated to prevent further dilution of albumin and clotting factors that can result from the administration of large volumes of crystalloids or synthetic colloids. Clear guidelines for the use of FFP in veterinary patients are lacking (196). To the authors' knowledge, there are no studies investigating the effects of FFP specifically when used for fluid resuscitation.
Several retrospective studies have documented the reasons for FFP administration in small animals. In a 1999 study, 74 dogs received FFP to provide albumin in 63%, coagulation factors in 47%, α-macroglobulin in 10%, and immunoglobulins in 13% of patients (197). Another retrospective study compared the use of FFP in 283 dogs and 25 cats between two decades (1996–1998 and 2006–2008) (198). The main reasons for FFP use were coagulopathies, hypoalbuminemia, and acute pancreatitis (i.e., provision of α-macroglobulin). In the later decade, significantly more FFP was used for the treatment of coagulopathies, while significantly less FFP was used to counteract hypoalbuminemia or support patients with acute pancreatitis. Fresh frozen plasma has been advocated for administration to people with pancreatitis, and, by extrapolation, to dogs (199). The rationale for the use is to supplement plasma α-macroglobulin. This is a broad-acting protease inhibitor that prevents intravascular damage caused by circulating activated pancreatic proteases. Alpha-macroglobulin becomes depleted during the systemic inflammation (200). A multicenter RCT in people from 1991 demonstrated no benefit of administering high-volume FFP to patients with acute severe pancreatitis (201). Hence, the practice to use FFP in people with pancreatitis has long been overturned. One retrospective study compared the outcome of dogs with pancreatitis that had been or had not been given FFP (202). In that study, dogs that had received FFP had a higher mortality, and no benefit was apparent. As the authors did not control for the severity of illness, and FFP is more likely to be administered to more severely affected patients, this might have biased the results (202). Two recent retrospective studies evaluated FFP transfusions in cats. One study in 36 cats found that coagulopathies were the most common reasons for FFP transfusion (94% of cats) (203). The other study evaluated FFP transfusions in 121 cats and found coagulopathies (83%), hemorrhage (35%), and hypotension (25%) to be the main reasons for administration (204). Median doses reported in dogs are 14 ml/kg (small dogs) and 5 ml/kg (large dogs), due to the administration per unit or ½ unit per dog (197). Another study reported median doses of 15–18 ml/kg (198). One study reported median doses of 6 ml/kg in cats (204).
Two retrospective studies evaluated effects of FFP transfusion in dogs. Median prothrombin time and activated partial thromboplastin time were significantly decreased after plasma transfusion. Notably, pre- and post-transfusion serum albumin and COP were not different in patients receiving FFP. In addition, no association was found between the volume of infused FFP and outcome (198). Another study focusing on adverse effects of blood product administration in dogs reported 8% mild adverse reactions after FFP transfusions (205) compared with 22% after pRBC administrations. This contrasts with the findings in people where transfusion reactions after administration of FFP are more common than after pRBC transfusions and include life-threatening acute lung injury, and circulatory overload (185). The aforementioned retrospective studies also evaluated effects of FFP administration in cats. In one study on 36 cats, coagulation parameters seemed to improve in 90% of the cats after transfusion (203). Fifteen percent of the cats experienced potential transfusion reactions, including respiratory signs, fever, and gastro-intestinal signs. In the other study (121 cats), Doppler blood pressure increased, and they were significantly less likely to be coagulopathic after transfusion. Similarly, 16% of the cats had possible reactions, such as increased body temperature (64% of cats with reactions) and tachypnea/dyspnea (47% of cats with reactions) (204).
Recently, cryopoor plasma (CPP), a byproduct of the production of cryoprecipitate, has been used as an albumin source (206–208). The albumin concentration in canine CPP is significantly higher than in FFP, suggesting that it may be a possible treatment for hypoalbuminemia in dogs while avoiding volume overload (207). A retrospective study of 10 critically ill dogs found that plasma albumin increased after a CPP CRI (206). The dogs received a median total dose of 31 ml/kg CPP over a median duration of 16 h and a mean rate of administration of 1.8 ml/kg/h. In a recent case report (a 44-kg dog with septic shock secondary to enterectomy dehiscence), CPP was used as a source of canine albumin and intravascular volume. In this case, CPP was provided for a total volume of 58 units CPP over 9 days (approximately CPP 227 ml/kg). The authors proposed that CPP CRI may be a viable alternative to CSA (and HSA) for oncotic support and albumin replacement, but multiple units from different donors may increase the risk of an adverse reaction. Cryopoor plasma is only available in specialized institutions or commercial veterinary blood banks.
Recommendations for Plasma Products Use in Small Animals
With the decline in use of synthetic colloids and in countries where albumin solutions are either not available or not popular, plasma products might be the only natural colloid alternative. However, the current evidence is not sufficient to enable strong recommendations for the use of plasma products in small animals. Allogenic plasma transfusions seem to have a low risk for adverse effects, although cats seem to be more sensitive than dogs. As an albumin source, the administration of plasma (either FFP or CPP) appears to be relatively safe, although large volumes might be necessary. Fresh frozen plasma contains ~30 g/L (3.0 g/dl) of albumin. If the goal of the plasma transfusion is to achieve adequate albumin supplementation, substantial volume per kilogram is needed, particularly when dealing with ongoing albumin loss [i.e., 22 ml/kg of plasma to raise the albumin concentration by 5 g/L (0.5 g/dl)] (152). Administering such volumes, potentially derived from various donors, should be weighed against the risk of volume overload and other transfusion reactions. The use of CPP rather than FFP, where volume expansion is not required, might prevent volume overload. Because these products are usually administered per unit (or ½ unit), because of the necessity to thaw, dose regimens (in ml/kg) vary depending on patient size. Fresh frozen plasma should not be used as a first-line volume expander unless specifically indicated. This is because it is a valuable and expensive resource that should not be wasted, and it is a potential source of adverse reactions in already debilitated animals. Furthermore, it technically cannot be administered as a first-line volume expander due to the necessity to thaw, which takes 30–45 min (209). However, if other indications for FFP administration (e.g., overt or imminent coagulopathy) are present, FFP can be considered as part of resuscitative efforts. While established dosing regimens for FFP as a volume expander do not exist, the authors recommend similar doses as other natural or synthetic colloids.
Hemoglobin-Based Oxygen Carrier (HBOC) Solutions
Hemoglobin-based oxygen carrier (HBOC) solutions consist of chemically purified and polymerized bovine hemoglobin (Hb) suspended in a crystalloid carrier fluid. The hemoglobin in those solutions is a colloid with a high molecular weight (up to 250 kDa in some) and a relatively high COP. Several products have been developed over the last few decades, seeking the advantage of a long shelf-life and low infectious risk alternative to pRBC transfusions (210). The safety and efficacy of HBOCs has been evaluated in various species mostly through experimental studies and have yielded conflicting results. In hemorrhagic shock models, HBOCs seem to show promising efficacy in restoring cardiovascular parameters and in some studies parallel the oxygen carrying capacity of pRBCs (211–219). However, they are associated with considerable adverse effects such as systemic vasoconstriction, pulmonary and systemic hypertension, reactive oxygen species formation, and renal and myocardial injury (220, 221). As a consequence, HBOCs are not currently authorized for use in people (222).
The use of HBOCs has been described two small-scale clinical studies in dogs. In a prospective randomized study in 20 dogs with GDV, five times smaller volumes of Oxyglobin (OPK Biotech Netherlands BV) were required to achieve resuscitation end-points as compared with HES 450/0.7 (223). Another prospective randomized study compared the use of Oxyglobin and pRBC in 12 anemic dogs with babesiosis. The authors concluded that Oxyglobin had comparable effects with pRBC, with the exception that dogs treated with pRBC had a quicker return to habitus and appetite (224). In a retrospective study about the clinical use of Oxyglobin in 48 cats, a high risk of life-threatening circulatory overload was found in cats suffering from cardiac disease (225). Oxyglobin was authorized by the European Medicines Agency and Food and Drug Administration for use in anemic dogs in 1999 (212–214). However, it has been withdrawn from the market in most countries. Recently, a new highly purified low molecular weight (65 kDa) bovine blood–derived HBOC (Oxapex; New A Innovation Ltd., Kowloon, Hong Kong) has been authorized for use in anemic dogs in New Zealand and awaiting authorization in Australia, the USA, Canada, and the EU (Table 1). A canine hemorrhagic shock model showed improved hemodynamics, lactate, base excess, and tissue oxygenation after administration of various doses of Oxapex (226). In this study, high doses led to significant increases in pulmonary artery pressures (226). Further studies are required to establish the safety and efficacy of this new product.
Conclusion
Colloids, both natural and synthetic, were originally used with narrow indications. For instance, the original purpose of synthetic colloids was a cheap, long shelf-life “bridging fluid” to remain in the intravascular space while soldiers wounded on the frontline were transported to the field hospital where further stabilization took place. The original purpose of natural colloids was to replace missing blood components (albumin, clotting factors, fibrinogen) in various patients. In the decades that followed, their indications have expanded far beyond their original purpose, such as perioperative fluid therapy for HES and volume expansion for albumin. The expansion of their indications to potentially higher doses and use over longer periods of time (specifically for HES) may have contributed to more and more severe adverse effects being observed and, in some instances, has led to severe restrictions in their use.
Admittedly, veterinary emergency and critical care medicine does, to some extent, take into account the guidelines adopted in human medicine. However, veterinary medicine cannot directly follow the “attitudes” adopted in human medicine because of species differences, different patient cohorts, the availability and safety of some products, and the need to incorporate the owner's financial considerations into the decision-making process. Therefore, species-specific guidelines should be established.
We are living in an era where fluids are recognized as drugs with indications, contraindications, and adverse effects. Therefore, to ensure rational utilization of colloids in the face of weak and contradicting evidence, the authors recommend prescribing colloids on a case-by-case basis, similarly to prescribing any other drug.
Author Contributions
K-NA and IDY performed the literature search and wrote the article. All authors contributed to the article and approved the submitted version.
Funding
The series Fluid Therapy In Animals was organized by Senior Editor William Muir and the article processing charges associated with this manuscript are being paid for by a grant from Lincoln Memorial University.
Conflict of Interest
The authors declare that the research was conducted in the absence of any commercial or financial relationships that could be construed as a potential conflict of interest.
Supplementary Material
The Supplementary Material for this article can be found online at: https://www.frontiersin.org/articles/10.3389/fvets.2021.624049/full#supplementary-material
Abbreviations
AKI, acute kidney injury; COP, colloid osmotic pressure; CRI, constant rate infusion; CSA, canine serum albumin; EG, endothelial glycocalyx; ESL, endothelial surface layer; FFP, fresh frozen plasma; Hb, hemoglobin; HBOC, hemoglobin-based oxygen carrier; HES, hydroxyethyl starch; HSA, human serum albumin; EU, European Union; HES, hydroxyethyl starch; HSA, human serum albumin solutions; kDa, kilodalton; pRBC, packed red blood cell; RCT, randomized clinical trial; RRT, renal replacement therapy.
References
1. Hahn RG. Colloid fluids. In: Hahn RG, editor. Clinical Fluid Therapy in the Perioperative Setting. Cambridge: Cambridge University Press. (2016). p. 10–20. doi: 10.1017/CBO9781316401972.005
2. Adamik KN, Yozova ID, Regenscheit N. Controversies in the use of hydroxyethyl starch solution in small animal emergency and critical care. J Vet Emerg Crit Care. (2015) 25:20–47. doi: 10.1111/vec.12283
3. Finfer S, Liu B, Taylor C, Bellomo R, Billot L, Cook D, et al. Resuscitation fluid use in critically ill adults: an international cross-sectional study in 391 intensive care units. Crit Care. (2010) 14:R185. doi: 10.1186/cc9293
4. Glover PA, Rudloff E, Kirby R. Hydroxyethyl starch: a review of pharmacokinetics, pharmacodynamics, current products, and potential clinical risks, benefits, and use. J Vet Emerg Crit Care. (2014) 24:642–61. doi: 10.1111/vec.12208
5. Yozova ID, Howard J, Sigrist NE, Adamik KN. Current trends in volume replacement therapy and the use of synthetic colloids in small animals-An internet-Based survey (2016). Front Vet Sci. (2017) 4:140. doi: 10.3389/fvets.2017.00140
6. Hammond NE, Taylor C, Finfer S, Machado FR, An Y, Billot L, et al. Patterns of intravenous fluid resuscitation use in adult intensive care patients between 2007 and 2014: an international cross-sectional study. PLoS ONE. (2017) 12:e0176292. doi: 10.1371/journal.pone.0176292
7. Gaudette S, Hughes D, Boller M. The endothelial glycocalyx: structure and function in health and critical illness. J Vet Emerg Crit Care. (2020) 30:117–34. doi: 10.1111/vec.12925
8. Reitsma S, Slaaf DW, Vink H, van Zandvoort MA, oude Egbrink MG. The endothelial glycocalyx: composition, functions, and visualization. Pflugers Arch. (2007) 454:345–59. doi: 10.1007/s00424-007-0212-8
9. Pries AR, Secomb TW, Gaehtgens P. The endothelial surface layer. Pflugers Arch. (2000) 440:653–66. doi: 10.1007/s004240000307
10. Woodcock TE, Woodcock TM. Revised starling equation and the glycocalyx model of transvascular fluid exchange: an improved paradigm for prescribing intravenous fluid therapy. Br J Anaesth. (2012) 108:384–94. doi: 10.1093/bja/aer515
11. Becker BF, Chappell D, Bruegger D, Annecke T, Jacob M. Therapeutic strategies targeting the endothelial glycocalyx: acute deficits, but great potential. Cardiovasc Res. (2010) 87:300–10. doi: 10.1093/cvr/cvq137
12. Hahn RG, Lyons G. The half-life of infusion fluids: an educational review. Eur J Anaesthesiol. (2016) 33:475–82. doi: 10.1097/EJA.0000000000000436
13. Hahn RG, Dull RO, Zdolsek J. The extended starling principle needs clinical validation. Acta Anaesthesiol Scand. (2020) 64:884–7. doi: 10.1111/aas.13593
14. Hughes D, Boag A. Fluid therapy with macromolecular plasma volume expanders. In: DiBartola SP, editor. Fluid, Electrolyte, and Acid-Base Disorders in Small Animal Practice 4th ed. Missouri: Elsevier Saunders. (2012). p. 647–64. doi: 10.1016/B978-1-4377-0654-3.00034-2
15. Puyana J. Resuscitation of hypovolemic shock. In: Fink M AE, Vincent JL, Kochanek PM, editor. Textbook of Critical Care. 5th ed. Philadelphia: WB Saunders CO. (2005). p. 1939–40.
16. Jacob M, Chappell D, Hofmann-Kiefer K, Helfen T, Schuelke A, Jacob B, et al. The intravascular volume effect of ringer's lactate is below 20%: a prospective study in humans. Crit Care. (2012) 16:R86. doi: 10.1186/cc11344
17. Haupt MT, Rackow EC. Colloid osmotic pressure and fluid resuscitation with hetastarch, albumin, and saline solutions. Crit Care Med. (1982) 10:159–62. doi: 10.1097/00003246-198203000-00004
18. Rackow EC, Falk JL, Fein IA, Siegel JS, Packman MI, Haupt MT, et al. Fluid resuscitation in circulatory shock: a comparison of the cardiorespiratory effects of albumin, hetastarch, and saline solutions in patients with hypovolemic and septic shock. Crit Care Med. (1983) 11:839–50. doi: 10.1097/00003246-198311000-00001
19. Bruckner UB, Albrecht M, Frey L, Hein LG. Fluid resuscitation in canine traumatic-hemorrhagic shock: long-term comparison of hydroxyethyl starch vs. Ringer's lactate. Prog Clin Biol Res. (1987) 236B:197–204.
20. Chen S, Zhu X, Wang Q, Li W, Cheng D, Lei C, et al. The early effect of voluven, a novel hydroxyethyl starch (130/0.4), on cerebral oxygen supply and consumption in resuscitation of rabbit with acute hemorrhagic shock. J Trauma. (2009) 66:676–82. doi: 10.1097/TA.0b013e318173e7da
21. Friedman Z, Berkenstadt H, Preisman S, Perel A. A comparison of lactated ringer's solution to hydroxyethyl starch 6% in a model of severe hemorrhagic shock and continuous bleeding in dogs. Anesth Analg. (2003) 96:39–45, table of contents. doi: 10.1213/00000539-200301000-00009
22. Reinhart K, Rudolph T, Bredle DL, Cain SM. O2 uptake in bled dogs after resuscitation with hypertonic saline or hydroxyethylstarch. Am J Physiol. (1989) 257(1 Pt 2):H238–43. doi: 10.1152/ajpheart.1989.257.1.H238
23. Boura C, Caron A, Longrois D, Mertes PM, Labrude P, Menu P. Volume expansion with modified hemoglobin solution, colloids, or crystalloid after hemorrhagic shock in rabbits: effects in skeletal muscle oxygen pressure and use versus arterial blood velocity and resistance. Shock. (2003) 19:176–82. doi: 10.1097/00024382-200302000-00015
24. Zazzeron L, Gattinoni L, Caironi P. Role of albumin, starches and gelatins versus crystalloids in volume resuscitation of critically ill patients. Curr Opin Crit Care. (2016) 22:428–36. doi: 10.1097/MCC.0000000000000341
25. Jacob M, Rehm M, Orth V, Lotsch M, Brechtelsbauer H, Weninger E, et al. [Exact measurement of the volume effect of 6% hydoxyethyl starch 130/0.4 (Voluven) during acute preoperative normovolemic hemodilution]. Anaesthesist. (2003) 52:896–904. doi: 10.1007/s00101-003-0557-0
26. Muir WW 3rd, Wiese AJ. Comparison of lactated ringer's solution and a physiologically balanced 6% hetastarch plasma expander for the treatment of hypotension induced via blood withdrawal in isoflurane-anesthetized dogs. Am J Vet Res. (2004) 65:1189–94. doi: 10.2460/ajvr.2004.65.1189
27. Nelson A, Statkevicius S, Schott U, Johansson PI, Bentzer P. Effects of fresh frozen plasma, ringer's acetate and albumin on plasma volume and on circulating glycocalyx components following haemorrhagic shock in rats. Intensive Care Med Exp. (2016) 4:6. doi: 10.1186/s40635-016-0080-7
28. Silverstein D, Aldrich J, Haskins S, Drobatz K, Cowgill L. Assessment of changes in blood volume in response to resuscitative fluid administration in dogs. J Vet Emerg Crit Care. (2005) 15:185–92. doi: 10.1111/j.1476-4431.2005.00138.x
29. Hahn RG. Understanding volume kinetics. Acta Anaesthesiol Scand. (2020) 64:570–8. doi: 10.1111/aas.13533
30. Hilberath JN, Thomas ME, Smith T, Jara C, Fitzgerald DJ, Wilusz K, et al. Blood volume measurement by hemodilution: association with valve disease and re-evaluation of the allen formula. Perfusion. (2015) 30:305–11. doi: 10.1177/0267659114547250
31. Hedin A, Hahn RG. Volume expansion and plasma protein clearance during intravenous infusion of 5% albumin and autologous plasma. Clin Sci (Lond). (2005) 108:217–24. doi: 10.1042/CS20040303
32. Hahn RG, Bergek C, Geback T, Zdolsek J. Interactions between the volume effects of hydroxyethyl starch 130/0.4 and ringer s acetate. Crit Care. (2013) 17:R104. doi: 10.1186/cc12749
33. Svensen C, Hahn RG. Volume kinetics of ringer solution, dextran 70, and hypertonic saline in male volunteers. Anesthesiology. (1997) 87:204–12. doi: 10.1097/00000542-199708000-00006
34. Fleck A, Raines G, Hawker F, Trotter J, Wallace PI, Ledingham IM, et al. Increased vascular permeability: a major cause of hypoalbuminaemia in disease and injury. Lancet. (1985) 1:781–4. doi: 10.1016/S0140-6736(85)91447-3
35. Christensen P, Andersson J, Rasmussen SE, Andersen PK, Henneberg SW. Changes in circulating blood volume after infusion of hydroxyethyl starch 6% in critically ill patients. Acta Anaesthesiol Scand. (2001) 45:414–20. doi: 10.1034/j.1399-6576.2001.045004414.x
36. Margraf A, Herter JM, Kuhne K, Stadtmann A, Ermert T, Wenk M, et al. 6% Hydroxyethyl starch (HES 130/0.4) diminishes glycocalyx degradation and decreases vascular permeability during systemic and pulmonary inflammation in mice. Crit Care. (2018) 22:111. doi: 10.1186/s13054-017-1846-3
37. Qiu YZ, Sun H, Li F. [Effect of fluid resuscitation on capillary permeability and vascular endothelial growth factor in dogs with septic shock]. Zhongguo Wei Zhong Bing Ji Jiu Yi Xue. (2007) 19:270–3.
38. Tian J, Lin X, Guan R, Xu JG. The effects of hydroxyethyl starch on lung capillary permeability in endotoxic rats and possible mechanisms. Anesth Analg. (2004) 98:768–74, table of contents. doi: 10.1213/01.ANE.0000099720.25581.86
39. Marx G, Pedder S, Smith L, Swaraj S, Grime S, Stockdale H, et al. Attenuation of capillary leakage by hydroxyethyl starch (130/0.42) in a porcine model of septic shock. Crit Care Med. (2006) 34:3005–10. doi: 10.1097/01.CCM.0000242755.74063.ED
40. Wisselink W, Patetsios P, Panetta TF, Ramirez JA, Rodino W, Kirwin JD, et al. Medium molecular weight pentastarch reduces reperfusion injury by decreasing capillary leak in an animal model of spinal cord ischemia. J Vasc Surg. (1998) 27:109–16. doi: 10.1016/S0741-5214(98)70297-6
41. Chi OZ, Lu X, Wei HM, Williams JA, Weiss HR. Hydroxyethyl starch solution attenuates blood-brain barrier disruption caused by intracarotid injection of hyperosmolar mannitol in rats. Anesth Analg. (1996) 83:336–41. doi: 10.1213/00000539-199608000-00023
42. Oz MC, FitzPatrick MF, Zikria BA, Pinsky DJ, Duran WN. Attenuation of microvascular permeability dysfunction in postischemic striated muscle by hydroxyethyl starch. Microvasc Res. (1995) 50:71–9. doi: 10.1006/mvre.1995.1039
43. Zikria BA, Subbarao C, Oz MC, Shih ST, McLeod PF, Sachdev R, et al. Macromolecules reduce abnormal microvascular permeability in rat limb ischemia-reperfusion injury. Crit Care Med. (1989) 17:1306–9. doi: 10.1097/00003246-198912000-00012
44. Collis RE, Collins PW, Gutteridge CN, Kaul A, Newland AC, Williams DM, et al. The effect of hydroxyethyl starch and other plasma volume substitutes on endothelial cell activation; an in vitro study. Intensive Care Med. (1994) 20:37–41. doi: 10.1007/BF02425053
45. Groeneveld AB, Teule GJ, Bronsveld W, van den Bos GC, Thijs LG. Increased systemic microvascular albumin flux in septic shock. Intensive Care Med. (1987) 13:140–2. doi: 10.1007/BF00254801
46. Norberg A, Rooyackers O, Segersvard R, Wernerman J. Albumin kinetics in patients undergoing major abdominal surgery. PLoS ONE. (2015) 10:e0136371. doi: 10.1371/journal.pone.0136371
47. Komaromi A, Estenberg U, Hammarqvist F, Rooyackers O, Wernerman J, Norberg A. Simultaneous assessment of the synthesis rate and transcapillary escape rate of albumin in inflammation and surgery. Crit Care. (2016) 20:370. doi: 10.1186/s13054-016-1536-6
48. Kozar RA, Peng Z, Zhang R, Holcomb JB, Pati S, Park P, et al. Plasma restoration of endothelial glycocalyx in a rodent model of hemorrhagic shock. Anesth Analg. (2011) 112:1289–95. doi: 10.1213/ANE.0b013e318210385c
49. Adamson RH, Clark JF, Radeva M, Kheirolomoom A, Ferrara KW, Curry FE. Albumin modulates s1P delivery from red blood cells in perfused microvessels: mechanism of the protein effect. Am J Physiol Heart Circ Physiol. (2014) 306:H1011–7. doi: 10.1152/ajpheart.00829.2013
50. Jacob M, Bruegger D, Rehm M, Welsch U, Conzen P, Becker BF. Contrasting effects of colloid and crystalloid resuscitation fluids on cardiac vascular permeability. Anesthesiology. (2006) 104:1223–31. doi: 10.1097/00000542-200606000-00018
51. Jacob M, Bruegger D, Rehm M, Stoeckelhuber M, Welsch U, Conzen P, et al. The endothelial glycocalyx affords compatibility of starling's principle and high cardiac interstitial albumin levels. Cardiovasc Res. (2007) 73:575–86. doi: 10.1016/j.cardiores.2006.11.021
52. Zeng Y, Adamson RH, Curry FR, Tarbell JM. Sphingosine-1-phosphate protects endothelial glycocalyx by inhibiting syndecan-1 shedding. Am J Physiol Heart Circ Physiol. (2014) 306:H363–72. doi: 10.1152/ajpheart.00687.2013
53. Rehm M, Zahler S, Lotsch M, Welsch U, Conzen P, Jacob M, et al. Endothelial glycocalyx as an additional barrier determining extravasation of 6% hydroxyethyl starch or 5% albumin solutions in the coronary vascular bed. Anesthesiology. (2004) 100:1211–23. doi: 10.1097/00000542-200405000-00025
54. Milford EM, Reade MC. Resuscitation fluid choices to preserve the endothelial glycocalyx. Crit Care. (2019) 23:77. doi: 10.1186/s13054-019-2369-x
55. Aldecoa C, Llau JV, Nuvials X, Artigas A. Role of albumin in the preservation of endothelial glycocalyx integrity and the microcirculation: a review. Ann Intensive Care. (2020) 10:85. doi: 10.1186/s13613-020-00697-1
56. Borrelli A, Maurella C, Lippi I, Ingravalle F, Botto A, Tarducci A, et al. Evaluation of the effects of hydroxyethyl starch (130/0.4) administration as a constant rate infusion on plasma colloid osmotic pressure in hypoabluminemic dogs. J Vet Emerg Crit Care. (2020) 30:550–7. doi: 10.1111/vec.13003
57. Smiley LE. The use of hetastarch for plasma expansion. Probl Vet Med. (1992) 4:652–67. doi: 10.1111/j.1939-1676.1996.tb02066.x
58. Moore LE, Garvey MS. The effect of hetastarch on serum colloid oncotic pressure in hypoalbuminemic dogs. J Vet Intern Med. (1996) 10:300–3.
59. Gauthier V, Holowaychuk MK, Kerr CL, Bersenas AM, Wood RD. Effect of synthetic colloid administration on hemodynamic and laboratory variables in healthy dogs and dogs with systemic inflammation. J Vet Emerg Crit Care. (2014) 24:251–8. doi: 10.1111/vec.12188
60. Smiley LE, Garvey MS. The use of hetastarch as adjunct therapy in 26 dogs with hypoalbuminemia: a phase two clinical trial. J Vet Intern Med. (1994) 8:195–202. doi: 10.1111/j.1939-1676.1994.tb03215.x
61. Liss D. Colloid Therapy for Critically Ill Patients. (2012). Available online at: https://todaysveterinarypractice.com/todays-technician-colloid-therapy-for-critically-ill-patients/ (accessed June 04, 2021).
62. Trepanier L. (ed). Practical Tips for Fluid and Colloid Therapy. Birmingham: WSAVA/FECAVA/BSAVA World Congress 2012 (2012). doi: 10.22233/20412495.0912.24 Available online at: https://www.vin.com/apputil/content/defaultadv1.aspx?id=5328368&pid=11349 (accessed June 04, 2021).
63. Brooks A, Thomovsky E, Johnson P. Natural and synthetic colloids in veterinary medicine. Top Companion Anim Med. (2016) 31:54–60. doi: 10.1053/j.tcam.2016.08.003
64. Wong C, Koenig A. The colloid controversy: are colloids bad and what are the options? Vet Clin North Am Small Anim Pract. (2017) 47:411–21. doi: 10.1016/j.cvsm.2016.09.008
65. Wiedermann CJ, Joannidis M. Accumulation of hydroxyethyl starch in human and animal tissues: a systematic review. Intensive Care Med. (2014) 40:160–70. doi: 10.1007/s00134-013-3156-9
66. Dickenmann M, Oettl T, Mihatsch MJ. Osmotic nephrosis: acute kidney injury with accumulation of proximal tubular lysosomes due to administration of exogenous solutes. Am J Kidney Dis. (2008) 51:491–503. doi: 10.1053/j.ajkd.2007.10.044
67. Ertmer C, Kohler G, Rehberg S, Morelli A, Lange M, Ellger B, et al. Renal effects of saline-based 10% pentastarch versus 6% tetrastarch infusion in ovine endotoxemic shock. Anesthesiology. (2010) 112:936–47. doi: 10.1097/ALN.0b013e3181d3d493
68. Huter L, Simon TP, Weinmann L, Schuerholz T, Reinhart K, Wolf G, et al. Hydroxyethylstarch impairs renal function and induces interstitial proliferation, macrophage infiltration and tubular damage in an isolated renal perfusion model. Crit Care. (2009) 13:R23. doi: 10.1186/cc7726
69. Moran M, Kapsner C. Acute renal failure associated with elevated plasma oncotic pressure. N Engl J Med. (1987) 317:150–3. doi: 10.1056/NEJM198707163170306
70. Hartog CS, Reuter D, Loesche W, Hofmann M, Reinhart K. Influence of hydroxyethyl starch (HES) 130/0.4 on hemostasis as measured by viscoelastic device analysis: a systematic review. Intensive Care Med. (2011) 37:1725–37. doi: 10.1007/s00134-011-2385-z
71. Kozek-Langenecker SA. Effects of hydroxyethyl starch solutions on hemostasis. Anesthesiology. (2005) 103:654–60. doi: 10.1097/00000542-200509000-00031
72. Brunkhorst FM, Engel C, Bloos F, Meier-Hellmann A, Ragaller M, Weiler N, et al. Intensive insulin therapy and pentastarch resuscitation in severe sepsis. N Engl J Med. (2008) 358:125–39. doi: 10.1056/NEJMoa070716
73. Myburgh JA, Finfer S, Bellomo R, Billot L, Cass A, Gattas D, et al. Hydroxyethyl starch or saline for fluid resuscitation in intensive care. N Engl J Med. (2012) 367:1901–11. doi: 10.1056/NEJMoa1209759
74. Perner A, Haase N, Guttormsen AB, Tenhunen J, Klemenzson G, Aneman A, et al. Hydroxyethyl starch 130/0.42 versus ringer's acetate in severe sepsis. N Engl J Med. (2012) 367:124–34. doi: 10.1056/NEJMoa1204242
75. Chappell D, Jacob M. Twisting and ignoring facts on hydroxyethyl starch is not very helpful. Scand J Trauma Resusc Emerg Med. (2013) 21:85. doi: 10.1186/1757-7241-21-85
76. Guidet B, Martinet O, Boulain T, Philippart F, Poussel JF, Maizel J, et al. Assessment of hemodynamic efficacy and safety of 6% hydroxyethylstarch 130/0.4 vs. 0.9% NaCl fluid replacement in patients with severe sepsis: The CRYSTMAS study. Crit Care. (2012) 16:R94. doi: 10.1186/cc11358
77. James MF, Michell WL, Joubert IA, Nicol AJ, Navsaria PH, Gillespie RS. Resuscitation with hydroxyethyl starch improves renal function and lactate clearance in penetrating trauma in a randomized controlled study: the fIRST trial (Fluids in resuscitation of severe trauma). Br J Anaesth. (2011) 107:693–702. doi: 10.1093/bja/aer229
78. Annane D, Siami S, Jaber S, Martin C, Elatrous S, Declere AD, et al. Effects of fluid resuscitation with colloids vs crystalloids on mortality in critically ill patients presenting with hypovolemic shock: the cRISTAL randomized trial. JAMA. (2013) 310:1809–17. doi: 10.1001/jama.2013.280502
79. Editors-in-Chief statement regarding published clinical trials conducted without IRB approval by Joachim Boldt. Minerva anestesiologica. (2011) 77:562–3.
80. Farrugia A. The boldt affair: correcting a collective failure. Anesth Analg. (2012) 115:207. doi: 10.1213/ANE.0b013e318256faf2
82. Gattas DJ, Dan A, Myburgh J, Billot L, Lo S, Finfer S, et al. Fluid resuscitation with 6 % hydroxyethyl starch (130/0.4 and 130/0.42) in acutely ill patients: systematic review of effects on mortality and treatment with renal replacement therapy. Intensive Care Med. (2013) 39:558–68. doi: 10.1007/s00134-013-2840-0
83. Haase N, Perner A, Hennings LI, Siegemund M, Lauridsen B, Wetterslev M, et al. Hydroxyethyl starch 130/0.38-0.45 versus crystalloid or albumin in patients with sepsis: systematic review with meta-analysis and trial sequential analysis. BMJ. (2013) 346:f839. doi: 10.1136/bmj.f839
84. Mutter TC, Ruth CA, Dart AB. Hydroxyethyl starch (HES) versus other fluid therapies: effects on kidney function. Cochr Database Syst Rev. (2013) 7:CD007594. doi: 10.1002/14651858.CD007594.pub3
85. Zarychanski R, Abou-Setta AM, Turgeon AF, Houston BL, McIntyre L, Marshall JC, et al. Association of hydroxyethyl starch administration with mortality and acute kidney injury in critically ill patients requiring volume resuscitation: a systematic review and meta-analysis. JAMA. (2013) 309:678–88. doi: 10.1001/jama.2013.430
86. Adamik KN, Yozova ID. Starch wars-New episodes of the saga. Changes in regulations on hydroxyethyl starch in the European Union. Front Vet Sci. (2018) 5:336. doi: 10.3389/fvets.2018.00336
87. Kancir AS, Pleckaitiene L, Hansen TB, Ekelof NP, Pedersen EB. Lack of nephrotoxicity by 6% hydroxyethyl starch 130/0.4 during hip arthroplasty: a randomized controlled trial. Anesthesiology. (2014) 121:948–58. doi: 10.1097/ALN.0000000000000413
88. Pagel JI, Rehm M, Kammerer T, Hulde N, Speck E, Briegel J, et al. Hydroxyethyl starch 130/0.4 and its impact on perioperative outcome: a Propensity score matched controlled observation study. Anesth Analg. (2018) 126:1949–56. doi: 10.1213/ANE.0000000000002778
89. Futier E, Garot M, Godet T, Biais M, Verzilli D, Ouattara A, et al. Effect of hydroxyethyl starch vs saline for volume replacement therapy on death or postoperative complications among high-Risk patients undergoing major abdominal surgery: the fLASH randomized clinical trial. JAMA. (2020) 323:225–36. doi: 10.1001/jama.2019.20833
90. Ertmer C, Zwissler B, Van Aken H, Christ M, Spohr F, Schneider A, et al. Fluid therapy and outcome: a prospective observational study in 65 german intensive care units between 2010 and 2011. Ann Intensive Care. (2018) 8:27. doi: 10.1186/s13613-018-0364-z
91. Unal MN, Reinhart K. Understanding the harms of hES: a Review of the evidence to date. Turk J Anaesthesiol Reanim. (2019) 47:81–91. doi: 10.5152/TJAR.2019.72681
92. Wiedermann CJ. Clinical trials in volume resuscitation with hydroxyethyl starch: focus on risk of bias. Turk J Anaesthesiol Reanim. (2020) 48:258–60. doi: 10.5152/TJAR.2020.56563
93. Kancir AS, Johansen JK, Ekeloef NP, Pedersen EB. The effect of 6% hydroxyethyl starch 130/0.4 on renal function, arterial blood pressure, and vasoactive hormones during radical prostatectomy: a randomized controlled trial. Anesth Analg. (2015) 120:608–18. doi: 10.1213/ANE.0000000000000596
94. Jacob M, Fellahi JL, Chappell D, Kurz A. The impact of hydroxyethyl starches in cardiac surgery: a meta-analysis. Crit Care. (2014) 18:656. doi: 10.1186/s13054-014-0656-0
95. Boyd CJ, Claus MA, Raisis AL, Cianciolo R, Bosio E, Hosgood G, et al. Evaluation of biomarkers of kidney injury following 4% succinylated gelatin and 6% hydroxyethyl starch 130/0.4 administration in a canine hemorrhagic shock model. J Vet Emerg Crit Care. (2019) 29:132–42. doi: 10.1111/vec.12814
96. Diniz MS, Teixeira-Neto FJ, Celeita-Rodriguez N, Girotto CH, Fonseca MW, Oliveira-Garcia AC, et al. Effects of 6% tetrastarch and lactated ringer's solution on extravascular lung water and markers of acute renal injury in hemorrhaged, isoflurane-Anesthetized healthy dogs. J Vet Intern Med. (2018) 32:712–21. doi: 10.1111/jvim.14853
97. Hayes G, Benedicenti L, Mathews K. Retrospective cohort study on the incidence of acute kidney injury and death following hydroxyethyl starch (HES 10% 250/0.5/5:1) administration in dogs (2007-2010). J Vet Emerg Crit Care. (2015) 26:35–40. doi: 10.1111/vec.12412
98. Sigrist NE, Kalin N, Dreyfus A. Changes in serum creatinine concentration and acute kidney injury (AKI) grade in dogs treated with hydroxyethyl starch 130/0.4 from 2013 to 2015. J Vet Intern Med. (2017) 31:434–41. doi: 10.1111/jvim.14645
99. Sigrist NE, Kalin N, Dreyfus A. Effects of hydroxyethyl starch 130/0.4 on serum creatinine concentration and development of acute kidney injury in nonazotemic cats. J Vet Intern Med. (2017) 31:1749–56. doi: 10.1111/jvim.14813
100. Yozova ID, Howard J, Adamik KN. Retrospective evaluation of the effects of administration of tetrastarch (hydroxyethyl starch 130/0.4) on plasma creatinine concentration in dogs (2010-2013): 201 dogs. J Vet Emerg Crit Care. (2016) 26:568–77. doi: 10.1111/vec.12483
101. Yozova ID, Howard J, Adamik KN. Effect of tetrastarch (hydroxyethyl starch 130/0.4) on plasma creatinine concentration in cats: a retrospective analysis (2010-2015). J Feline Med Surg. (2017) 19:1073–9. doi: 10.1177/1098612X16676160
102. Boyd C, Claus M, Sharp C, Raisis A, Hosgood G, Smart L. Biomarkers of acute kidney injury in dogs after 6% hydroxyethyl starch 130/0.4 or hartmann's solution: a Randomized blinded clinical trial. J Vet Emerg Crit. (2019) 29:13056. doi: 10.1111/vec.13056
103. Schmid SM, Cianciolo RE, Drobatz KJ, Sanchez M, Price JM, King LG. Postmortem evaluation of renal tubular vacuolization in critically ill dogs. J Vet Emerg Crit Care. (2019) 29:279–87. doi: 10.1111/vec.12837
104. Classen J, Adamik KN, Weber K, Rubenbauer S, Hartmann K. In vitro effect of hydroxyethyl starch 130/0.42 on canine platelet function. Am J Vet Res. (2012) 73:1908–12. doi: 10.2460/ajvr.73.12.1908
105. Falco S, Bruno B, Maurella C, Bellino C, D'Angelo A, Gianella P, et al. In vitro evaluation of canine hemostasis following dilution with hydroxyethyl starch (130/0.4) via thromboelastometry. J Vet Emerg Crit Care. (2012) 22:640–5. doi: 10.1111/j.1476-4431.2012.00816.x
106. Reuteler A, Axiak-Flammer S, Howard J, Adamik KN. Comparison of the effects of a balanced crystalloid-based and a saline-based tetrastarch solution on canine whole blood coagulation and platelet function. J Vet Emerg Crit Care. (2017) 27:23–34. doi: 10.1111/vec.12556
107. Wurlod VA, Howard J, Francey T, Schweighauser A, Adamik KN. Comparison of the in vitro effects of saline, hypertonic hydroxyethyl starch, hypertonic saline, and two forms of hydroxyethyl starch on whole blood coagulation and platelet function in dogs. J Vet Emerg Crit Care. (2015) 25:474–87. doi: 10.1111/vec.12320
108. Albrecht NA, Howard J, Kovacevic A, Adamik KN. In vitro effects of 6 % hydroxyethyl starch 130/0.42 solution on feline whole blood coagulation measured by rotational thromboelastometry. BMC Vet Res. (2016) 12:155. doi: 10.1186/s12917-016-0767-8
109. Diniz MS, Teixeira-Neto FJ, Goncalves DS, Celeita-Rodriguez N, Girotto CH, Fonseca MW, et al. Effects of 6% tetrastarch or lactated ringer's solution on blood coagulation in hemorrhaged dogs. J Vet Intern Med. (2018) 32:1927–33. doi: 10.1111/jvim.15327
110. McBride D, Hosgood G, Raisis A, Smart L. Platelet closure time in anesthetized greyhounds with hemorrhagic shock treated with hydroxyethyl starch 130/0.4 or 0.9% sodium chloride infusions. J Vet Emerg Crit Care. (2016) 26:509–15. doi: 10.1111/vec.12468
111. Boyd CJ, Claus MA, Raisis AL, Hosgood G, Sharp CR, Smart L. Hypocoagulability and platelet dysfunction are exacerbated by synthetic colloids in a canine hemorrhagic shock model. Front Vet Sci. (2018) 5:279. doi: 10.3389/fvets.2018.00279
112. Iannucci C, Dirkmann D, Howard J, Adamik KN. A prospective randomized open-label trial on the comparative effects of 6% hydroxyethyl starch 130/0.4 versus polyionic isotonic crystalloids on coagulation parameters in dogs with spontaneous hemoperitoneum. J Vet Emerg Crit Care. (2020) 31:32–42. doi: 10.1111/vec.13018
113. Smart L, Jandrey KE, Kass PH, Wierenga JR, Tablin F. The effect of hetastarch (670/0.75) in vivo on platelet closure time in the dog. J Vet Emerg Crit Care. (2009) 19:444–9. doi: 10.1111/j.1476-4431.2009.00464.x
114. Ertmer C, Rehberg S, Van Aken H, Westphal M. Relevance of non-albumin colloids in intensive care medicine. Best Pract Res Clin Anaesth. (2009) 23:193–212. doi: 10.1016/j.bpa.2008.11.001
115. Food and Drug Administration. List of Drug Products That Have Been Withdrawn or Removed From the Market for Reasons of Safety or Effectiveness. 63 FR 54082 (1998). Available online at: https://www.gpo.gov/fdsys/pkg/FR-1998-10-08/pdf/98-26923.pdf (accessed June 04, 2021).
116. Kief H, Engelbart K, Arnold G, Bahr H. [Vacuolar reabsorption of native and digested gelatin (so-called osmotic nephrosis)]. Virchows Arch B Cell Pathol. (1968) 1:240–50.
117. Schick MA, Isbary TJ, Schlegel N, Brugger J, Waschke J, Muellenbach R, et al. The impact of crystalloid and colloid infusion on the kidney in rodent sepsis. Intensive Care Med. (2010) 36:541–8. doi: 10.1007/s00134-009-1704-0
118. Kastrup M, Carl M, Spies C, Sander M, Markewitz A, Schirmer U. Clinical impact of the publication of s3 guidelines for intensive care in cardiac surgery patients in germany: results from a postal survey. Acta Anaesthesiol Scand. (2013) 57:206–13. doi: 10.1111/aas.12009
119. Moeller C, Fleischmann C, Thomas-Rueddel D, Vlasakov V, Rochwerg B, Theurer P, et al. How safe is gelatin? A systematic review and meta-analysis of gelatin-containing plasma expanders vs crystalloids and albumin. J Crit Care. (2016) 35:75–83. doi: 10.1016/j.jcrc.2016.04.011
120. Bayer O, Reinhart K, Kohl M, Kabisch B, Marshall J, Sakr Y, et al. Effects of fluid resuscitation with synthetic colloids or crystalloids alone on shock reversal, fluid balance, and patient outcomes in patients with severe sepsis: a prospective sequential analysis. Crit Care Med. (2012) 40:2543–51. doi: 10.1097/CCM.0b013e318258fee7
121. Smith S. The use of haemaccel. Vet Anaesth Analg. (1977) 7: LXXXI-LXXXVI. doi: 10.1111/j.1467-2995.1977.tb00466.x
122. Glowaski MM, Moon-Massat PF, Erb HN, Barr SC. Effects of oxypolygelatin and dextran 70 on hemostatic variables in dogs. Vet Anaesth Analg. (2003) 30:202–10. doi: 10.1046/j.1467-2995.2003.00111.x
123. Buck RK, Bester L, Boustead KJ, Kadwa AR, Zeiler GE. Blood acid-base, haematological and haemostatic effects of hydroxyethyl starch (130/0.4) compared to succinylated gelatin colloid infusions in normovolaemic dogs. J S Afr Vet Assoc. (2020) 91:e1–e9. doi: 10.4102/jsava.v91i0.1990
124. Svensén C, Rodhe P. Intravascular volume replacement therapy. In: Hugh C. Hemmings TDE, editor. Pharmacology and Physiology for Anesthesia. W.B. Saunders, Philadelphia, PA (2013). p. 574–92. doi: 10.1016/B978-1-4377-1679-5.00033-8
125. Atik M. Dextran 40 and dextran 70. A review. Arch Surg. (1967) 94:664–72. doi: 10.1001/archsurg.1967.01330110080011
126. Ferraboli R, Malheiro PS, Abdulkader RC, Yu L, Sabbaga E, Burdmann EA. Anuric acute renal failure caused by dextran 40 administration. Ren Fail. (1997) 19:303–6. doi: 10.3109/08860229709026293
127. Schortgen F, Girou E, Deye N, Brochard L, Group CS. The risk associated with hyperoncotic colloids in patients with shock. Intensive Care Med. (2008) 34:2157–68. doi: 10.1007/s00134-008-1225-2
128. de Jonge E, Levi M. Effects of different plasma substitutes on blood coagulation: a comparative review. Crit Care Med. (2001) 29:1261–7. doi: 10.1097/00003246-200106000-00038
129. Batlle J, del Rio F, Lopez Fernandez MF, Martin R, Lopez Borrasca A. Effect of dextran on factor vIII/von willebrand factor structure and function. Thromb Haemost. (1985) 54:697–9. doi: 10.1055/s-0038-1660100
130. Eriksson M, Saldeen T. Effect of dextran on plasma tissue plasminogen activator (t-PA) and plasminogen activator inhibitor-1 (PAI-1) during surgery. Acta Anaesthesiol Scand. (1995) 39:163–6. doi: 10.1111/j.1399-6576.1995.tb04036.x
131. Sigurjonsson J, Hedman D, Bansch P, Schott U. Comparison of dextran and albumin on blood coagulation in patients undergoing major gynaecological surgery. Perioper Med (Lond). (2018) 7:21. doi: 10.1186/s13741-018-0100-0
132. Ljungstrom KG, Renck H, Hedin H, Richter W, Wiholm BE. Hapten inhibition and dextran anaphylaxis. Anaesthesia. (1988) 43:729–32. doi: 10.1111/j.1365-2044.1988.tb05741.x
133. Ljungstrom KG. Safety of dextran in relation to other colloids–ten years experience with hapten inhibition. Infusionsther Transfusionsmed. (1993) 20:206–10. doi: 10.1159/000222845
134. Druml W, Polzleitner D, Laggner A, Lenz K, Ulrich W. Dextran-40, acute renal failure, and elevated plasma oncotic pressure. N Engl J Med. (1988) 318:252–4. doi: 10.1056/NEJM198801283180414
135. Matheson NA, Diomi P. Renal failure after the administration of dextran 40. Surg Gynecol Obstet. (1970) 131:661–8.
136. Morgan T, Little JM. Renal failure and low-molecular-weight dextran. Br Med J. (1967) 1:635. doi: 10.1136/bmj.1.5540.635-c
137. Mailloux L, Swartz CD, Capizzi R, Kim KE, Onesti G, Ramirez O, et al. Acute renal failure after administration of low-molecular weight dextran. N Engl J Med. (1967) 277:1113–8. doi: 10.1056/NEJM196711232772103
138. Hvidt LN, Perner A. High dosage of dextran 70 is associated with severe bleeding in patients admitted to the intensive care unit for septic shock. Dan Med J. (2012) 59:A4531.
139. Rasmussen AM, Jakobsen R, Strom T, Carlsson M, Dahler-Eriksen B, Toft P. More complications in patients with septic shock treated with dextran compared with crystalloids. Dan Med J. (2015) 62:A5018.
140. Ljungstrom KG. The antithrombotic efficacy of dextran. Acta Chir Scand Suppl. (1988) 543:26–30.
141. Allen DA, Schertel ER, Muir WW III, Valentine AK. Hypertonic saline/dextran resuscitation of dogs with experimentally induced gastric dilatation-volvulus shock. Am J Vet Res. (1991) 52:92–6.
142. Horton JW, Walker PB. Small-volume hypertonic saline dextran resuscitation from canine endotoxin shock. Ann Surg. (1991) 214:64–73. doi: 10.1097/00000658-199107000-00011
143. Schertel ER, Allen DA, Muir WW, Brourman JD, DeHoff WD. Evaluation of a hypertonic saline-dextran solution for treatment of dogs with shock induced by gastric dilatation-volvulus. J Am Vet Med Assoc. (1997) 210:226–30.
144. Schertel ER, Allen DA, Muir WW, Hansen BD. Evaluation of a hypertonic sodium chloride/dextran solution for treatment of traumatic shock in dogs. J Am Vet Med Assoc. (1996) 208:366–70.
145. Velasco IT, Rocha e Silva M, Oliveira MA, Oliveira MA, Silva RI. Hypertonic and hyperoncotic resuscitation from severe hemorrhagic shock in dogs: a comparative study. Crit Care Med. (1989) 17:261–4. doi: 10.1097/00003246-198903000-00012
146. Fantoni DT, Auler Junior JO, Futema F, Cortopassi SR, Migliati ER, Faustino M, et al. Intravenous administration of hypertonic sodium chloride solution with dextran or isotonic sodium chloride solution for treatment of septic shock secondary to pyometra in dogs. J Am Vet Med Assoc. (1999) 215:1283–7.
147. Concannon KT, Haskins SC, Feldman BF. Hemostatic defects associated with two infusion rates of dextran 70 in dogs. Am J Vet Res. (1992) 53:1369–75.
148. Zhao JX, Wang B, You GX, Wang Y, Chen G, Wang Q, et al. Hypertonic saline dextran ameliorates organ damage in beagle hemorrhagic shock. PLoS ONE. (2015) 10:e0136012. doi: 10.1371/journal.pone.0136012
149. Raoufinia R, Mota A, Keyhanvar N, Safari F, Shamekhi S, Abdolalizadeh J. Overview of albumin and its purification methods. Adv Pharm Bull. (2016) 6:495–507. doi: 10.15171/apb.2016.063
150. AG A-ahsCB. Albumin (Human) 25% Solution ALBURX® 25. Available online at: https://dailymed.nlm.nih.gov/dailymed/fda/fdaDrugXsl.cfm?setid=ad74b7f5-b380-47ab-b19d-eef92c8eb7a4&type=display (accessed June 04, 2021).
151. Fanali G, di Masi A, Trezza V, Marino M, Fasano M, Ascenzi P. Human serum albumin: from bench to bedside. Mol Aspects Med. (2012) 33:209–90. doi: 10.1016/j.mam.2011.12.002
152. Mazzaferro E, Rudloff E, Kirby R. The role of albumin replacement in the critically ill veterinary patient. J Vet Emerg Crit Care. (2002) 12:113–24. doi: 10.1046/j.1435-6935.2002.00025.x
153. Chan D, Freeman L, Rozanski E, Rush J. Colloid osmotic pressure of parenteral nutrition components and intravenous fluids. J Vet Emerg Crit. (2001) 11:269–307. doi: 10.1111/j.1476-4431.2001.tb00065.x
154. Damiani E, Ince C, Orlando F, Pierpaoli E, Cirioni O, Giacometti A, et al. Effects of the infusion of 4% or 20% human serum albumin on the skeletal muscle microcirculation in endotoxemic rats. PLoS ONE. (2016) 11:e0151005. doi: 10.1371/journal.pone.0151005
155. Goldwasser P, Feldman J. Association of serum albumin and mortality risk. J Clin Epidemiol. (1997) 50:693–703. doi: 10.1016/S0895-4356(97)00015-2
156. Vincent JL, Dubois MJ, Navickis RJ, Wilkes MM. Hypoalbuminemia in acute illness: is there a rationale for intervention? A meta-analysis of cohort studies and controlled trials. Ann Surg. (2003) 237:319–34. doi: 10.1097/01.SLA.0000055547.93484.87
157. Caironi P, Gattinoni L. The clinical use of albumin: the point of view of a specialist in intensive care. Blood Transfus. (2009) 7:259–67.
158. Caironi P, Tognoni G, Masson S, Fumagalli R, Pesenti A, Romero M, et al. Albumin replacement in patients with severe sepsis or septic shock. N Engl J Med. (2014) 370:1412–21. doi: 10.1056/NEJMoa1305727
159. Caraceni P, Domenicali M, Tovoli A, Napoli L, Ricci CS, Tufoni M, et al. Clinical indications for the albumin use: still a controversial issue. Eur J Intern Med. (2013) 24:721–8. doi: 10.1016/j.ejim.2013.05.015
160. Wiedermann CJ. Phases of fluid management and the roles of human albumin solution in perioperative and critically ill patients. Curr Med Res Opin. (2020) 36:1961–73. doi: 10.1080/03007995.2020.1840970
161. European Association for the Study of the Liver. Electronic address eee, European Association for the Study of the L. EASL clinical practice guidelines for the management of patients with decompensated cirrhosis. J Hepatol. (2018) 69:406–60. doi: 10.1016/j.jhep.2018.03.024
162. Juce J. Use of blood components in the intensive care unit. In: Parrillo J, Dellinger R, editors. Critical Care Medicine - Principles of Diagnosis and Management in the Adult. 3rd ed: Philadelphia, PA: Mosby. (2008). p. 1655–75. doi: 10.1016/B978-032304841-5.50082-0
163. Cochrane Injuries Group Albumin R. Human albumin administration in critically ill patients: systematic review of randomised controlled trials. BMJ. (1998) 317:235–40. doi: 10.1136/bmj.317.7153.235
164. Finfer S, Bellomo R, Boyce N, French J, Myburgh J, Norton R, et al. A comparison of albumin and saline for fluid resuscitation in the intensive care unit. N Engl J Med. (2004) 350:2247–56. doi: 10.1056/NEJMoa040232
165. Lewis SR, Pritchard MW, Evans DJ, Butler AR, Alderson P, Smith AF, et al. Colloids versus crystalloids for fluid resuscitation in critically ill people. Coch Database Syst Rev. (2018) 8:CD000567. doi: 10.1002/14651858.CD000567.pub7
166. Kongsgaard UE, Holtan A, Perner A. Changes in colloid solution sales in nordic countries. Acta Anaesthesiol Scand. (2018) 62:522–30. doi: 10.1111/aas.13057
167. Rhodes A, Evans LE, Alhazzani W, Levy MM, Antonelli M, Ferrer R, et al. Surviving sepsis campaign: international guidelines for management of sepsis and septic shock: 2016. Intensive Care Med. (2017) 43:304–77. doi: 10.1007/s00134-017-4683-6
168. Peters T. All About Albumin: Biochemistry, Genetics and Medical Applications. San Diego: Academic Press (1996). p. c1996.
169. Cohn LA, Kerl ME, Lenox CE, Livingston RS, Dodam JR. Response of healthy dogs to infusions of human serum albumin. Am J Vet Res. (2007) 68:657–63. doi: 10.2460/ajvr.68.6.657
170. Francis AH, Martin LG, Haldorson GJ, Lahmers KK, Luther TY, Alperin DC, et al. Adverse reactions suggestive of type iII hypersensitivity in six healthy dogs given human albumin. J Am Vet Med Assoc. (2007) 230:873–9. doi: 10.2460/javma.230.6.873
171. Mazzaferro EM, Balakrishnan A, Hackner SG, Forman M, Foster JD, Calabro J, et al. Delayed type iII hypersensitivity reaction with acute kidney injury in two dogs following administration of concentrated human albumin during treatment for hypoalbuminemia secondary to septic peritonitis. J Vet Emerg Crit Care. (2020) 30:574–80. doi: 10.1111/vec.12976
172. Powell C, Thompson L, Murtaugh RJ. Type III hypersensitivity reaction with immune complex deposition in 2 critically ill dogs administered human serum albumin. J Vet Emerg Crit Care. (2013) 23:598–604. doi: 10.1111/vec.12085
173. Trow AV, Rozanski EA, Delaforcade AM, Chan DL. Evaluation of use of human albumin in critically ill dogs: 73 cases (2003-2006). J Am Vet Med Assoc. (2008) 233:607–12. doi: 10.2460/javma.233.4.607
174. Mathews K, Barry M. The use of 25% human serum albumin: outcome and efficacy in raising serum albumin and systemic blood pressure in critically ill dogs and cats. J Vet Emerg Crit Care. (2005) 15:110–8. doi: 10.1111/j.1476-4431.2005.00141.x
175. Vigano F, Perissinotto L, Bosco VR. Administration of 5% human serum albumin in critically ill small animal patients with hypoalbuminemia: 418 dogs and 170 cats (1994-2008). J Vet Emerg Crit Care. (2010) 20:237–43. doi: 10.1111/j.1476-4431.2010.00526.x
176. Horowitz FB, Read RL, Powell LL. A retrospective analysis of 25% human serum albumin supplementation in hypoalbuminemic dogs with septic peritonitis. Can Vet J. (2015) 56:591–7.
177. Loyd KA, Cocayne CG, Cridland JM, Hause WR. Retrospective evaluation of the administration of 25% human albumin to dogs with protein-losing enteropathy: 21 cases (2003-2013). J Vet Emerg Crit Care. (2016) 26:587–92. doi: 10.1111/vec.12484
178. Vigano F, Blasi C, Carminati N, Giudice E. Prospective review of clinical hypersensitivity reactions after administration of 5% human serum albumin in 40 critically ill cats. Top Companion Anim Med. (2019) 35:38–41. doi: 10.1053/j.tcam.2019.03.004
179. Martin LG, Luther TY, Alperin DC, Gay JM, Hines SA. Serum antibodies against human albumin in critically ill and healthy dogs. J Am Vet Med Assoc. (2008) 232:1004–9. doi: 10.2460/javma.232.7.1004
180. Ohmori K, Masuda K, Maeda S, Kaburagi Y, Kurata K, Ohno K, et al. IgE reactivity to vaccine components in dogs that developed immediate-type allergic reactions after vaccination. Vet Immunol Immunopathol. (2005) 104:249–56. doi: 10.1016/j.vetimm.2004.12.003
181. Craft EM, Powell LL. The use of canine-specific albumin in dogs with septic peritonitis. J Vet Emerg Crit Care. (2012) 22:631–9. doi: 10.1111/j.1476-4431.2012.00819.x
182. Enders B, Musulin S, Holowaychuk M, Hale A. Repeated infusion of lyophilized canine albumin safely and effectively increases serum albumin and colloid osmotic pressure in healthy dogs (abstract). J Vet Emerg Crit. (2018) 28:S5. doi: 10.1111/vec.12758
183. Balakrishnan A, Silverstein D. Shock fluids and fluid challange. In: Silverstein DS, Hopper K, editors. Small Animal Critical Care Medicine. 2nd ed. St. Louis, MO: Saunders. (2015). p. 321–7. doi: 10.1016/B978-1-4557-0306-7.00060-X
184. Boller E, Otto C. Sepsis and septic shock. In: Silverstein D, Hopper K, editors. Small Animal Critical Care Medicine. 2nd Edition ed. Saunders. (2015). p. 472–80. doi: 10.1016/B978-1-4557-0306-7.00091-X
185. Yang L, Stanworth S, Hopewell S, Doree C, Murphy M. Is fresh-frozen plasma clinically effective? An update of a systematic review of randomized controlled trials. Transfusion. (2012) 52:1673–86; quiz doi: 10.1111/j.1537-2995.2011.03515.x
186. Supapannachart S, Siripoonya P, Boonwattanasoontorn W, Kanjanavanit S. Neonatal polycythemia: effects of partial exchange transfusion using fresh frozen plasma, haemaccel and normal saline. J Med Assoc Thai. (1999) 82 (Suppl. 1):S82–6.
187. Trimble AS, Osborn JJ, Kerth WJ, Gerbode F. The prophylactic use of fresh frozen plasma after extracorporeal circulation. J Thorac Cardiovasc Surg. (1964) 48:314–6. doi: 10.1016/S0022-5223(19)32798-9
188. Triulzi DJ, editor. Blood Transfusion Therapy: A Physician's Handbook. 7th edn. ed. Bethesda: American Association of Blood Banks (2002).
189. Vittecoq D, Mattlinger B, Barre-Sinoussi F, Courouce AM, Rouzioux C, Doinel C, et al. Passive immunotherapy in aIDS: a randomized trial of serial human immunodeficiency virus-positive transfusions of plasma rich in p24 antibodies versus transfusions of seronegative plasma. J Infect Dis. (1992) 165:364–8. doi: 10.1093/infdis/165.2.364
190. von Sommoggy S, Fraunhofer J, Jelen-Esselborn S, Stemberger A. [Coagulation changes during aortofemoral bifurcation bypass: is volume and plasma substitution possible with hydroxyethyl starch alone?]. Anaesthesist. (1990) 39:353–60.
191. Yarranton H, Cohen H, Pavord SR, Benjamin S, Hagger D, Machin SJ. Venous thromboembolism associated with the management of acute thrombotic thrombocytopenic purpura. Br J Haematol. (2003) 121:778–85. doi: 10.1046/j.1365-2141.2003.04360.x
192. Williamson LM, Cardigan R, Prowse CV. Methylene blue-treated fresh-frozen plasma: what is its contribution to blood safety? Transfusion. (2003) 43:1322–9. doi: 10.1046/j.1537-2995.2003.00483.x
193. Williamson LM, Llewelyn CA, Fisher NC, Allain JP, Bellamy MC, Baglin TP, et al. A randomized trial of solvent/detergent-treated and standard fresh-frozen plasma in the coagulopathy of liver disease and liver transplantation. Transfusion. (1999) 39:1227–34. doi: 10.1046/j.1537-2995.1999.39111227.x
194. Belcher PR, Muriithi EW. Coronary artery bypass grafting surgery without the routine application of blood products: is it feasible? Eur J Cardiothorac Surg. (2001) 20:1078–9. doi: 10.1016/S1010-7940(01)00935-6
195. Chapman J, Hick R. Monitoring the nation's blood supply. Transfusion. (2003) 43:1639; author reply −40. doi: 10.1046/j.1537-2995.2003.00552.x
196. Beer KS, Silverstein DC. Controversies in the use of fresh frozen plasma in critically ill small animal patients. J Vet Emerg Crit Care. (2015) 25:101–6. doi: 10.1111/vec.12280
197. Logan JC, Callan MB, Drew K, Marryott K, Oakley DA, Jefferies L, et al. Clinical indications for use of fresh frozen plasma in dogs: 74 dogs (October through december 1999). J Am Vet Med Assoc. (2001) 218:1449–55. doi: 10.2460/javma.2001.218.1449
198. Snow SJ, Ari Jutkowitz L, Brown AJ. Trends in plasma transfusion at a veterinary teaching hospital: 308 patients (1996-1998 and 2006-2008). J Vet Emerg Criti Care. (2010) 20:441–5. doi: 10.1111/j.1476-4431.2010.00557.x
199. Cuschieri A, Wood RA, Cumming JR, Meehan SE, Mackie CR. Treatment of acute pancreatitis with fresh frozen plasma. Br J Surg. (1983) 70:710–2. doi: 10.1002/bjs.1800701205
200. Murtaugh RJ, Jacobs RM. Serum antiprotease concentrations in dogs with spontaneous and experimentally induced acute pancreatitis. Am J Vet Res. (1985) 46:80–3.
201. Leese T, Holliday M, Watkins M, Thomas WM, Neoptolemos JP, Hall C, et al. A multicentre controlled clinical trial of high-volume fresh frozen plasma therapy in prognostically severe acute pancreatitis. Ann R Coll Surg Engl. (1991) 73:207–14.
202. Weatherton LK, Streeter EM. Evaluation of fresh frozen plasma administration in dogs with pancreatitis: 77 cases (1995-2005). J Vet Emerg Crit Care. (2009) 19:617–22. doi: 10.1111/j.1476-4431.2009.00483.x
203. Mansi ET, Waldrop JE, Davidow EB. Retrospective evaluation of the indications, safety and effects of fresh frozen plasma transfusions in 36 cats (2014-2018). J Feline Med Surg. (2020) 22:696–704. doi: 10.1177/1098612X19876728
204. Lane WG, Sinnott-Stutzman VB. Retrospective evaluation of fresh frozen plasma use in 121 cats: 2009-2016. J Vet Emerg Crit Care. (2020) 30:558–66. doi: 10.1111/vec.12972
205. Bruce JA, Kriese-Anderson L, Bruce AM, Pittman JR. Effect of premedication and other factors on the occurrence of acute transfusion reactions in dogs. J Vet Emerg Crit Care. (2015) 25:620–30. doi: 10.1111/vec.12327
206. Culler CA, Balakrishnan A, Yaxley PE, Guillaumin J. Clinical use of cryopoor plasma continuous rate infusion in critically ill, hypoalbuminemic dogs. J Vet Emerg Crit Care. (2019) 29:314–20. doi: 10.1111/vec.12834
207. Culler CA, Iazbik C, Guillaumin J. Comparison of albumin, colloid osmotic pressure, von willebrand factor, and coagulation factors in canine cryopoor plasma, cryoprecipitate, and fresh frozen plasma. J Vet Emerg Crit Care. (2017) 27:638–44. doi: 10.1111/vec.12671
208. Ropski MK, Guillaumin J, Monnig AA, Townsend K, McLoughlin MA. Use of cryopoor plasma for albumin replacement and continuous antimicrobial infusion for treatment of septic peritonitis in a dog. J Vet Emerg Crit Care. (2017) 27:348–56. doi: 10.1111/vec.12583
209. Westphal RG, Tindle B, Howard PL, Golden EA, Page GA. Rapid thawing of fresh frozen plasma. Am J Clin Pathol. (1982) 78:220–2. doi: 10.1093/ajcp/78.2.220
210. Day T. Current development and use of hemoglobin-based oxygen-carrying (HBOC) solutions. J Vet Emerg Crit. (2003) 13:77–93. doi: 10.1046/j.1435-6935.2003.00084.x
211. Spahn DR, Leone BJ, Reves JG, Pasch T. Cardiovascular and coronary physiology of acute isovolemic hemodilution: a review of nonoxygen-carrying and oxygen-carrying solutions. Anesth Analg. (1994) 78:1000–21. doi: 10.1213/00000539-199405000-00029
212. Vlahakes GJ, Lee R, Jacobs EE Jr., LaRaia PJ, Austen WG. Hemodynamic effects and oxygen transport properties of a new blood substitute in a model of massive blood replacement. J Thorac Cardiovasc Surg. (1990) 100:379–88. doi: 10.1016/S0022-5223(19)35530-8
213. Bosman RJ, Minten J, Lu HR, Van Aken H, Flameng W. Free polymerized hemoglobin versus hydroxyethyl starch in resuscitation of hypovolemic dogs. Anesth Analg. (1992) 75:811–7. doi: 10.1213/00000539-199211000-00028
214. Harringer W, Hodakowski GT, Svizzero T, Jacobs EE Jr., Vlahakes GJ. Acute effects of massive transfusion of a bovine hemoglobin blood substitute in a canine model of hemorrhagic shock. Eur J Cardiothorac Surg. (1992) 6:649–54; discussion 54. doi: 10.1016/1010-7940(92)90189-5
215. Standl T, Horn P, Wilhelm S, Greim C, Freitag M, Freitag U, et al. Bovine haemoglobin is more potent than autologous red blood cells in restoring muscular tissue oxygenation after profound isovolaemic haemodilution in dogs. Can J Anaesth. (1996) 43:714–23. doi: 10.1007/BF03017957
216. Driessen B, Jahr JS, Lurie F, Gunther RA. Inadequacy of low-volume resuscitation with hemoglobin-based oxygen carrier hemoglobin glutamer-200 (bovine) in canine hypovolemia. J Vet Pharmacol Ther. (2001) 24:61–71. doi: 10.1046/j.1365-2885.2001.00307.x
217. Driessen B, Jahr JS, Lurie F, Griffey SM, Gunther RA. Effects of haemoglobin-based oxygen carrier hemoglobin glutamer-200 (bovine) on intestinal perfusion and oxygenation in a canine hypovolaemia model. Br J Anaesth. (2001) 86:683–92. doi: 10.1093/bja/86.5.683
218. Cheung AT, Jahr JS, Driessen B, Duong PL, Chan MS, Lurie F, et al. The effects of hemoglobin glutamer-200 (bovine) on the microcirculation in a canine hypovolemia model: a noninvasive computer-assisted intravital microscopy study. Anesth Analg. (2001) 93:832–8. doi: 10.1097/00000539-200110000-00007
219. Moon PF, Bliss SP, Posner LP, Erb HN, Nathanielsz PW. Fetal oxygen content is restored after maternal hemorrhage and fluid replacement with polymerized bovine hemoglobin, but not with hetastarch, in pregnant sheep. Anesth Analg. (2001) 93:142–50. doi: 10.1097/00000539-200107000-00029
220. Buehler PW, D'Agnillo F, Schaer DJ. Hemoglobin-based oxygen carriers: from mechanisms of toxicity and clearance to rational drug design. Trends Mol Med. (2010) 16:447–57. doi: 10.1016/j.molmed.2010.07.006
221. Winslow RM. Oxygen: the poison is in the dose. Transfusion. (2013) 53:424–37. doi: 10.1111/j.1537-2995.2012.03774.x
222. Mozzarelli A, Ronda L, Faggiano S, Bettati S, Bruno S. Haemoglobin-based oxygen carriers: research and reality towards an alternative to blood transfusions. Blood Transfus. (2010) 8(Suppl. 3):s59–68. doi: 10.2450/2010.010S
223. Haak CE, Rudloff E, Kirby R. Comparison of hb-200 and 6% hetastarch 450/0.7 during initial fluid resuscitation of 20 dogs with gastric dilatation-volvulus. J Vet Emerg Crit Care. (2012) 22:201–10. doi: 10.1111/j.1476-4431.2012.00726.x
224. Zambelli AB, Leisewitz AL. A prospective, randomized comparison of oxyglobin (HB-200) and packed red blood cell transfusion for canine babesiosis. J Vet Emerg Crit Care. (2009) 19:102–12. doi: 10.1111/j.1476-4431.2009.00386.x
225. Weingart C, Kohn B. Clinical use of a haemoglobin-based oxygen carrying solution (Oxyglobin) in 48 cats (2002-2006). J Feline Med Surg. (2008) 10:431–8. doi: 10.1016/j.jfms.2007.10.012
Keywords: albumin, dextran, gelatin, HBOC, hydroxyethyl starch, fresh frozen plasma, fluid therapy
Citation: Adamik K-N and Yozova ID (2021) Colloids Yes or No? - a “Gretchen Question” Answered. Front. Vet. Sci. 8:624049. doi: 10.3389/fvets.2021.624049
Received: 30 October 2020; Accepted: 05 May 2021;
Published: 02 July 2021.
Edited by:
Dez Hughes, Independent Researcher, Melbourne, AustraliaReviewed by:
K. Humm, Royal Veterinary College (RVC), United KingdomAmanda Boag, IVC Evidensia, United Kingdom
Copyright © 2021 Adamik and Yozova. This is an open-access article distributed under the terms of the Creative Commons Attribution License (CC BY). The use, distribution or reproduction in other forums is permitted, provided the original author(s) and the copyright owner(s) are credited and that the original publication in this journal is cited, in accordance with accepted academic practice. No use, distribution or reproduction is permitted which does not comply with these terms.
*Correspondence: Katja-Nicole Adamik, katja.adamik@vetsuisse.unibe.ch