- 1General Diagnostic Department, Istituto Zooprofilattico Sperimentale del Lazio e della Toscana “M. Aleandri”, Rome, Italy
- 2Fondazione Bioparco, Rome, Italy
- 3Zoomarine Acquatic Park, Torvaianica, Rome, Italy
Avian malaria is a parasitic disease of birds caused by protozoa belonging to the genus Plasmodium, within the order Haemosporida. Penguins are considered particularly susceptible, and outbreaks in captive populations can lead to high mortality. We used a multidisciplinary approach to investigate the death due to avian malaria, occurred between 2015 and 2019, in eight African penguins (Spheniscus demersus) kept in two Italian zoos located in central Italy, and situated about 30 km apart. We also provided information about the presence and circulation of Plasmodium spp. in mosquitoes in central Italy by sampling mosquitoes in both zoos where penguin mortalities occurred. In the eight dead penguins, gross and histopathological lesions were consistent with those previously observed by other authors in avian malaria outbreaks. Organs from dead penguins and mosquitoes collected in both zoos were tested for avian malaria parasites by using a PCR assay targeting the partial mitochondrial conserved region of the cytochrome b gene. Identification at species level was performed by sequencing analysis. Plasmodium matutinum was detected in both dead penguins and in mosquitoes (Culex pipiens), while Plasmodium vaughani in Culex pipiens only. Parasites were not found in any of the PCR tested Aedes albopictus samples. Based on our phylogenetic analysis, we detected three previously characterized lineages: Plasmodium matutinum LINN1 and AFTRU5, P. vaughani SYAT05. In Culex pipiens we also identified two novel lineages, CXPIP32 (inferred morphospecies Plasmodium matutinum) and CXPIP33 (inferred morphospecies P. vaughani). Significantly, LINN1 and AFTRU5 were found to be associated to penguin deaths, although only LINN1 was detected both in penguins (along the years of the study) and in Culex pipiens, while AFTRU5 was detected in a single penguin dead in 2017. In conclusion, in our study Plasmodium matutinum was found to cause avian malaria in captive penguins kept in Europe, with Culex pipiens being its most probable vector. Our results are in agreement with previous studies suggesting that Culex pipiens is one of the main vectors of Plasmodium spp. in Europe and the Northern Hemisphere. Zoos maintaining captive penguins in temperate areas where Culex pipiens is abundant should be well aware of the risks of avian malaria, and should put every effort to prevent outbreaks, in particular during the periods when the number of vectors is higher.
Introduction
Avian malaria is a parasitic disease of birds caused by protozoa belonging to the genus Plasmodium, within the order Haemosporida (1, 2). The genus Plasmodium is the largest genus within this order and currently consists of more than 50 species worldwide distributed both in tropical and temperate areas (1–7). Avian malaria identification at species level has traditionally relied on morphological analyses of parasites seen on blood smears. More recently, molecular characterization has led to the description of multiple parasites lineages, and these lineages have been gradually assigned to their respective morphospecies (2, 6, 8, 9). DNA-barcoding of multicellular eukaryotes often target sections of mitochondrial genes, specifically of the cytochrome c oxidase subunit I and of the cytochrome b (cyt b). In particular, the cyt b has became the reference gene for DNA-barcoding approaches of avian haemosporidians (2). Plasmodium spp. have been reported from almost all avian orders, Galliformes, Columbiformes and Passeriformes being those presenting the greatest parasite diversity (7, 10). In particular, the cosmopolitan P. relictum has been recorded in over 400 bird species, belonging to 70 families (3, 7, 10). In Europe, several Plasmodium species have been so far detected in both birds and mosquitoes, such as P. relictum, P. vaughani (2, 11–15), P. elongatum and P. matutinum (2, 4, 5, 16, 17). However, as in some studies dealing with avian malaria specific identification of the parasite was not performed, neither morphologically nor through molecular or combined approaches, this list of species should be considered presumably incomplete (18). Indeed, the issue of relating molecular genetic data to classical taxonomy, primarily based on the morphology of blood stages, and the vector and host susceptibility of the parasites, has already been raised (2).
Despite the widespread diffusion of the parasites, avian malaria is not considered a major cause of epidemics or mortalities in natural bird populations that co-evolved with these protozoa (19). Conversely, it is responsible of significant mortalities in captive birds in zoos or collections, when naive bird species are for the first time exposed to the infection, in particular during the spring-summer seasons (1, 3). Avian Plasmodium species are transmitted by mosquitoes of many genera within the family Culicidae (10). Different species within the genus Culex (Cx.) are considered the major vectors in the Northern Hemisphere and in particular Cx. pipiens (1, 11, 19–21).
Several species of penguins have been recognized to be highly susceptible to avian malaria in both tropical and temperate areas (1, 6, 22). In particular, the presence of Plasmodium infection has been frequently reported both in rehabilitation centers (6, 23–27) and in captive penguin colonies in zoos (9, 17, 28–38), where the disease often caused high morbidity and mortality.
In the Northern Hemisphere and in particular in Europe, the majority of documented avian malaria cases in captive penguins have been caused by P. relictum (39–45) and P. elongatum (17), although it has to be considered that in some cases identification at species level was not reported (1, 35). As wild birds, in particular passerines, are frequently infected with Plasmodium spp. (3), the Plasmodium species infecting penguins in a zoo would substantially reflect those present in the local avifauna (27). However, possible differences regarding the virulence of different lineages in different species have been suggested and need to be further investigated (9, 20).
At present, in Italy, information regarding the epidemiology of avian malaria and the impact of the disease in both wild and captive birds is rather few. Some earlier and more recent studies reported the presence of different Plasmodium species in wild birds without evidence of disease, including P. matutinum, P. giovannolai (16, 46), P. relictum, and P. vaughani (47), P. circumflexum and P. polare (48). In another study on blood-fed mosquitoes from northern Italy, Martínez-de la Puente et al. (21) reported the presence of six Plasmodium lineages in different mosquito species, including P. relictum and P. vaughani. In this study, Cx. pipiens showed by far the highest parasite prevalence, although one Plasmodium lineage was also detected in Aedes (Ae.) albopicus (21).
Following the death presumptively attributed to avian malaria in African penguins kept in two zoos located in Central Italy, a multidisciplinary study was carried out in order to describe the pathological findings, to identify and characterize by molecular methods the Plasmodium species and lineages responsible for these deaths, and to evaluate the mosquito species possibly involved in the transmission of the disease.
Materials and Methods
Studied Populations and Locations
During the summer seasons between 2015 and 2019 the deaths of eight African penguins (Spheniscus demersus), tentatively attributed to avian malaria, were recorded among the colonies of two zoos located in Central Italy (Lazio region, Ecoregion: Mediterranean forests, woodlands, and scrub) and situated about 30 km apart, named Bioparco (41.91696 N, 12.48817 E) and Zoomarine (41.63364 N, 12.45661 E). Zoomarine recorded five dead penguins (animal IDs Z1-Z5), while Bioparco three dead penguins (animal IDs B1-B3) (Table 1). These avian malaria deaths accounted for 80% (8/10) of the total penguin deaths at the two colonies during the study period. In all cases, deaths occurred suddenly without any apparent avian malaria-associated clinical sign. Information about zoo of origin, age of the animals and date of deaths of the eight penguins is reported in Table 1.
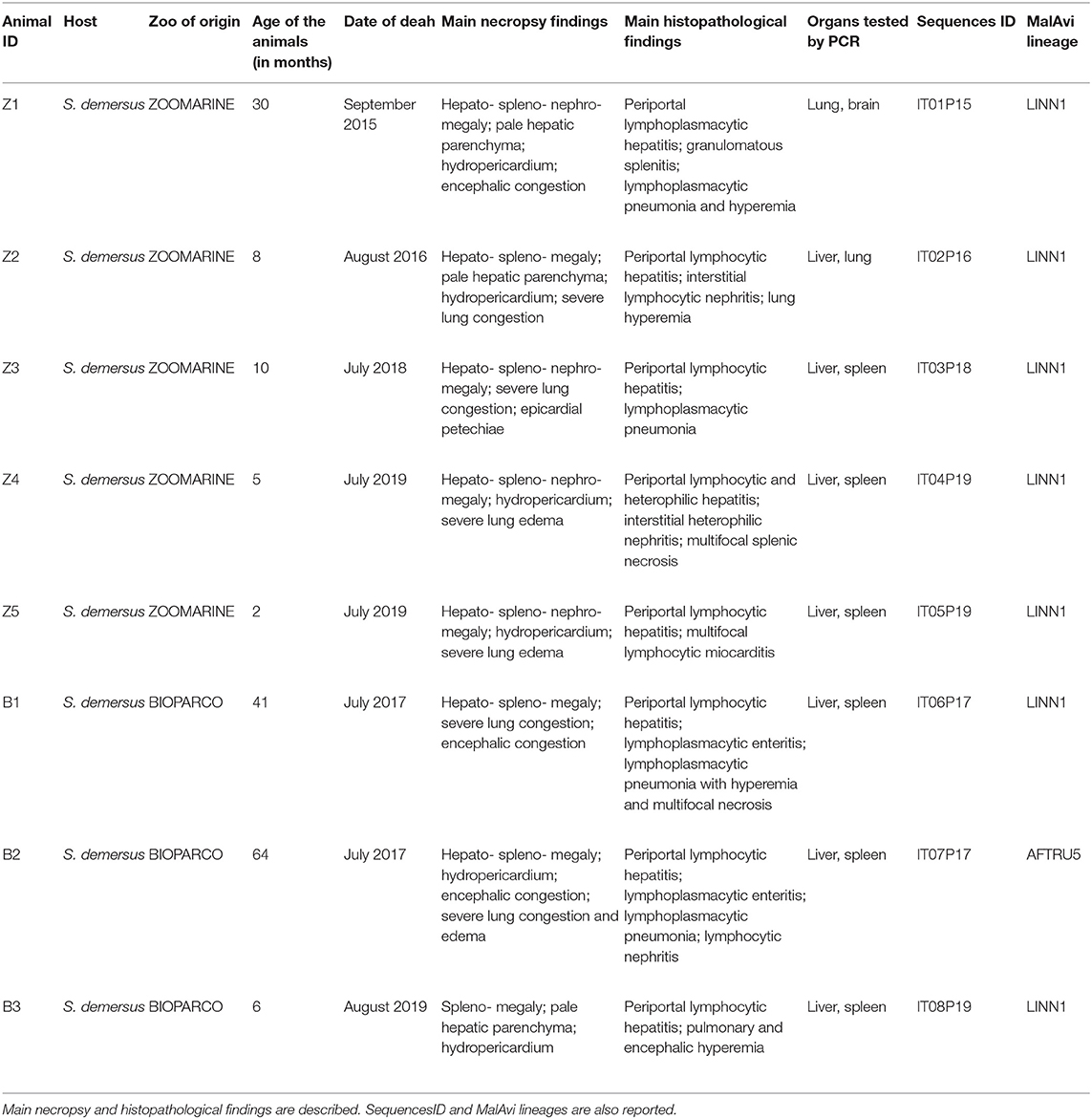
Table 1. Summary of the main metadata (animal ID, age, date of death) of the eight dead penguins examined from both zoos (Bioparco and Zoomarine), and positive for P. matutinum by PCR and molecular identification.
The Bioparco is one of the oldest zoological gardens in Europe, founded in 1,911. It is located in the city center of Rome (Italy), covers an area of 18 ha and houses about 1,000 specimens belonging to almost 200 species of mammals, birds and reptiles. Animals live in large spaces with reconstruction of the natural habitats suitable for each species. During the study period the zoo hosted an average population of S. demersus of about 20–25 animals.
Zoomarine is a “didactic” theme park (mainly focused on marine life) located in the town of Torvaianica (Rome, Italy) open to the public since 2005. It covers an area of 40 ha and houses about 350 animals of 36 different species (mammals, birds, and reptiles). During the study period the zoo hosted an average population of S. demersus of about 15 animals.
In both zoos conventional prophylaxis measures commonly established in zoos to protect the colonies from the risk of avian malaria (22), including vector control and periodic treatment of the animals with antimalarial drugs, were implemented.
During the study period, dead penguins were sent to the Istituto Zooprofilattico Sperimentale del Lazio e della Toscana “M. Aleandri” (IZSLT) to ascertain the causes of death.
Pathology and Histopathology
On all eight animals a complete necropsy was performed. Samples of multiple organs were collected and fixed in 10% neutral buffered formalin for histopathological examination. For biomolecular analyses, liver and spleen were regularly collected and tested, as they represent the target organs for the diagnosis of avian malaria, but for samples IDs Z1 and Z2 for which brain and lungs were tested instead of the spleen, respectively (Table 1).
Whenever considered pertinent, samples for additional virological and bacteriological analyses were also collected (data not shown).
Mosquitoes Collection
In the period September-October 2019, mosquitoes samplings were carried out in both zoos where penguin mortalities occurred. Adult mosquitoes were sampled using traps of the model Italian Mosquito Trap (IMT) (a modified CDC light trap with an insulated bucket holding the dry ice, hence the CO2 is dispensed from above) lured with 1 kg of dry ice (CO2) and Gravid Traps lured with a mixture of water and hay soiled with guinea pig feces and urine. Traps were located as close as possible to the penguin enclosures. For each sampling session, three IMT traps and one Gravid trap run from late afternoon to 8:30–10:00 of the following morning. A total of five sampling sessions at Bioparco and six at Zoomarine were performed. Noteworthy, at Bioparco, in order to try to protect penguins from mosquitoes, the animal health managers had placed in their enclosure two traps model BG-Mosquitaire (Biogents) baited with CO2 supplied with a gas cylinder and continuously operating. During each sampling session, mosquitoes caught by these traps were also collected.
Considering the different traps used during each sampling session, 21 and 18 catches were performed at Bioparco and Zoomarine, respectively, comprehensive of the collections of the two BG-Mosquitaire.
Mosquitoes were morphologically identified at the laboratory of Parasitology and Entomology- General Diagnostic Department of IZSLT, following the identification keys by Severini et al. (49). During the sorting and identification process, all mosquitoes were checked for the presence of blood in the abdomen. After identification, whole mosquito females were pooled in groups of about 30–50 specimens (for a total of 38 pools) and transferred for molecular analyses (Table 2).
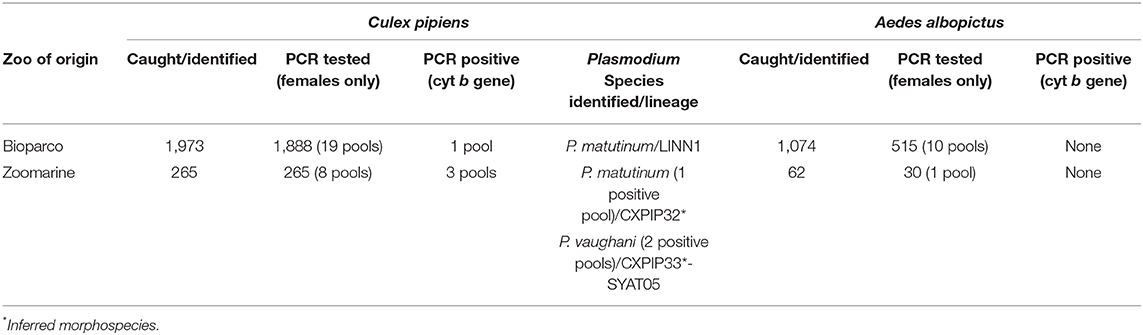
Table 2. Number of mosquitoes caught/identified at both zoos (Bioparco and Zoomarine) and tested in pools by PCR for the presence of Plasmodium spp.
Plasmodium Molecular Identification and Characterization
Total DNA was extracted from the above-mentioned penguin organs and from pooled mosquitoes. Before extraction, the mosquito pools were placed in tubes containing beads and mechanically homogenized using the Tissuelyser (Qiagen) at 30 Hz for 3 min, followed by a centrifugation at 17 g for 10 min.
From both organs and mosquitoes pools, DNA extraction was performed using an automated system (QIAsymphony SP Qiagen) with the DSP Virus/Pathogen Mini Kit, following the manufacturer's instructions.
Extracted DNA was subjected to a simplex PCR assay for the detection of the three haemosporidian genera (Plasmodium, Haemoproteus and Leucocytozoon), using as a target the partial mitochondrial conserved region of the cytochrome b gene (cyt b). The PCR was performed with the primers HaemNFI (5′-catatattaagagaaitatggag-3′) and HaemNR3 (5′-atagaaagataagaaataccattc-3′) described by Hellgren et al. (50), using the following protocol: Master Mix was prepared with 5 μL of distilled water, 12.5 μL of Platinum™ Hot Start PCR Master Mix 2X (Invitrogen), 1.25 μL of each M13-tailed primer (10 pMol/ μL), with a final volume of 20 μL, then 5 μL of the template was added.
PCR started with an initial denaturation step of 3 min at 95°C, followed by 35 cycles of 15 s at 95°C, 15 s at 50°C, 5 s at 72°C, and a final extension at 72°C for 3 min. Negative and positive controls for both DNA extraction and PCR reaction processes were also included. For sequencing, positive PCR-products (570 bp) were purified using an enzymatic cleanup ExoSAP-IT™ kit. Amplicons were Sanger sequenced on a 3,500 Series Genetic Analyzer with BigDye Terminator chemistry (Applied Biosystems, USA) using the same primers. Sequence data analysis and trimming was performed using the CLC DNA workbench® software version 5.7.1. The resulting sequences were examined for the presence of overlapping peaks to exclude potential co-infections and compared using BLAST (online version1, blastn algorithm) (51). The cyt b sequences were also compared for similarity to sequences available at the MalAvi database2.
For further characterization, the multiple sequence alignment (MSA) of the Sanger sequences was performed by using MUSCLE algorithm with default settings (53).
For the phylogenetic analysis, all the cyt b sequences obtained from mosquito pools were included, while to avoid clutter of the tree, only one cyt b sequence (when identical among organs) from each dead penguin was included. In addition, three Plasmodium sequences publicly available (54) were used as references (P. matutinum AFTRU5, GenBank MK652236; P. matutinum LINN1, GenBank MK652235; P. vaughani SYAT05, GenBank MK652243). All Plasmodium cyt b sequences previously reported in the phylogenetic tree of Valkiūnas et al. (5), except for P. juxtanucleare GALLUS03, which showed low quality, were also included. Finally, P. relictum ATCC 30141, GenBank AY099032.1 and P. lutzi TFUS05 (6) sequences were included. Leucocytozoon schoutedeni GALLUS06 was used as outgroup.
The length of the final alignment used to build the phylogenetic tree was 454 bp This length refers to the smallest cyt b sequence obtained following alignment. The alignment file and the p-distance matrix calculated with R are provided as Supplementary Data Sheets 1, 2, respectively.
All the sequences obtained in this study and used for the phylogenetic analyses were submitted to the European Nucleotide Archive (ENA)3 under the study accession number PRJEB41796.
A comparative genomics analysis of the MSA, with Bayesian inference of phylogeny, was performed by using MrBayes version 3.2 (55). The General Time Reversible Model including invariable sites and variation among sites (GTR + I + G) was used. Two independent runs were performed. Each analysis was run for a total of five million generations with a sampling frequency of every 100 generations. Before constructing a majority-rule consensus tree, 25% of the initial trees in each run were discarded as “burn in” periods.
Results
Pathology and Histopathology
At necropsy, gross lesions were almost similar in all the eight penguins examined, regardless of the origin (Bioparco or Zoomarine). The livers were generally moderately megalic, with pale and degenerated parenchyma, and the spleen were severely enlarged, in some cases up to six times the normal size. Lungs were severely affected, either congested or oedematous, or both. In almost all cases (7 out of 8), a severe serous pericardial effusion was observed. Other remarkable lesions were hyperaemia of the intestinal serosa (4/8), red discolouration of the cerebral parenchyma (3/8) and nephromegaly (3/8). In one case, multiple white thickened plaques were observed on the air sacs.
Inflammatory and degenerative lesions were microscopically observed in multiple organs, in particular in the liver, lungs and brain. A moderate to severe lymphocytic periportal hepatitis was regularly observed, with pronounced haemosiderosis and multifocal necrosis in few cases. Lungs were diffusely affected, severely congested with multifocal hemorrhages and a mild to moderate inflammatory lymphocytic infiltrate; small multifocal foci of necrosis were observed in three cases. In the spleen, a moderate lymphoreticular hyperplasia was evident in all cases and necrosis was observed in two animals. In the brain, the most evident changes were congestion and perivascular oedema. In all the examined organs, numerous 20 to 80 μm in diameter round to elongate structures (schizonts), filled with uncountable 1−3 μm in size intensely basophilic nuclei (merozoites), were observed inside the cytoplasm of endothelial or reticuloendothelial cells (intracellular exoerythrocytic stage), suggestive of the presence of Plasmodium spp. (Supplementary Figure 1); the resulting obstruction of the lumen of the capillaries was particularly evident in brain, kidney and the lung tissue sections. A summary of the main necropsy and histopathological findings are reported in Table 1.
Gross and histopathological lesions, together with the presence of merozoites and absence of other evident causes of death (data not shown), confirmed the diagnosis of avian malaria.
Mosquitoes Collection
In both zoos, Cx. pipiens was the dominant species (1,973 specimens at Bioparco, 265 at Zoomarine), followed by Ae. albopictus (1,074 specimens at Bioparco, 62 at Zoomarine). Other minor species identified were Culiseta longiareolata at Bioparco (five specimens), and Ae. vexans at Zoomarine (six specimens). These latter minority species were not tested by PCR for the presence of Haemosporida.
As expected, the working continuously BG-Mosquitaire traps gave the highest catch rate.
The number of mosquitoes identified, as well as the number of female mosquitoes tested (in pool) by PCR for the presence of avian haemosporidian protozoa (Plasmodium, Haemoproteus and Leucocytozoon) are reported in Table 2. None of the PCR tested mosquitoes resulted even partially engorged of blood in the abdomen. Detailed information about the number of mosquitoes of each species collected in each sampling session (for each type of trap), is reported in Supplementary Table 1.
Plasmodium Identification and Characterization
All the analyzed organs (n = 16) of the eight dead penguins from both zoos tested PCR positive (Supplementary Figure 2). Blast analysis demonstrated a nucleotide identity with P. matutinum for all the obtained penguin sequences. As for the mosquito pools, four Cx. pipiens pools tested PCR positive, while the Ae. albopictus pools tested all negative. Sequence analysis of Cx. pipiens pools demonstrated the presence of two Plasmodium species detected at Zoomarine, namely P. vaughani (two positive pools) and P. matutinum (one positive pool), while at Bioparco only P. matutinum was detected (Table 2). Assuming that in each positive pool just one mosquito was positive for Plasmodium spp., the estimated minimum infection rate (MIR) at Bioparco was 0.05% (1/1,888), while at Zoomarine 1.13% (3/265). Specifically at Zoomarine, P. vaughani MIR was 0.75% (2/265), while P. matutinum MIR was 0.38% (1/265).
Analyzing the chromatograms, no evidence of double peaks (suggestive of mixed infections) was detected in any of the sequences. Overall, eight P. matutinum sequences selected from the dead penguins (one for each penguin), and all the four sequences from the positive Cx. pipiens pools (two identified as P. matutinum and two as P. vaughani) were included in the phylogenetic analysis.
From the obtained tree it can be inferred that all but one of the P. matutinum sequences detected in this study were identical to two previously characterized and closely related lineages (LINN1 and AFTRU5, Figure 1). In a Cx. pipiens pool collected at Zoomarine (ID IT01M19) we also identified one novel lineage, not 100% identical to LINN1 (p-distance = 0.0022, Supplementary Data Sheet 2). LINN1 and AFTRU5 were found to be associated to penguin deaths, although only LINN1 was detected both in penguins (along the years of the study) and in Cx. pipiens, while AFTRU5 was detected in a single penguin dead in 2017 (ID IT07P17). One of the two P. vaughani sequences (IT03M19) obtained from Cx. pipiens pools collected at Zoomarine was identical to a previously characterized lineage (SYAT05), while the other (ID IT02M19), was slightly different (p-distance = 0.0044, Supplementary Data Sheet 2).
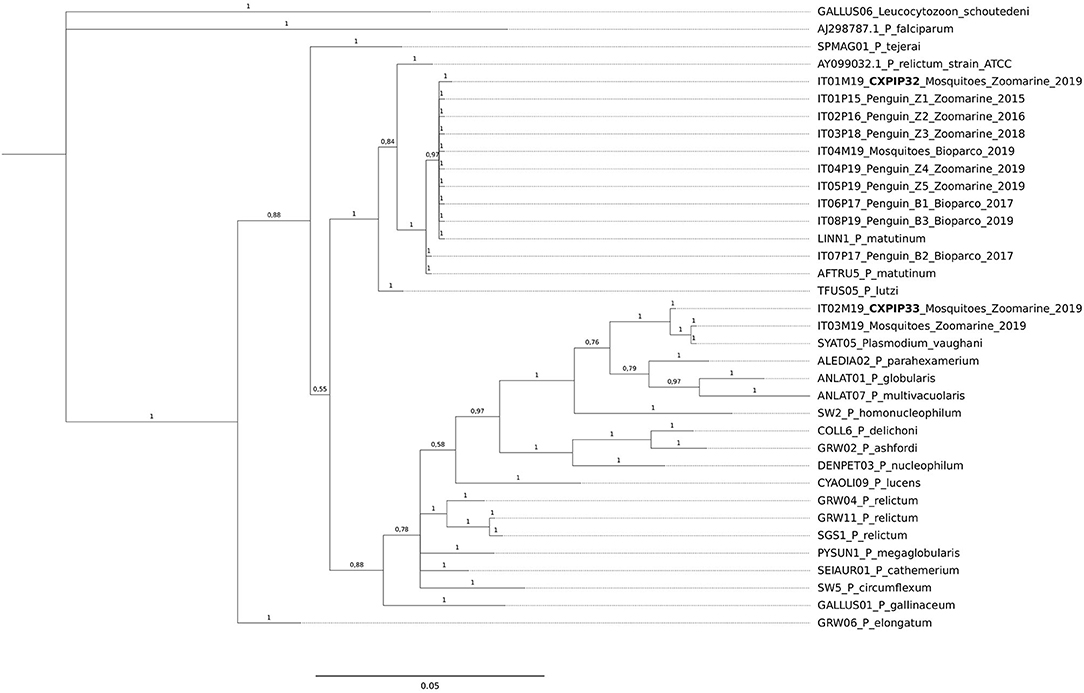
Figure 1. Bayesian tree of n = 36 mitochondrial cyt b sequences of Plasmodium species, including 12 Plasmodium sequences obtained in this study from penguins and from mosquito pools, 23 reference lineages from MalAvi database and one ATCC from GenBank. Branch lengths are drawn proportionally to the extent of changes (scale bar is shown). Values adjacent to nodes represent posterior probabilities. IDs of the sequences obtained from penguins and mosquito pools in relation to the animal IDs, location and year of death/trapping, are reported. In bold the two newly identified MalAvi lineages.
Sequences IT01M19 and IT02M19 obtained from Cx. pipiens were assigned the MalAvi lineage names CXPIP32 and CXPIP33, respectively; phylogenetic analysis suggests they correspond to P. matutinum and P. vaughani, respectively.
Discussion
We used a multidisciplinary approach to investigate the death due to avian malaria in eight African penguins (S. demersus) kept in two Italian zoos, thus providing new information regarding the pathology, molecular identification and phylogenetic characterization of a Plasmodium (P. matutinum) causing infection in these captive birds. We also provided information about the presence and circulation of avian Plasmodium species in mosquitoes from Central Italy.
Gross pathological and histopathological findings detected in the dead penguins were consistent with those previously observed by other authors (22, 26, 29, 37, 38). Lesions in all the animals were acute to peracute, with massive diffusion of the parasites in tissues macrophages, mainly in liver and spleen and representing an exoerythrocytic pathway of infection, with endothelial involvement in more advanced cases. This pathway has already been described and in penguins it is considered the most frequent way of replication of Plasmodium spp. (1, 29, 37, 38).
Regarding the vectors, our results are in agreement with previous studies and provide strong evidence that Cx. pipiens is one of the main vectors of Plasmodium spp. associated with avian malaria in Europe and the Northern Hemisphere (12, 15, 20). Indeed, this species has been already reported as responsible for avian malaria transmission to captive penguins in zoos (1, 15, 56). Importantly, in our study avian haemosporidia were not found in Ae. albopictus. The role of this latter species as possible vector of avian malaria is quite controversial. Some authors reported the detection of these parasites in Ae. albopictus, even though with low prevalence values (21, 57), some others reported the absence of Plasmodium spp. in this mosquito species (58, 59). Beyond these findings, it is important to remind that Ae. albopictus has a marked preference for mammals (15, 60, 61), hence it is unlikely that this species will play an epidemiologically relevant role in the transmission of avian malaria.
Regarding the Plasmodium spp. MIR values in Cx. pipiens, when compared to data reported in other studies, our estimates appear quite low. In fact, the overall MIR at Zoomarine was 1.13%, similar to the total MIR (0.99%) reported in the same mosquito species by Schoener et al. (4) in a three-year study in Eastern Austria, but significantly lower than the maximum MIR (5.26%). Even more marked are the differences when comparing our findings with those described by Lalubin et al. (20) and Martínez-de la Puente et al. (21) reporting prevalence values of 15.4% and 30% in Cx. pipiens in Switzerland and North-Eastern Italy, respectively. Our results are of some concern, since even with a very low MIR, it seems that both transmission and disease can occur causing mortality in captive penguins. Nevertheless, it should be taken into account that our investigation on mosquitoes was not specifically aimed at the definition of prevalence estimates, and these results need to be confirmed and further investigated.
Regarding the higher number of dead penguins and positive mosquito pools recorded at Zoomarine compared to Bioparco, we cannot rule out that differences of the habitat, wild bird community and/or management (e.g., measures put in place to limit mosquito exposure), might have played an important epidemiological role. The greater habitat heterogeneity in the surroundings of Zoomarine (e.g., cultivated fields, streams, proximity to sea and to two Natural Parks) may have positively influenced key community parameters (e.g. species richness, abundance and distribution), increasing the frequency of vector-host contacts (62, 63). Indeed, the two sites significantly differed in environmental features related with suitable mosquito breeding habitats. For instance, a stream in the proximity of the penguin enclosure at Zoomarine, where most of the mosquito collections were performed, has been recognized as the most probable larval development site. Nevertheless, being the stream under public ownership, the water could not be treated with larvicids, as instead it is usually done at Bioparco for standing waters close to the enclosures. Given the tendency of Cx. pipiens to feed on avian hosts (64), the water stream, providing habitat for both vectors and birds, may have increased the frequency of reservoir-vector-penguin contacts.
On the genomic side, we detected three previously characterized lineages (LINN1, AFTRU5, SYAT05) and identified two novel lineages in mosquitoes, CXPIP32 (inferred morphospecies P. matutinum) and CXPIP33 (inferred morphospecies P. vaughani). Interestingly, LINN1 and AFTRU5 were found to be associated to penguin deaths, although only LINN1 was detected both in penguins (along the years of the study, in both zoos) and in Cx. pipiens, while AFTRU5 was detected in a single penguin dead in 2017 at Zoomarine.
On the other hand, SYAT05, CXPIP32, and CXPIP33 were detected only in Cx. pipiens. SYAT05 is considered a common generalist avian malaria parasite (2, 7), but so far in Italy it has been previously detected only in Cx. pipiens samples collected in the north-east (21) and in skylark (Alauda arvensis) in southern Italy (47).
P. matutinum and related lineages have already been associated with mortality in penguins and other birds. Sijbranda et al. (37) analyzing archived tissues using a nested PCR for Plasmodium spp. followed by DNA sequencing, revealed that two wild little penguins (Eudyptula minor) found dead on New Zealand beaches were infected with lineage LINN1. Vanstreels et al. (6) investigating an outbreak of avian malaria in Magellanic penguins (Spheniscus magellanicus) at a rehabilitation center in southeast Brazil identified a Plasmodium sp. PHPAT01 infection in a penguin with post-mortem lesions suggestive of avian malaria. This lineage was already determined to have caused the death of a Magellanic penguin in a previous study (27). The morphospecies of PHPAT01 has yet to be determined, but the authors suggest it is most closely related to P. lutzi and P. matutinum (6). Dinhopl et al. (65) reported that partial cyt b sequences deposited as lineages AFTRU5 or LINN1 and tentatively attributed to the species P. lutzi, showed homology to two previously obtained sequences from two captive penguins kept in the Vienna zoo. Finally Spottiswoode et al. (9), in a study conducted in captive penguins (Sphenisciformes spp.), eiders (Somateria spp.), and inca terns (Larosterna inca) in a North American zoological collection, reported P. matutinum LINN1 in several necropsied terns, but only in a healthy penguin subjected to surveillance. Our results would confirm the pathogenicity of P. matutinum (LINN1 and AFTRU5) in penguins; interestingly we detected this same species causing mortalities in two different zoos situated about 30 km apart and through several years. To note that Corradetti et al. (66) already reported the presence of P. matutinum in a wild bird in Italy (redwing, Turdus iliacus), although in this case the identification at species level was only phenotypic (microscopic examination). P. matutinum has also been previously reported as most closely related to P. tejerai (cyt b lineage SPMAG01), a common malaria parasite of American birds, based on partial cyt b sequences and on phenotypic characteristics (5). Indeed, P. tejerai is considered highly lethal for Spheniscus magellanicus, a species closely related to African penguin, with 75% (12/16) case-fatality rate among individuals found infected at rehabilitation centers in Brazil (27).
Avian malaria is a well-known and relevant problem for penguins kept at zoos or rehabilitation centers worldwide (1, 22, 26, 27). The susceptibility to the disease caused by Plasmodium in penguin species is usually interpreted in the light of a poor parasite adaptation of the hosts, due to their naïve status in their original area of distribution (19, 22). In these situations, without any kind of prophylactic and protective measure, there is a high risk of registering continuous clinical cases and deaths in colonies. In this scenario, both at Bioparco and Zoomarine, preventive measures are adopted, in particular during the seasons when adult mosquitoes are active. Specifically, at Bioparco, strategies aiming at reducing the number of mosquitoes around the penguins using different approaches are adopted. These strategies are: the use of traps (BG-Mosquitaire), the use of powerful fans able to create a strong air flow in the area of the enclosure where animals use to spend the night, the use of water vents in areas not reached by the air fans, the spraying of the nests surroundings with neem oil and the treating of standing waters with larvicides (67). Furthermore, both at Bioparco and Zoomarine, according to renowned protocols (22), penguins are periodically treated with chloroquine phosphate and at Zoomarine also with primaquine. These measures surely limited the impact of avian malaria on the two colonies, in particular at Bioparco, even though they were not sufficient to fully prevent the occurrence of clinical cases and deaths.
Avian malaria, in Italy, is poorly known and investigated. Very few studies in the past focused on haemosporidian infections, due to the perceived limited clinical relevance of these parasites, usually quietly coexisting with their natural hosts. Indeed, silent or subclinical infections are frequent in many avian vertebrate hosts, possibly imposing fitness costs based on trade-off mechanisms, ranging from decreased survival to decreased fecundity in certain species (68). The presence of two colonies of African penguin brought to the light something that otherwise could have gone unnoticed. Interestingly, the deaths occurred in 2017 in the penguin colony of the Bioparco of Rome (n = 2 animals, IDs B1 and B2, Table 1) occurred immediately after the translocation of these animals from a zoo located in Northern Italy. The B1 subject was also the only dead penguin in which a P. matutinum AFTRU5 lineage was detected, a lineage not found in the collected mosquitoes. Remarkably, AFTRU05 has been already recorded in mosquitoes in northern Italy (21). Hence, it cannot be ruled out that the two birds, or at least B1, arrived in Rome already infected, and that the stress of the translocation might have favored a reactivation of the disease. Indeed, this kind of stress is a well-known risk factor for developing clinical signs of avian malaria in captive birds (22) and should be carefully considered.
Zoos maintaining captive penguins in temperate areas where Cx. pipiens is abundant should be well aware of the risks of avian malaria, and should put every effort to prevent outbreaks, in particular during the periods when the number of vectors is higher.
Data Availability Statement
The datasets presented in this study can be found in online repositories. The names of the repository/repositories and accession number(s) can be found in the article/Supplementary Material.
Author Contributions
MI, FR, CD, CC, KF, FM, and AB: conceived and designed the experiments. MI, FR, CC, FS, AM, RO, and AB: performed the experiments. ED, MI, PA, VC, AC, CC, CD, and AB: analyzed the data. MI, CC, ED, FR, VC, AC, CD, and AB: wrote the paper.
Conflict of Interest
The authors declare that the research was conducted in the absence of any commercial or financial relationships that could be construed as a potential conflict of interest.
Acknowledgments
We would like to thank Dr. Pilar Di Cerbo from Bioparco and Dr. Annalisa Duri from Zoomarine for their technical assistance.
Supplementary Material
The Supplementary Material for this article can be found online at: https://www.frontiersin.org/articles/10.3389/fvets.2021.621974/full#supplementary-material
Supplementary Figure 1. Histopathological pictures (Hematoxylin-eosin). (a) Spheniscus demersus, liver. Moderate lymphocytic periportal hepatitis with intralesional merozoites (arrows). (b) Spheniscus demersus, brain. Intra-endothelial merozoites obstructing the lumen of the brain capillary (arrow).
Supplementary Figure 2. Gel image of the PCR results of the cyt b target obtained from the automated capillary electrophoresis high-resolution system QIAxcel System® (Qiagen), from left to right: Lane 1: Size Marker (QX DNA Size Marker 100 bp−2.5 kb). Lanes 2-17: cyt b amplicon from dead penguins (lane 11, weak positive sample). Lanes 18-21: cyt b amplicon from mosquito pools. Lane 22: Negative control (Nuclease-Free Water). Lane 23: Positive control (Plasmodium spp. DNA, IZSLT collection).
Supplementary Data Sheet 1. Alignment of the 454 bp cyt b sequences used to build the phylogenetic tree.
Supplementary Data Sheet 2. p-distance matrix of the 454 bp cyt b sequences used to build the phylogenetic tree, calculated with R.
Supplementary Table 1. Detailed information about the number of mosquitoes of each species collected in each sampling session, for each type of trap, with the results of molecular identification and MalAvi lineage.
Footnotes
References
1. Vanstreels RE, Braga E, Catao-Dias J. Blood parasites of penguins: a critical review. Parasitology. (2016) 143:931–56. doi: 10.1017/S0031182016000251
2. Harl J, Himmel T, Valkiūnas G, Ilgunas M, Bakonyi T, Weissenböck H. Geographic and host distribution of haemosporidian parasite lineages from birds of the family Turdidae. Malar J. (2020) 19:335. doi: 10.1186/s12936-020-03408-0
3. Bennett G, Bishop M, Peirce M. Checklist of the avian species of Plasmodium Marchiafava & Celli, 1885 (Apicomplexa) and their distribution by avian family and Wallacean life zones. Syst Parasitol. (1993) 26:171–9. doi: 10.1007/BF00009724
4. Schoener E, Uebleis S, Butter J, Nawratil M, Cuk C, Flechl E, et al. Avian Plasmodium in Eastern Austrian mosquitoes. Malar. J. (2017) 16:389–401. doi: 10.1186/s12936-017-2035-1
5. Valkiūnas G, Ilgunas M, Bukauskaite D, Palinauskas V, Bernotiene R, Iezhova T. Molecular characterization and distribution of Plasmodium matutinum, a common avian malaria parasite. Parasitology. (2017) 144:1726–35. doi: 10.1017/S0031182017000737
6. Vanstreels RE, Dutra D, Ferreira-Junior F, Hurtado R, Egert L, Mayorga LFS, et al. Epidemiology, hematology, and unusual morphological characteristics of Plasmodium during an avian malaria outbreak in penguins in Brazil. Parasitol Res. (2019) 118:3497–508. doi: 10.1007/s00436-019-06459-8
7. Nourani L, Zakeri S, Dinparast Djadid N. Dynamics of prevalence and distribution pattern of avian Plasmodium species and its vectors in diverse zoogeographical areas - A review. Infect Genet Evol. (2020) 8:1042–44. doi: 10.1016/j.meegid.2020.104244
8. Valkiūnas G, Zehtindjiev P, Dimitrov D, KriŽanauskiene A, Iezhova Bensch T S. Polymerase chain reaction-based identification of Plasmodium (Huffia) elongatum, with remarks on species identity of haemosporidian lineages deposited in GenBank. Parasitol Res. (2008) 102:1185–93. doi: 10.1007/s00436-008-0892-9
9. Spottiswoode N, Bartlett S. Conley K, Seimon T, Griffin D, Sykes J, 4th. Analysis of Plasmodium lineages identified in captive penguins (Sphenisciformes spp.), eiders (Somateria spp.), and inca terns (Larosterna inca) in a North American zoological collection. J Zoo Wildl Med. (2020) 51:140–9. doi: 10.1638/2019-0078
10. Valkiūnas G. Avian Malaria Parasites and Other Haemosporidia. Florida: CRC press. (2005) doi: 10.1201/9780203643792
11. Glaizot O, Fumagalli L, Iritano K, Lalubin F, Van Rooyen J, Christe P. High prevalence lineage diversity of avian malaria in wild populations of great tits (Parus major) mosquitoes (Culex pipiens). PLoS ONE. (2012) 7:e34964. doi: 10.1371/journal.pone.0034964
12. Ventim R, Ramos J, Osorio H, Lopes R, Pérez-Tris J, Mendes L. Avian malaria infections in western European mosquitoes. Parasitol Res. (2012) 111:637–45. doi: 10.1007/s00436-012-2880-3
13. Ferraguti M, Martínez-de la Puente J, Muñoz J, Roiz D, Ruiz S, Soriguer R, et al. Avian Plasmodium in Culex and Ochlerotatus mosquitoes from Southern Spain: effects of season and host-feeding source on parasite dynamics. PLoS ONE. (2013) 8:e66237. doi: 10.1371/journal.pone.0066237
14. Ziegyte R, Bernotiene R, Bukauskaite D, Palinauskas V, Iezhova T, Valkiūnas G. Complete sporogony of Plasmodium relictum (lineages pSGS1 and pGRW11) in mosquito Culex pipiens pipiens form molestus, with implications to avian malaria epidemiology. J Parasitol. (2014) 100:878–82. doi: 10.1645/13-469.1
15. Martínez-de la Puente J, Soriguer R, Senar J, Figuerola J, Bueno-Mari R, Montalvo T. Mosquitoes in an urban zoo: identification of blood meals, flight distances of engorged females, and avian malaria infections. Front Vet Sci. (2020) 7:460. doi: 10.3389/fvets.2020.00460
16. Corradetti A, Morcos Neri W I. Sulle produzione di forme endoistiocitare nei canarini infettati con il ceppo italiano di Plasmodium matutinum derivato da Turdus iliacus. Parassitologia. (1962) 4:105–108
17. Dinhopl N, Mostegl M, Richter B, Nedorost N, Maderner A, Fragner K, et al. Application of in-situ hybridization for the detection and identification of avian malaria parasites in paraffin wax-embedded tissues from captive penguins. Avian Pathol. (2011) 40:315–20. doi: 10.1080/03079457.2011.569533
18. Valkiūnas, Ilgunas GM. Keys to the avian malaria parasites. Malar J. (2018) 17:212. doi: 10.1186/s12936-018-2359-5
19. LaPointe D, Atkinson C, Samuel M. Ecology and conservation biology of avian malaria. Ann N Y Acad Sci. (2012) 1249:211–26. doi: 10.1111/j.1749-6632.2011.06431.x
20. Lalubin F, Delédevant A, Glaizot O, Christe P. Temporal changes in mosquito abundance (Culex pipiens), avian malaria prevalence and lineage composition. Parasites Vectors. (2013) 6:307–14. doi: 10.1186/1756-3305-6-307
21. Martínez-de la Puente J, Muñoz J, Capelli G, Montarsi F, Soriguer R, Arnoldi D, et al. Avian malaria parasites in the last supper: identifying encounters between parasites and the invasive Asian mosquito tiger and native mosquito species in Italy. Malaria J. (2015) 14:32–38. doi: 10.1186/s12936-015-0571-0
22. Grilo M, Vanstreels RE, Wallace R, García-Párraga D, Braga E, Chitty J, et al. Malaria in penguins – current perceptions. Avian Pathol. (2016) 45:393–407. doi: 10.1080/03079457.2016.1149145
23. Brossy J. Malaria in wild and captive Jackass penguins Spheniscus demersus along the southern African coast. Ostrich. (1992) 63:10–2. doi: 10.1080/00306525.1992.9634174
24. Grim K C, van der Merwe E, Sullivan M, Parsons N, McCutchan Cranfield T M. Plasmodium juxtanucleare associated with mortality in black-footed penguins (Spheniscus demersus) admitted to a rehabilitation center. J Zoo Wildl Med. (2003) 34:250–5. doi: 10.1638/02-070
25. Silveira P, Belo N, Lacorte G, Kolesnikovas CK, Vanstreels RE, Steindel M, et al. Parasitological and new molecular-phylogenetic characterization of the malaria parasite Plasmodium tejerai in South American penguins. Parasitol Int. (2013) 62:165–71. doi: 10.1016/j.parint.2012.12.004
26. Vanstreels RE, Kolesnikovas CK, Sandri S, Silveira P, Belo N, Ferreira Junior F, et al. Outbreak of avian malaria associated to multiple species of Plasmodium in Magellanic Penguins undergoing rehabilitation in Southern Brazil. PLoS ONE. (2014) 9:e94994. doi: 10.1371/journal.pone.0094994
27. Vanstreels RE, Silva-Filho R, Kolesnikovas CK, Bhering RC, Ruoppolo V, Epiphanio S, et al. Epidemiology and pathology of avian malaria in penguins undergoing rehabilitation in Brazil. Vet Res. (2015) 46:30. doi: 10.1186/s13567-015-0160-9
28. Fleischman R, Squire R, Sladen WL, Melby E. Malaria (Plasmodium elongatum) in captive African penguins (Spheniscus demersus). J Am Vet Med Assoc. (1968) 153:928–35.
29. Stoskopf M, Beier J. Avian malaria in African Black-Footed Penguins. J Am Vet Med Assoc. (1979) 175:994–7.
30. Fix A, Waterhouse C, Greiner E. Stoskopf M. Plasmodium relictum as a cause of avian malaria in wild-caught Magellanic penguins (Spheniscus magellanicus). J Wildl Dis. (1988) 24:610–9. doi: 10.7589/0090-3558-24.4.610
31. Cranfield M, Graczyk T, Beall F, Ialeggio D, Shaw M, Skjoldager M. Subclinical avian malaria infections in African black-footed penguins (Spheniscus demersus) and induction of parasite recrudescence. J Wildl Dis. (1994) 30:372–6. doi: 10.7589/0090-3558-30.3.372
32. Graczyk T, Cranfield M, Brossy J, Cockrem J, Jouventin P, Seddon P. Detection of avian malaria infections in wild and captive penguins. J Helm Soc Wash. (1995) 62:135–41.
33. Ko K, Kang S, Jung J, Bae J, Kim J. Avian malaria associated with Plasmodium spp. infection in a penguin in Jeju Island Korean. J Vet Res. (2008) 48:197–201.
34. Bueno M, Lopez RP, Menezes RM, CostaNascimento M, Lima GFM, Araújo RA, et al. Identification of Plasmodium relictum causing mortality in penguins (Spheniscus magellanicus) from São Paulo Zoo, Brazil. Vet Parasitol. (2010) 173:123–7. doi: 10.1016/j.vetpar.2010.06.026
35. Leclerc A, Chavatte J-, Landau I, Snounou G, Petit T. Morphologic and molecular study of hemoparasites in wild corvids and evidence of sequence identity with Plasmodium DNA detected in captive black-footed penguins (Spheniscus demersus). J Zoo Wildl Med. (2014) 45:577–88. doi: 10.1638/2013-0257R.1
36. Vanstreels RE, Capellino F, Silveira P, Braga E, Rodríguez-Heredia S, Loureiro J, et al. Avian malaria (Plasmodium spp.) in captive Magellanic Penguins (Spheniscus magellanicus) from northern Argentina, 2010. J Wildl Dis. (2016) 52:734–7. doi: 10.7589/2015-08-219
37. Sijbranda D, Hunter S, Howe L, Lenting B, Argilla L, Gartrell B. Cases of mortality in little penguins (Eudyptula minor) in New Zealand associated with avian malaria. N Z Vet J. (2017) 65:332–7. doi: 10.1080/00480169.2017.1359124
38. Taunde P, Bianchi M, Perles L, da Silva F, Guim T, Stadler R, et al. Pathological and molecular characterization of avian malaria in captive Magellanic penguins (Spheniscus magellanicus) in South America. Parasitol Res. (2019) 118:599–606. doi: 10.1007/s00436-018-6155-5
39. Scott H. Report on the deaths occurring in the Society's Gardens during the year 1926. Proc Zool Soc Lond. (1927) 97:173–98. doi: 10.1111/j.1096-3642.1927.tb02254.x
40. Rodhain J. L'infection a Plasmodium relictum chez les pingouins (in French). Ann Parasitol Hum Comp. (1939) 17:139–57. doi: 10.1051/parasite/1939-1940172139
41. Fantham H, Porter A. On a Plasmodium (Plasmodium relictum var. spheniscidae, n var), observed in four species of penguins. Proc Zool Soc Lond. (1944) 114:279–92. doi: 10.1111/j.1096-3642.1944.tb00222.x
42. Rewell R. Report of the pathologist for the year 1947. Proc Zool Soc Lond. (1948) 118:501–14. doi: 10.1111/j.1096-3642.1948.tb00390.x
43. Rodhain J, Andrianne V. Deux nouveaux cas d'infestation par Plasmodium chez des pingouins (in French). Anna Parasitol Hum Comp. (1952) 32:573–7. doi: 10.1051/parasite/1952276573
44. Grünberg W, Kutzer E. Infektionen mit Plasmodium praecox bei Humboldt- (Spheniscus humboldti) und Brillenpinguinen (Spheniscus magellanicus) (in German). Zentralblatt Bakteriol Parasitenkunde Infektionskrankheiten Hygiene. (1963) 189:511–20.
45. Cereghetti N, Wenker C, Hoby S, Müller P, Marti H, Lengeler C. Avian malaria and its prevention strategies in the Zoo Basel. In: Swiss Society for Hospital Hygiene, Swiss Society for Microbiology and Swiss Society for Tropical Medicine and Parasitology, St. Gallen, Switzerland. Proceedings of the Joint Annual Meeting of the Swiss Society for Infectious Diseases. (2012). p. 169.
46. Corradetti A, Verolini F, Neri I. Plasmodium (Haemamoeba) giovannolai n. sp parassita di Turdus merula. Parassitologia. (1963) 5:11–8.
47. Zehtindjiev P, KriŽanauskiene A, Scebba S, Dimitrov D, Valkiūnas G, Hagemann. A., et al. Haemosporidian infections in skylarks (Alauda arvensis): a comparative PCR-based and microscopy study on the parasite diversity and prevalence in southern Italy and the Netherlands. Eur J Wildl Res. (2012) 58:335–44. doi: 10.1007/s10344-011-0586-y
48. Ebani V, Nardoni S, Giani M, Rocchigiani G, Archin T, Altomonte I, et al. Molecular survey on the occurrence of avian haemosporidia, Coxiella burnetii and Francisella tularensis in waterfowl from central Italy. Int J Parasitol Parasites Wildl. (2019) 10:87–92. doi: 10.1016/j.ijppaw.2019.07.008
49. Severini F, Toma L, Di Luca M, Romi R. Le zanzare italiane: generalità e identificazione degli adulti (Diptera, Culicidae). Fragm Entomol. (2009) 41:213–372. doi: 10.4081/fe.2009.92
50. Hellgren O, Waldenström J, Bensch S. A new PCR assay for simultaneous studies of Leucocytozoon, Plasmodium, and Haemoproteus from avian blood. J Parasitol. (2004) 90:797–802. doi: 10.1645/GE-184R1
51. Kent W. BLAT—the BLAST–like alignment tool. Genome Res. (2002) 12:656–664. doi: 10.1101/gr.229202
52. Bensch S, Hellgren O. Perez-Tris J. MalAvi. A public database of malaria parasites and related haemosporidians in avian hosts based on mitochondrial cytochrome b lineages. Mol Ecol Res. (2009) 9:1353–8. doi: 10.1111/j.1755-0998.2009.02692.x
53. Edgar R. MUSCLE: multiple sequence alignment with high accuracy and high throughput. Nucleic Acids Res. (2004) 32:1792–7. doi: 10.1093/nar/gkh340
54. Harl J, Himmel T, Valkiūnas G, Weissenböck H. The nuclear 18S ribosomal DNAs of avian haemosporidian parasites. Malar J. (2019) 18:305. doi: 10.1186/s12936-019-2940-6
55. Huelsenbeck J, Ronquist F. MRBAYES: Bayesian inference of phylogenetic trees. Bioinformatics. (2001) 17:754–5. doi: 10.1093/bioinformatics/17.8.754
56. Ejiri H, Sato Y, Kim K, Hara T, Tsuda Y, Imura T, et al. Entomological study on transmission of avian malaria parasites in a zoological garden in Japan: blood meal identification and detection of avian malaria parasite DNA from bloodfed mosquitoes. J Med Entomol. (2011) 48:600–7. doi: 10.1603/ME10197
57. Ejiri H, Sato Y, Sasaki E, Sumiyama D, Tsuda Y, Sawabe K, et al. Detection of avian Plasmodium spp. DNA sequences from mosquitoes captured in Minami Daito Island of Japan. J Vet Med Sci. (2008) 70:1205–10. doi: 10.1292/jvms.70.1205
58. Kim K, Tsuda Y, Yamada A. Bloodmeal identification and detection of avian malaria parasite from mosquitoes (Diptera: Culicidae) inhabiting coastal areas of Tokyo Bay, Japan. J Med Entomol. (2009) 46:1230–4. doi: 10.1603/033.046.0535
59. Trout Fryxell R, Thompson Lewis T, Peace H, Hendricks Paulsen BD. Identification of avian malaria (Plasmodium sp.) and canine heartworm (Dirofilaria immitis) in the mosquitoes of Tennessee. J Parasitol. (2014) 100:455–62. doi: 10.1645/13-443.1
60. Sawabe K, Isawa H, Hoshino K, Sasaki T, Roychoudhury S, Higa Y, et al. Host-feeding habits of Culex pipiens and Aedes albopictus (Diptera: Culicidae) collected at the urban and suburban residential areas of Japan. J Med Entomol. (2010) 47:442–50. doi: 10.1603/ME09256
61. Egizi A, Healy S. Fonseca D. Rapid blood meal scoring in anthropophilic Aedes albopictus and application of PCR blocking to avoid pseudogenes. Infect Genet Evol. (2013) 16:122–8. doi: 10.1016/j.meegid.2013.01.008
62. Marzluff J. Worldwide urbanization and its effects on birds. In: Marzluff JM, Bowman R, Donnelly R, editors. An Urbanizing World. Boston: Kluwer Academic (2001). p. 331–364. doi: 10.1007/978-1-4615-1531-9_2
63. Ferenc M, Sedláček O, Fuchs R, Dinetti M, Fraissinet M, Storch D. Are cities different? Patterns of species richness and beta diversity of urban bird communities and regional species assemblages in Europe. Glob Ecol Biogeogr. (2013) 23:479–89. doi: 10.1111/geb.12130
64. González M, Prosser S, Hernández-Triana L, Alarcón-Elbal P, Goiri F, López S, et al. Avian feeding preferences of Culex pipiens and Culiseta spp. along an urban-to-wild gradient in northern Spain. Front Ecol Evol. (2020) 8:568835. doi: 10.3389/fevo.2020.568835
65. Dinhopl N, Nedorost N, Mostegl M, Weissenbacher-Lang C, Herbert Weissenböck H. In situ hybridization and sequence analysis reveal an association of Plasmodium spp. with mortalities in wild passerine birds in Austria. Parasitol Res. (2015) 114:1455–62. doi: 10.1007/s00436-015-4328-z
66. Corradetti A, Neri I, Scanga M. Segnalazione in Italia di Plasmodium praecox var. matutinum in Turdus iliacus, e separazione di questo plasmodio da Plasmodium praecox come specie distinta: Plasmodium matutinum Huff, 1937. Parassitologia. (1960) 2:333–43.
67. AZA Penguin Taxon Advisory Group. Association of Zoos and Aquariums, editors. Penguin (Spheniscidae) Care Manual. Silver Spring (2014).
Keywords: avian malaria, Plasmodium spp., Plasmodium matutinum, Plasmodium vaughani, mosquitoes, mortality, penguins, zoo
Citation: Iurescia M, Romiti F, Cocumelli C, Diaconu EL, Stravino F, Onorati R, Alba P, Friedrich KG, Maggi F, Magliano A, Ermenegildi A, Carfora V, Caprioli A, De Liberato C and Battisti A (2021) Plasmodium matutinum Transmitted by Culex pipiens as a Cause of Avian Malaria in Captive African Penguins (Spheniscus demersus) in Italy. Front. Vet. Sci. 8:621974. doi: 10.3389/fvets.2021.621974
Received: 28 October 2020; Accepted: 11 February 2021;
Published: 16 March 2021.
Edited by:
Armanda Bastos, University of Pretoria, South AfricaReviewed by:
Annelise Botes, Stellenbosch University, South AfricaRalph Vanstreels, Marine Animal Research and Rehabilitation Institute (IPRAM), Brazil
Josué Martínez-de la Puente, University of Granada, Spain
Copyright © 2021 Iurescia, Romiti, Cocumelli, Diaconu, Stravino, Onorati, Alba, Friedrich, Maggi, Magliano, Ermenegildi, Carfora, Caprioli, De Liberato and Battisti. This is an open-access article distributed under the terms of the Creative Commons Attribution License (CC BY). The use, distribution or reproduction in other forums is permitted, provided the original author(s) and the copyright owner(s) are credited and that the original publication in this journal is cited, in accordance with accepted academic practice. No use, distribution or reproduction is permitted which does not comply with these terms.
*Correspondence: Andrea Caprioli, andrea.caprioli@izslt.it