- 1Graduate School, Chiang Mai University, Chiang Mai, Thailand
- 2Bee Protection Laboratory, Department of Biology, Faculty of Science, Chiang Mai University, Chiang Mai, Thailand
- 3Key Laboratory of Pollinating Insect Biology of the Ministry of Agriculture, Institute of Apicultural Research, Chinese Academy of Agricultural Science, Beijing, China
- 4Environmental Science Research Center, Faculty of Science, Chiang Mai University, Chiang Mai, Thailand
Honeybee drones are male bees that mate with virgin queens during the mating flight, consequently transferring their genes to offspring. Therefore, the health of drones affects the overall fitness of the offspring and ultimately the survivability of the colony. Honeybee viruses are considered to be a major threat to the health of honeybees. In the present study, we demonstrated the pattern of common honeybee viruses in various tissues of drones in the western honeybee, Apis mellifera, and the eastern honeybee, Apis cerana. Drones were collected during the mating flight and analyzed using quantitative real-time (qRT-PCR) to detect the presence of seven honeybee viruses. The qRT-PCR result revealed that three honeybee viruses, namely Black Queen Cell Virus (BQCV), Deformed Wing Virus (DWV), and Chinese Sacbrood Virus (CSBV), were detected in the reproductive tissues of A. mellifera and A. cerana drones. The results from qRT-PCR showed that the Israeli Acute Paralysis Virus (IAPV) was only detected in A. mellifera drone body tissues. Moreover, the prevalence of DWV and BQCV in the drones collected from A. mellifera colonies was significantly higher than that of A. cerana. In addition, virus multiple infections were higher in A. mellifera drones compared to those in A. cerana. CSBV was found predominantly in the reproductive tissues of A. cerana drones. This study is the first report describing the presence of the CSBV in reproductive tissues of A. mellifera drones. Our results may reflect the preference of honeybee viruses in honeybee species and may provide a piece of interesting evidence for understanding the virus transmission in A. cerana.
Introduction
Apis species (the western honeybee, Apis mellifera, and the eastern honeybee, Apis cerana) are economically valuable pollinators of agricultural crops and are also a source of honeybee products such as honey, pollen, royal jelly, wax, venom, and their larvae (1, 2). The recent loss of honeybee colonies and reduced population of native and wild bees as a result of various stressors, including pathogens, pesticides, habitat loss, and lack of nutritional resources, are global concerns (3, 4). In particular, pathogens and parasites that cause infectious diseases have a major impact on the health of honeybees (5). For instance, Deformed Wing Virus (DWV) infection causes wing deformity, decreased body size, and a reduction in life span in adult honeybees (6, 7). To increase the fitness of the colony, newly emerged queens mate with ~15 drones (8, 9). The natural mating process enables viral transmission through semen to the queen and consequently the eggs, resulting in an infection in honeybee colonies and potentially their products (10–14).
More than 20 honeybee viruses have been identified, and studies have characterized the impact on the health of honeybees and on survival of colonies (15). The common honeybee viruses, such as Acute Bee Paralysis Virus (ABPV), Black Queen Cell Virus (BQCV), Chronic Bee Paralysis Virus (CBPV), DWV, Israeli Acute Paralysis Virus (IAPV), Kashmir Bee Virus (KBV), and Sacbrood Virus (SBV), have been found in honeybee colonies (16). However, despite the increasing knowledge of honeybee virus infections, most studies focus on honeybee workers (17–21) and queens (12, 14, 22–24), and there is little evidence of virus infection in the honeybee drones. Previous studies have shown that honeybee viruses are present in drones (11, 24, 25), but little research has focused on a pattern of honeybee virus infection in drones.
This study aimed to provide a better understanding of the virus infection pattern in honeybee drones. Honeybee drones of two honeybee species (A. mellifera and A. cerana) were collected to (i) characterize the occurrence of major honeybee viruses in drones and (ii) compare the patterns of honeybee virus distribution in the reproductive tissues [mucus glands (secrete the white mucous substance) and seminal vesicles (storage of sperm)] of drones. These studies have provided evidence for understanding insight into virus transmission in A. mellifera and A. cerana colony.
Materials and Methods
Drone Samples
Samples of A. cerana drones (n = 103) in managed hives were collected from five colonies in Miyun district (Latitude 40.614586, Longitude 117.04722), Beijing, China, and samples of A. mellifera drones (n = 116) from seven colonies were obtained at the Institute of Apicultural Research (Latitude 39.995372, Longitude 116.211416), Chinese Academy of Agricultural Science (CAAS), Beijing, China in 2016. All A. cerana and A. mellifera colonies were queenright and kept in standard hives. Drones were collected on the alighting board of a hive during mating flight times of each species (26), following the method reported in an earlier study (27). Ten drones were placed with 20 workers from the same colony in the holding cage (28). All of the holding cages were placed into an incubator at 34°C and 60% RH until dissection.
Dissection and Sperm Count
After collection, the individual drone was weighed on a digital balance (scale 0.1 mg) for whole-body mass and then immediately dissected for the reproductive organs (seminal vesicles and mucus glands, Supplementary Figure 1) from the abdomen. Drones were pinned on the thorax (dorsal side up) onto the dissection stage, and dissecting scissors were inserted into the sides of the abdomen to cut the reproductive organs. The reproductive organs were placed on the dissection stage, and then the seminal vesicles were cut off the mucus glands with scissors (27, 29). The body, seminal vesicles, and mucus glands were placed into separate 1.5 ml sterile microcentrifuge tubes and stored at −80°C until RNA extraction. For the spermatozoa count, a single seminal vesicle from each drone was transferred into 0.9% NaCl solution. Total spermatozoa from the seminal vesicle were estimated using a hemocytometer (Fuchs-Rosenthal) under a light microscope (29).
RNA Extraction and cDNA Synthesis
Total RNA was extracted from the remaining body tissues, seminal vesicles, and mucus glands of an individual honeybee drone using TRIzol reagent (Invitrogen, Carlsbad, CA, USA) according to the protocol of the manufacturer and stored in RNase-free water at −80°C. One hundred ng of total RNA was reverse transcribed with random hexamer primers to the first-strand cDNA using a PrimeScript II 1st strand cDNA Synthesis Kit (Takara Bio, Shiga, Japan) as per the instructions of the manufacturer.
Quantitative Real-Time PCR (qRT-PCR) Amplification for Seven Honeybee Viruses Present in Different Honeybee Drone Tissues
The presence of seven honeybee viruses, namely ABPV, BQCV, CBPV, CSBV, DWV, IAPV, and KBV, was determined by qRT-PCR using the LineGene 9600 series (Bioer Technology, China). Amplification was performed in 20 μl reaction volumes using SYBR Green Master Mix: SYBR Premix Ex Taq II (Tli RNase H Plus; Takara Bio, Shiga, Japan), consisting of 10 μl 2x SYBR Premix ExTaq II (Tli RNaseH Plus), 0.8 μl of 10 μM of each primer and β-actin (Supplementary Table 1), 6.4 μl of nuclease-free water, and 2 μl of cDNA. The run cycle was programed to preincubate at 95°C for 3 min to activate DNA Taq polymerase, and then, 40 cycles of denaturation at 95°C for 30 s, annealing at 60°C for 1 min, and extension at 72°C for 30 s, followed by a melt-curve dissociation analysis were performed. The melting curve was examined at the end of the amplification process to ensure the specificity of PCR products. All reactions were performed in triplicate. In addition, the no-template negative control (H2O) was run in every qRT-PCR reaction, and the reference gene, β-actin, was used as an internal control. The qRT-PCR data were expressed as the threshold cycle (Cq) value and normalized against the Cq value of the reference gene (β-actin) to the target genes (ΔCq) (30). The end products from qRT-PCR were purified and sequenced to confirm the results. All sequences were compared against sequences published in GenBank and presented in Supplementary Table 2 and Supplementary Figure 2.
Statistical Analyses
The frequencies of simultaneous infection prevalence of the honeybee virus were compared among different A. mellifera and A. cerana drone tissues using a Chi-square test. A one-way ANOVA was used to compare relative differences of the honeybee virus across drone tissues from A. mellifera and A. cerana drones. The significant difference of each honeybee virus between drone tissues was analyzed using a t-test. The physical quality of A. mellifera and A. cerana drone data were shown as means ± SEM. P-values of <0.05 were considered significant.
Results
Physical Fitness and Sperm Quantity of A. mellifera and A. cerana Drone Used in This Study
The number of sperms, whole body weight, mucus glands weight, and seminal vesicle weight from A. mellifera and A. cerana drones were quantified. The sperm quantity indicated that drone samples were mature with A. mellifera producing ~6.08 ± 2.10 × 106 sperm cell/ml in the seminal vesicle. The whole body, seminal vesicle, and mucus glands weights of A. mellifera were 222.500 ± 20.148, 21.000 ± 6.716, and 3.000 ± 1.648 mg, respectively (Supplementary Table 3). The eastern honeybee, A. cerana, produced ~0.47 ± 0.20 × 106 sperm cell/ml in the seminal vesicle. The whole body, seminal vesicle, and mucus glands weights of A. cerana were 103.563 ± 12.507, 11.968 ± 6.126, and 1.500 ± 0.900 mg, respectively (Supplementary Table 3).
Presence of Honeybee Viruses in Tissues of Drones
To investigate the presence of honeybee viruses, the body tissue, seminal vesicle, and mucus gland samples were taken from the same drone samples. A total of 69 honeybee drones were screened for the seven honeybee viruses, namely ABPV, BQCV, CBPV, CSBV, DWV, IAPV, and KBV.
Apis mellifera drones: BQCV, CSBV, DWV, and IAPV were detected in one or more A. mellifera drone tissues, while ABPV, CBPV, and KBV were not detected (Figure 1A). DWV was found in 79% (n = 33/42) of body tissue samples, 71% (n = 30/42) of mucus gland samples, and 55% (n = 23/42) of seminal vesicle samples. BQCV was found in 74% (n = 31/42) of body tissue samples, 71% (n = 30/42) of mucus gland samples, and 50% (n = 21/42) of seminal vesicle samples. CSBV was found in 7% (n = 3/42) of body tissue samples, 29% (n = 12/42) of mucus gland samples, and 26% (n = 11/42) of seminal vesicle samples. IAPV was found only in 14% (n = 6/42) of body tissue samples (Figure 1A).
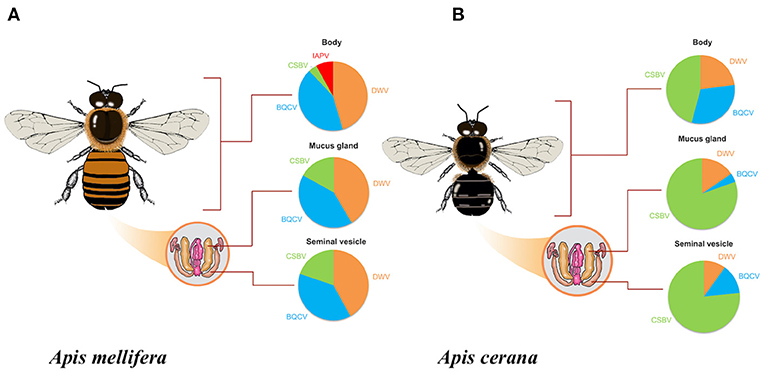
Figure 1. Percentage of honeybee virus infection in each drone tissue (A) Apis mellifera drones (B) Apis cerana drones for Black Queen Cell Virus (BQCV); Chinese Sacbrood Virus (CSBV); Deformed Wing Virus (DWV); and Israeli Acute Paralysis Virus (IAPV).
Apis cerana drones: BQCV, CSBV, and DWV were detected in one or more A. cerana drone tissues, while ABPV, CBPV, KBV, and IAPV were not detected (Figure 1B). DWV was found in 22% (n = 6/27) of body tissue samples, 15% (n = 4/27) of mucus gland samples, and 11% (n = 3/27) of seminal vesicle samples. BQCV was found in 30% (n = 8/27) of body tissue samples, 4% (n = 1/27) of mucus gland samples, and 15% (n = 4/27) of seminal vesicle samples. CSBV was found in 44% (n = 12/27) of body tissue samples, 78% (n = 21/27) of mucus gland samples, and 85% (n = 23/27) of seminal vesicle samples (Figure 1B).
The Occurrence of Multiple Honeybee Virus Infections in Tissues of Drones
Simultaneous infections of two or three viruses were detected in A. mellifera and A. cerana drones. Altogether, the following combination of virus infections was observed: dual infection, BQCV-DWV, BQCV-CSBV, BQCV-IAPV, and DWV-CSBV; triple infection, BQCV-CSBV-DWV. A detailed description of the detection of multiple honeybee virus infections in individual drone samples is summarized in Table 1.
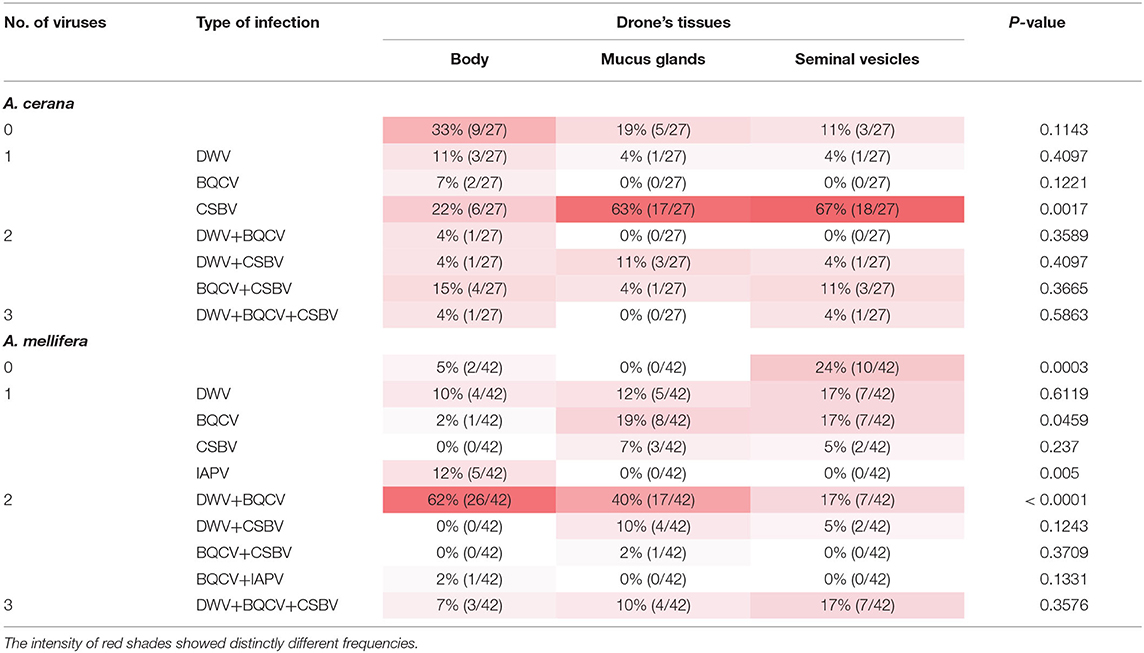
Table 1. Frequencies of simultaneous honeybee virus infections in Apis cerana and Apis mellifera drones.
Apis mellifera drones: Honeybee virus infection was detected in 95% (n = 40/43) of drone body samples. Among the infected drone body samples, 64% (n = 27/42) showed coinfection with two viruses (BQCV-DWV and BQCV-IAPV), and 7% (n = 3/42) showed coinfection with three viruses (BQCV-CSBV-DWV). Dual infection occurred in 52% (n = 22/42) of drone mucus gland samples. The most prevalent dual infection was the DWV and BQCV pair (40% of samples; n = 17/42). Coinfections with the triple infection of BQCV-CSBV-DWV were found in 10% (n = 4/42). In seminal vesicles of A. mellifera drones, we found higher rates of dual infection (21% of samples; n = 9/42) and triple infections (17% of samples; n = 7/42). The virus combination results are summarized in Table 1.
Apis cerana drones: The most prevalent single virus infection in drone body samples are DWV, BQCV, and CSBV, with frequencies of 11% (n = 3/27), 7% (n = 2/27), and 22% (n = 6/27), respectively. Multiple virus infections were 22% (n = 6/27) for dual infection and 4% (n = 1/27) for triple infection. Also, the most prevalent coinfection patterns were BQCV-DWV, BQCV-CSBV, and DWV-CSBV, and BQCV-CSBV-DWV. About 70% of the mucus gland contained at least one virus, mainly DWV, and CSBV, whereas two virus infections were found in 15% (n = 4/27) of the mucus gland. No viruses were found in 19% (n = 5/27). We found that 70% (n = 19/27) of seminal vesicle samples showed single infection, the majority of which were CSBV, and the remaining 15% (n = 4/27) showed dual-infection (DWV-CSBV, BQCV-CSBV), and 4% (n = 1/27) for triple infection (DWV-BQCV-CSBV).
Levels of Honeybee Viruses in Drone Tissues
Relative value viral analyses of A. mellifera and A. cerana drone tissues showed that all samples were negative for ABPV, CBPV, and KBV. However, four honeybee viruses, BQCV, DWV, CSBV, and IAPV, were detected. In A. cerana, DWV levels were significantly different among various tissues (P = 0.0016; Figure 2A). Both mucus glands (P = 0.0011) and bodies (P = 0.0331) had higher DWV levels than seminal vesicles. The levels of BQCV were also significantly different among A. cerana drone tissues (P = 0.0458; Figure 2B). However, the levels of CSBV displayed no significant difference among A. cerana drone tissues (P = 0.8173; Figure 2C). In A. mellifera, the viral levels (DWV, BQCV, CSBV) showed no significant difference among drone tissues (P = 0.1352, 0.2400, 0.9314, respectively; Figure 3).
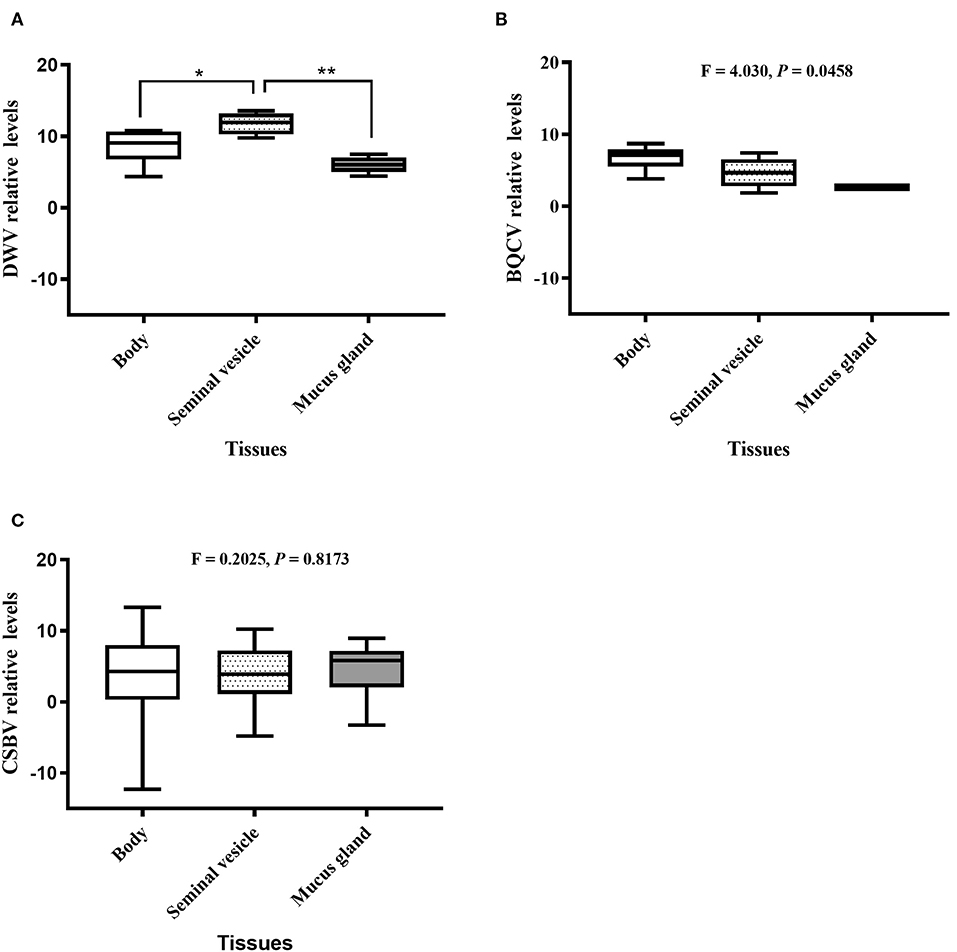
Figure 2. The relative levels of honeybee viruses in each Apis cerana drone tissue measured by quantitative real-time (qRT)-PCR. (A) DWV relative levels. (B) BQCV relative levels. (C) CSBV relative levels. *P < 0.05, **P < 0.01.
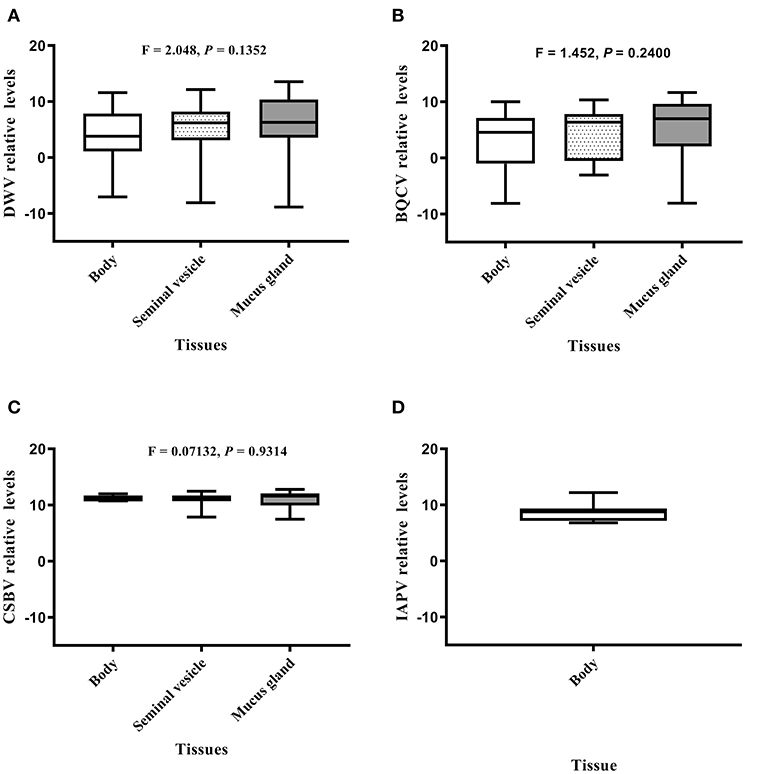
Figure 3. The IAPV relative levels of honeybee viruses in each Apis mellifera drone tissue measured by quantitative real-time (qRT)-PCR. (A) DWV relative levels. (B) BQCV relative levels. (C) CSBV relative levels. (D) IAPV relative levels.
Discussion
The present study focused on the viral distribution pattern in drones of two commercial honeybee species. The presence of viruses in A. cerana drone tissues was different from those in A. mellifera drones. We found that the prevalence of DWV and BQCV in A. mellifera drones was relatively high compared to A. cerana drones. CSBV was dominant in all tissues of A. cerana drones, especially in mucus glands and seminal vesicle tissues. Similarly, BQCV and DWV were the primary viruses detected in A. mellifera workers (17), whereas CSBV was the primary virus detected in A. cerana workers (18, 31). Previous studies characterized CSBV to exhibit host-specificity and regional diversity (32). Based on our findings, these differences may suggest virus-host specificity and/or tissue types of the drone.
Deformed Wing Virus is known to be the most widespread and is present in all castes of A. mellifera (12, 17, 23, 25). Additionally, DWV infections of A. cerana workers have been reported in Chinese apiaries (17). Previous studies reported the presence of DWV in seminal vesicles (24), mucus gland (33), and semen (11) of A. mellifera drones, suggesting that queens may be infected during natural mating or instrumental insemination (22, 25, 34). If the honeybee queens were infected with DWV, the virus could also be vertically transmitted (35). In addition, the presence of BQCV has recently been found in A. mellifera semen obtained from healthy drones (10). Similar to the results of this study, DWV and BQCV were detected in the reproductive organs of drones of both honeybee species, indicating potential sexual transmission of both viruses in honeybees. Lines of evidence have shown that DWV and BQCV infection weakened the colony and could result in colony loss (20, 36). Thus, the detection of DWV and BQCV in the reproductive organs of drones is indicative of overall colony health.
The drone reproductive organ of both honeybee species was positive for CSBV, which is a geographic strain of SBV isolated from Chinese honeybee (A. cerana). CSBV poses a serious threat to the A. cerana beekeeping industry and crop pollination ecosystem services (21). CSBV causes the Chinese sacbrood disease (CSD), and the 1972 outbreak that occurred in the Guangdong province, China destroyed more than 50% of local Chinese A. cerana honeybee colonies. CSD reemerged in Liaoning, China, in 2008 spread to nearly 75% of all A. cerana colonies, thereby causing the collapse of Chinese A. cerana colonies (37). Recently, CSBV was found to be infecting the ovary of the queen and worker and the semen of the drone and was linked to vertical transmission, food-borne transmission, and venereal transmission in A. cerana colonies (18). Our finding is consistent with previous studies and indicated that the prevalence of CSBV in reproductive organs of A. cerana drones further substantiated vertical and venereal transmissions. Moreover, our finding showed that the CSBV infection could spread in various drone tissues as it was also found in the body, mucus glands, and seminal vesicles of A. mellifera drones. The presence of CSBV in seminal vesicles suggested that CSBV may also be able to transmit in A. mellifera colonies through semen. However, further studies will need to be performed to elucidate the virulence of CSBV to A. mellifera. Moreover, as both A. mellifera and A. cerana shared the habitat or were even co-cultured in the same apiaries (38), CSBV could cause infection by crossing the species barrier and potentially kill A. mellfera larvae.
We detected high rates of multiple infections in the reproductive tissues of A. mellifera drones, in contrast to high rates of single infections in A. cerana drones. Multiple infections in A. mellifera were likely due to the infestation of ectoparasitic mites and honeybee pathogens [e.g., Nosema ceranae (38, 39)], which lead to multiple infections of honeybee viruses in the honeybee colonies. Reports have shown that Varroa mite was associated with infections of multiple honeybee viruses, such as DWV, KBV, and IAPV (40–42). Although several studies have revealed that honeybee viruses have been detected in semen (10, 11, 18) and the reproductive system of drones (24, 33), their impacts on drone fertility are still unknown. Therefore, further studies are needed to elucidate the negative effects of viruses on drone health and reproductive fitness.
The worlwide rapid loss of honeybee population and the Colony Collapse Disorder has been recognized since 2006. The beekeepers reported the losses of 50–90% of honeybees, and many areas have suffered from such loss including the US, Europe, and many parts of Asia (2–5). To date, the current status of honeybee populations is continuing to decline worldwide. Honeybee viruses are known to be one of the biotic factors causing colony losses. The finding obtained from our study displayed the honeybee virus patterns in different parts of honeybee drones and a possible sexual transmission route for viruses between colonies of two Apis species, which are domesticated in China.
Data Availability Statement
The original contributions presented in the study are included in the article/Supplementary Material, further inquiries can be directed to the corresponding author/s.
Ethics Statement
Ethical review and approval was not required for the animal study because this study involved the use of invertebrate animal (honeybees) that were collected at a commercial farm in Miyun district, Beijing, China, and an apiary maintained at the Institute of Apicultural Research, Chinese Academy of Agricultural Science, CAAS, Beijing, China, and no ethical approval was needed.
Author Contributions
PC and PPh developed the study concept, design in collaboration with JL, performed experiments and analysis, and wrote and revised the manuscript. PPh, PC, and WL participated in the experimental design and interpretation of the data. PPh, PPa, and TY performed experiments. All authors read and approved the manuscript.
Funding
This work was supported by a CMU 50th Anniversary Ph.D. Grant from Chiang Mai University (Grant no. PHD/013/2557). This study was financially supported by the Chiang Mai University fund to PC and PPh.
Conflict of Interest
The authors declare that the research was conducted in the absence of any commercial or financial relationships that could be construed as a potential conflict of interest.
Acknowledgments
We also thank Ms. Liu Shan, and Dr. Kitiphong Khongphinitbunjong for their helpful comments.
Supplementary Material
The Supplementary Material for this article can be found online at: https://www.frontiersin.org/articles/10.3389/fvets.2021.608700/full#supplementary-material
References
1. Gallai N, Salles JM, Settele J, Vaissière BE. Economic valuation of the vulnerability of world agriculture confronted with pollinator decline. Ecol Econ. (2009) 68:810–21. doi: 10.1016/j.ecolecon.2008.06.014
2. Liu Z, Chen C, Niu Q, Qi W, Yuan C, Su S, et al. Survey results of honey bee (Apis mellifera) colony losses in China (2010–2013). J Apic Res. (2016) 55:29–37. doi: 10.1080/00218839.2016.1193375
3. Potts SG, Biesmeijer JC, Kremen C, Neumann P, Schweiger O, Kunin WE. Global pollinator declines: trends, impacts and drivers. Trends Ecol Evol. (2010) 25:345–53. doi: 10.1016/j.tree.2010.01.007
4. Graystock P, Yates K, Darvill B, Goulson D, Hughes WOH. Emerging dangers: deadly effects of an emergent parasite in a new pollinator host. J Invertebr Pathol. (2013) 114:114–9. doi: 10.1016/j.jip.2013.06.005
5. vanEngelsdorp D, Meixner MD. A historical review of managed honey bee populations in Europe and the United States and the factors that may affect them. J Invertebr Pathol. (2010) 103:S80–95. doi: 10.1016/j.jip.2009.06.011
6. Bailey L. Honey bee pathology. Annu Rev Entomol. (1968) 13:191–212. doi: 10.1146/annurev.en.13.010168.001203
7. Yue C, Genersch E. RT-PCR analysis of Deformed wing virus in honeybees (Apis mellifera) and mites (Varroa destructor). J Gen Virol. (2005) 86:3419–24. doi: 10.1099/vir.0.81401-0
8. Tarpy DR, Page RE. No behavioral control over mating frequency in queen honey bees (Apis mellifera L.): implications for the evolution of extreme polyandry. Am Nat. (2000) 155:820–7. doi: 10.1086/303358
9. Tarpy DR, Nielsen R, Nielsen DI. A scientific note on the revised estimates of effective paternity frequency in Apis. Insect Soc. (2004) 51:203–4. doi: 10.1007/s00040-004-0734-4
10. Prodělalová J, Moutelíková R, Titěra D. Multiple virus infections in western honeybee (Apis mellifera L.) ejaculate used for instrumental insemination. Viruses. (2019) 11:306. doi: 10.3390/v11040306
11. Yue C, Schröder M, Bienefeld K, Genersch E. Detection of viral sequences in semen of honeybees (Apis mellifera): evidence for vertical transmission of viruses through drones. J Invertebr Pathol. (2006) 92:105–8. doi: 10.1016/j.jip.2006.03.001
12. Chen Y, Pettis JS, Feldlaufer MF. Detection of multiple viruses in queens of the honey bee Apis mellifera L. J Invertebr Pathol. (2005) 90:118–21. doi: 10.1016/j.jip.2005.08.005
13. Shen M, Cui L, Ostiguy N, Cox-Foster D. Intricate transmission routes and interactions between picorna-like viruses (kashmir bee virus and sacbrood virus) with the honeybee host and the parasitic varroa mite. J Gen Virol. (2005) 86:2281–9. doi: 10.1099/vir.0.80824-0
14. Mutinelli F. The spread of pathogens through trade in honey bees and their products (including queen bees and semen): overview and recent developments. Rev Sci Tech Oie. (2011) 30:257–71. doi: 10.20506/rst.30.1.2033
15. Brutscher LM, McMenamin AJ, Flenniken ML. The buzz about honey bee viruses. PLoS Pathogens. (2016) 12:e1005757. doi: 10.1371/journal.ppat.1005757
16. Cox-Foster DL, Conlan S, Holmes EC, Palacios G, Evans JD, Moran NA, et al. A metagenomic survey of microbes in honey bee colony collapse disorder. Science. (2007) 318:283–7. doi: 10.1126/science.1146498
17. Ai H, Yan X, Han R. Occurrence and prevalence of seven bee viruses in Apis mellifera and Apis cerana apiaries in China. J Invertebr Pathol. (2012) 109:160–4. doi: 10.1016/j.jip.2011.10.006
18. Shan L, Liuhao W, Jun G, Yujie T, Yanping C, Jie W, et al. Chinese sacbrood virus infection in Asian honey bees (Apis cerana cerana) and host immune responses to the virus infection. J. Invertebr. Pathol. (2017) 150:63–9. doi: 10.1016/j.jip.2017.09.006
19. Meixner MD, Francis RM, Gajda A, Kryger P, Andonov S, Uzunov A, et al. Occurrence of parasites and pathogens in honey bee colonies used in a European genotype-environment interactions experiment. J Apic Res. (2014) 53:215–29. doi: 10.3896/IBRA.1.53.2.04
20. Daughenbaugh KF, Martin M, Brutscher LM, Cavigli I, Garcia E, Lavin M, et al. Honey bee infecting Lake Sinai Viruses. Viruses. (2015) 7:3285–309. doi: 10.3390/v7062772
21. Gong H-R, Chen X-X, Chen YP, Hu F-L, Zhang J-L, Lin Z-G, et al. Evidence of Apis cerana Sacbrood virus Infection in Apis mellifera. Appl Environ Microbiol. (2016) 82:2256–62. doi: 10.1128/AEM.03292-15
22. Amiri E, Meixner MD, Kryger P. Deformed wing virus can be transmitted during natural mating in honey bees and infect the queens. Sci Rep. (2016) 6:33065. doi: 10.1038/srep33065
23. Gauthier L, Ravallec M, Tournaire M, Cousserans F, Bergoin M, Dainat B, et al. Viruses associated with ovarian degeneration in Apis mellifera L. queens. PLoS ONE. (2011) 6:e16217. doi: 10.1371/journal.pone.0016217
24. Fievet J, Tentcheva D, Gauthier L, De Miranda J, Cousserans F, Colin ME, et al. Localization of deformed wing virus infection in queen and drone Apis mellifera L. Virol. J. (2006) 3:16. doi: 10.1186/1743-422X-3-16
25. Yañez O, Jaffé R, Jarosch A, Fries I, Moritz RFA, Paxton RJ, et al. Deformed wing virus and drone mating flights in the honey bee (Apis mellifera): implications for sexual transmission of a major honey bee virus. Apidologie. (2012) 43:17–30. doi: 10.1007/s13592-011-0088-7
26. Koeniger N, Koeniger G. Reproductive isolation among species of the genus Apis. Apidologie. (2000) 31:313–39. doi: 10.1051/apido:2000125
27. Mananya P, Siriwat W, Nikolaus K, Gudrun K. Instrumental insemination of Apis mellifera queens with hetero- and conspecific spermatozoa results in different sperm survival. Apidologie. (2004) 35:503–11. doi: 10.1051/apido:2004043
28. Gina R., Williams GR, Mehmann MM, Yañez O, de Miranda JR, Neumann P. Sex-specific differences in pathogen susceptibility in honey bees (Apis mellifera). PLoS ONE. (2014) 9:e85261. doi: 10.1371/journal.pone.0085261
29. Cobey SW, Tarpy DR, Woyke J. Standard methods for instrumental insemination of Apis mellifera queens. J Apic Res. (2013) 52:1–18. doi: 10.3896/IBRA.1.52.4.09
30. Livak KJ, Schnittgen TD. Analysis of relative gene expression data using Real-Time Quantitative PCR and the 2 ΔΔCt method. Methods. (2001) 25:402–8. doi: 10.1006/meth.2001.1262
31. Hassanyar AK, Huang S, Li Z, Rizwan M, Mehmood S, Raza MF, et al. Prevalence of bee viruses in Apis cerana cerana populations from different locations in the Fujian Province of China. MicrobiologyOpen. (2019) 8:e00830. doi: 10.1002/mbo3.830
32. Choe SE, Thuy NTD, Hyun BH, Noh JH, Lee HS, Lee C-H, et al. Genetic and phylogenetic analysis of South Korean sacbrood virus isolates from infected honey bees (Apis cerana). Vet Microbiol. (2011) 157:32–40. doi: 10.1016/j.vetmic.2011.12.007
33. Colonello-Frattini NA, Hartfelder K. Differential gene expression profiling in mucus glands of honey bee (Apis mellifera) drones during sexual maturation. Apidologie. (2009) 40:481–95. doi: 10.1051/apido/2009009
34. de Miranda JR, Fries I. Venereal and vertical transmission of deformed wing virus in honeybees (Apis mellifera L.). J Invertebr Pathol. (2008) 98:184–9. doi: 10.1016/j.jip.2008.02.004
35. Amiri E, Kryger P, Meixner MD, Strand MK, Tarpy DR, Rueppell O. Quantitative patterns of vertical transmission of deformed wing virus in honey bees. PLoS ONE. (2018) 13:e0195283. doi: 10.1371/journal.pone.0195283
36. Martin SJ, Highfield AC, Brettell L, Villalobos EM, Budge GE, Powell M, et al. Global honey bee viral landscape altered by a parasitic mite. Science. (2012) 336:1304–6. doi: 10.1126/science.1220941
37. Hu Y, Fei D, Jiang L, Wei D, Li F, Diao Q, et al. A comparison of biological characteristics of three strains of Chinese sacbrood virus in Apis cerana. Sci Rep. (2016) 6:37424. doi: 10.1038/srep37424
38. Chantawannakul P, de Guzman LI, Li JL, Williams GR. Parasites, pathogens, and pests of honeybees in Asia. Apidologie. (2016) 47:301–24. doi: 10.1007/s13592-015-0407-5
39. Chaimanee V, Pettis JS, Chen Y, Evans JD, Khongphinitbunjong K, Chantawannakul P. Susceptibility of four different honey bee species to Nosema ceranae. Vet Parasitol. (2013) 193:260–5. doi: 10.1016/j.vetpar.2012.12.004
40. Chen Y, Pettis JS, Evans JD, Kramer M, Feldlaufer MF. Transmission of kashmir bee virus by the ectoparasitic mite Varroa destructor. Apidologie. (2004) 35:441–8. doi: 10.1051/apido:2004031
41. Di Prisco G, Pennacchio F, Caprio E, Boncristiani HF Jr, Evans JD, Chen Y. Varroa destructor is an effective vector of Israeli acute paralysis virus in the honeybee, Apis mellifera. J Gen Virol. (2011) 92:151–5. doi: 10.1099/vir.0.023853-0
Keywords: Apis mellifera, A. cerana, drone reproductive, CSBV, venereal transmission
Citation: Phokasem P, Liuhao W, Panjad P, Yujie T, Li J and Chantawannakul P (2021) Differential Viral Distribution Patterns in Reproductive Tissues of Apis mellifera and Apis cerana Drones. Front. Vet. Sci. 8:608700. doi: 10.3389/fvets.2021.608700
Received: 25 September 2020; Accepted: 10 February 2021;
Published: 24 March 2021.
Edited by:
Jesus Hernandez, Consejo Nacional de Ciencia y Tecnología (CONACYT), MexicoReviewed by:
Richou Han, Guangdong Institute of Applied Biological Resources, ChinaFrancisco Rivera-Benítez, Instituto Nacional de Investigaciones Forestales, Agrícolas y Pecuarias (INIFAP), Mexico
Copyright © 2021 Phokasem, Liuhao, Panjad, Yujie, Li and Chantawannakul. This is an open-access article distributed under the terms of the Creative Commons Attribution License (CC BY). The use, distribution or reproduction in other forums is permitted, provided the original author(s) and the copyright owner(s) are credited and that the original publication in this journal is cited, in accordance with accepted academic practice. No use, distribution or reproduction is permitted which does not comply with these terms.
*Correspondence: Panuwan Chantawannakul, cGFudXdhbiYjeDAwMDQwO2dtYWlsLmNvbQ==; Jilian Li, YnVtYmxlYmVlbGpsJiN4MDAwNDA7aG90bWFpbC5jb20=