- 1Doctorado en Ciencias Agropecuarias, Universidad Autónoma Metropolitana Xochimilco, Ciudad de México, Mexico
- 2Laboratorio de Biología Molecular, Departamento de Producción Agrícola y Animal, Universidad Autónoma Metropolitana Xochimilco, Ciudad de México, Mexico
- 3Laboratorio de Microbiología Agropecuaria, Universidad Autónoma Metropolitana Xochimilco, Ciudad de México, Mexico
- 4Departamento de Producción Agrícola y Animal, Universidad Autónoma Metropolitana Xochimilco, Ciudad de México, Mexico
- 5Centro Universitario UAEM Amecameca, Universidad Autónoma del Estado de México, Amecameca, Mexico
- 6Universidad Autónoma Chapingo, Texcoco, Mexico
A polyherbal feed mixture containing (Achyrantes aspera, Trachyspermum ammi, Citrullus colocynthis, Andrographis paniculata, and Azadirachta indica) was evaluated in growing calves through blood chemistry, blood biometry, and gene expression during the pre-ruminant to weaning period. Forty Holstein calves (initial BW 45.6 ± 3.2 kg; 22.8 ± 0.9 days post birth) from a dairy farm were randomly assigned to the following treatments: 0, 3, 4, and 5 g/d of a polyherbal mixture, dosed in colloid gels with gelatin. Calves were housed in individual outdoor boxes with ad libitum access to a 21.5% CP calf starter and water and fed individually with a mixture of milk and a non-medicated milk replacer (22% CP). Blood samples were collected on day 59 for blood chemistry, blood biometry, and gene expression analysis in leukocyte through microarray assays. Immunoglobulins were quantified by enzyme-linked immunosorbent assay. The animals treated with the polyherbal mixture showed a quadratic effect on final body weight, daily weight gain, final hip height, and final thoracic girth. The best performance results were obtained with a treatment dose of 4 g/d. The serum IgG increased linearly with the treatment doses. Gene set enrichment analysis of upregulated genes revealed that the three biological processes with higher fold change were tight junction, mucin type O-Glycan biosynthesis, and intestinal immune network for IgA production. Also, these upregulated genes influenced arachidonic acid metabolism, and pantothenate and CoA biosynthesis. Gene ontology enrichment analysis indicated that the pathways enriched were PELP1 estrogen receptor interacting protein pathways, nuclear receptors in lipid metabolism and toxicity, tight junction, ECM-receptor interaction, thyroid hormone signaling pathways, vascular smooth muscle contraction, ribosome function, glutamatergic synapse pathway, focal adhesion, Hippo, calcium, and MAPK signaling pathways.
Introduction
In dairy farms, respiratory and enteric diseases can occur, causing a high rate of mortality in young animals, significant economic losses (1, 2), and lower resistance to caloric stress (3, 4). In these animals, the feed intake is affected, which seriously compromises the development of the immune system (5). These diseases require the use of antibiotics which have been banned as growth promotors in several countries (6). There is an increase in public pressure for the usage of these drugs in animals, and therefore, the evaluation of feed plant additives with beneficial effects in adult ruminants on indicators of health and production are critical for raising calves (7–9).
In adult ruminants, a polyherbal mixture with phosphatidylcholine (PCho) and other nutraceutical metabolites results in improved performance, and health suggesting it may have beneficial effects on calves (10–12). Dietary PCho stimulates the IL-2 formation in the spleen and CD25, CD28, CD71 expression (13), improving the immune response. Choline is part of 1-alkyl-2-acetyl-sn-glycero-3-phosphocholine (platelet-activating factor), a recognized activator of immune response (14, 15), and rumen-protected choline can improve the immune response and health in cows (16–18); however, evaluations in calves before weaning are missing.
The objective of this study was to evaluate the effect of an herbal product, with PCho elaborated with medicinal plants from India (Achyrantes aspera, Trachyspermum ammi, Citrullus colocynthis, Andrographis paniculata, and Azadirachta indica), on the productive performance and immune response in Holstein calves. These plants have already been evaluated by our researching group (10–12) and the results obtained showed beneficial effects on production in adult animals. However, the activity of these plants on young animals is unknown.
Materials and Methods
Animals, Diet, and Experimental Design
The experimental procedures were performed in accordance with the recommendations of the CIOMS (19), observing the standards for ethics, biosafety, and animal well-being approved by the institutional committee. The experiment was conducted in a commercial farm located in the central part of the northern region of México (25°39' 14.4 “N 103°27' 27.8” W; elevation 1,139 m). The climate was semi-dry, with extreme temperatures, with an annual rainfall of 250.6 mm. Average temperature was 20.21°C, with a maximum of 33.60°C and a minimum of 5.59°C. Forty Holstein calves (initial BW 45.6 ± 3.2 kg) received individual doses of a polyherbal mixture (BioCholine, Indian Herbs). The doses were defined based on metabolic weight using results from sheep experiments (10, 12) and were as follows: 0, 3, 4, and 5 grams per day (g/d). The herbal products were prepared in colloid gels with gelatin to ensure individual daily consumption in the morning during the 90 days that the experiment lasted. The polyherbal-gelatin mixture (PGM) was prepared 1 day before administration. Calves were clinically healthy at the beginning of the experiment and had an age of 22.8 ± 0.9 days post birth. Calves were weighted and measured at the beginning and at the end of the experiment (90 days of evaluation). During weighing, the hip and wither heights as well as the thoracic girth were measured (20). All calves received colostrum following birth, and immunoglobulins in the blood were measured by refractometry.
Calves were individually placed in outdoor boxes (2.00 × 1.25 m), with water ad libitum, and fed twice a day (7.00 am and 17.00 pm). They received a mixture of milk (56%) and a non-medicated milk replacer (44% milk replacer Nu-3; Grupo Nu-3 balanced food, Guanajuato, México) with 4% moisture, 22% crude protein, 15% fat, 0.1% crude fiber, 6.0% ash, and 52.9% nitrogen-free extract (NFE), prepared with 130 g/L water. The milk powder (143 g/L) was reconstituted in hot water (65°C) and fed at a temperature of 39°C in a container; prior to feeding, it was mixed with milk at 130 g/L. Milk replacer was offered from day 4 of age twice a day (4 L each time) and was reduced to one meal on day 25 as starter intake was augmented. Form the second day of life, a commercial concentrate composed of sorghum grain, rolled corn, soybean meal, and cane molasses was offered from a bucket. The milk starter used was Premium Initiation Weaning Premature (Nuplen, Durango, México) with 13.0% moisture, 21.5% crude protein, 3.0% fat, 8.0% crude fiber, 7.0% ash, and 47.0% NFE. The starter concentrate was offered from the third day of age and was gradually increased.
Samples and Data Collection
Daily intake of milk and starter concentrate was recorded. The variation in consumption between days was evaluated as an indicator of stability. Feed conversion was estimated as the ratio of kg of dry matter (DM) intake/kg of BW gain, including DM from starter, milk replacer, and milk starter. Calves were evaluated daily following the morning milk feeding between 06:00 and 10:00 to check for diarrhea, pneumonia, otitis, and other diseases. On day 59 of the experiment, pre-prandial blood samples of all animals were collected from the jugular vein using vacutainer tubes with sodium citrate, EDTA, and without anticoagulant. These blood samples were maintained under refrigeration (4°C) until use. Tubes without anticoagulant were centrifuged (Sigma 2-16 k, Germany) at 3,500 x g for 20 min to obtain blood serum, which was stored in Eppendorf tubes and kept in a freezer at −20°C until analysis. Cholesterol, glucose, total protein, albumin, and bilirubin were determined with an autoanalyzer Kontrolab 2017. The blood sample collected in disodium-EDTA was used for complete blood count (CBC). The CBC leukocyte differential count and the hematocrit were determined in the hematology analyzer QS Kontrolab EasyVet.
Immunoglobulin Analyses
Calves were immunized against Clostridium spp. (Covexin 10 vaccine) on day 40 of the experiment, and serum obtained from the blood samples collected with sodium citrate was used to evaluate the antibodies against the antigen (Clostridium spp.). The antigen was bound to the microtiter plate and incubated overnight at room temperature. For antibody evaluation, 100 μL of the serum sample was added to each well and incubated (1 h, 37°C). Plates were decanted, and 100 μL of bovine IgG specific secondary antibody was added and incubated (1 h, 37°C). Then, 100 μL of TMB (3.3′, 5.5′-tetramethylbenzidine liquid) was added to each well, and the reaction was stopped with 100 μL of H2SO4 (1%). The plates were analyzed in an ELISA reader (Model 350, BioRad) at a wavelength of 540 nm.
RNA Extraction From Blood Samples
Blood samples with sodium citrate from the control and the polyherbal treated group (4 g/d) were processed using SRL solution (Tris–HCl 10 mM pH 8, MgCl2 5 mM, and NaCl 10 mM) from a total volume of 24 mL of blood per experimental unit (calf). Total RNA was extracted from the leukocyte package using Trizol (Invitrogen). The RNA pellet was suspended in 20 μL of H2O DEPC 0.1% water and stored at −80°C until processing. RNA purity was determined by electrophoresis on a 1% agarose gel. The RNA samples were treated with DNases (Promega). The purified RNA was quantified by spectrometry using A260-A280 (21). Two pools of RNA (control and treatment) were prepared from eight biological repetitions (30 ug RNA) and stored at −70°C for 24 h and subsequently centrifuged for 15 min at 4°C.
Microarray Analyses
Microarray analyses were carried out at the Unidad de Microarreglos del Instituto de Fisiología Celular (UNAM) in Mexico City, using a heterologous mouse M22K_10_16 chip to evaluate the differential expression of the genes. The arrangement evaluated 24,341 genes, and 10 μg of total RNA as synthesized to cDNA with the incorporation of nucleotides dUTP-Alexa555 and dUTP-Alexa647, using the CyScribe First-Strand cDNA Amersham labeling kit. Fluorophore incorporation was analyzed at an absorbance of 555 nm for Cy3 and 655 nm for Cy5. Equal amounts of cDNA were hybridized using the HybIT2 solution (TeleChem International INC). The arrangements were incubated for 14 h at 42°C, with three consecutive washes using 1 x SCC, 0.05% SDS at room temperature. We used the equipment and software ScanArray 4000 (Packard BioChips) for acquisition and quantification of the images of the arrangements. Microarray data analysis was conducted with the genArise software (http://www.ifc.unam.mx/genarise/). The list of genes considered upregulated (Up) and downregulated (Down) was analyzed with the bioinformatics tool DAVID [Functional Annotation Bioinformatics Microarray Analysis, (https://david.ncifcrf.gov/)], which allows the grouping of genes based on their functional similarity.
Gene Ontology Analysis
Gene set enrichment analysis using gene ontology was applied to extract biological meaning from the identified differentially expressed transcripts; gene ontology terms with a P-value <0.05 were considered as enriched. The Database for Annotation, Visualization, and Integrated Discovery (DAVID) 6.8 Bioinformatic resource was used for gene set enrichment (22).
Validation of Differential Genes Expressed Using Quantitative Real-Time PCR
First-strand cDNA was synthetized from 1,500 ng of total RNA using Oligo(dT) and RevertAid Reverse Transcriptase (Thermo Scientific). Specific primers for PPARβ and GAPDH were designed with Primer-BLAST (https://www.ncbi.nlm.nih.gov/tools/primer-blast/) (Table 1). Primers for PPARα and ACOXI were taken from previous studies (Table 1) (23). The qPCR was performed on a real-time PCR system Rotor-GeneQ (Qiagen), using Sybr Green for detection (Thermo Scientific). Amplification for PPARα and ACOXI was carried out for one cycle at 95°C for 10 min, followed by 45 cycles at 95°C for 15 s, 60°C for 60 s, and 70°C for 30 s. Amplification for PPARβ and Relb was carried out for one cycle at 95°C for 2 min, followed by 45 cycles at 95°C for 30 s, 57°C for 30 s, and 72°C for 30 s. Glyceraldehyde-3-phosphate dehydrogenase (GAPDH) was used as internal control for normalization. The 2−ΔΔCt method was used to determine the relative mRNA quantification (24).
Statistical Analyses
The Shapiro-Wilk test was used to test the normal distribution of variables. Data were analyzed using the R software (25), and orthogonal linear and quadratic polynomials were used to evaluate the effects of the polyherbal additive. The model used was: Yij = μ + τi + eij, in which μ is the mean value, τi is the treatment effect (fixed), and eij is the error term. The initial body weight was used as a covariate in daily gain and final BW (26). The number of cases of diseases and the doses of antibiotics were analyzed using the Kruskal Wallis test. If the quadratic effect was significant, the optimal concentration for daily gain was estimated using the first derived from the quadratic equation (27).
Results
Performance and Health Parameters in Calves
The animals treated with the polyherbal mixture showed a quadratic response in final BW, average daily gain (ADG), final hip height, and final thoracic girth, with the best numeric performance results with a dose of 4 g/d (Table 2). However, the optimal dose estimated by regression to maximum ADG was 2.47 ± 0.87 g/d of the polyherbal mixture. Starter intake was stimulated at a dose of 4 g (quadratic P < 0.001), whereas liquid ingestion showed a linear increase (P < 0.001), compensating for the reduction in solid consumption with no effect on feed conversion (Table 2). All calves received colostrum following birth, and immunoglobulins in the blood were measured by refractometry, the results obtained showed a concentration of 6.54± 0.525 g/dl. A reduction in otitis (quadratic effect P < 0.10) was observed with intermediate doses of polyherbal in the calves (Table 2), and a linear increment (P < 0.10) of anti-Clostrdium spp. IgG was detected by enzyme-linked immunosorbent assay.
Blood Chemistry and Biometry
Blood chemistry changes are shown in Table 3. The treatment with polyherbal mixture caused a reduction in glucose levels (linear P < 0.01), with a quadratic increase in B-OH butyrate (P < 0.05). Globulin levels were reduced (P < 0.10 quadratic) and albumin levels showed a quadratic increment (P < 0.05), resulting in a reduction in the albumin globulin ratio (quadratic P < 0.01). Urea showed a reduction (P < 0.10), whereas uric acid was not affected. The bilirubin level increased linearly (P < 0.01) with the polyherbal treatment; however, liver enzymes [alkaline phosphatase, ALP; lactate dehydrogenase, LDH; and aspartate aminotransferase, AST (GOT)] were not affected. Serum calcium and phosphorus remained unchanged. The results of the biometry are presented in Table 4. There was a reduction in neutrophils in band (P < 0.05). Lymphocytes, basophiles, and plasma protein were reduced with lower or intermediate doses of BioCholine (quadratic effect, P < 0.10).
Gene Expression Variations
The heterologous microarray represented ~22,000 transcripts, of which 2,442 were differentially expressed; a total of 1,093 and 1,349 transcripts were up- and downregulated, respectively. A total of 264 genes with values of +2.0 to 5.5 were upregulated and 401 genes with values of −2.0 to −5.8 were downregulated. The 30 most strongly up- and downregulated transcripts in blood leukocytes of weaning calves supplemented with polyherbal mixture are presented in Tables 5, 6. The most strongly upregulated transcripts include functional categories such as proto-oncogene (Jun, Lck, Pdgfra), tyrosine protein kinase (Tyro3, Lck, Pdgfra), immunoglobulin domain (Tyro3, Lrn2, Pdgfra, Pdgfrl), and ATP-binding (Abcc8, Tyro3, Entpd2, Lck, Pdgfra, Plk1) (Table 5). The most strongly downregulated transcripts include functional categories such as phosphoprotein (Erc1, Rab11fip2, Rpusd2, Sh3d19, Tal1, Baz1a, Cobl, Cry2, Dapk1, Glycam1, Grhpr, Hmg20b, Kif2c, Lig1, Polr3d, Ptpn6, Sel1, Xirp2), protein transport (Erc1, Rab11fip2, Xpo5, Serp1), and ubiquitin-like modifier conjugation (Tal1, Cry2, Dapk1, Hmg20b, Kif2c, Polr3d) (Table 6).
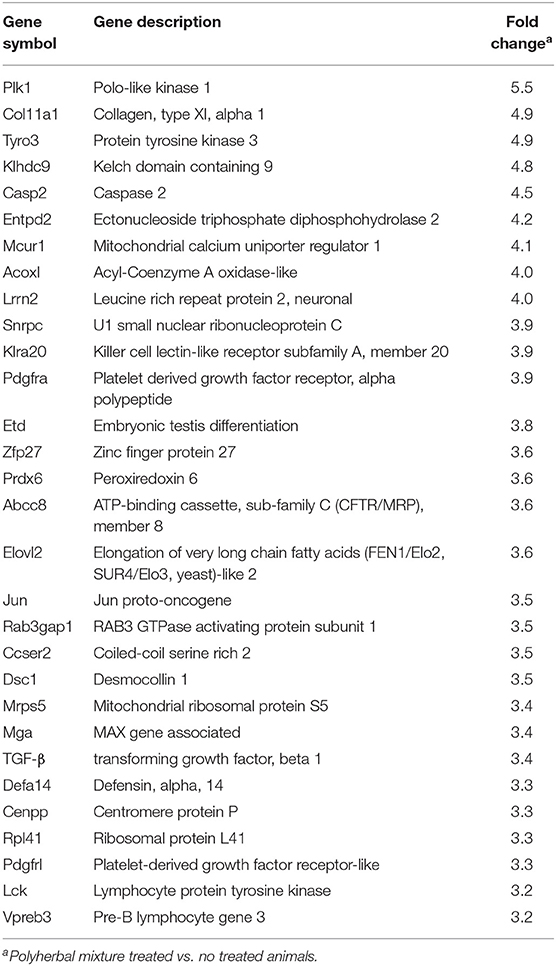
Table 5. Microarray analysis of differential gene expression profile in peripheral blood cells (upregulated genes).
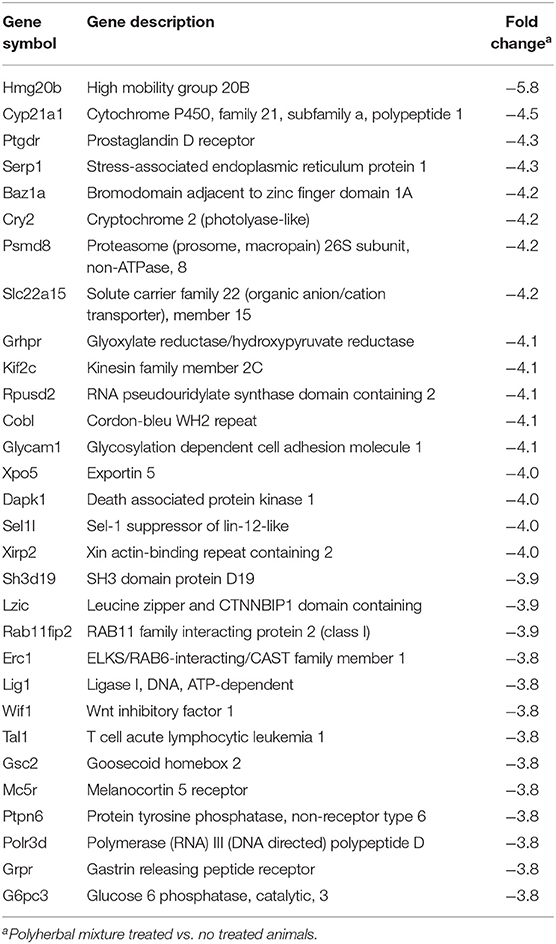
Table 6. Microarray analysis of differential gene expression profile in peripheral blood cells (downregulated genes).
Gene Set Enrichment Analysis (GSEA) of upregulated genes (Table 7) revealed that the three biological processes with higher fold change were tight junction (Fc: 3.7; P = 0.00002), mucin type O-Glycan biosynthesis (Fc: 3.7; P = 0.044), and intestinal immune network for IgA production (Fc: 3.4; P = 0.015). We observed reduced expression of genes related with tight junction (Rab13, Src, Afdn, Cdc42, Llgl2, Mpp5, Myh10, Myh9, Myl9, Pard6g, Prkci Tjp1); among these genes, there were four code for claudins (Cldn-7, Cldn8, Cldn9, Cldn16). The expression of gcnt3 gene was reduced (Fc = −1.6); this gene is coded to the enzyme responsible for synthesis of all known β6 N-acetylglucosaminides. The treatment with polyherbal mixture reduced the expression of cytokines necessary to promote terminal differentiation and switching of immunoglobulin A class of B cells, explaining the downregulation of the genes involved in the intestinal immune network for IgA production (Cd40lg, Cd86, H2-DMb1, Il10, Madcam1, Tgfβ1, Tnfrsf13b). The microarray did not identify differentially expressed transcripts that codified for Tnf-α, Ifn, Il-6, and Il8, which code for inflammatory cytokines. In contrast, a reduced expression of Tgfβ1 (Fc: −3.4) and Il-100 (Fc: −2.5) was shown.
Analysis of gene enrichment with positive regulation identified pantothenate and CoA biosynthesis (P = 0.03), arachidonic acid metabolism (P = 0.01), and diabetes mellitus type 2 (P = 0.01) (Table 7). The treatment with polyherbal mixture increased the expression of four genes whose transcription is related to pantothenate and CoA biosynthesis (Enpp1, Enpp3, Pank4, and Vnn3).
Analysis of total up and downregulated transcripts (Figure 1), indicates an important modulator effect of polyherbal mixture treatment on Pelp1 modulation of estrogen receptor activity (Fold change, Fc = 4.4; Src, Esr1, and Mapk1), nuclear receptors in lipid metabolism and toxicity (Fc = 2.4; Abca1, Abcb1b, Cyp1a2, Cyp27b1, Nr1h3, Nr1i3, PPARa, PPARd, Rara, and Rarg), and tight junction (Fc: 2.4).
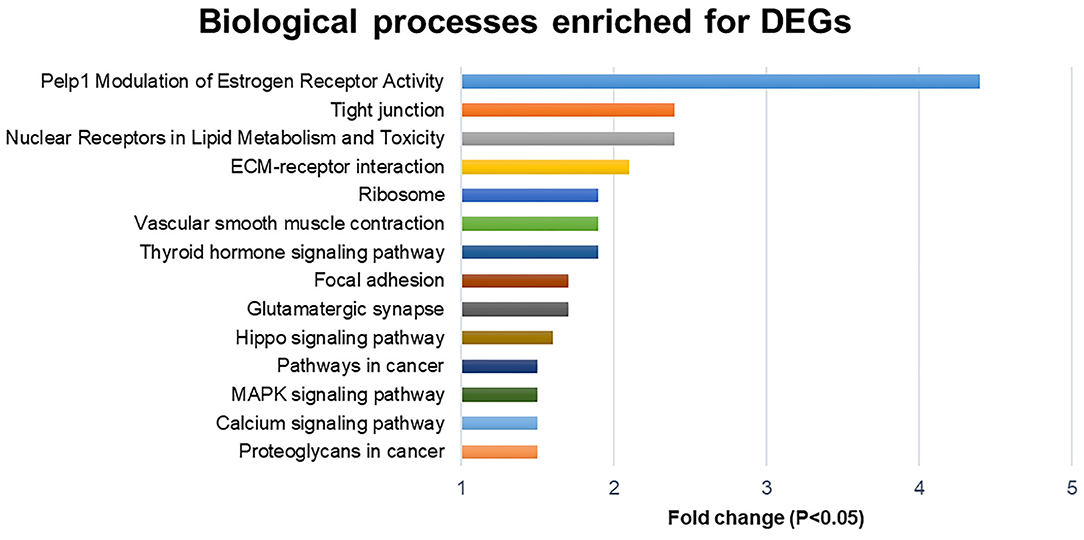
Figure 1. Biological processes enriched for differentially expressed genes (DEGs; down and upregulated transcripts).
Analysis of gene expression by qPCR (Table 8) indicates that PPARα and PPARβ gene transcription were upregulated (P = 0.15) and downregulated (P = 0.05), respectively.
Discussion
Calf Parameters
The polyherbal mixture provides conjugates of choline (mainly PCho) and secondary metabolites that, at low doses, have nutraceutical properties (11), which explains the improved response in growth (quadratic effect). Growing calves have a high protein synthesis, and choline participates as a methyl donor (via betaine) necessary for the methylation of DNA, RNA, and proteins (28, 29), in addition to the synthesis of the phospholipids of cell membranes and plasma lipoproteins (30) and to multiple functions in the organism (31). Few choline evaluations have been reported in calves since deficiencies of this nutrient were reported in the 1950s (29). Although it is included in milk replacers and starters, as suggested by the NRC (32), it is not supplemented after weaning. However, studies with growing lambs show a quadratic response to supplementation in the form of rumen-protected choline (RPC) or with the polyherbal mixture evaluated here (11, 12, 30). The positive response to choline is due to the rapid growth rate of Holstein cattle after weaning, and genetic selection can lead to increased requirements for this nutrient. Some RPC assessments have been made in steers in growing and finishing stages. In these studies, it was shown that when the animals approach to mature weights, the response to the nutrient is lower. Response to RPC was also reduced as the animal approaches mature weight (33–35).
In calves, few phytogenic feed additives with essential oils have been evaluated (36), which report higher intake of starter and better feed efficiencies and gain, whereas other authors did not find intake responses (37, 38). It is not clear if the changes in consumption showed in this investigation were due to some secondary metabolites of polyherbal mixture or by the conjugates of choline. In lambs that responded to the same polyherbal mixture in ADG, intake was not affected (12). However, in an experiment with graded levels of RPC, intake showed a quadratic response parallel to the gain response (39). Changes in thoracic girth in intermediate doses indicate greater rumen development, but there are few reports where nutrients in the diet affected these variables, and generally, those changes are not noticeable (40–42).
Regarding the evaluated health parameters, the reduction in otitis can be explained by some antibacterial metabolites in the polyherbal mixture (11), by the choline antioxidant capacity (43) that may play a preventive role in otitis (44), or by improving the immune response (45); this is important as in calves, otitis is commonly linked to pneumonia (46). However, more studies are necessary to confirm these results. The polyherbal mixture improved the immune response to vaccination, as observed in the quantifications of serum immunoglobulins in some feed plant additives (8), and could be related with the upregulation of some genes such as Tyro3, Lrn2, Pdgfra, and Pdgfrl.
Blood Chemistry and Biometry
The blood chemistry values were in the normal range for calves (47, 48). Only glucose showed a slight reduction in animals supplemented with herbal choline. The polyherbal mixture contains Azadirachta indica, which has shown hypoglycemic effects (49). However, these effects do not affect leukocytes, which was indicated by the downregulation of the expression (−3.8 fold lower than in the control group) of the g6pc3 gene, which codes for glucose-6-phosphatase involved in the homeostatic regulation of glucose in monocytes and neutrophils. The major function of glucose 6-phosphatase-β is to provide recycled glucose to the cytoplasm of neutrophils to maintain normal function (50).
The change in butyrate may be an indicator of ruminal development. Glucose is reduced because as calves are growing, they depend more on volatile fatty acids. Serum calcium and phosphorus also indicate that the feed additive did not affect the mineral balance of these elements, regardless of differences in feed intake of starter (51, 52). The calves were healthy, and minor changes in some metabolites were detected, which were, however, not associated with infectious agents or chronic inflammatory processes. Urea blood reductions indicate an adequate general situation of protein metabolism (53), confirmed by uric acid, a metabolic product of protein metabolism; the creatinine levels indicate that the kidney function was not affected (54).
The main serum indicators used to evaluate liver alterations include AST(GOT), ALT, bilirubin, total protein, and total albumin (55) and were not altered by the treatment with polyherbal mixture, confirming that the liver was not damaged. Changes in bilirubin levels can be explained by the reduction in the expression of ugt2b37 (UDP-glucuronosyltransferase), which codes for the enzyme involved in bilirubin metabolism (56) and catalyzes the transformation of unconjugated or indirect bilirubin into conjugated or direct bilirubin by the addition of glucuronic acid. Some herbal supplements and extracts with polyphenolic compounds can have inhibitory effects on the gene responsible for glucoronidation of bilirubin (57). Since the calves in our study were healthy, presumably cross-reactions from some metabolites from herbal choline could be responsible for the increase in bilirubin, rather than liver injury. According to a previous study, serum bilirubin concentration is not considered as a sensitive indicator of liver disease in young calves (55).
In other experiments with feed plant additives, serum protein levels were not affected (36), and the results may vary depending on the mechanism of action of phytobiotics, secondary metabolites, and doses. Liver enzymes as ALT is the most specific test to detect liver dysfunction as well as LDH (55), suggesting that the polyherbal mixture evaluated does not have hepatotoxic metabolites and its choline conjugates could have an antioxidant effect (58) and other beneficial effects for liver function of this nutrient (59). The Polyherbal mixture has several chemical compounds, and some of them have already been reported to possess bactericidal properties (11, 12). Therefore, this could affect the structure and composition of the microbiota, affecting the ruminal fermentation. These could explain the lack of correlation between the starter intake and blood B-OH butyrate.
The biometry results indicate that calves were healthy and within the reported values (60, 61), similar to those shown for 1-month-old calves (62). The reduction in neutrophils in band was not correlated with the presence of bacterial disease (63). In fact, the polyherbal mixture treatment stimulated genes such as Defa14, recognized as antimicrobial peptides (64). In one experiment, aflatoxin B1 decreased neutrophils in calves, and the administration of choline helped to reduce the toxic adverse effects (65).
Gene Expression Variations
Microarray analysis allowed us to detect significant changes in gene expression induced by metabolites in the polyherbal mixture. The analysis of changes in gene expression by bioinformatics analysis (GSEA) resulted in the identification of specific metabolic processes involved in calf metabolism. Although this work only evaluates gene expression in leukocytes, the variations in the expression observed in these cells indicates variations produced in other cells.
The changes in gene expression could explain some variations observed in calf performance and changes in blood variables. The possible physiological implication of this variation raises important questions about the plant metabolites. For example, the reduction in the expression of gcnt3 gene and its implications may be important since this gene coded the enzyme responsible for the synthesis of all β6 N-acetylglucosaminides, which play an important role in O-linked glycosylation in mucin biosynthesis and, consequently, has implications on the functional state of the mucosa of the intestinal and respiratory tracts (66).
In calves treated with polyherbal mixture, the expression of Claudin genes in leukocytes was reduced. Claudins are the main transmembrane proteins that regulate the intestinal epithelial permeability, controlling ion and microbial product diffusion through the epithelial cell layer (67, 68). Malmuthuge et al. (69) have reported that the diet regulates the expression of Claudin 4 and occludin, altering the absorption of macromolecules. Considering that modification in expression of these genes was induced by metabolites in the polyherbal mixture, the same response could be present in cells of the gastrointestinal tract, thereby modifying intestinal permeability and the absorption of macromolecules.
The genetic enrichment analysis provided a perspective on the changes induced by the polyherbal treatment at different metabolic stages. Increased pantothenate and CoA biosynthesis (Table 7) has important consequences on energy metabolism, which were manifested in calf growth, and expression of Pank4, which encodes pantothenate kinase, a regulatory enzyme in CoA biosynthesis. The gene Pank4 phosphorylates the vitamin pantothenate to 4′-phosphopantothenate, which is converted into CoA and participates in the tricarboxylic acid cycle, fatty acid metabolism, and numerous other reactions of intermediary metabolism (70). The Pank4 mRNA is upregulated at high glucose concentrations to allow higher flux through the tricarboxylic acid cycle (71).
Coenzyme A biosynthesis and the increased PPARα gene expression induced by choline have important implications. Here, PPARα stands out because the group of nuclear proteins called PPAR (peroxisome proliferator-activated receptor) plays a key role in the regulation of metabolic pathways such as lipid metabolism (72), and it is possible that the over-expression of the genes Acoxl (acetyl CoA-oxidase) and the gene Cpt2 (carnitine palmitoyl transferase 2) is mediated by the positive regulation of PPARα and by other effects of choline, such as antioxidant effects, lipotropic effects, or through the activity of GSH-Px (43).
The treatment with polyherbal mixture also stimulated a group of genes associated with nuclear receptors in lipid metabolism and toxicity (Figure 1). There are PPAR receptors for fatty acids and cytochrome P450 genes involved in lipid metabolism; some genes of cytochrome P450 are related to the detoxification of environmental toxins and the metabolism of xenobiotics and drugs (73); this explains the beneficial effects of choline in calves with aflatoxins (65). The ECM-receptor interaction pathway is involved in adipose tissues and important for adipogenesis and functional macromolecules (glycosaminoglycans, collagen, elastin, fibronection, and lammin), but there are complex interactions with other cells, which need to be addressed (74).
Ribosomal gene stimulation suggests that polyherbal treatment simulates protein synthesis, which coincides with the growth response observed in calves; the ribosomes are the cellular organelles responsible for protein synthesis in cells (75). The effects of glutamatergic synapse pathway stimulation in calves are unclear, but it is known that glutamate is an important neurotransmitter in the central nervous system and that its stimulation affects receptors, channels, and neurotransmitter transporters (76), with potential effects on the stress response. In their review, Musazzi et al. (77) present evidence that glucocorticoids and acute stress increase extracellular glutamate levels, affecting glutamate release in the brain; the authors highlight that chronic stress may reduce glutamate metabolism. In this sense, animals in confinement can be chronically stressed, and calves receiving a dose of 3 g/d showed better health and improved weight gain. This leads us to infer that this route could have reduced stress.
Focal adhesion processes may have improved the health conditions of calves since they participated in cell migration and invasion (78). Liu et al. (79) have demonstrated that the phytoestrogen genistein at high concentrations reduced the damage in estradiol-induced cardiovascular cells, affecting the focal adhesion pathway. The Hippo pathway plays a role in organ size and morphogenesis control as well as cancer development (80). Growth is an important parameter in calves, and changes in morphometric measurements were observed in this study.
The overall effects reflect the combined effects of conjugates of choline and secondary metabolites from polyherbal mixture (11) on the metabolism of lipids and glucose (59, 81) and on the immune response (13, 17, 18); this is in agreement with the demonstrated beneficial effects of natural products from traditionally used medicinal plants (72), and it can have been relative compared with the gene expression in experiments with synthetic choline products (82).
The treatment with polyherbal mixture could have an important effect in PPARα gene expression. It is important for immunity in calves because it is predominantly expressed in T and B cells (83). Also, PPARα is an important regulator of gene expression in rumen epithelium during the period from pre-ruminant to ruminant and can be involved in the mediation of energy metabolism within the rumen epithelium to support rumen development and differentiation of the ruminal papillae during weaning (84, 85). Metabolically, PPAR receptors induce the proliferation of peroxisomes in cells, a process that generates the transcription of the acyl-CoA oxidase (AcoxI) gene (86); however, AcoxI gene validation by qPCR did not confirm the microarray results where AcoxI was upregulated. On the other hand, the increased PPARα gene transcription (Table 8) can be explained by the mechanism of action of choline conjugates, mainly phosphatidylcholine, which we assume is the main metabolite involved in the stimulation of gene expression of Pla2g6, Pla2g4e, and Pla2g2d, which encode phospholipases A2, involved in the hydrolysis of arachidonic acid in the C2 position of phosphatidylcholine (87). The release of arachidonic acid stimulates the expression of the Ptgds and Ptgis genes, which encode enzymes involved in the cyclooxygenase pathway for the production of prostaglandins and prostacyclines. The genes Alox12 and Alox15 encode enzymes involved in the lipoxygenase pathway, synthesizing the acids 12-HPETE (12-hydroperoxyeicosatetraenoic acid), 15-HPETE (15-hydroperoxyeicosatetraenoic acid), and Cyp4a10, which encode monooxygenase to synthesize 20-hydroxyeicosatetraenoic acid (20-HETE). These fatty acids derived from arachidonic acid are incorporated into the β-oxidation process, but they also act as agonists of the expression of the PPARα gene (88), a transcription factor that reduces the inflammatory response by sub-regulation of genes induced by cytokines, an effect attributed to the direct interaction of PPARα with the p65 subunit of NF-kB, reducing its link with DNA (89). This would explain the reduction in the expression of Rel and RelA genes of NF-kB. The absence of differentially expressed genes (Tnfα, Ifn, Il2, Il6, Il8) that code for proinflammatory cytokines and the reduced production of profibrotic factors such as Tgf-β1 (Table 5) would also explain the reduction in the expression of anti-inflammatory interleukin 10 gene in calves (90).
In addition, the activation of the transcription factor PPARα by arachidonic acid or other metabolites derived from arachidonic acid, such as 20-HETE, and the binding with its receptor cis-9 retinoic acid reduced the expression of the Scd1, Nr1h3, Gyk2, and Aqp7 genes, involved in gluconeogenesis, lipogenesis, and cholesterol metabolism, and increased the expression of genes Acsl4, Cyp4a1, Acoxl, Cpt2, and Mmp10, involved in fatty acid transport and oxidation and in adipocyte differentiation (90, 91).
In contrast to PPARα, the downregulation of PPARβ gene transcription is explained for a specific agonist. All PPAR receptors have a ligand-binding domain (LBD), enabling PPAR activation by polar structure ligands, particularly by fatty acids (91). Arachidonic acid (AA) and AA metabolites connect with LBD and act as natural activators of PPARα (92). However, Naruhn et al. (93) identified that 15-HETE strongly induces the expression of PPARβ and, conversely, inhibition of 15-HETE synthesis reduces PPARβ transcriptional activity. In this sense, upregulated genes encode for lipoxygenases that synthetize 12-HPETE and 15-HPETE (arachidonate 12-lipoxygenase and arachidonate 15-lipoxygenase), but microarray did not identify differentially expressed genes that encoded for glutathione peroxidase (Gpx), responsible for the synthesis of 12-HETE and 15-HETE from 12-HPETE and 15-HPETE.
In weaning calves, stress triggers inflammatory responses (94). Prolonged stress suppresses immune functions to enable survival, consequently increasing disease susceptibility (95). Lewis et al. (45) have shown that phosphatidylcholine can stimulate the immune response, resulting in up-regulated PPARα gene activation, modulating the pro-inflammatory response and preventing its excessive activation (91). Optimizing calf health from birth to weaning will impact long-term health and animal welfare (96), allowing the animals to perform better in their first calving and lactation (97).
Conclusions
The polyherbal mixture treatment, at doses of 4 g/d, could improve growth and health status during the pre-ruminant to the weaning period through modification of gene expression. Gene expression analysis confirmed that polyherbal treatment could improve the metabolism of lipids, carbohydrates, proteins, and also immune response. However, more studies are necessary to provide evidence of this aspect. Our results confirm the usefulness of plant compounds in animal feed.
Data Availability Statement
The raw data supporting the conclusions of this article will be made available by the authors, without undue reservation.
Ethics Statement
The animal study was reviewed and approved by the Academic Committee of Doctorado en Ciencias Agropecuarias at Universidad Autónoma Metropolitana- Xochimilco, Mexico City, Mexico.
Author Contributions
CD and AG performed the experiments and wrote section of the manuscript. EM and DM contributed to conception of the study, participated in its design, and helped to draft the manuscript. GM and PH participated in the design of the experiments and performed the statistical analysis. MP carried out the immunoassays. EE and AL participated in the coordination of the fieldwork. LV carried out the animal care. All authors contributed to manuscript revision, read, and approved the submitted version.
Funding
This experiment was supported by the Universidad Autónoma Metropolitana and Universidad Autónoma del Estado de México.
Conflict of Interest
The authors declare that the research was conducted in the absence of any commercial or financial relationships that could be construed as a potential conflict of interest.
Acknowledgments
The authors thank Nuproxa México, S.A., Nuproxa Switzerland, and Indian Herbs Co for donating the herbal products. Thanks, are also extended to Lorena Chávez, Simón Guzmán, and Jorge Ramírez for the analysis of microarrays. DNA microarray unit of the National Laboratory for Technological Support to Genomic Sciences from the UNAM.
References
1. NAHMS, National Animal Health Monitoring System. Dairy Herd Management Practices Focusing on Preweaned Heifers. Fort Collins, CO: USDA, Animal and Plant Health Inspection Service, Veterinary Services (1993).
2. USDA, -APHIS. (2012). Dairy Heifer Raiser 2011: A Study of Operations that Specialize in Raising Dairy Heifers. Fort Collins, CO: USDA-APHIS-VS, CEAH, National Animal Health Monitoring System (NAHMS).
3. Tao S, Dahl GE. Heat stress effects during late gestation on dry cows and their calves. J. Dairy Sci. (2013) 96:4079–93. doi: 10.3168/jds.2012-6278
4. Roland L, Drillich M, Klein-Jöbstl D, Iwersen M. Influence of climatic conditions on the development, performance, and health of calves. J. Dairy Sci. (2016) 99:2438–52. doi: 10.3168/jds.2015-9901
5. Obedat BS, Cobb CJ, Sellers MD, Pepper-Yowell AR, Earleywine TJ, Ballou MA. Plane nutrition during the preweaning period but not the grower phase influences the neutrophil activity of Holstein calves. J. Dairy Sci. (2013) 96:7155–66. doi: 10.3168/jds.2013-6699
6. Jouany JP, Morgavi DP. Use of ‘natural’ products as alternatives to antibiotic feed additives in ruminant production. Animal. (2007) 1:1443–66. doi: 10.1017/S1751731107000742
7. Dutil L, Irwin R, Finley R, Ng LK, Avery B, Boerlin P. Ceftiofur resistance in Salmonella enterica serovar Heidelberg from chicken meat and humans. Can. Emerg. Infect. Dis. (2010) 16:48–54. doi: 10.3201/eid1601.090729
8. Frankic T, Voljc M, Salobir J, Rezar V. Use of herbs and spices and their extracts in animal nutrition. Acta Agric. Slov. (2009) 94:95–102. Available online at: http://aas.bf.uni-lj.si/zootehnika/94-2009/PDF/94-2009-2-95-102.pdf
9. Greathead H. Plants and plant extracts for improving animal productivity. Proc. Nutr. Soc. (2003) 62:279–90. doi: 10.1079/PNS2002197
10. Crosby M, Mendoza-Martínez GD, Relling A, Vázquez VA, Lee-Rangel HA, Martínez JA. Influence of supplemental choline on milk yield, fatty acid profile, and postpartum weight changes in suckling ewes. J. Dairy Sci. (2017) 100:125. doi: 10.1016/S0022-0302(20)30813-4
11. Mendoza GD, Oviedo MF, Pinos JM, Lee-Rangel HA, Vázquez A, Flores R. Milk production in dairy cows supplemented with herbal choline and methionine. Rev. Fac. Cien. Agrar. Univ. Nac. Cuyo. (2019) 1:1853–8665. Available online at: http://revistas.uncu.edu.ar/ojs/index.php/RFCA/article/view/3076
12. Martínez-Aispuro JA, Mendoza GD, Cordero-Mora JL, Ayala-Monter MA, Sánchez-Torres MT, Figueroa-Velasco JL, et al. Evaluation of an herbal choline feed plant additive in lamb feedlot rations. R Bras. Zootec. (2019) 48:1–7. doi: 10.1590/rbz4820190020
13. Lewis ED, Richard C, Goruk S, Dellschaft NS, Curtis JM, Jacobs RL, et al. The form of choline in the maternal diet affects immune development in suckled rat offspring. J. Nutr. (2015) 146:823–30. doi: 10.3945/jn.115.225888
14. Prescott SM, Zimmerman GA, Stafforini DM, McIntyre TM. Platelet-activating factor and related lipid mediators. Annu. Rev. Biochem. (2000) 69:419–45. doi: 10.1146/annurev.biochem.69.1.419
15. McIntyre TM, Prescott SM, Stafforini DM. The emerging roles of PAF acetyl hydrolase. J. Lipid Res. (2009) 50:255–9. doi: 10.1194/jlr.R800024-JLR200
16. Lima FS, Sa-Filho MF, Greco LF, Santos JEP. Effects of feeding rumen-protected choline on incidence of diseases and reproduction of dairy cows. Vet. J. (2012) 193:140–5. doi: 10.1016/j.tvjl.2011.09.019
17. Sun F, Cao Y, Cai C, Li S, Yu C, Yao J. Regulation of nutritional metabolism in transition dairy cows: energy homeostasis and health in response to post-ruminal choline and methionine. PloS ONE. (2016) 11:e0160659. doi: 10.1371/journal.pone.0160659
18. Vailati-Riboni M, Zhou Z, Jacometo CB, Minuti A, Trevisi E, Luchini DN, et al. Supplementation with rumen-protected methionine or choline during the transition period influences whole-blood immune response in periparturient dairy cows. J. Dairy Sci. (2017) 100:3958–68. doi: 10.3168/jds.2016-11812
19. CIOMS. Council for International Organizations of Medical Sciences. International Guiding Principles for Biomedical Research Involving Animals. (2012). Available Online at: https://cioms.ch/wp-content/uploads/2017/01/ResarchInvolvingAnimals.pdf (accessed December 31, 2020).
20. Parish AJ, Bourg MB, Marks LM, Simmons BN, Smith T. Evaluation of different methods of cattle hip height data collection. Prof. Anim. Sci. (2012) 28:292–9. doi: 10.15232/S1080-7446(15)30358-2
21. Fleige S, Pfaf MW. RNA integrity and the effect on the real-time qRT-PCR performance. Mol. Aspects Med. (2006) 27:126–39. doi: 10.1016/j.mam.2005.12.003
22. Sherman TB, Lempicki AR. Systematic and integrative analysis of large gene lists using DAVID bioinformatics resources. Nat. Protoc. (2009) 4:44–57. doi: 10.1038/nprot.2008.211
23. Oliveira DM, Chalfun-Junior A, Chizzotti ML, Barreto HG, Coelho TC, Paiva LV, et al. Expression of genes involved in lipid metabolism in the muscle of beef cattle fed soybean or rumen-protected fat, with or without monensin supplementation. J. Anim. Sci. (2014) 92:5426–36. doi: 10.2527/jas.2014-7855
24. Livak KJ, Schmittgen TD. Analysis of relative gene expression data using real-time quantitative PCR and the 2– ΔΔCT method. Methods. (2001) 25:402–8. doi: 10.1006/meth.2001.1262
25. Mirman D. Growth Curve Analysis and Visualization using R. The R Series. London: Chapman & Hall/CRC Press; Taylor & Francis Group (2016).
26. Steel RGD, Torrie JH. Principles and Procedures of Statistics a Biometrical Approach. 2nd ed. New York, NY: McGraw-Hill Book Co. (1980).
27. Draper NR, Smith H. Applied Regression Analysis. 3rd ed. New York, NY: Wiley (1998). doi: 10.1002/9781118625590
28. Hollenbeck CB. An introduction to the nutrition and metabolism of choline. Cent. Nerv. Syst. Agents Med. Chem. (2012) 12:100–13. doi: 10.2174/187152412800792689
29. Zeisel SH. A brief history of choline. Ann. Nutr. Metab. (2012) 61:254–8. doi: 10.1159/000343120
30. Li Z, Vance DE. Thematic review series: glycerolipids. Phosphatidylcholine and choline homeostasis. J. Lipid Res. (2008) 49:1187–94. doi: 10.1194/jlr.R700019-JLR200
31. Zeisel SH, Da Costa KA. Choline: an essential nutrient for public health. Nutr. Rev. (2009) 67:615–23. doi: 10.1111/j.1753-4887.2009.00246.x
32. NRC. Nutrient Requirements of Beef Cattle: Update 2000. 7th Revised ed. Washington, DC: National Academy of Sciences (2000).
33. Pinotti L, Paltanin C, Campagnoli A, Cavassini P, Dell'Orto V. Rumen protected choline supplementation in beef cattle: effect on growth performance. Ital. J. Anim. Sci. (2009) 8:322–24. doi: 10.4081/ijas.2009.s2.322
34. Bindel DJ, Drouillard JS, Titgemeyer EC, Wessels RH, Loest CA. Effects of ruminally protected choline and dietary fat on performance and blood metabolites of finishing heifers. J. Anim. Sci. (2000) 78:2497–503. doi: 10.2527/2000.78102497x
35. Hajilou M, Dehghan-Banadaky M, Zali A, Rezayazdi K. The effects of dietary L-carnitine and rumen-protected choline on growth performance, carcass characteristics and blood and rumen metabolites of Holstein Young bulls. J. Appl. Anim. Res. (2014) 42:89–96. doi: 10.1080/09712119.2013.822807
36. Hill TM, Aldrich JM, Schlotterbeck RL, Bateman H. Amino Acids, fatty acids, and fat sources for calf milk replacers. Prof. Anim. Sci. (2007) 23:401–8. doi: 10.15232/S1080-7446(15)30995-5
37. Santos FHR, De Paula RM, Lezier D, Silva TJ, Santos G, Bittar CMM. Essential oils for dairy calves: effects on performance, scours rumen fermentation and intestinal fauna. Animal. (2015) 9:958–65. doi: 10.1017/S175173111500018X
38. Froehlich AK, Abdelsalam WK, Chase C, Koppien-Fox J, Casper PD. Evaluation of essential oils and prebiotics for newborn dairy calves. J. Anim. Sci. (2017) 95:3772–82. doi: 10.2527/jas.2017.1601
39. Li H, Wang H, Yu L, Wang M, Liu S, Sun L, et al. Effects of supplementation of rumen-protected choline on growth performance, meat quality and gene expression in longissimus dorsi muscle of lambs. Arch. Anim. Nutr. (2015) 69:340–50. doi: 10.1080/1745039X.2015.1073001
40. Pino F, Urrutia NL, Gelsinger SL, Gehman AM, Heinrichs AJ. Long-term effect of organic trace minerals on growth, reproductive performance, and first lactation in dairy heifers. Prof. Anim. Sci. (2018) 34:51–8. doi: 10.15232/pas.2017-01680
41. Jiang X, Liu X, Liu S, Li Y, Zhao HB, Zhang YG. Growth, rumen fermentation and plasma metabolites of Holstein male calves fed fermented corn gluten meal during the postweaning stage. Anim. Feed Sci. Technol. (2019) 249:1–9. doi: 10.1016/j.anifeedsci.2019.01.012
42. Saldana DJ, Jones CM, Gehman AM, Heinrichs AJ. Effects of once-versus twice-a-day feeding of pasteurized milk supplemented with yeast-derived feed additives on growth and health in female dairy calves. J. Dairy Sci. (2019) 102:3654–60. doi: 10.3168/jds.2018-15695
43. Zhu J, Wu Y, Tang Q, Leng Y, Cai W. The effects of choline on hepatic lipid metabolism, mitochondrial function and antioxidative status in human hepatic C3A cells exposed to excessive energy substrates. Nutrients. (2014) 6:2552–71. doi: 10.3390/nu6072552
44. Yilmaz T, Koçcan EG, Besler HT, Yilmaz G, Gürsel B. The role of oxidants and antioxidants in otitis media with effusion in children. Otolaryngol. Head Neck Surg. (2004) 131:797–803. doi: 10.1016/j.otohns.2004.07.001
45. Lewis ED, Goruk S, Richard C, Dellschaft NS, Curtis JM, Jacobs RL, et al. Feeding a diet devoid of choline to lactating rodents restricts growth and lymphocyte development in offspring. Br. J. Nutr. (2016) 116:1001–12. doi: 10.1017/S0007114516002919
46. Teixeira AGV, Lima FS, Bicalho MLS, Kussler A, Lima SF, Felippe MJ, et al. Effect of an injectable trace mineral supplement containing selenium, copper, zinc, and manganese on immunity, health, and growth of dairy calves. J. Dairy Sci. (2014) 97:4216–26. doi: 10.3168/jds.2013-7625
47. Lindholm-Perry AK, Kuehn LA, McDaneld TG, Miles JR, Workman AM, Chitko-McKown CG, et al. Complete blood count data and leukocyte expression of cytokine genes and cytokine receptor genes associated with bovine respiratory disease in calves. BMC Res. Notes. (2018) 11:786. doi: 10.1186/s13104-018-3900-x
48. Knowles GT, Edwards EJ, Bazeley JK, Brown NS, Butterworth A, Warriss P. Changes in blood biochemical and hematological profile of neonatal calves with age. Vet. Rec. (2000) 147:593–8. doi: 10.1136/vr.147.21.593
49. Khosla P, Bhanwra S, Singh J, Seth S, Srivastava RK. A study of hypoglycemic effects of Azadirachta indica (Neem) in normal and alloxan diabetic rabbits. Indian J. Physiol. Pharmacol. (2000) 44:69–74. Available online at: https://ijpp.com/IJPP%20archives/2000_44_1/69-74.pdf
50. Zhao H, Raines LN, Huang SC. Carbohydrate and amino acid metabolism as hallmarks for innate immune cell activation and function. Cells. (2020) 9:562. doi: 10.3390/cells9030562
51. Jezek J, Klopcic M, Klinkon M. Influence of age on biochemical parameters in calves. Bull. Vet. Inst. Pulawy. (2006) 50:211–4. Available online at: http://www.piwet.pulawy.pl/bulletin/images/stories/pdf/20062/20062211214.pdf
52. Nozad S, Ramin GA, Rezaie AS. Diurnal variations in milk macro-mineral concentrations in Holstein dairy cows in Urmia, Iran. Vet. Res. Forum. (2012) 3:281–5. doi: 10.2298/AVB1101003N
53. Issi M, Gül Y, Başbug O. Evaluation of renal and hepatic functions in cattle with subclinical and clinical ketosis. Turk. J. Vet. Anim. Sci. (2016) 40:47–52. doi: 10.3906/vet-1505-16
54. Eggum OB, Juliano OB, Maningat CC. Protein and energy utilization of rice milling fractions by rats. Plant Food Hum. Nutr. (1982) 31:371–6. doi: 10.1007/BF01094048
55. Dufour DR, Lott JA, Nolte FS, Gretch DR, Koff RS, Seeff LB. Diagnosis and monitoring of hepatic injury. I. Performance characteristics of laboratory tests. Clin. Chem. (2000) 46:2027–49. doi: 10.1093/clinchem/46.12.2027
56. Rowland A, Miners JO, Mackenzie PI. The UDP-glucuronosyltransferases: their role in drug metabolism and detoxification. Int. J. Biochem. Cell Biol. (2013) 45:1121–32. doi: 10.1016/j.biocel.2013.02.019
57. Mohamed MEF, Frye RF. Effects of herbal supplements on drug glucuronidation. Review of clinical, animal, and in vitro studies. Planta Med. (2011) 77:311–21. doi: 10.1055/s-0030-1250457
58. Biswas S, Giri S. Importance of choline as essential nutrient and its role in prevention of various toxicities. Prague Med. Rep. (2015) 116:5–15. doi: 10.14712/23362936.2015.40
59. Mehedint MG, Zeisel SH. Choline's role in maintaining liver function: new evidence for epigenetic mechanisms. Curr. Opin. Clin. Nutr. Metab. Care. (2013) 16:339–45. doi: 10.1097/MCO.0b013e3283600d46
60. Leach RJ, Chitko-McKown CG, Bennett GL, Jones SA, Kachman SD, Keele JW, et al. The change in differing leukocyte populations during vaccination to bovine respiratory disease and their correlations with lung scores, health records, and average daily gain. J. Anim. Sci. (2013) 91:3564–73. doi: 10.2527/jas.2012-5911
61. Roland L, Drillich M, Iwersen M. Hematology as a diagnostic tool in bovine medicine. J. Vet. Diagn. Invest. (2014) 26:592–8. doi: 10.1177/1040638714546490
62. Benesi FJ, Teixeira C, Leal ML, Lisboa JA, Mirandola R, Shecaira CL, et al. Leukograms of healthy Holstein calves within the first month of life. Pesq. Vet. Bras. (2012) 32:352–6. doi: 10.1590/S0100-736X2012000400013
63. Honda T, Uehara T, Matsumoto G, Arai S, Sugano M. Neutrophil left shift and white blood cell count as markers of bacterial infection. Clin. Chim. Acta. (2016) 457:46–53. doi: 10.1016/j.cca.2016.03.017
64. Cunliffe RN. Alpha-defensins in the gastrointestinal tract. Mol. Immunol. (2003) 40:463–7. doi: 10.1016/S0161-5890(03)00157-3
65. Naseer O, Khan JA, Khan MS, Omer MO, Chishti GA, Sohail ML, et al. Comparative efficacy of silymarin and choline chloride (liver tonics) in preventing the effects of aflatoxin B1 in bovine calves. Pol. J. Vet. Sci. (2016) 19:545–51. doi: 10.1515/pjvs-2016-0068
66. Hansson GC. Mucus and mucins in diseases of the intestinal and respiratory tracts. J. Intern Med. (2019) 285:479–90. doi: 10.1111/joim.12910
67. Ulluwishewa D, Anderson R, McNabb W, Moughan P, Wells J, Roy N. Regulation of tight junction permeability by intestinal bacteria and dietary components. J. Nutr. (2011) 141:769–76. doi: 10.3945/jn.110.135657
68. Edward JH. Clinical laboratory evaluation of small intestinal function. Vet. Clin. North Am. Small Anim. Pract. (1999) 29:441–69. doi: 10.1016/S0195-5616(99)50030-2
69. Malmuthuge N, Li M, Goonewardene AL, Oba M, Guan LL. Effect of calf starter feeding on gut microbial diversity and expression of genes involved in host immune responses and tight junctions in dairy calves during weaning transition. J. Dairy Sci. (2013) 96:3189–200. doi: 10.3168/jds.2012-6200
70. Rock CO, Calder RB, Karim MA, Jackowski S. Pantothenate kinase regulation of the intracellular concentration of coenzyme A. J. Biol. Chem. (2000) 275:1377–83. doi: 10.1074/jbc.275.2.1377
71. Li Y, Chang Y, Zhan L, Feng Q, Liu Z, Zhang Y, et al. High glucose upregulates pantothenate kinase 4 (PanK4) and thus affects M2-type pyruvate kinase (Pkm2). Mol. Cell Biochem. (2005) 277:117–25. doi: 10.1007/s11010-005-5535-1
72. Wang L, Waltenberger B, Pferschy-Wenzig EM, Blunder M, Liu X, Malainer C, et al. Natural product agonists of peroxisome proliferator-activated receptor gamma (PPARγ): a review. Biochem. Pharmacol. (2014) 92:73–89. doi: 10.1016/j.bcp.2014.07.018
73. Slenter DN, Kutmon M, Hanspers K, Riutta A, Windsor J, Nunes N, et al. WikiPathways: a multifaceted pathway database bridging metabolomics to other omics research. Nucleic Acids Res. (2017) 46:661–7. doi: 10.1093/nar/gkx1064
74. Lee HJ, Jang M, Kim H, Kwak W, Park W, Hwang JY, et al. Comparative transcriptome analysis of adipose tissues reveals that ECM-receptor interaction is involved in the depot-specific adipogenesis in cattle. PLoS ONE. (2013) 8:e66267. doi: 10.1371/journal.pone.0066267
75. Yoshihama M, Uechi T, Asakawa S, Kawasaki K, Kato S, Higa S, et al. The human ribosomal protein genes: sequencing and comparative analysis of 73 genes. Genome Res. (2002) 12:379–90. doi: 10.1101/gr.214202
76. Korte M, Schmitz D. Cellular and system biology of memory: timing, molecules, and beyond. Physiol. Rev. (2016) 96:647–93. doi: 10.1152/physrev.00010.2015
77. Musazzi L, Treccani G, Mallei A, Popoli M. The action of antidepressants on the glutamate system: regulation of glutamate release and glutamate receptors. Biol. Psychiatry. (2013) 73:1180–8. doi: 10.1016/j.biopsych.2012.11.009
78. Zhang J, Hochwald SN. The role of FAK in tumor metabolism and therapy. Pharmacol. Therapeut. (2014) 142:154–63. doi: 10.1016/j.pharmthera.2013.12.003
79. Liu B, Xu L, Yu X, Jiao X, Yan J, Li W, et al. Genistein inhibited estradiol-induced vascular endothelial cell injury by downregulating the FAK/Focal adhesion pathway. Cell Physiol. Biochem. (2018) 49:2277–92. doi: 10.1159/000493830
80. Zhao B, Li L, Lei Q, Guan KL. The Hippo–YAP pathway in organ size control and tumorigenesis: an updated version. Genes Dev. (2010) 24:862–74. doi: 10.1101/gad.1909210
81. Serviddio G, Giudetti AM, Bellanti F, Priore P, Rollo T, Tamborra R, et al. Oxidation of Hepatic Carnitine Palmitoyl Transferase-I (CPT-I) impairs fatty acid beta-oxidation in rats fed a methionine-choline deficient diet. PLoS ONE. (2011) 6:e24084. doi: 10.1371/journal.pone.0024084
82. Goselink RMA, Van Baal J, Widjaja HCA, Dekker RA, Zom RLG, De Veth MJ, et al. Effect of rumen-protected choline supplementation on liver and adipose gene expression during the transition period in dairy cattle. J. Dairy Sci. (2013) 96:1102–16. doi: 10.3168/jds.2012-5396
83. Fritsche K. Fatty acids as modulators of the immune response. Annu. Rev. Nutr. (2006) 26:45–73. doi: 10.1146/annurev.nutr.25.050304.092610
84. Connor EE, Baldwin RL, Li CJ, Li RW, Chung H. Gene expression in bovine rumen epithelium during weaning identifies molecular regulators of rumen development and growth. Funct. Integr. Genomics. (2013) 13:133–42. doi: 10.1007/s10142-012-0308-x
85. Naeem A, Drackley JK, Stamey J, Loor JJ. Role of metabolic and cellular proliferation genes in ruminal development in response to enhanced plane of nutrition in neonatal Holstein calves. J. Dairy Sci. (2012) 95:1807–20. doi: 10.3168/jds.2011-4709
86. Bionaz M, Chen S, Khan JM, Loor JJ. Functional role of PPARs in ruminants: potential targets for fine-tuning metabolism during growth and lactation. PPAR Res. (2013) 684159:28. doi: 10.1155/2013/684159
87. Varga T, Czimmerer Z, Nagy L. PPARs are a unique set of fatty acid regulated transcription factors controlling both lipid metabolism and inflammation. Biochim. Biophys. Acta Mol. Basis Dis. (2011) 1812:1007–22. doi: 10.1016/j.bbadis.2011.02.014
88. Chinetti G, Fruchart CJ, Staels B. Peroxisome proliferator-activated receptors (PPARs): nuclear receptors at the crossroads between lipid metabolism and inflammation. Inflamm. Res. (2000) 49:497–505. doi: 10.1007/s000110050622
89. Zuñiga J, Cancino M, Medina F, Varela P, Vargas R, Tapia G, et al. N-3 PUFA supplementation triggers PPAR-α activation and PPAR-α/NF-kB interaction: anti-inflammatory implications in liver ischemia-reperfusion injury. PloS ONE. (2011) 6:e28502. doi: 10.1371/journal.pone.0028502
90. Subramanian V, Seemann I, Merl-Pham J, Hauck MS, Stewart AF, Atkinson JM, et al. Role of TGF Beta and PPAR alpha signaling pathways in radiation response of locally exposed heart: integrated global transcriptomics and proteomics analysis. J. Proteome Res. (2017) 16:307–18. doi: 10.1021/acs.jproteome.6b00795
91. Korbecki J, Bobiński R, Dutka M. Self regulation of the inflammatory response by peroxisome proliferator activated receptors. Inflamm. Res. (2019) 68:443–58. doi: 10.1007/s00011-019-01231-1
92. Barry OP, Kazanietz MG, Praticò D, FitzGerald GA. Arachidonic acid in platelet microparticles up-regulates cyclooxygenase-2-dependent prostaglandin formation via a protein kinase C/mitogen-activated protein kinase-dependent pathway. J. Biol. Chem. (1999) 274:7545–56. doi: 10.1074/jbc.274.11.7545
93. Naruhn S, Maissner W, Adhikary T, Kaddatz K, Klein T, Watzer B, et al. 15-hydroxyeicosatetraenoic acid is a preferential peroxisome proliferator-activated receptor β/δ agonist. Mol. Pharmacol. (2010) 77:171–84. doi: 10.1124/mol.109.060541
94. Martin LB. Stress and immunity in wild vertebrates: timing is everything. Gen. Comp. Endocrinol. (2009) 163:70–6. doi: 10.1016/j.ygcen.2009.03.008
95. Salak-Johnson JL, McGlone JJ. Making sense of apparently conflicting data: stress and immunity in swine and cattle. J. Anim. Sci. (2007) 85:E81–8. doi: 10.2527/jas.2006-538
96. Hulbert LE, Moisá SJ. Stress, immunity, and the management of calves. J. Dairy Sci. (2016) 99:3199–216. doi: 10.3168/jds.2015-10198
Keywords: growth performance, Holstein calves, animal nutrition, nutrigenomics, feed plant additive
Citation: Díaz Galván C, Méndez Olvera ET, Martínez Gómez D, Gloria Trujillo A, Hernández García PA, Espinosa Ayala E, Palacios Martínez M, Lara Bueno A, Mendoza Martínez GD and Velázquez Cruz LA (2021) Influence of a Polyherbal Mixture in Dairy Calves: Growth Performance and Gene Expression. Front. Vet. Sci. 7:623710. doi: 10.3389/fvets.2020.623710
Received: 30 October 2020; Accepted: 21 December 2020;
Published: 26 January 2021.
Edited by:
Ahmed E. Kholif, National Research Center, EgyptReviewed by:
Peter Erickson, University of New Hampshire, United StatesTarek Abdelfattah Morsy, National Research Center, Egypt
Copyright © 2021 Díaz Galván, Méndez Olvera, Martínez Gómez, Gloria Trujillo, Hernández García, Espinosa Ayala, Palacios Martínez, Lara Bueno, Mendoza Martínez and Velázquez Cruz. This is an open-access article distributed under the terms of the Creative Commons Attribution License (CC BY). The use, distribution or reproduction in other forums is permitted, provided the original author(s) and the copyright owner(s) are credited and that the original publication in this journal is cited, in accordance with accepted academic practice. No use, distribution or reproduction is permitted which does not comply with these terms.
*Correspondence: Estela Teresita Méndez Olvera, ZXRtZW5kZXomI3gwMDA0MDtjb3JyZW8ueG9jLnVhbS5teA==