- Jiangxi Province Key Laboratory of Animal Nutrition/Engineering Research Center of Feed Development, College of Animal Science and Technology, Jiangxi Agricultural University, Nanchang, China
The experiment was conducted to investigate the effects of dietary supplementation with Puerarin on meat quality, muscle antioxidant ability, and muscle fiber characteristics of beef cattle under a hot environment in summer. Thirty-two 15 ± 1.5-month-old Jinjiang bulls (291.65 ± 8.84 kg) were randomly divided into four groups with dietary Puerarin at 0 (control), 200 (Pue200), 400 (Pue400), and 800 (Pue800) mg/kg in the feed concentrate (n = 8). The feeding trial lasted for 60 days after a 10-day adaptation period (July 1–September 8); the average values of temperature, relative humidity and temperature, and humidity index were 30.68°C, 68.05%, and 81.81, respectively. The growth performance on day 40 of the experiment period was calculated. After 60 days' experimental period, four Jinjiang cattle per treatment from the control group, Pue400 group, and Pue800 group were slaughtered. Compared with the control group, the Pue400 and Pue800 groups improved the growth performance of beef cattle; the Pue800 group elevated the activities of superoxide dismutase, total antioxidant capacity, and glutathione peroxidase in the M. longissimus thoracis (LT) muscle. In the control group, the cell membrane was incomplete, and most of the mitochondria were elongated and in a fission state, while in the Pue400 and Pue800 groups, the cell membrane was clear and complete, and the mitochondria presented with round and oval shapes. Compared with the control group, the Pue400 and Pue800 groups reduced the shear force of the LT muscle, and the Pue400 group decreased the muscle fiber diameter and the myosin heavy-chain (MyHC)-IIb gene expression. Furthermore, the Pue400 and Pue800 groups decreased the ratio of AMP/ATP, the Pue800 group reduced the AMP-activated protein kinase α2 mRNA expression, and the Pue400 group improved the nuclear respiratory factor 1 mRNA expression. These results indicated that dietary supplementation with Puerarin might be beneficial to the meat quality of heat-stressed beef cattle by improving muscle antioxidant ability and reducing the MyHC-IIb muscle fiber composition. Based on the results of this study, we recommended 400 mg/kg Puerarin in the feed concentrate of beef cattle (~300 kg) for mitigation of heat stress.
Introduction
Heat stress caused by high temperature is one of the most critical environmental stressors challenging cattle production (1), particularly during the hot-humid season in subtropical regions such as South China. The oxidative stress induced by heat stress can impair cell membrane and mitochondrial integrity through lipid peroxidation, which adversely affects the muscle protein functionality and the sensory, nutritional, and shelf-life quality of animal products (2, 3). Moreover, muscle fiber is an important part of the muscle; the quantity and type of muscle fiber are the keys to determine meat quality. Generally, muscle fiber is classified into four categories: myosin heavy-chain (MyHC)-I (slow-oxidative), MyHC-IIa (fast-oxidative), MyHC-IIx (intermediary to MyHC-IIa and IIb), and MyHC-IIb (fast-glycolytic) (4). They can influence meat quality due to their different activities of glycolytic enzymes and contents of glycogen and lipid (5). Notably, previous studies showed that acute or chronic heat stress resulted in decreased slow muscle fiber composition and ultimately led to poor meat quality (6, 7). Therefore, heat stress mitigation is a significant demand for beef cattle welfare and an important guarantee for high-quality beef production.
Unraveling the heat stress-responsive mechanisms in beef cattle is of great importance to the development of active agents against heat stress. AMP-activated protein kinase (AMPK) is a serine/threonine kinase that plays a crucial role in regulating cellular and whole-body energy homeostasis under physiological stress conditions (8). When the body suffers from heat stress, ATP consumption increases, subsequently resulting in elevation of AMP/ATP ratio and further activating AMPK via phosphorylation of αThr172 (9, 10). AMPK can also be activated by cellular reactive oxygen species (ROS) and intracellular calcium (11). Once activated, AMPK phosphorylates a range of substrates to preserve energy expenditure by switching from anabolic to catabolic pathway (8). Among all the AMPK-mediated regulation pathways of energy homeostasis maintenance in response to stress, what meat scientists valued most is the regulation of skeletal muscle energy metabolism (3). Moreover, the activation of AMPK has been proven to cause muscle to assume a faster-contracting and more glycolytic property (12). Therefore, regulating the activity of AMPK may effectively relieve the negative impact of heat stress on beef quality.
Puerarin is a natural active isoflavone extracted from the traditional Chinese medicine (TCM) Radix Puerarin and has a wide range of functions, including anti-oxidative, anti-inflammation, and anti-apoptosis (13, 14). It has been reported that Puerarin could attenuate heat stress by suppressing ROS production and upregulating heat shock protein-72 expression in bovine Sertoli cells (15). Besides, Puerarin could alleviate autophagy by inhibiting the AMPK–mammalian target of rapamycin (mTOR)–Unc 51 like autophagy activating kinase 1 (ULK1) signaling pathway (16). A previous study showed that Puerarin was used as a superior animal feed additive, natural health food, and effective medicine in Asian countries (17). However, little attention has been paid to Puerarin's improvement effects on the meat quality of heat-stressed beef cattle, and whether this improvement is associated with the changes in muscle antioxidant ability and muscle fiber characteristics is still unclear. Therefore, this experiment was intended to study the effects of Puerarin on the meat quality, muscle antioxidant ability, muscle fiber characteristics, and AMPK pathway of beef cattle under high temperature in summer.
Materials and Methods
This experiment was approved by the Committee for the Care and Use of Experimental Animals at Jiangxi Agricultural University (JXAULL-20190015).
Preparation of Puerarin
Puerarin preparation (purity N 98.1%) was provided by the Department of Pharmaceutical Chemistry, Guangxi Medical University (Nanning, China). Puerarin is a white powder extracted from R. Puerarin. In these experimental diets, Puerarin was first mixed with the premix in a certain proportion and then formulated into a concentrate.
Animal Treatments and Experimental Diets
Thirty-two 15 ± 1.5-month-old healthy and uncastrated Jinjiang bulls (291.65 ± 8.84 kg) were randomly divided into four groups. Jinjiang cattle are a breed of Chinese indigenous beef cattle that are bred in the northwest of the Jiangxi Province. Dietary treatments were basal diet without any additive (control), basal diet + 200 mg/kg Puerarin in the feed concentrate (Pue200), basal diet + 400 mg/kg Puerarin in the feed concentrate (Pue400), and basal diet + 800 mg/kg Puerarin in the feed concentrate (Pue800). Each treatment consisted of eight replicates (pen) with one beef cattle per pen. Animals were housed indoors with solid concrete floor pens (1.25 m × 2 m). The feeding trial lasted for 60 days after a 10-day adaptation period (July 1–September 8; the average values of temperature, relative humidity and temperature, and humidity index were 30.68°C, 68.05%, and 81.81, respectively). According to the Chinese Feeding Standard for beef cattle (NY/T 815-2004), the basal diet was designed and met the nutrient requirements of 0.5 kg daily gain of 300 kg male cattle. The ingredient composition and nutrient levels of the basal diet were shown in Table 1. In this study, the level of Puerarin supplemented in the basal diet was referred to the study of Zhao et al. (18), who reported that the crossbred steers (Chinese Yellow × Augus, 565.2 ± 31.2 kg) fed a 90% concentrate diet supplemented with daidzein (500 mg/kg concentrate). Puerarin is a structural analog of daidzein. Based on these data, it can be calculated that the equal amount of Puerarin is 908 mg/kg basal diet in 565 kg of beef cattle. Therefore, we hypothesized that 291 kg of Jinjiang cattle could be fed 468 mg/kg Puerarin in basal diet. No antibiotic was included in the diets. The diet was provided twice daily (06:00, 15:30). All animals were offered feed and water ad libitum.
Growth Performance
Due to this study being conducted to investigate the effects of heat stress on meat quality of beef cattle, we recorded final body weight on day 40 of the experiment period to avoid the pre-slaughter stress induced by weighing. Therefore, the initial body weight (on the first day of the experiment period), final body weight (on day 40 of the experiment period), and dry matter intake of beef cattle in each replicate were recorded to calculate average daily gain (ADG), average dry matter intake (ADMI), and the ratio of feed to gain (F/G). ADG = (final body weights per replicate – initial body weights per replicate)/40 days, ADMI = dry matter intake per replicate/40 days, F/G = ADMI/ADG.
Sample Collection
At the end of the experiment, based on the earlier period data (day 1–40 of the experiment period) of growth performance, we found that the Pue200 group reduced the ADG and ADMI of beef cattle numerically compared with the control group, while the Pue400 and Pue800 groups significantly improved the ADG and ADMI of beef cattle. Therefore, four beef cattle per treatment with one cattle per pen with medium body weight were selected from the control, Pue400, and Pue800 groups for further analyses. A total of 12 beef cattle were gently led to the slaughterhouse on foot to avoid stress (the distance between feedlot and slaughterhouse was 0.7 km). At the day of slaughter, the average temperature and humidity index (THI) was 78.3.
All animals were sacrificed via electrical stunning, followed by jugular vein exsanguination. Immediately, the Musculus longissimus thoracis (LT) muscle sampled at the last rib of the left carcass was placed in liquid nitrogen to analyze the antioxidant index, adenosine phosphates, and the expression of genes mRNA. Then, the LT muscle samples were cut into shaped strips (1 cm × 1 cm × 1 cm), parallel to the muscle fiber direction, and placed in a 4% formaldehyde solution for the analysis of muscle fiber morphology. The other LT muscle samples were cut into shaped strips (1 mm × 1 mm × 1 mm), parallel to the muscle fiber direction, placed in a pre-cooled 2.5% glutaraldehyde for 2 h, and stored in the refrigerator at 4°C until for the analysis of skeletal muscle ultrastructure. At 30-min postmortem, the LT muscles from the last rib of the left carcass were removed, trimmed of subcutaneous fat and connective tissue, and vacuum-packed at 4°C for the measurement of meat quality.
Antioxidant Index Analyses
For biochemical assays, 0.5 g frozen LT muscle samples were ice bath homogenized in 4.5 ml ice-cold physiological saline for 1 min and then centrifuged (2,700 g, 4°C, 10 min). The supernatants were then subjected to the measurements of the content of malondialdehyde (MDA), the activities of superoxide dismutase (SOD) and glutathione peroxidase (GSH-PX), the total antioxidant capacity (T-AOC). The concentration of total protein in the LT muscle was determined by TP kit (Nanjing Jiancheng Bioengineering Institute, Nangjing, China) according to the instructions of the manufacturer. All of those antioxidant indices in the LT muscle were determined by commercial MDA, SOD, GSH-PX, and T-AOC kits (Nanjing Jiancheng Bioengineering Institute) and were normalized by total protein concentration in the LT muscle, respectively, according to the instructions of the manufacturer. The ROS content was determined by ROS kit (Nanjing Jiancheng Bioengineering Institute, Nangjing, China) according to the instructions of the manufacturer. The 2′,7′-dichlorofluorescin diacetate (DCFH-DA) is the most commonly used sensitive ROS probe.
Meat Quality
Muscle pH45min and pH24h were measured by using a pH electrode (HI99163N, Hanna, Padova, Italy). Each chop was measured three times at different areas, and the average value was obtained.
Meat color was measured using a spectrocolorimeter (WSC-S, Shanghai, China) at 24 h postmortem. Mean CIE L* (lightness), a* (redness), and b* (yellowness) values were collected from three different locations of the chops using freshly cut surface after being exposed to air for 20 min.
For the determination of cooking loss, at 48 h postmortem, samples of LT muscle (6 cm × 6 cm × 4 cm) were vacuum-packed in individual polyethylene vacuum bags and cooked in a water bath at 80°C to reach an internal temperature of 70°C. Then, the samples within their packaging bags were cooled in running water to room temperature, wiped with absorbent paper to remove residual moisture, and reweighed to calculate cooking loss. Then, the cooked samples were cut into shaped strips (1 cm × 1 cm × 3 cm), parallel to the muscle fiber direction. Warner–Bratzler shear force was measured using a C-LM3B shear apparatus (Northeast Agricultural University, Harbin, China) with a load cell of 15 kg and a crosshead speed of 200 mm/min. Six replicates of each sample were measured.
Muscle Fiber Morphology
The LT muscle samples in 4% formaldehyde solution were taken out and then dehydrated, paraffin-embedded, sliced, hematoxylin–eosin-stained, and sealed. The sections were taken photographs with a microscope (Motic BA210; Motic Medical Diagnostic Systems, Co., Ltd., Xiamen, China) at a magnification of 400×. Motic Images Advanced 3.2 software was used to analyze pictures, including muscle fiber diameter and density. Five views were captured in each section.
Transmission Electron Microscopy
The LT muscle samples in 2.5% glutaraldehyde were taken out and then washed with 0.1 mol/L phosphate buffer followed by postfixation of 1% osmium tetraoxide. Samples were then washed with 0.1 mol/L phosphate buffer again, dehydrated by gradient of increasing concentrations of alcohol, embedded with epon resin, and sliced. Transmission electron microscopy (TEM) images were obtained on a Hitachi H-7100 transmission electron microscope.
Adenosine Phosphate Analyses
The levels of adenosine phosphates (ATP, ADP, and AMP) in the LT muscle were determined by high-performance liquid chromatography (HPLC) according to the method of Li et al. (19). Briefly, 0.5 g of frozen muscle sample was homogenized in 2.5 ml of 7% ice-cold perchloric acid at 13,500 rpm for 30 s in an ice bath and then centrifuged (15,000 × g, 4°C, 10 min). The supernatant (850 μl) was then neutralized with 0.85 M KOH (850 μl) and centrifuged again (15,000 × g, 4°C, 10 min) to remove KClO4. The neutralized supernatant was filtered through a 0.45-μm filter before injection into a Waters-2695 Alliance HPLC system (Waters, Milford, MA, USA). The column was a Kromasil 5 μm C18, 250 × 4.6 mm (Feinano, Tianjin, China). The chromatographic conditions were as follows: mobile phase A, HPLC grade methanol; mobile phase B, phosphate buffer (2.5 mM tetra-butylammonium hydrogen sulfate, 0.04 M potassium dihydrogen orthophosphate, 0.06 M dipotassium hydrogen orthophosphate, pH 7.0), filtered through a 0.45-μm membrane; mobile A/mobile B, 13.5%/86.5%. The column temperature was set at 30°C, the injection volume was 10 μl, and UV detection was at 254 nm. The characteristic running time was 15 min, the flow rate was maintained at 1.0 ml/min, and sample measurement was set to auto-sequence injection. Peaks were identified and quantified using standard curves.
Total RNA Isolation and mRNA Expression Analyses
Total RNA was isolated from the LT sample using the phenol and guanidine isothiocyanate-based TRIzol reagent (Invitrogen, USA) according to the manufacturer's protocol. The purity and quantity of total RNA were measured by a NanoDrop 1000 spectrophotometer (Thermo Scientific, Wilmington, DE, USA) at 260 and 280 nm. Total RNA was treated with DNase I (Takara Biotechnology Co. Ltd., Dalian, China) to remove DNA and transcribed to cDNA using a PrimeScript RT Master Mix kit (Takara Biotechnology Co. Ltd., China) following the manufacturer's instructions. The mRNA expressions were determined and calculated according to the method of Li et al. (20). Briefly, real-time PCR was carried out in optical 96-well plates on an ABI 7500 Real-Time PCR System (Applied Biosystems, Foster City, CA, USA) and SYBR Premix Ex Taq Kit (Takara Biotechnology Co., Ltd., China). Primers used for real-time PCR are presented in Table 2 and were synthesized by Invitrogen. The amplification was performed in a total volume of 20 μl, containing 10 μl of SYBR Premix Ex Taq, 0.4 μl of each primer (10 μM), 6.8 μl of sterilized double-distilled water, and 2 μl of cDNA. The program was as follows: 95°C for 30 s, followed by 40 cycles of 95°C for 5 s, 58°C for 31 s, and 70°C for 30 s, and collected the fluorescence signal at 58°C. The amplification of the glyceraldehyde-3-phosphate dehydrogenase (GAPDH) housekeeping genes was used for each sample to normalize the expression of the selected genes. Relative gene expression was calculated using the 2−ΔΔCt method.
Statistical Analyses
All data analyses were statistically analyzed by one-way ANOVA with SPSS statistical software (Ver. 20 for windows, SPSS). All data were normally distributed via the Shapiro–Wilk test. Tukey multiple range test was used to compare differences among the treatment groups. The level of statistical significance was present at P < 0.05. Values were expressed as mean ± SE.
Results
Growth Performance
As shown in Table 3, compared with the control and Pue200 group, dietary supplementation with 400 and 800 mg/kg Puerarin improved the ADG of beef cattle (P < 0.05). The ADMI of beef cattle in the Pue800 group was higher than that in the control group (P < 0.05). However, there was no difference in the initial and final body weights and F/G of cattle among all treatments.
Antioxidant Index
As presented in Table 4, the Pue800 group tended to reduce the level of ROS compared with the control group (P = 0.051). The SOD activity in the Pue400 and Pue800 groups was higher than that in the control group (P < 0.05). In comparison to the control group, the Pue800 group increased the activities of T-AOC and GSH-PX in the LT muscle (P < 0.05). However, an insignificant effect was presented on the MDA content.
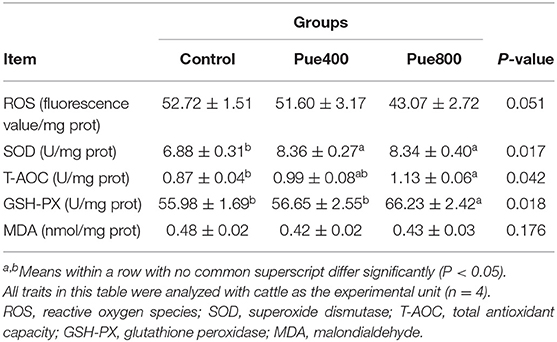
Table 4. Effects of Puerarin on antioxidant index in M. longissimus thoracis of beef cattle under hot environment.
Transmission Electron Microscopy
As shown in Figure 1, in the control group, the skeletal muscle cell boundaries were not clearly visible and had an incomplete membrane. Besides, the mitochondrial structure was deformed, most of the mitochondria were elongated and in a fission state. In the Pue400 and Pue800 groups, the cell membrane was clear and complete, and the mitochondrial structure was standard, which presented with round and oval shapes. The mitochondrial cristae in the Pue400 group were normal with clear structures, whereas this was not the case in the Pue800 group.
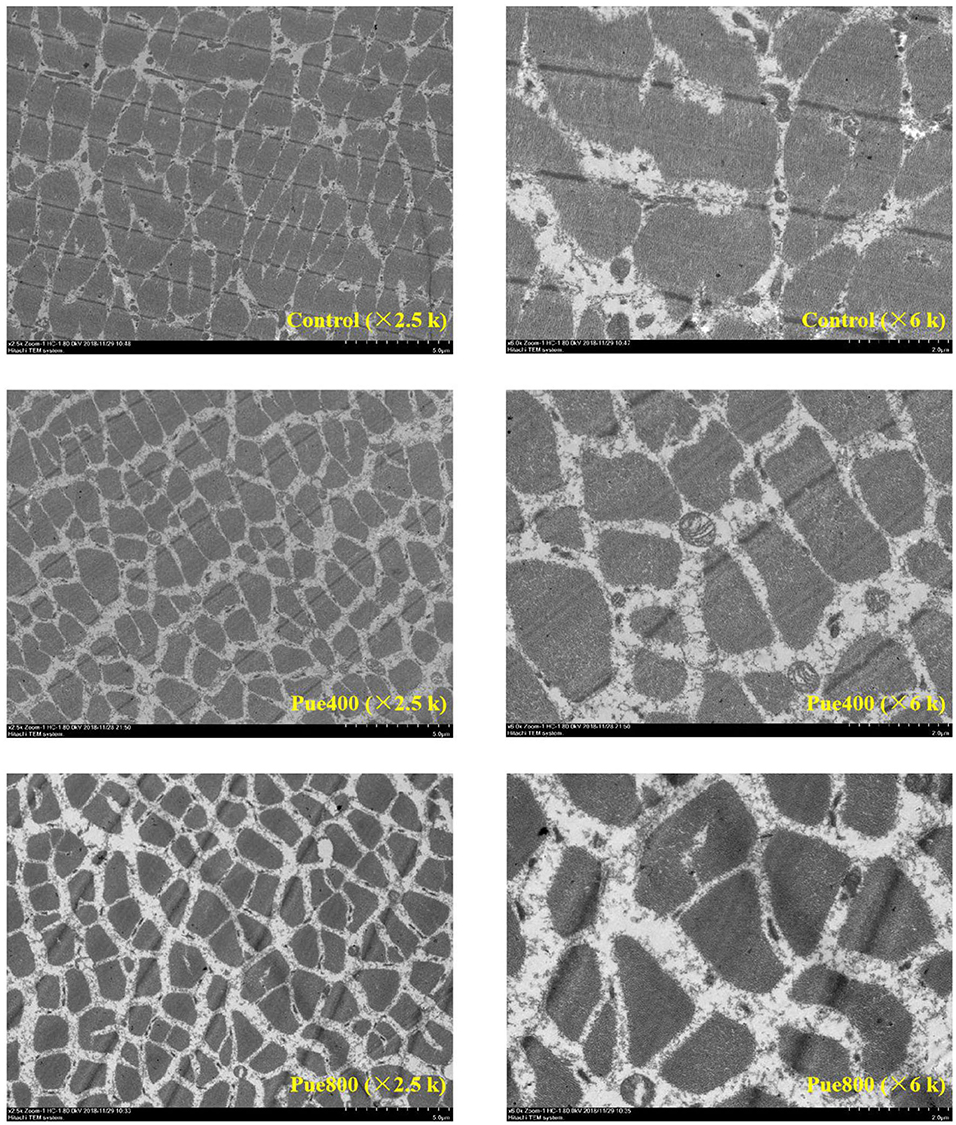
Figure 1. Effects of Puerarin on the skeletal muscle ultrastructure in M. longissimus thoracis of beef cattle under hot environment.
Meat Quality
As shown in Table 5, diet supplemented with 400 and 800 mg/kg Puerarin reduced the shear force of LT muscle compared with the control group (P < 0.05), while there was no significant difference in pH45min value, pH24h value, lightness (L*) values, redness (a*) values, yellowness (b*) values, and cooking loss of the LT muscle among groups.
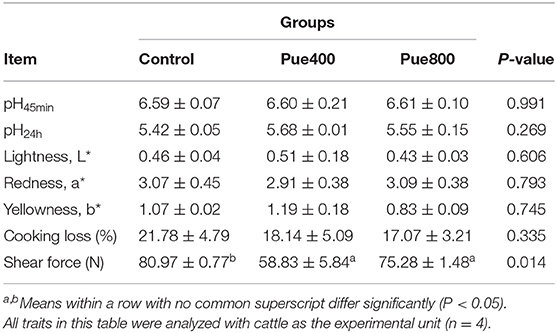
Table 5. Effects of Puerarin on the meat quality in M. longissimus thoracis of beef cattle under hot environment.
Muscle Fiber Morphology
As shown in Figure 2, diet supplemented with 400 mg/kg Puerarin reduced the diameter (Figure 2B) of the LT muscle compared with the control group (P < 0.05). However, there was no difference in the muscle fiber density (Figure 2C) among groups.
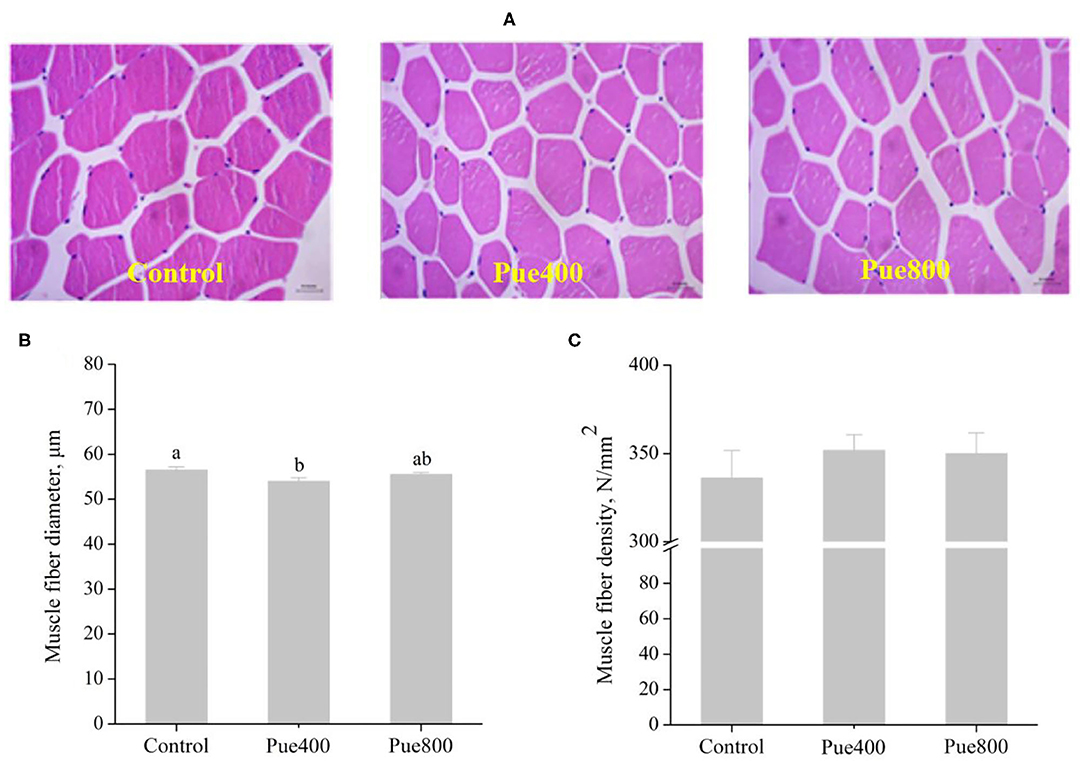
Figure 2. Effects of Puerarin on muscle fiber morphology (A), muscle fiber diameter (B), and muscle fiber density (C) in M. longissimus thoracis of beef cattle under hot environment.
Muscle Fiber Type
As shown in Figure 3, diet supplemented with 400 mg/kg Puerarin reduced the MyHC-IIb gene expression compared with the control group (P < 0.05). No difference was noticed about the mRNA expression of MyHC-I, MyHC-IIa, and MyHC-IIx among treatments.
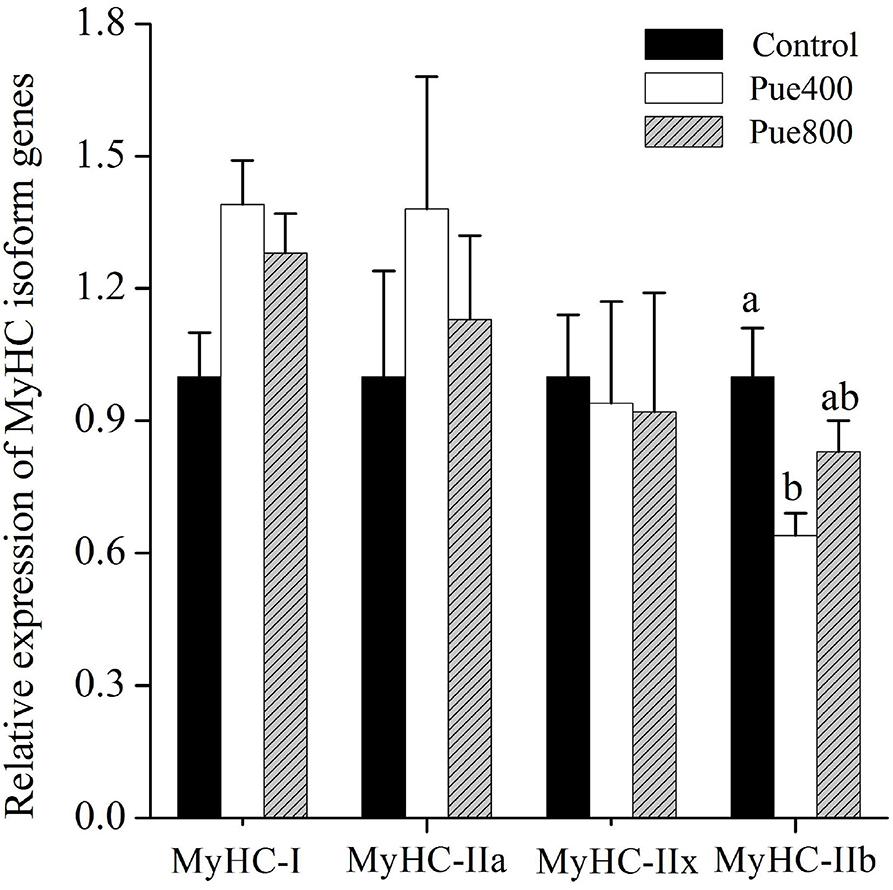
Figure 3. Effects of Puerarin on the relative mRNA expressions of myosin heavy-chain (MyHC) isoform genes in M. longissimus thoracis of beef cattle under hot environment (n = 4). (■): dietary supplementation with 0 mg/kg Puerarin in the feed concentrate; (□): dietary supplementation with 400 mg/kg Puerarin in the feed concentrate; (): dietary supplementation with 800 mg/kg Puerarin in the feed concentrate.
Adenosine Nucleotides
As presented in Table 6, diet supplemented with 400 mg/kg Puerarin improved the content of ATP compared with the control group (P < 0.05), and the ratio of AMP/ATP in the Pue400 and Pue800 groups were lower than those in the control group (P < 0.05). However, no difference was noticed about the contents of ADP and AMP among groups.
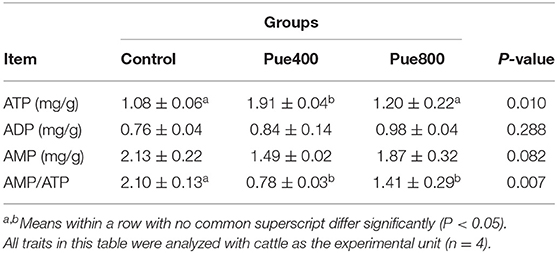
Table 6. Effects of Puerarin on the contents of adenosine nucleotides in M. longissimus thoracis of beef cattle under hot environment.
AMPK Signal Pathway-Related Gene Expressions
As shown in Figure 4, compared with the control group, the Pue800 group reduced the mRNA expression of AMPKα2 (P < 0.05). Moreover, the Pue400 group improved the mRNA expression of nuclear respiratory factor 1 (Nrf1) (P < 0.05). However, an insignificant effect was presented on the receptor serine/threonine kinase 1 [liver kinase B1 (LKB1)] and peroxisome proliferator-activated receptor γ coactivator 1α (PGC-1α) gene expression.
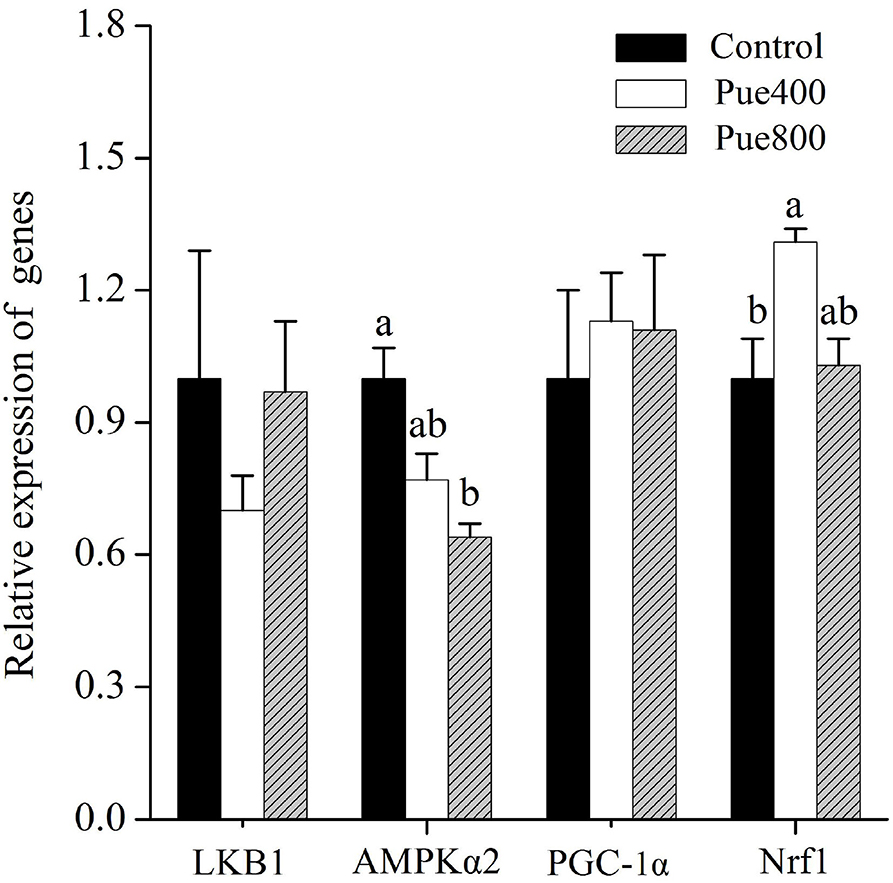
Figure 4. Effects of Puerarin on the relative mRNA expression of LKB1, AMPKα2, PGC-1α, and Nrf1 in M. longissimus thoracis of beef cattle under hot environment (n = 4). (■): dietary supplementation with 0 mg/kg Puerarin in the feed concentrate; (□): dietary supplementation with 400 mg/kg Puerarin in the feed concentrate; (): dietary supplementation with 800 mg/kg Puerarin in the feed concentrate. LKB1, receptor serine/threonine kinase 1; AMPKα2, adenosine monophosphate-activated protein kinase α2; PGC-1α, peroxisome proliferator-activated receptor-γ coactivator-1α; Nrf1, nuclear respiratory factor 1.
Discussion
The results of our previous study showed that the average daily THI values during the experimental period were higher than 79 for 54 out of 60 days (unpublished data), which indicated that the experimental beef cattle were in a state of heat stress according to the report of Livestock Conservation, Inc. (LCI) (21). It is widely known that heat stress leads to the decline of production performance, which has been suggested to be partly caused by the reduction of dry matter intake (22). In this study, diet supplemented with Puerarin improved the ADG of beef cattle, which might be partly attributed to the increased ADMI induced by Puerarin supplementation.
Numerous studies show that farm animals exposed to a hot environment are also related to an occurrence of oxidative stress and further result in a disorder of free radical metabolism and excessive production of ROS (2, 23). Subsequently, the high level of muscle ROS may increase the risk of oxidative reactions during the processes involved in the conversion of muscle into meat. One previous study found that pretreatment with Puerarin protected bovine Sertoli cells (bSCs) from heat stress by suppressing ROS production and changing the SOD, catalase (CAT), and GSH-PX activities and MDA content (15). Another study reported that Puerarin supplementation reduced the level of ROS and increased the activities of SOD and CAT in the kidney of diabetic mice (24). Similarly, in this study, dietary supplementation with Puerarin markedly increased the activities of SOD, GSH-PX, and T-AOC and reduced the content of MDA in the LT muscle of heat-stressed beef cattle. There was little research conducted on the effect of Puerarin on the antioxidant capacity of beef cattle. For ruminant, the isoflavones are readily hydrolyzed by rumen microbes, and the major metabolic transformation of isoflavones is performed in the rumen (25). There is evidence that Puerarin can be transformed into daidzein by bacterial enzymes in the large intestine of rats (26), and daidzein can be partly metabolized to equol by ruminal microorganisms (25). Daidzein and equol also have intrinsic antioxidant activities (27). Therefore, we speculated that Puerarin might have antioxidant effects for beef cattle in the form of daidzein, equol, and itself.
The tenderness of meat is the most important quality attribute influencing the consumer decision to purchase. There are complex interactions among various biochemical traits across multiple muscles affecting meat tenderness. One of the key events is the mitochondrial function. In skeletal muscle, mitochondria are abundant, and the power houses of cells function in muscle energy metabolism. Meanwhile, mitochondria are the main sites for ROS production; accumulation of damaged or dysfunctional mitochondria promotes an increase in ROS production (28). Mitochondrial functions are intrinsically linked to their morphology and membrane ultrastructure (29). Under physiological conditions, mitochondria are dynamic organelles that undergo permanent fission and fusion. While under heat stress conditions, the hypothalamic–pituitary–adrenal (HPA) axis was activated, which further induces calcium overload and promotes mitochondrial fission through phosphorylation of dynamin-related protein 1 (DRP1) (30). Our results were consistent with the theory that showed that heat stress disturbs the integrity of skeletal muscle cell membrane and keeps the most mitochondria in a fission state, while dietary supplementation with 400 mg/kg Puerarin promoted the integrity of cell membrane and maintained the mitochondrial morphology in round and oval shapes. Consistent with our results, Chen et al. (31) reported that Puerarin could improve the function of mitochondria in the muscle of diabetic rats by upregulating mitochondrial biogenesis. These results suggested that Puerarin could attenuate oxidative stress induced by heat stress in beef cattle, which may help improve meat tenderness by inhibiting the oxidation of muscle proteins, enzymes, and lipid initiated by free radicals and protecting the structure and functional integrity of muscle mitochondria and enzymes (32). Consistently, our results showed that Puerarin supplementation decreased the shear force of the LT muscle compared with the control group.
Besides, long-term heat stress has been reported to increase the muscle fiber diameter and lead to a higher shear force of meat (33). Generally, the thinner and denser the muscle fiber, the more significant tenderness of the meat (34). Fortunately, in the current study, our results showed that dietary supplementation with Puerarin reduced the muscle fiber diameter of the LT muscle, which was also advantageous for increased tenderness. Many factors influence muscle fiber traits, among them, the muscle fiber type is one of the most pivotal factors with fine oxidative fibers and coarse glycolytic fiber (35). Previous studies reported that muscle with more slow-twitch type I fibers might improve tenderness in cattle (36), while an increasing proportion of MyHC-IIb fiber type in muscle usually lead to higher lightness and drip loss in raw meat (37). Correspondingly, in the present study, dietary supplementation with Puerarin decreased the mRNA expression of MyHC-IIb of the LT muscle in heat-stressed beef cattle. It has been accepted that heat stress stimulates the glycolysis process (38) and resulted in a decrease in slow muscle fiber composition (7). Therefore, we speculated that Puerarin's modulation on muscle fiber type was dependent on heat stress relief. However, further investigation is required to determine the specific regulation mechanism of muscle fiber type transformation induced by Puerarin.
Previous research showed that AMPK played an essential role in promoting muscle to assume a faster-contracting, more glycolytic nature (12). Recent studies reported that Puerarin alleviated autophagy by inhibiting the phosphorylation of AMPK (16). Similarly, our results showed that dietary supplementation with Puerarin decreased the mRNA expression of AMPK. The AMP/ATP ratio, as an essential regulator of AMPK activity (9), was also reduced by dietary supplementation with Puerarin. In the summer, oxidative stress induced by heat exposure leads to mitochondrial damage and impairing ATP synthesis (39), which ultimately results in a higher AMP/ATP ratio. Fortunately, Xue et al. (40) reported that Puerarin could stabilize mitochondrial potential by protecting the mitochondrial function. Correspondingly, in this study, the decreased AMP/ATP ratio in the LT muscle might correlate with the increased content of ATP induced by the benefit of Puerarin on mitochondrial function, whereas LKB1, which is also an upstream activator of AMPK, did not vary significantly among treatments.
PGC-1α, as a transcriptional activator, is shown to upregulate the slow fiber gene expression. While Nrf1 is the downstream target gene of PGC-1α, it has also been reported to involve the transformation of muscle fiber type (41). In this study, dietary supplementation with Puerarin numerically increased the mRNA expression of PGC-1α, whereas it significantly increased the Nrf1 gene expression. Similarly, in C2C12 cells, treatment with Puerarin increased the protein expression levels of PGC-1α and Nrf1 (42). Therefore, we speculated that Puerarin affected muscle fiber type of beef cattle via regulating AMPK and Nrf1 gene expressions under heat stress.
Conclusion
In conclusion, dietary supplementation with Puerarin could decrease the meat shear force of beef cattle under high temperature. Moreover, the improvement of meat quality was via improving muscle antioxidant ability and reducing the MyHC-IIb muscle fiber composition of the heat-stressed beef cattle. Based on the results of this study, we recommended 400 mg/kg Puerarin in the feed concentrate of beef cattle (~300 kg) for mitigation of heat stress.
Data Availability Statement
The datasets presented in this article are not readily available because the study data are owned by Jiangxi Province Key Laboratory of Animal Nutrition. Access to these data would require additional approval beyond that of the authors. Requests to access these datasets should be directed to Dr. Yanjiao Li, yanjiaoli221@163.com.
Ethics Statement
This experiment was approved by the Committee for the Care and Use of Experimental Animals at Jiangxi Agricultural University (JXAULL-20190015).
Author Contributions
YL, HS, and XS designed the overall study. HS, XZ, MQ, TP, BG, and YH performed the experiments. YL and XS wrote the manuscript. All authors contributed to the article and approved the submitted version.
Funding
This work was supported by the National Natural Science Foundation of China (No. 31660672), the China Agricultural Research System (CARS) Program sponsored by the Ministry of Agriculture of China (No. CARS-37), the 2018 China Postdoctoral Science Foundation's 64th Batch of Funded Projects (2018M640605).
Conflict of Interest
The authors declare that the research was conducted in the absence of any commercial or financial relationships that could be construed as a potential conflict of interest.
Acknowledgments
The authors appreciate all the help from our colleagues and collaborators.
References
1. Silanikove N. Effects of heat stress on the welfare of extensively managed domestic ruminants. Livest Prod Sci. (2000) 67:1–18. doi: 10.1016/S0301-6226(00)00162-7
2. Kikusato M, Yoshida H, Furukawa K, Toyomizu M. Effect of heat stress-induced production of mitochondrial reactive oxygen species on NADPH oxidase and heme oxygenase-1 mRNA levels in avian muscle cells. J Therm Biol. (2015) 52:8–13. doi: 10.1016/j.jtherbio.2015.04.005
3. Xing T, Gao F, Tume RK, Zhou G, Xu X. Stress effects on meat quality: a mechanistic perspective. Compr Rev Food Sci F. (2019) 18:380–401. doi: 10.1111/1541-4337.12417
4. Pette D, Staron RS. Myosin isoforms, muscle fiber types, and transitions. Microsc Res Techniq. (2000) 50:500–9. doi: 10.1002/1097-0029(20000915)50:6<500::AID-JEMT7>3.0.CO;2-7
5. Joo ST, Kim GD, Hwang YH, Ryu YC. Control of fresh meat quality through manipulation of muscle fiber characteristics. Meat Sci. (2013) 95:828–36. doi: 10.1016/j.meatsci.2013.04.044
6. Liu QW. Effects of Constant High Ambient Temperature on Muscle Fibre Types and Nutrient Utilization of Growing Pigs. Dissertation/Doctor's Thesis. Guangzhou: South China Agricultural University (2009).
7. Wang SB, Deng L, Zhao J, Zhu XT, Shu G, Wang LN, et al. Effects of heat stress on antioxidant capacity and gastrocnemius musclefiber types of broilers. J South China Agric Univ. (2015) 36:23–8. doi: 10.7671/j.issn.1001-411X.2015.06.004
8. Hardie DG, Ross FA, Hawley SA. AMPK: a nutrient and energy sensor that maintains energy homeostasis. Nat Rev Mol Cell Biol. (2012) 13:251–62. doi: 10.1038/nrm3311
9. Chan AY, Dyck JR. Activation of AMP-activated protein kinase (AMPK) inhibits protein synthesis: a potential strategy to prevent the development of cardiac hypertrophy. Can J Physiol Pharm. (2005) 83:24–8. doi: 10.1139/y04-107
10. Karagounis LG, Hawley JA. 2009. The 5′ adenosine monophosphate-activated protein kinase: regulating the ebb and flow of cellular energetics. Int J Biochem Cell B. (2009) 41:2360–3. doi: 10.1016/j.biocel.2009.07.004
11. Witczak CA, Sharoff CG, Goodyear LJ. AMP-activated protein kinase in skeletal muscle: from structure and localization to its role as a master regulator of cellular metabolism. Cell Mol Life Sci. (2008) 65:3737–55. doi: 10.1007/s00018-008-8244-6
12. Park SK, Sheffler TL, Spurlock ME, Grant AL, Gerrard DE. Chronic activation of 5′-AMP-activated protein kinase changes myosin heavy chain expression in growing pigs. J Anim Sci. (2009) 87:3124–33. doi: 10.2527/jas.2009-1989
13. Chung MJ, Sung NJ, Park CS, Kweon DK, Mantovani A, Moon TW, et al. Antioxidative and hypocholesterolemic activities of water-soluble Puerarin glycosides in HepG2 cells and in C57 BL/6J mice. Eur J Pharmacol. (2008) 578:159–70. doi: 10.1016/j.ejphar.2007.09.036
14. Jiang M, Yun Q, Niu G, Gao Y, Shi F, Yu S. Puerarin prevents inflammation and apoptosis in the neurocytes of a murine Parkinson's disease model. Genet Mol Res. (2016) 15:1–9. doi: 10.4238/gmr.15047501
15. Cong X, Zhang Q, Li H, Jiang Z, Cao R, Gao S, et al. Puerarin ameliorates heat stress-induced oxidative damage and apoptosis in bovine Sertoli cells by suppressing ROS production and upregulating Hsp72 expression. Theriogenology. (2017) 88:215–27. doi: 10.1016/j.theriogenology.2016.09.033
16. Wang JF, Mei ZG, Fu Y, Yang SB, Zhang SZ, Huang WF, et al. Puerarin protects rat brain against ischemia/reperfusion injury by suppressing autophagy via the AMPK-mTOR-ULK1 signaling pathway. Neural Regen Res. (2018) 13:989–98. doi: 10.4103/1673-5374.233441
17. Xia DZ, Zhang PH, Fu Y, Yu WF, Ju MT. Hepatoprotective activity of Puerarin against carbon tetrachloride-induced injuries in rats: a randomized controlled trial. Food Chem Toxicol. (2013) 59:90–5. doi: 10.1016/j.fct.2013.05.055
18. Zhao XH, Yang ZQ, Bao LB, Wang CY, Zhou S, Gong JM, et al. Daidzein enhances intramuscular fat deposition and improves meat quality in finishing steers. Exp Biol Med. (2015) 240:1152–7. doi: 10.1177/1535370214564755
19. Li YJ, Gao T, Li JL, Zhang L, Gao F, Zhou GH. Effects of dietary starch types on early postmortem muscle energy metabolism in finishing pigs. Meat Sci.. (2017) 133:204–9. doi: 10.1016/j.meatsci.2017.07.008
20. Li YJ, Li JL, Zhang L, Gao F, Zhou GH. Effects of dietary starch types on growth performance, meat quality and myofibre type of finishing pigs. Meat Sci.. (2017) 131:60–7. doi: 10.1016/j.meatsci.2017.04.237
22. Westwood CT, Lean IJ, Garvin JK. Factors influencing fertility of holstein dairy cows: a multivariate description. J Dairy Sci. (2002) 85:3225–37. doi: 10.3168/jds.S0022-0302(02)74411-1
23. Turk R, Podpečan O, Mrkun J, Flegar-Meštrić Z, Perkov S, Zrimšek P. The effect of seasonal thermal stress on lipid mobilisation, antioxidant status and reproductive performance in dairy cows. Reprod Domest Anim. (2015) 50:595–603. doi: 10.1111/rda.12534
24. Xu X, Zheng N, Chen Z, Huang W, Liang T, Kuang H. Puerarin, isolated from Pueraria lobata (Willd.), protects against diabetic nephropathy by attenuating oxidative stress. Gene. (2016) 591:411–6. doi: 10.1016/j.gene.2016.06.032
25. Lundh T. Metabolism of estrogenic isoflavones in domestic animals. Exp Biol Med. (1995) 208:33–9. doi: 10.3181/00379727-208-43828
26. Prasain JK, Jones K, Brissie N, Moore R, Wyss JM, Barnes S. Identification of Puerarin and its metabolites in rats by liquid chromatography– tandem mass spectrometry. J Agric Food Chem. (2004) 52:3708–712. doi: 10.1021/jf040037t
27. Rimbach G, De Pascual-Teresa S, Ewins B, Matsugo S, Uchida Y, Minihane A, et al. Antioxidant and free radical scavenging activity of isoflavone metabolites. Xenobiotica. (2003) 33:913–25. doi: 10.1080/0049825031000150444
28. Tal MC, Sasai M, Lee HK, Yordy B, Shadel GS, Iwasaki A. Absence of autophagy results in reactive oxygen species-dependent amplification of RLR signaling. Proc Natl Acad Sci USA. (2009) 106:2770–75. doi: 10.1073/pnas.0807694106
29. Vincent AE, Ng YS, White K, Davey T, Mannella CA, Falkous G, et al. The spectrum of mitochondrial ultrastructural defects in mitochondrial myopathy. Sci Rep. (2016) 6:30610. doi: 10.1038/srep30610
30. Bo T, Yamamori T, Suzuki M, Sakai Y, Yamamoto K, Inanami O. 2018 Calmodulin-dependent protein kinase II (CaMKII) mediates radiation-induced mitochondrial fission by regulating the phosphorylation of dynamin-related protein 1 (Drp1) at serine 616. Biochem Biophys Res Commyn. (2018) 495:1601–7. doi: 10.1016/j.bbrc.2017.12.012
31. Chen X, Wang L, Wu Y, Song S, Min H, Yang Y, et al. Effect of Puerarin in promoting fatty acid oxidation by increasing mitochondrial oxidative capacity and biogenesis in skeletal muscle in diabetic rats. Nutr Diabetes. (2018) 8:1–13. doi: 10.1038/s41387-017-0009-6
32. Warner RD, Dunshea FR, Ponnampalam EN, Cottrell JJ. Effects of nitric oxide and oxidation in vivo and postmortem on meat tenderness. Meat Sci. (2005) 71:205–17. doi: 10.1016/j.meatsci.2005.04.008
33. Huang T. Effects of Honeysuckle Extract on Skeletal Muscle Morphology and Meat Quality in Beef Cattle under Hot Environment, Dissertation/Master's Thesis, Nanchang: Jiangxi Agricultural University (2015).
34. Gwartney BL, Jones SJ, Calkins CR. Response time of broiler chickens to cimaterol: meat tenderness, muscle composition fiber size, and carcass characteristics. J Anim Sci. (1992) 70:2144–50. doi: 10.2527/1992.7072144x
35. Lefaucheur L. A second look into fibre typing-Relation to meat quality. Meat Sci. (2010) 84:257–70. doi: 10.1016/j.meatsci.2009.05.004
36. Maltin CA, Sinclair KD, Warriss PD, Grant CM, Porter AD, Delday MI, et al. The effects of age at slaughter, genotype and finishing system on the biochemical properties, muscle fibre type characteristics and eating quality of bull beef from suckled calves. Anim Sci. (1998) 66:341–8. doi: 10.1017/S1357729800009462
37. Choe JH, Choi YM, Lee SH, Shin HG, Ryu YC, Hong KC, et al. The relation between glycogen, lactate content and muscle fiber type composition, and their influence on postmortem glycolytic rate and pork quality. Meat Sci. (2008) 80:355–62. doi: 10.1016/j.meatsci.2007.12.019
38. Wu SY, Fang Z, Xue B, Liu LZ, Yang Y. A review of effects of heat stress on substance and energy metabolism in muscle. Agric Sci Technol. (2015) 16:1011–1013
39. Belhadj Slimen I, Najar T, Ghram A, Abdrrabba M. Heat stress effects on livestock: molecular, cellular and metabolic aspects, a review. J Anim Physiol Anim Nutr (Berl). (2016) 100:401–12. doi: 10.1111/jpn.12379
40. Xue B, Wang L, Zhang Z, Wang R, Xia XX, Han PP, et al. Puerarin may protect against Schwann cell damage induced by glucose fluctuation. J Nat Med-Tokyo. (2017) 71:472–81. doi: 10.1007/s11418-016-1067-0
41. Schuler M, Ali F, Chambon C, Duteil D, Bornert JM, Tardivel A, et al. PGC1α expression is controlled in skeletal muscles by PPARβ, whose ablation results in fiber-type switching, obesity, and type 2 diabetes. Cell Metab. (2006) 4:407–14. doi: 10.1016/j.cmet.2006.10.003
Keywords: Puerarin, beef cattle, heat stress, meat quality, muscle fiber type
Citation: Li Y, Shang H, Zhao X, Qu M, Peng T, Guo B, Hu Y and Song X (2021) Radix Puerarin Extract (Puerarin) Could Improve Meat Quality of Heat-Stressed Beef Cattle Through Changing Muscle Antioxidant Ability and Fiber Characteristics. Front. Vet. Sci. 7:615086. doi: 10.3389/fvets.2020.615086
Received: 08 October 2020; Accepted: 30 November 2020;
Published: 15 January 2021.
Edited by:
Zora Varadyova, Slovak Academy of Sciences, SlovakiaReviewed by:
Mabrouk Elsabagh, Kafrelsheikh University, EgyptShad Mahfuz, Sylhet Agricultural University, Bangladesh
Copyright © 2021 Li, Shang, Zhao, Qu, Peng, Guo, Hu and Song. This is an open-access article distributed under the terms of the Creative Commons Attribution License (CC BY). The use, distribution or reproduction in other forums is permitted, provided the original author(s) and the copyright owner(s) are credited and that the original publication in this journal is cited, in accordance with accepted academic practice. No use, distribution or reproduction is permitted which does not comply with these terms.
*Correspondence: Xiaozhen Song, songxz1234@163.com