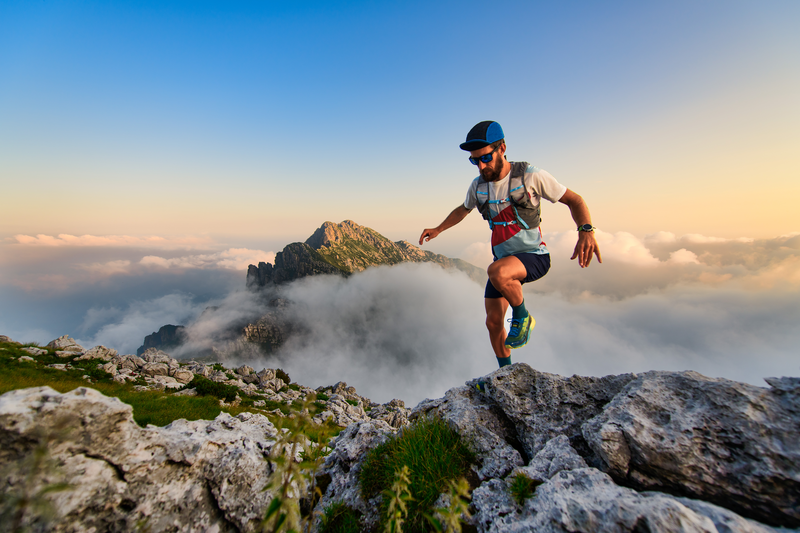
94% of researchers rate our articles as excellent or good
Learn more about the work of our research integrity team to safeguard the quality of each article we publish.
Find out more
ORIGINAL RESEARCH article
Front. Vet. Sci. , 26 January 2021
Sec. Animal Nutrition and Metabolism
Volume 7 - 2020 | https://doi.org/10.3389/fvets.2020.602280
This article is part of the Research Topic Natural Bioactives and Inflammation View all 6 articles
Despite inflammation being a protective natural defense against imbalance stressors in the body, chronic inflammation could lead to the deterioration of immune response, low production, and poor performance in livestock as well as severe economic losses to the farmers. Postbiotics produced by Lactiplantibacillus plantarum has been reported recently to be a natural source of antioxidant, promoting growth performance, anti-inflammation, and immune responses. However, the effects of fermentation media on the compositions of L. plantarum postbiotic have not been reported elsewhere. Hence, a comparative study was conducted to compare the volatile compounds, organic acid composition, and antioxidant and antimicrobial activities of postbiotics produced by six strains of L. plantarum cultivated by using formulated media and the commercial de Man, Rogosa, and Sharpe (MRS) medium as a control. Postbiotics RG14, RI11, and UL4 produced by using formulated media exhibited higher inhibitory activity against Pediococcus acidilactici 446, Escherichia coli E-30, Salmonella enterica CS3, and vancomycin-resistant Enterococci except for Listeria monocytogenes LS55. As for the antioxidant activity, hydroxyl radical scavenging activity was enhanced in formulated media, whereas reducing power activity was the highest in postbiotic RI11. Three organic acids, namely, acetic acid, caproic acid, and lactic acid, were detected in the postbiotic produced by various L. plantarum strains. The concentration of acetic acid was influenced by the fermentation media, whereas caproic acid was detected as the highest in postbiotic RG11. Lactic acid was the predominant compound detected in all the postbiotics and had the significantly highest concentration in postbiotic RS5 when produced by using the MRS medium. Intermediary and pyrrole compounds were the other main compounds that were detected by using GC-MS. Positive correlations were found between organic acid production and inhibitory activity, as well as antioxidant activity exhibited by postbiotics. In conclusion, the compositions and functional characteristics of postbiotics produced by the six strains of L. plantarum were strain-dependent and affected greatly by the fermentation medium. The effects of postbiotic composition on the functional characteristics of postbiotics were elucidated in this study to warrant their applications as a promising beneficial natural growth promoter for the livestock industry.
Inflammation is an essential natural defense process that occurs when there is an imbalance of stressors caused by pathogens, allergens, radiation, toxin, and oxidative stress in the body (1). As a series of protective mechanisms involving the molecular reactions and cellular activity, inflammation speeds up tissue repair to prevent permanent damage (2–4). The inflammatory process also helps to build immunity against inevitable microbial challenges such as Salmonella sp. and Eimeria sp. infections by stimulating rapid expression of pro-inflammatory cytokines, maintains homeostasis, and improves vaccine efficacy (5, 6), and hence enhances the resistance of diseases in commercially farmed animals.
Although inflammation is a fundamental process to fight infection, prolonged and chronic inflammation leads to damaging consequences such as tissue and organ damage, and mortality (2). In livestock farming, numerous factors induce the occurrence of inflammation, including the intensification of production, poor quality, and contamination in feedstuffs as well as genetic selection (7). As a result, this will contribute to poor growth performance and production due to low nutrient absorption, loss of appetite, impaired intestinal architecture, and leaky gut (4, 8), which lead to a large amount of energy consumption on the immune system instead of growing and production (9). Consequently, inflammation reduces the economic viability of farmers and food product quality, and endangers animal health. To encounter this phenomenon, natural bioactive ingredients synthesized by probiotic bacteria serve as potential regulators of inflammation (10, 11).
Lactiplantibacillus plantarum (L. plantarum) or previously known as Lactobacillus plantarum (12) has been shown to synthesize various beneficial and economically important extracellular metabolites known as postbiotic, which contains organic acids such as lactic acid and other short-chain fatty acids (SCFAs). Unlike previously reported pre-, pro-, and paraprobiotic, postbiotic is a bioactive soluble compound or peptide that is produced from complex metabolic fermentation activity during the growth of lactic acid bacteria (13, 14). Postbiotics confer health benefits on the host or food (15) to prevent infection, antitumor, immunomodulatory, and antiatherosclerotic effects and improved wound healing (16), as compared to cell-free supernatant preparations. Moreover, postbiotics are well documented for their anti-inflammatory and broad spectrum of antimicrobial activities (13, 17, 18). Other compounds that are secreted along with organic acids include vitamins, enzymes, extracellular proteins, indole, immune signaling compounds, cofactors, extracellular vesicles, and complex agents that are involved in regulating the intestinal epithelial barrier to promote better gut health (19). The antimicrobial peptides of bacteriocin molecule present in the postbiotic function as an antimicrobial agent against various pathogens, i.e., Salmonella sp. and Escherichia coli (20–22). Furthermore, supplementation of L. plantarum postbiotics in feed improves the growth performance of livestock (23, 24), laying performance (25), villus height (25, 26), antioxidant activity in the blood (27), and immune response (24), and reduces adverse impacts on the physiological activities of broilers due to heat stress (28). However, the production of postbiotic metabolites is affected by various factors such as temperature and pH (29), strains of producer bacteria, and nutrient components of the growth medium (30, 31).
Bacterial fermentation technology has gained vast attention as it produces compounds that have economic value for various applications such as biofuel, pharmaceutical products, and chemicals for various industries (32). Bacteria require sufficient carbon, nitrogen, and other nutrients in the growth medium to improve growth, metabolic activity, and production of vital metabolites (33). As reported previously, the optimization of fermentation media enhanced the bacteriocin inhibitory of postbiotic produced by L. plantarum I-UL4 (31). Moreover, L. plantarum postbiotics exerted higher inhibitory activity against various pathogenic bacteria such as E. coli, Salmonella enterica, Listeria monocytogenes, and VRE when inulin is included in the fermentation media (22). This further promotes the growth performance, growth-related gene expression, meat quality, and fecal microbiota in broiler chickens (21, 34). From the studies, fermentation media have been reported to affect the secretion of metabolic products and biological activities of postbiotic.
Although there are extensive reports on the benefits of L. plantarum postbiotic as a natural growth promoter to replace antibiotic growth promoter (AGP), the link between formulated media and the composition, antioxidant activity, and antimicrobial activity of postbiotics is yet to be explored. Therefore, the objective of this current research was to compare the composition and functional characteristics of postbiotics produced by six strains of L. plantarum using different formulated media. The effect of postbiotic composition on the functional characteristics of postbiotics would be elucidated in this study to warrant their applications as a promising beneficial natural growth promoter for the livestock industry.
The six strains of L. plantarum that were used in this study, namely, RG11, RG14, RI11, RS5, TL1, and UL4, which were previously isolated from Malaysian fermented foods (35, 36), and obtained from the Laboratory of Industrial Biotechnology, Department of Bioprocess Technology, Faculty of Biotechnology and Biomolecular Sciences, Universiti Putra Malaysia. The L. plantarum cultures were maintained and revived as described by Foo et al. (37). Pediococcus acidilactici 446, which was employed as a positive indicator bacterium, was cultured in an MRS medium, while the pathogenic bacteria E. coli E-30 and S. enterica CS3 were propagated in a Luria Bertani (LB) medium. L. monocytogenes LS55 was cultured in Listeria enrichment broth (LEB), and vancomycin-resistant Enterococci was cultivated in a brain heart infusion (BHI) medium. All the pathogens were revived twice for 48 h and 24 h at 37°C with agitation at 150 rpm using an incubator shaker according to the method described by Kareem et al. (22).
The active L. plantarum strains were washed once with sterile 0.85% (w/v) NaCl (Merck, Darmstadt, Germany) solution and adjusted to 109 CFU/mL to be used as a 10% (v/v) inoculum according to the method described by Mohamad Zabidi et al. (38). The postbiotics of the six strains of L. plantarum were prepared at 30°C for 24 h according to the method described by Kareem et al. (22) by using MRS as a control medium and their respective formulated media as shown in Table 1. The postbiotics were harvested after centrifugation at 10,000 × g for 15 min at 4°C and filtered through a 0.22 μm cellulose acetate membrane (Sartorius Minisart, Germany) to remove the residue of viable producer cells.
Table 1. Medium composition of de Man, Rogosa, and Sharpe and formulated media for postbiotic production by six L. plantarum strains.
The postbiotics of all L. plantarum strains were diluted through two-fold serial dilution using NaCl solution (0.85% w/v). The diluted postbiotics were loaded at 20 μL for P. acidilactici 446 and 100 μL for E. coli E-30, S. enterica CS3, L. monocytogenes LS55, and VRE, respectively, into the pre-punched agar well according to the method described by Kareem et al. (22). All the plates were allowed to dry at room temperature for about 30–60 min. Then, the agar plates were overlaid with soft agar seeded with the respective bacterial cells before incubation at 37°C for 24–48 h. A clear inhibition zone with more than 0.1 cm formed around the well was measured and recorded as a positive inhibitory result. The experiment was conducted with three replicates. The inhibitory activity was determined as a modified arbitrary unit (MAU/ml) (31) by using the following formula:
The hydroxyl radical scavenging (HRS) activity was determined by following the method of Xing et al. (39) with slight modification. Each 250 μL sample was added with 250 μL of 2.5 mM 1,10-phenanthroline, 250 μL of PBS (0.1 M, pH 7.4), and 250 μL of 2.5 mM FeSO4 prior mixed with 250 μL of 20 mM H2O2. The test solution was incubated at 37°C for 90 min and absorbance was read at 536 nm using a UV spectrophotometer. HRS activity was determined using the following equation:
where As is the absorbance of the sample, Ac is the absorbance containing the deionized water, and Ab is the absorbance of the solution without the presence of the sample and H2O2. All the samples were tested with three replications.
The reducing power (RP) of the postbiotics was determined using the method of Xing et al. (39) with slight modification. The assay mixture contained 250 μL of the sample, 250 μL of PBS (0.2 M, pH 6.6), and 250 μL of 1% potassium ferricyanide before heating at 50°C for 20 min. The test solution was allowed to cool down to room temperature. Then, the mixture was added with 250 μL 10% trichloroacetic acid, centrifuged at a speed of 3,000 × g for 5 min at 4°C. The supernatant was collected and added with 250 μL deionized water. Absorbance was taken at 700 nm after adding 500 μL 0.1% iron (III) chloride. Ascorbic acid was used as the standard. All the samples were repeated in triplicates.
The SCFAs in postbiotics were determined by using gas chromatography according to Nakkarach et al. (40) with slight modifications. A 500 μL postbiotic produced by each strain was added with 500 μL 5 mM 4-methyl valeric acid, and 1 μL of the sample was injected into a gas chromatograph (GC, Agilent Technologies, USA). The GC was equipped with a flame ionization detector and a DB-FFAP 122-3232 fused-silica capillary column (30 m × 0.25 μm × 0.25 μm). The temperature of the column was set at 100°C, increased to 180°C with 10°C/min for accurate quantification. Temperatures for the detector, inlet, and oven were set at 250°C, 230°C, and 230°C, respectively. Nitrogen gas was used as the carrier gas with a flow of 35 mL/min, while methanol was used as the solvent. Acetic acid (10 mM), propionic acid (10 mM), butyric acid (10 mM), isobutyric acid (10 mM), isovaleric acid (10 mM), valeric acid (10 mM), caproic acid (10 mM), and 4-methyl valeric acid (5 mM) were used as standard solutions to identify the peaks. All the samples were tested in triplicates. The calculation of the acid concentration was done by using the following formula:
where A, 2 MV = peak heights of VFA and 4-methyl valeric acid in the standard mixture
a, and 2 mv = peak heights of VFA and 4-methyl valeric acid in a sample.
The lactic acid concentration (g/L) in postbiotics was determined according to Borshchevskaya et al. (41). Briefly the postbiotic was diluted using pure water before being added with 2 mL 0.2% iron (III) chloride. The absorbance of the test solutions was read at 390 nm using a UV-visible spectrophotometer (Agilent Technologies, USA) before 15 min upon mixing. A standard curve was plotted using standard lactic acid (L6661, Sigma Chemical, USA) at the concentration of 0–80 mg/mL. All the samples were tested with three replications.
The samples were prepared according to Kam et al. (42) with slight modifications. An equal volume of ethyl acetate (HPLC grade) was used to extract 30 mL of supernatants twice. The mixture of supernatant and ethyl acetate was stirred for about 15–20 min before allowing the separation into water and organic phase by using a separatory funnel. The collected water phase was allowed to re-extract again with ethyl acetate, while the organic phase was transferred into a Schott bottle. The organic phase produced from the second extraction was combined with the previously collected organic phase. Then, the organic phase was allowed to dry up by using a rotary evaporator (Buchi, Switzerland) at 40°C before being added with 500 μL of methanol (HPLC grade). The mixture was then allowed to dry in a fume chamber overnight (12–15 h) at room temperature. All the samples were added with 1 mL of methanol before being filtered with a syringe filter of 0.2 μm. The samples were analyzed using GC–MS (Shimadzu model GC-2010) connected with QP2010 Ultra as the analytical line. A column of RXI-5MS (30 m × 0.25 mm × 0.25 μm) was used with the injector temperature at 250°C in split mode. Helium was used as the carrier gas. The oven temperature was set at 50°C and was increased at 3°C/min to 300°C and held for 10 min. For the analytical part, the ion source was set at 200°C, EM voltage at 0.92 kV, and solvent cut time at 2 min. Scan mode was used and performed from the range of 40 to 700 m/z starting from 2.4 to 93.0 min. The identification of compounds was based on NIST Library 11 and Willey 229.
The collected data except for GC-MS analysis were analyzed using two-way analysis of variance (ANOVA) using the Statistical Analysis System (SAS) program (version 9.4) to determine the significant difference and interactions among the factors. Tukey's Honest Significant Difference Test was used to compare a significant difference of treatment means at P < 0.05. A correlation test was used to determine the relationship between the compositions of the postbiotics and the functional characteristics.
The inhibitory activities exhibited by each postbiotic against a positive indicator and pathogenic bacteria are shown in Table 2. Generally, the modified inhibitory activity of L. plantarum postbiotics was enhanced (P < 0.05) when produced by using formulated media except for the modified inhibitory activity against L. monocytogenes LS55. Postbiotic RG14 exhibited the highest (P < 0.05) inhibitory activity against a positive indicator, P. acidilactici 446. Moreover, the postbiotic RG14, RS5, and TL1 produced significantly (P < 0.05) stronger antimicrobial action for E. coli E-30, as compared to other strains of L. plantarum. Besides, postbiotics RG14 and RI11 had higher (P < 0.05) inhibitory activity against S. enterica CS3 in comparison to other postbiotics. Postbiotic RI11 and UL4 produced the highest and lowest (P < 0.05) inhibitory activities against VRE, whereas postbiotic RS5 exhibited the lowest (P < 0.05) inhibitory activity when tested against L. monocytogenes LS55, even though it showed no significant difference (P > 0.05) with RG11 and RG14.
Table 2. Inhibitory activity of L. plantarum postbiotics against positive indicator P. acidilactici 446, and pathogenic bacteria E. coli E-30, S. enterica CS3, VRE, and L. monocytogenes LS55.
The HRS activity was significantly higher (P < 0.05) for the postbiotics produced by the formulated media as compared to the control MRS medium, even though there was no significant difference among all the producer strains (Table 3). There was significant (P < 0.05) interaction between media and strains on the HRS activity. As for the RP activity, among all the postbiotics, RI11 was observed to have the highest (P < 0.05) activity but showed no significant difference (P > 0.05) with RG14 and UL4 (Table 3). However, there was no significant difference (P > 0.05) in the fermentation media used to produce postbiotics on RP activity. There was also no significant (P > 0.05) interaction found between media and strains on RP activity.
Table 3. Hydroxyl radical scavenging and reducing power activity of L. plantarum postbiotics produced by using different fermentation media.
The production of acetic acid was detected in all the L. plantarum strains. The highest concentration (P < 0.05) of acetic acid was recorded by postbiotic TL1 and UL4, even though UL4 had no significant (P > 0.05) difference among other postbiotics (Table 4). There was no significant difference (P > 0.05) detected on the media used to produce acetic acid. In comparison, postbiotic RG11 produced the highest caproic acid, while the lowest caproic acid production was detected in TL1 and UL4 (Table 4). On the other hand, the formulated fermentation media had significantly increased (P < 0.05) the production of caproic acid in postbiotic metabolite.
Table 4. Acetic, caproic, and lactic acid concentration of L. plantarum postbiotics produced by using different fermentation media.
As the major acid secreted by lactic acid bacteria, postbiotic RS5 produced the highest (P < 0.05) concentration of lactic acid (Table 4). Interestingly, the commercial control MRS medium exhibited a greater effect (P < 0.05) on the formation of lactic acid, even though postbiotic TL1 that grown in a commercial control MRS medium produced the significantly lowest (P < 0.05) yield of lactic acid.
The volatile compound profiles of postbiotics are shown in Table 5. Altogether, there were 16 organic compounds including ester, acids, and pyrrole compounds that were detected from the postbiotics produced by various strains of L. plantarum using GC-MS. Postbiotic RI11 produced by using formulated media contained the highest numbers of volatile compounds, unlike other postbiotics that contained only six types of volatile compounds. Nevertheless, there were 10 volatile compounds identified from postbiotic UL4 produced by using a control MRS medium, 9 volatile compounds from the postbiotics RI11 and RS5, 8 volatile compounds from the postbiotics RG11 and RG14, and 7 volatile compounds from the postbiotic TL1. The relative peak area of lactic acid, which was found to be predominant in all postbiotics (70.65%–93.41%), was noted higher in postbiotic produced by using formulated media in comparison to those postbiotics produced by using a control MRS medium (14.52–70.87%). Moreover, pentanoic acid, 2-hydroxy-4-methyl-, methyl ester, and 1,4-diaza-2,5-dioxo-3-isobutyl bicycle [4.3.0] nonane were detected at the least level in the postbiotics produced by various strains of L. plantarum.
The correlations between the six commonly found compounds in all the postbiotics with the inhibitory activity and antioxidant activity are shown in Table 6. A positive correlation (P < 0.05) was demonstrated between acetic acid production with inhibitory activity against P. acidilactici 446, S. enterica CS3, and L. monocytogenes LS55. A significant positive (P < 0.05) can also be detected between caproic acid production with the inhibitory activity against E. coli E-30, L. monocytogenes LS55, and VRE. The lactic acid production in the postbiotics had a significant positive correlation (P < 0.05) with inhibitory activity against S. enterica CS3 and HRS activity. Moreover, the propanoic acid, 2-hydroxy-methyl ester, 1,4-diaza-2,5-dioxo-3-isobutyl bicyclo [4.3.0] nonane, 2-3-dihydro-3,5-dihydroxy-6-methyl-4H-Pyran-4-one, and pyrrolo [1,2-a] pyrazine-1,4-dione, hexahydro-3-(2-methylpropyl) demonstrated a positive relationship with the HRS and RP activities, even though no significant correlation (P > 0.05) was detected between those compounds with all the inhibitory activity. However, the result revealed that acetoin exhibited a negative relationship with the inhibitory activity against S. enterica CS3 and HRS activity.
Table 6. Correlation test (R) between L. plantarum postbiotics composition and functional characteristics.
The present study was conducted to elucidate the potential of the postbiotic metabolite produced by six strains of L. plantarum to replace AGPs, which have been used to curb the effects of inflammation in poultry. Generally, antibiotics have been widely used in livestock production as an antimicrobial against various pathogens such as E. coli, Salmonella sp., Campylobacter sp., Pasteurella multocida, Mycoplasma sp., Clostridium perfringens, and Chlamydia psittaci (43, 44). However, antibiotic abuse has accelerated the prevalence of antimicrobial-resistant bacteria and their transmissions to humans via the food chain (45, 46). In the present study, the growth of L. plantarum strains in formulated media produced postbiotic metabolites with enhanced biological functionalities, such as antimicrobial and antioxidant activities, through the production of the bioactive ingredients secreted extracellularly during the growth of producer cells. The higher inhibitory activity exerted by postbiotics produced by using formulated media could be attributed to the higher concentration of yeast extract that contains amino acids and growth factors (47). Thus, when incorporated in the formulated media, it had further promoted good bacterial cell growth and bacteriocin production as reported by Ooi et al. (31) and Abbasiliasi et al. (47). On top of that, the ingredients in MRS such as meat extract and peptone not only inhibit the growth but also reduce the antimicrobial activity of the bacteria (31, 48). For the carbon source, glucose has been proven to be the best ingredient for bacteriocin production due to its simple structure as compared to other carbon sources such as sucrose (31, 49).
Furthermore, the inhibitory activity of postbiotics was influenced by bacteriocin production and organic acid production. There are two classes of bacteriocin genes, namely, plantaricin EF and plantaricin W, which have been proven to be harbored by the novel L. plantarum I-UL4 (50), which are responsible for the production of plantaricin Ef and plantaricin W bacteriocins, respectively (51). The positive correlation (P < 0.05) between acetic acid, lactic acid, and caproic acid with inhibitory activities against various pathogens implying the organic acid production would further enhance the bacteriocin inhibitory activity of postbiotics. In addition, the secretion of a high concentration of organic acids and antioxidant compounds have been reported to contribute substantially to the inhibitory activity of postbiotic metabolites produced by various strains of L. plantarum (22, 52, 53).
In the livestock industry, inflammation due to bacterial infection is a common and profound challenge that caused an immense amount of loss and less profitable. The negative effects of bacterial infection can be avoided by using postbiotics since they exhibited a broad spectrum of antimicrobial activities against gram-positive and gram-negative pathogenic bacteria due to the presence of bacteriocin (52, 53) and organic acids (54, 55). The inhibitory activity exhibited by L. plantarum postbiotics in this experiment was in agreement with the previous reports (21, 22, 55) where the metabolite produced by L. plantarum strains exhibited antimicrobial effects on P. acidilactici, S. enterica, E. coli, VRE, and L. monocytogenes. This study also showed that postbiotic produced by using formulated media demonstrated a higher capability to inhibit the growth and proliferation of detrimental pathogenic bacteria, indicating that the postbiotic metabolites of L. plantarum strains were a potential alternative to AGP that exhibited probiotic effect without the inclusion of living cells (55).
The natural source of antioxidants has been used to counteract the implication due to oxidative stress and reactive oxygen species. Since oxidative stress antagonistically influences the occurrence of inflammation, cellular damage, and disease susceptibility in the body (56, 57), the postbiotic produced by L. plantarum has the potential to reduce the oxidation of proteins and lipids particularly through two mechanisms, namely, HRS and RP. Unlike other previous studies that reported on the DPPH and ABTS activities of L. plantarum (27, 58), two other assays were used in the current research to elucidate further the antioxidant activity of postbiotic produced by L. plantarum. HRS measured the scavenging activity of the most reactive radical, hydroxyl radical, which is related to lipid peroxidation and biomolecules of cells (59). In the present study, the HRS activity of the postbiotics was increased from 29 to 32% produced by using a control MRS medium to 31–37% when produced by using formulated media. Additionally, the postbiotic has an RP of 1.9–2.9 mg/L of ascorbic acid regardless of the fermentation medium used. The results obtained in this study were consistent with previous studies reported (60, 61) for having RP through the inhibition of the oxidation process by converting hydroperoxides to hydroxyoctadecadienoic acids and iron chelators in postbiotic due to the presence of various intracellular antioxidants such as pyrrole compounds in the postbiotic (62). Moreover, studies also revealed that the difference in the antioxidant activity of postbiotics depends on mechanisms such as the metal ion chelating ability, the antioxidant enzyme system, and the antioxidant metabolites present in the postbiotic (63). Therefore, postbiotic can be exploited as a supplement and feed additive to prevent inflammation due to oxidative stress-related diseases (55, 64). The antioxidant activity of postbiotics could be contributed by the formation of pyrrole and cyclic compounds as shown in Table 5.
The antioxidant activity exerted by L. plantarum helps to scavenge and inhibit free radicals against oxidation processes (35, 65). In the in vitro study conducted by Chen et al. (65), the fermentation of papaya juice by using L. plantarum produced higher antioxidant activities as compared to L. acidophilus. The in-feed supplementation of L. plantarum postbiotics has been documented to reduce the negative effect stimulated by hepatic injury in mice (66) and to improve glutathione peroxidase (GPX) in blood serum and ruminal fluid in post-weaning lamb (27), as well as to enhance the activities of total antioxidant capacity (T-AOC), catalase (CAT), and GPX while reducing alpha-1-acid glycoprotein (α1-AGP) and ceruloplasmin in broiler blood plasma (67). Therefore, L. plantarum postbiotic can be used as a promising natural source of antioxidants to reduce the effects of heat stress in animals. Moreover, the supplementation of L. plantarum has been shown to impede lipid peroxidation and suppressed oxidative stress in serum and liver induced by aflatoxin AFB1 (68).
The SCFAs are known for their ability to down-regulate the production of pro-inflammatory cytokines expression and act on respective cells such as neutrophils and macrophages responsible for inducing inflammation (69). Besides acting as a regulator to intestinal health and mucous production to restrict the adhesion of pathogens on the epithelial cells (70–73), SCFAs are also responsible for the maintenance of the tight junction integrity to reduce the risk of intestinal inflammation (73), provide nutrient to hepatocytes (74), and influence hormonal regulation (75). Moreover, acetic acid also involves in the synthesis of cholesterol and fatty acids. Nevertheless, caproic acid and lactic acid are strong antimicrobial agents (76, 77) in decreasing the colonization of pathogenic bacteria in the gut while enhancing the digestibility of protein and mineral, respectively (68, 69). This agreed with the findings of this study where the high production of both VFAs detected in postbiotics inhibited the proliferation of pathogens. The high concentration of acetic acid retarded the growth of P. acidilactici 446, S. enterica CS3, and L. monocytogenes LS55, whereas the caproic acid exhibited the antimicrobial activity against E. coli E-30, L. monocytogenes LS55, and VRE.
All L. plantarum strains employed in this study produced lactic acid as the predominant metabolite product during the growth, which is a distinguishing characteristic of lactic acid bacteria (78). Lactic acid lowers the environmental pH and disrupts the process of nutrient absorption and energy uptake of bacteria by breaking down the cell membrane via lysis (75, 79). Hence, low pH conditions due to the presence of organic acids prohibited the proliferation and survival of pathogens. This supported the result that the higher secretion of lactic acid promoted antimicrobial action against the growth of salmonella. The high correlation between the lactic acid and HRS could indicate that lactic acid can be used as an antioxidant agent, which, in turn, can further enhance the antimicrobial activity of the postbiotic. As a result, even if there is a risk of pathogen infection, the development of the inflammation process will be altered by immunomodulating gut health through a microbial ecosystem (80). Lactic acid is well-reported for its antimicrobial activity against pathogenic and zoonotic bacteria in livestock farming such as S. enteritidis, E. coli, L. monocytogenes, P. aeruginosa, S. aureus, E. faecalis, and yeast (81–83). Besides, Van Thu et al. (21) had reported the production of acetic acid and lactic acid by the combination of postbiotics produced by various L. plantarum strains.
The presence of different concentrations of organic acids in the postbiotic produced by different strains of L. plantarum may be possibly due to the effects of fermentation media on heterofermentative biochemical pathways involving citrate metabolism (84), whereby the production of acetic acid was lower than the lactic acid as shown by various L. plantarum strains used in this study. Despite the fact that there is a paucity of knowledge on the effects of fermentation media on the expression of gene related to organic acid production in each strain of bacteria, this study provided the fundamental knowledge on the influence of different fermentation media and bacteria strains on the production of organic acid in postbiotics.
The production of compounds such as organic acids, acetoin, intermediary compounds, and pyrrole compounds are commonly found in the postbiotic after bacterial fermentation. Acetoin, for example, is closely related to food flavor, and it is also involved in the production of the amino acid (85). Furthermore, previous studies documented that cyclic and pyrrole compounds such as 1,4-diaza-2,5-dioxo-3-isobutyl bicyclo [4.3.0] nonane, 2-3-dihydro-3,5-dihydroxy-6-methyl-4H-Pyran-4-one, and pyrrolo [1,2-a] pyrazine-1,4-dione, hexahydro-3-(2-methylpropyl) are strong antioxidant and antifungal agents (86–90). Therefore, the secretion of pyrrole and cyclic compounds in the postbiotics enhanced the antimicrobial activity against various pathogenic bacteria and the ability to inhibit or prevent the oxidation process.
Several studies have revealed that viable probiotic cells and the corresponding postbiotic of L. plantarum possessed anti-inflammatory properties. Toshimitsu et al. (91) reported that L. plantarum induced IL-10 production and ameliorated the metabolic effect of type 2 diabetes in mice. Furthermore, probiotic L. plantarum has been demonstrated to improve the gut barrier function and up-regulated inflammatory mediators when induced with lipopolysaccharides (92), and posed an immunomodulatory effect on intestinal epithelial and mononuclear cells (93). Similarly, the supplementation of postbiotics produced by L. plantarum strains to livestock ameliorated the inflammation incidence by up-regulated anti-inflammatory cytokines and down-regulated pro-inflammatory cytokines, while modulating immune response to prevent dysbiosis (21, 24, 28, 94). Moreover, postbiotic L. plantarum strains exerted a cytotoxicity effect on various cancer cells without causing hemolysis on erythrocytes obtained from humans and several animal species (95). This implies that the postbiotics of L. plantarum strains contain beneficial compounds such as organic acids, pyrrole compounds, and other intermediary compounds, which contributed to high antioxidant, antibacterial, anti-inflammatory, antimicrobial, and anticancer properties (96, 97). Besides that, this agreed with the finding of a positive correlation between the secretion of acetic acid, caproic acid, and various pyrrole and cyclic compounds detected in all the postbiotics with inhibitory activity and antioxidant activity. In other words, enhancement of postbiotic compositions would greatly enhance its functional characteristics.
In conclusion, the effect of formulated media on the compositions and functional characteristics of postbiotics produced by the six strains of L. plantarum was elucidated in this study. The composition and functional characteristics of postbiotics were strain-dependent, whereby postbiotic RG11, RI11, and RS5 exhibited higher inhibitory and antioxidant activities, which contained a higher concentration of acetic acid, caproic acid, and lactic acid. The current study also revealed that fermentation media were a prior important factor that exerted a great impact on the functionality characteristics of postbiotics such as the production of pyrrole compounds and other intermediary compounds, which contributed to high antioxidant, antibacterial, anti-inflammatory, antimicrobial, and anticancer properties. The postbiotics of L. plantarum strains produced by using a formulated medium warrant promising potential to be applied as a natural bioactive growth promoter for the livestock industry.
The raw data supporting the conclusions of this article will be made available by the authors, without undue reservation.
HMC, TCL, HLF, and EL took part in designing the experiment. HMC and NEA performed the experiment and data analysis, and wrote the paper. All authors participated in reading, provided a critical review, and approved the final manuscript.
This study was supported by the Fundamental Research Grant Scheme (FRGS/1/2017/WAB01/UPM/01/1) received from the Ministry of Higher Education, Malaysia.
The authors declare that the research was conducted in the absence of any commercial or financial relationships that could be construed as a potential conflict of interest.
1. Hussain T, Tan B, Yin Y, Blachier F, Tossou MC Rahu N. Oxidative stress and inflammation: what polyphenols can do for us? Oxid Med Cell Longev. (2016) 2016:7432797. doi: 10.1155/2016/7432797
2. Arulselvan P, Fard MT, Tan WS, Gothai S, Fakurazi S, Norhaizan ME, et al. Role of antioxidants and natural products in inflammation. Oxid Med Cell Longev. (2016) 2016:5276130. doi: 10.1155/2016/5276130
3. Qian M, Fang X, Wang X. Autophagy and inflammation. Clin Transl Medi. (2017) 6:24–35. doi: 10.1186/s40169-017-0154-5
4. Broom LJ, Kogut MH. Inflammation: friend or foe for animal production? Poult Sci. (2018) 97:510–14. doi: 10.3382/ps/pex314
5. Stober CB, Lange UG, Roberts MT, Alcami A, Blackwell JM. IL-10 from regulatory T cells determines vaccine efficacy in murine Leishmania major infection. J Immunol. (2005) 175:2517–24. doi: 10.4049/jimmunol.175.4.2517
6. Pitt JM, Stavropoulos E, Redford PS, Beebe AM, Bancroft GJ, Young DB, et al. Blockade of IL-10 signaling during bacillus Calmette-Guerin vaccination enhances and sustains Th1, Th17 and innate lymphoid IFN-γ and IL-17 responses and increases protection to Mycobacterium tuberculosis infection. J Immunol. (2012) 189:4079–87. doi: 10.4049/jimmunol.1201061
7. Cardoso Dal Pont G, Farnell M, Farnell Y, Kogut MH. Dietary factors as triggers of low-grade chronic intestinal inflammation in poultry. Microorganisms. (2020) 8:139–48. doi: 10.3390/microorganisms8010139
8. Jiang Z, Schatzmayr G, Mohnl M, Applegate TJ. Net effect of an acute phase response—partial alleviation with probiotic supplementation. Poult Sci. (2010) 89:28–33. doi: 10.3382/ps.2009-00464
9. Humphrey BD, Klasing KC. Modulation of nutrient metabolism and homeostasis by the immune system. World Poultry Sci J. (2004) 60:90–100. doi: 10.1079/WPS20037
10. Lescheid DW. Probiotics as regulators of inflammation: a review. Funct Food Health Dis. (2014) 4:299–11. doi: 10.31989/ffhd.v4i7.2
11. Azad M, Kalam A, Sarker M, Li T, Yin J. Probiotic species in the modulation of gut microbiota: an overview. Biomed Res Int. (2018) 2018: 9478630. doi: 10.1155/2018/9478630
12. Zheng J, Wittouck S, Salvetti E, Franz CM, Harris HM, Mattarelli P, et al. A taxonomic note on the genus Lactobacillus: description of 23 novel genera, emended description of the genus Lactobacillus Beijerinck 1901 and union of Lactobacillaceae and Leuconostocaceae. (2020) 70:2782–858. doi: 10.1099/ijsem.0.004107
13. Tsilingiri K, Rescigno M. Postbiotics: what else? Benef Microbes. (2013). 4:101. doi: 10.3920/BM2012.0046
14. Aguilar-Toala JE, Garcia-Varela R, Garcia HS, Mata-Haro V, Gonzalez-Cordova AF, Vallejo-Cordoba B, et al. Postbiotics: an evolving term within the functional foods fields. Trends Food Sci Technol. (2018) 75:105–14. doi: 10.1016/j.tifs.2018.03.009
15. Moradi M, Kousheh SA, Almasi H, Alizadeh A, Guimaraes JT, Yilmaz N, et al. Postbiotics produced by lactic acid bacteria: the next frontier in food safety. Compr Rev Food Sci Food Saf . (2020) 19:3390–415. doi: 10.1111/1541-4337.12613
16. Zolkiewicz J, Marzec A, Ruszcynski M, Feleszko W. Postbiotics—a step beyond pre- and probiotics. Nutrients. (2020) 12:2189–206. doi: 10.3390/nu12082189
17. Moradi M, Mardani K, Tajik H. Characterization and application of postbiotics of Lactobacillus spp. on Listeria monocytogenes in vitro and in food models. LWT. (2019) 111:457–64. doi: 10.1016/j.lwt.2019.05.072
18. Rajakovich LJ, Balskus EP. Metabolic functions of the human gut microbiota: the role of metalloenzymes. Nat Prod Rep. (2019) 36:593–25. doi: 10.1039/C8NP00074C
19. Liu Q, Yu ZM, Tian FW, Zhao J, Zhai Q, Chen W. Surface components and metabolites of probiotics for regulation of intestinal epithelial barrier. Microb Cell Fact. (2020) 19:23–35. doi: 10.1186/s12934-020-1289-4
20. Foo HL, Loh TC, Law FL, Lim YS, Kufflin CN, Rusul G. Effect of feeding L. plantarum I-UL4 isolated from Malaysian Tempeh on growth performance, fecal flora and lactic acid bacteria and plasma cholesterol concentrations in post weaning rats. J Food Sci Biotechnol. (2003). 12:403–8.
21. Van Thu T, Foo HL, Loh TC, Bejo MH. Inhibitory activity and organic acid concentrations of metabolite combinations produced by various strains of Lactobacillus plantarum. Afr J Biotechnol. (2011) 10:1359–63.
22. Kareem KY, Ling FH, Chwen LT, Foong OM, Asmara SA. Inhibitory activity of postbiotic produced by strains of Lactobacillus plantarum using reconstitute media supplemented with inulin. Gut Pathogens. (2014) 6:23–9. doi: 10.1186/1757-4749-6-23
23. Loh TC, Thu TV, Foo HL, Bejo MH. Effects of different levels of metabolite combination produced by Lactobacillus plantarum on growth performance, diarrhoea, gut environment and digestibility of postweaning piglets. J Appl Animal Res. (2013). 41:200–7. doi: 10.1080/09712119.2012.741046
24. Izuddin WI, Loh TC, Foo HL, Samsudin AA, Humam AM. Postbiotic L. plantarum RG14 improves ruminal epithelium growth, immune status and upregulates the intestinal barrier function in post-weaning lambs. Sci Rep. (2019). 9:1–10. doi: 10.1038/s41598-019-46076-0
25. Choe DW, Loh TC, Foo HL, Hair-Bejo M, Awis QS. Egg production, faecal pH and microbial population, small intestine morphology and plasma and yolk cholesterol in laying hens given liquid metabolites produced by Lactobacillus plantarum strains. Br Poult Sci. (2012) 53:106–15. doi: 10.1080/00071668.2012.659653
26. Loh TC, Thanh NT, Foo HL, Hair-Bejo M, Azhar BK. Feeding different levels of metabolite combinations on growth performance, fecal microflora, volatile fatty acid and villi height in broilers. Anim Sci J. (2010) 81:205–14. doi: 10.1111/j.1740-0929.2009.00701.x
27. Izuddin WI, Humam AM, Loh TC, Foo HL, Samsudin AA. Dietary postbiotic Lactobacillus plantarum improves serum and ruminal antioxidant activity and upregulates hepatic antioxidant enzymes and ruminal barrier function in post-weaning lambs. Antioxidants. (2020) 9:250–42. doi: 10.3390/antiox9030250
28. Humam AM, Loh TC, Foo HL, Samsudin AA, Mustapha NM, Zulkifli I, et al. Effects of feeding different postbiotics produced by Lactobacillus plantarum on growth performance, carcass yield, intestinal morphology, gut microbiota composition, immune status and growth gene expression in broilers under heat stress. Animals. (2019) 9:644–65. doi: 10.3390/ani9090644
29. Paynter MJB, Brown KA, Hayasaka SS. Factors affecting the production of an antimicrobial agent, plantaricin F, by Lactobacillus plantarum BF001. Lett Appl Microbiol. (1997) 24:159–65. doi: 10.1046/j.1472-765X.1997.00356.x
30. Bunch AW, Harris RE. The manipulation of microorganisms for the production of secondary metabolites. Biotechnol Genet Eng Rev. (2013) 4:117–44. doi: 10.1080/02648725.1986.10647825
31. Ooi MF, Mazlan N, Foo HL, Loh TC, Mohammad R, Rahim RA, et al. Effects of carbon and nitrogen sources on bacteriocin-inhibitory activity of postbiotic metabolites produced by Lactobacillus plantarum I-UL4. Malays J Microbiol. (2015) 1192:176–84. doi: 10.21161/mjm.13014
32. Singh V, Haque S, Niwas R, Srivastava A, Pasupuleti M, Tripathi CKM. Strategies for fermentation medium optimization: an in-depth review. Front Microbiol. (2017) 7:2087. doi: 10.3389/fmicb.2016.02087
33. Makowski K, Matusiak K, Borowski S, Bielnicki J, Tarazeqicz A, Maroszynska M, et al. Optimization of a culture medium using Taguchi approach for the production of microorganisms active in odorous compound removal. Appl Sci. (2017) 7:756–63. doi: 10.3390/app7080756
34. Kareem KY, Loh TC, Foo HL, Asmara SA, Akit H, Abdulla AR, et al. Carcass, meat and bone quality of broiler chickens fed with postbiotic and prebiotic combinations. Int J Probiotics Prebiotics. (2015) 10:23–30.
35. Lee YA. Purification and characterisation of bacteriocin produced by Lactococcus lactis subsp. Lactis RW18 isolated from steamed fish (Rastrelliger sp.) (Master thesis). Universiti Putra Malaysia, Malaysia (2002).
36. Lim YS. Isolation of bacteriocinogenic lactic acid bacteria and purification of selected bacteriocins from traditional fermented foods (Master thesis). Universiti Putra Malaysia, Malaysia (2003).
37. Foo HL, Loh TC, Lai PW, Lim YS, Kufli CN, Gulam R. Effects of adding Lactobacillus plantarum I-UL4 metabolites in drinking water of rats. Pak J Nutr. (2003) 2:283–8. doi: 10.3923/pjn.2003.283.288
38. Mohamad Zabidi NA, Foo HL, Loh TC, Mohamad R, Abdul Rahim R. Enhancement of versatile extracellular cellulolytic and hemicellulolytic enzyme productions by Lactobacillus plantarum RI11 isolated from Malaysian food using renewable natural polymers. Molecules. (2020) 25:2607–39. doi: 10.3390/molecules25112607
39. Xing JL, Wang G, Zhang QX, Liu XM, Gu ZN, Zhang H, et al. Determining antioxidant activities of Lactobacilli cell-free supernatant by cellular antioxidant assay: a comparison with traditional methods. PLoS ONE. (2014) 10:3.e0119058. doi: 10.1371/journal.pone.0119058
40. Nakkarach A, Foo HL, Song AAL, Nitisinprasert S, Withayagiat U. Promising discovery of beneficial Escherichia coli in the human gut. Biotech. (2020) 10:296–309. doi: 10.1007/s13205-020-02289-z
41. Borshchevskaya LN, Gordeeva TL, Kalinina AN, Sineokii SP. Spectrophotometric determination of lactic acid. J Anal Chem. (2016) 71:755–8. doi: 10.1134/S1061934816080037
42. Kam WY, Aida WW, Sahilah AM, Maskat MY. Volatile compounds and lactic acid bacteria in spontaneous fermented sourdough. Sains Malays. (2011) 40:135–8.
43. Kabir SM. Avian colibacillosis and salmonellosis: a closer look at epidemiology, pathogenesis, diagnosis, control and public health concerns. Int J Environ Res Public Health. (2010) 7:89–114. doi: 10.3390/ijerph7010089
44. Nhung NT, Chansiripornchai N, Carrique-Mas JJ. Antimicrobial resistance in bacterial poultry pathogens: a review. Front Vet Sci. (2017) 4:126. doi: 10.3389/fvets.2017.00126
45. Cox LM. Antibiotics shape microbiota and weight gain across the animal kingdom. Anim Front. (2016) 6:8. doi: 10.2527/af.2016-0028
46. Aasen IM, Moretro T, Katla T, Axelsson L, Storro I. Influence of complex nutrients, temperature and pH on bacteriocin production by Lactobacillus sakei CCUG 42687. Appl Microbiol Biotechnol. (2000) 53:159–66. doi: 10.1007/s002530050003
47. Abbasiliasi S, Ta JS, Ibrahim TAT, Bashokouh F, Ramakrishnan NR, Mustafa S, et al. Fermentation factors influencing the production of bacteriocins by lactic acid bacteria: a review. Res Adv. (2017) 7:29395–420. doi: 10.1039/C6RA24579J
48. Todorov SD, Dicks LMT. Effect of medium components on bacteriocin production by Lactobacillus plantarum strains ST23LD and ST341LD, isolated from spoiled olive brine. Microbiol Res. (2006) 161:102. doi: 10.1016/j.micres.2005.06.006
49. Todorov SD, Oliveira R, Vaz-Velho M. Media optimization of bacteriocin ST22CH production by Lactobacillus sakei SE22CH isolated from salpicao, a traditional meat-product from Portugal. Chem Eng Trans. (2012) 27:283–8. doi: 10.3303/CET1227048
50. Tai HF, Foo HL, Abdul Rahim R, Loh TC, Abdullah MP, Yoshinobu K. Molecular characterisation of new organisation of plnEF and plw loci of bacteriocin genes harbour concomitantly in Lactobacillus plantarum I-UL4. Microb Cell Fact. (2015) 14:89–102. doi: 10.1186/s12934-015-0280-y
51. Moghadam MS, Foo HL, Leow TC, Rahim RA, Loh TC. Novel bacteriogenic Lactobacillus plantarum strains and their differentiation by sequence analysis of 16S rDNA, 16S-23S and 23S-5S intergenic spacer regions and randomly amplified polymorphic DNA analysis. Food Technol Biotechnol. (2010) 48:476–83.
52. Choe DW, Foo HL, Loh TC, Hair-Bejo M, Awis QS. Inhibitory property of metabolite combinations produced by various strains of Lactobacillus plantarum strains. Pertanika J Trop Agric Sci. (2013) 36:79–88.
53. Savagog A, Quattara ATC, Bassaole IN, Traore SA. Bacteriocins and lactic acid bacteria—a mini review. Afr J Biotechnol. (2006) 5:678–83.
54. Hu CH, Ren LQ, Zhou Y, Ye BC. Characterization of antimicrobial activity of three Lactobacillus plantarum strains isolated from China traditional dairy food. Food Sci Nutr. (2019) 7:1997–005. doi: 10.1002/fsn3.1025
55. Thu TV, Loh TC, Foo HL, Yaakub H, Bejo MH. Effects of liquid metabolite combinations produced by Lactobacillus plantarum on growth performance, faeces characteristics, intestinal morphology and diarrhoea incidence in postweaning piglets. Trop Anim Health Prod. (2011) 43:69–75. doi: 10.1007/s11250-010-9655-6
56. Ghezzi P. Role of glutathione in immunity and inflammation in the lung. Int J Gen Med. (2011) 4:105–13. doi: 10.2147/IJGM.S15618
57. Ji K, Jang NY, Kim YT. Isolation of lactic acid bacteria showing antioxidative and probiotic activities from kimchi and infant feces. J Microbiol Biotechnol. (2015) 25:1568–77. doi: 10.4014/jmb.1501.01077
58. Riane K, Sifour M, Ouled-Haddar H, Idoui T, Bounar S, Boussebt S. Probiotic properties and antioxidant efficiency of Lactobacillus plantarum 15 isolated from milk. J Microbiol, Biotechnol Food Sci. (2020) 9:516–20. doi: 10.15414/jmbfs.2019/20.9.3.516-520
59. Xing Y, Wang L, Rui X, Li W, Chen X, Jiang M, Dong M. Enhancement of the antioxidant activity capacity of soy whey by fermentation with Lactobacillus plantarum B1-6. J Funct Foods. (2015) 12:33–44. doi: 10.1016/j.jff.2014.10.033
60. Yu X, Li S, Yang D, Qiu L, Wu Y, Wang D, et al. A novel strain of Lactobacillus mucosae isolated from a Gaotian villager improves in vitro and in vivo antioxidant as well as biological properties in D-galactose-induced aging mice. J Dairy Sci. (2016) 99:903–14. doi: 10.3168/jds.2015-10265
61. Huo G, Yan F, Li B. Screening for potential novel probiotics with dipeptidyl peptidase IV-inhibiting activity for type 2 diabetes attenuation in vitro and in vivo. Front Microbiol. (2019) 10:2855–68. doi: 10.3389/fmicb.2019.02855
62. Wang Y, Wu Y, Wang Y, Xu H, Mei X, Yu D, et al. Antioxidant properties of probiotic bacteria. Nutrients. (2017) 9:521–35. doi: 10.3390/nu9050521
63. Yang X, Li L, Duan Y, Yang X. Antioxidant activity of Lactobacillus plantarum JM113 in vitro and its protective effect on broiler chickens challenged with deoxynivalenol. J Anim Sci. (2017) 95:837–46. doi: 10.2527/jas.2016.0789
64. Li S, Zhao Y, Zhang L, Zhang X, Huang L, Li D, et al. Antioxidant activity of Lactobacillus plantarum strains isolated from traditional Chinese fermented foods. Food Chem. (2012) 135:1914–9. doi: 10.1016/j.foodchem.2012.06.048
65. Chen RH, Chen WX, Chen HM, Zhang GF, Chen WJ. Comparative evaluation of the antioxidant capacities, organic acids and volatiles of papaya juices fermented by Lactobacillus acidophilus and Lactobacillus plantarum. J Food Qual. (2018) 2018:1–12. doi: 10.1155/2018/9490435
66. Lin XN, Xia YJ, Wang WQ, Yang YJ, Xiong ZQ, Lv F, et al. Lactic acid bacteria with antioxidant activities alleviating oxidized oil induced hepatic injury in mice. Front Microbiol. (2018) 9:2684–93. doi: 10.3389/fmicb.2018.02684
67. Humam AM, Loh TC, Foo HL, Izuddin WI, Zulkifli I, Samsudin AA, et al. Supplementation of postbiotic RI11 improves antioxidant enzyme activity, upregulated gut barrier genes, and reduced cytokine, acute phase protein, and heat shock protein 70 gene expression levels in heat-stressed broilers. Poultry Sci. (2020). doi: 10.1016/j.psj.2020.12.011. [Epub ahead of print].
68. Huang L, Duan C, Zhao Y, Gao L, Niu C, Xu J, et al. Reduction of aflatoxin B1 toxicity by Lactobacillus plantarum C88: a potential probiotic strain isolated from Chinese traditional fermented food “tofu”. PLoS ONE. (2017) 12:e0170109. doi: 10.1371/journal.pone.0170109
69. Vinolo MA, Rodrigues HG, Nachbar RT, Curi R. Regulation of inflammation by short chain fatty acids. Nutrients. (2011) 3:858–76. doi: 10.3390/nu3100858
70. Pelaseyed T, Bergstrom JH, Gustafsson JK, Ermund A, Birchenough GM, Schutte A, et al. The mucus and mucins of the goblet cells and enterocytes provide the first defense line of the gastrointestinal tract and interact with the immune system. Immunol Rev. (2014) 260:8–20. doi: 10.1111/imr.12182
71. Allen A, Flemstrom G. Gastroduodenal mucus bicarbonate barrier: protection against acid and pepsin. Am J Physiol Cell Physiol. (2005) 288:1–19. doi: 10.1152/ajpcell.00102.2004
72. Dalile B, Van Oudenhove L, Vervliet B, Verbeke K. The role of short-chain fatty acids in micorbiota-gut-brain communication. Nat Rev Gastroenterol Hepatol. (2019) 16:461–78. doi: 10.1038/s41575-019-0157-3
73. Lewis K, Lutgendorff F, Phan V, Soderholm JD, Sherman PM, McKay DM. Enhanced translocation of bacteria across metabolically stresses epithelia is reduced by butyrate. Inflamm Bowel Dis. (2010) 16:1138–48. doi: 10.1002/ibd.21177
74. Schonfeld P, Wojtczak L. Short-and medium-chain fatty acids in energy metabolism: the cellular perspective. J Lipid Res. (2016) 52:943–54. doi: 10.1194/jlr.R067629
75. Desbois AP, Smith VJ. Antibacterial free fatty acids: Activities, mechanisms of action and biotechnological potential. Appl Microbiol Biotechnol. (2010) 85:1629–42. doi: 10.1007/s00253-009-2355-3
76. Van Immerseel F, De Buck J, Boyen F, Bohez L, Pasmans F, Volf M, et al. Medium-chain fatty acids decrease colonization and invasion through hila suppression shortly after infection of chickens with Salmonella enterica serovar Enteritidis. Appl Environ Microbiol. (2004) 70:3582–7. doi: 10.1128/AEM.70.6.3582-3587.2004
77. Hajati H. Application of organic acids in poultry nutrition. Int J Avian Wildl Biol. (2018) 3:324–9. doi: 10.15406/ijawb.2018.03.00114
78. Mora-Villalobos JA, Montero-Zamora J, Barboza N, Rojas-Garbanzo C, Redondo-Solano UM, Schrodter L, et al. Multi-product lactic acid bacteria fermentations: a review. Fermentation. (2020) 6:23–44. doi: 10.3390/fermentation6010023
79. Bergsson G, Arnfinnsson J, Steingrimsson O, Thormar H. In vitro killing of Candida albicans by fatty acids and monoglycerides. Antimicrobl Agents Chemother. (2001) 45:3209–12. doi: 10.1128/AAC.45.11.3209-3212.2001
80. Servin AL. Antagonistic activities of lactobacilli and bifidobacteria against microbial pathogens. FEMS Microbiol Rev. (2004) 28:405–40. doi: 10.1016/j.femsre.2004.01.003
81. Alakomi HL, Skytta E, Saarela M, Mattila-Sandholm T, Latva-Kala K, Helander IM. Lactic acid permeabilizes gram-negative bacteria by disrupting the outer membrane. Appl Environ Microbiol. (2000) 66:2001–5. doi: 10.1128/AEM.66.5.2001-2005.2000
82. Wang C, Chang T, Yang H Cui M. Antibacterial mechanism of lactic acid on physiological and morphological properties of Salmonella enteritidis, Escherichia coli and Listeria monocytogenes. Food Control. (2015) 47:231–6. doi: 10.1016/j.foodcont.2014.06.034
83. Zalan Z, Hudacek J, Stetina J, Chumchalova J, Halasz A. Production of organic acids by Lactobacillus strains in three different media. Eur Food Res Technol. (2010) 230:395–404. doi: 10.1007/s00217-009-1179-9
84. Jankowska E, Chwialkowska J, Stodolny M, Oleskowicz-Popiel P. Volatile fatty acids production during mixed culture fermentation—the impact of substrate complexity and pH. Chem Eng J. (2017) 326:901–10. doi: 10.1016/j.cej.2017.06.021
85. Xiao ZJ, Wang XM, Huang YL, Huo FF, Zhu XK, Xi LJ, et al. Thermophilic fermentation of acetoin and 2,3-butanediol by a novel Geobacillus strain. Biotechnol Biofuels. (2012). 5:88–98. doi: 10.1186/1754-6834-5-88
86. Yu XY, Zhao MY, Liu F, Zeng ST, Hu J. Identification of 2-3,dihydro-3,5-dihydroxy-6methyl-4H-pyran-4-one as a strong antioxidant in glucose-histidine Maillard reaction products. Food Res Int. (2013). 51:397–403. doi: 10.1016/j.foodres.2012.12.044
87. Teoh YP, Mat MD. Mycelia growth and production of total flavonoids and 4H-pyran-4-one, 2,3-dihydro-3,5-dihydroxy-6-methyl- by Schizophyllum commune using a bubble column bioreactor considering aeration effect and mass transfer study. Chem Biochem Eng Q. (2014) 28:553–9. doi: 10.15255/CABEQ.2014.2024
88. Takaya Y, Furukawa T, Miura S. Antioxidant constituents in distillation residue of Awamori spirits. J Agric Food Chem. (2007). 55:75–9. doi: 10.1021/jf062029d
89. Manimaran M, Kannabiran K. Marine Streptomyces Sp. VITMK1 Derived Pyrrolo [1,2-A] Pyrazine-1,4-Dione, Hexahydro-3-(2-Methylpropyl) and its free radical scavenging activity. Open Bioactive Comp J. (2017) 5:23–30. doi: 10.2174/1874847301705010023
90. Kiran GS, Priyadharsini S, Sajayan A, Ravindran A, Selvin J. An antibiotic agent pyrrolo [1,2-a] pyrazine-1,4-dione, hexahydro isolated from a marine bacteria Bacillus tequilensis MSI45 effectively controls multi-drug resistant Staphylococcus aureus. R Sci Chem. (2018) 8:17837. doi: 10.1039/C8RA00820E
91. Toshimitsu T, Ozaki S, Mochizuki J, Furuichi K, Asami Y. Effects of Lactobacillus plantarum strain OLL2712 culture conditions on the anti-inflammatory activities for murine immune cells and obese and type 2 diabetes mice. Appl Environ Microbiol. (2017) 83:e03001-16. doi: 10.1128/AEM.03001-16
92. Lee CS, Kim SH. Anti-inflammatory and anti-osteoporotic potential of Lactobacillus plantarum A41 and L. fermentum SRK414 as probiotics. Probiotics Antimicrob Proteins. (2020) 12:623–34. doi: 10.1007/s12602-019-09577-y
93. Ferreira dos Santos T, Alves Melo T, Almeida ME, Passos Rezende R, Romano CC. Immunomodulatory effects of Lactobacillus plantarum LP62 on intestinal epithelial and mononuclear cells. Biomed Res Int. (2016) 2016:8404156. doi: 10.1155/2016/8404156
94. Kareem KY, Loh TC, Foo HL, Akit H, Samsudin AA. Effects of dietary postbiotic and inulin on growth performance, IGF1 and GHR mRNA expression, faecal microbiota and volatile fatty acids in broilers. BMC Vet Res. (2016) 12:163–72. doi: 10.1186/s12917-016-0790-9
95. Chuah LO, Foo HL, Loh TC, Noorjahan BMA, Yeap SK, NurElina AM, et al. Postbiotic metabolites produced by Lactobacillus plantarum strains exert selective cytotoxicity effects on cancer cells. BMC Complement Altern Med. (2019) 19:114–28. doi: 10.1186/s12906-019-2528-2
96. Ndagano D, Lamourex T, Dortu C, Vandermoten S, Thonart P. Antifungal activity of 2 lactic acid bacteria of the Weissella genus isolated from food. J Food Sci. (2011) 76:356–65. doi: 10.1111/j.1750-3841.2011.02257.x
Keywords: Lactiplantibacillus plantarum, postbiotic, natural bioactive ingredients, organic acids, antimicrobial, antioxidant, anti-inflammatory
Citation: Chang HM, Foo HL, Loh TC, Lim ETC and Abdul Mutalib NE (2021) Comparative Studies of Inhibitory and Antioxidant Activities, and Organic Acids Compositions of Postbiotics Produced by Probiotic Lactiplantibacillus plantarum Strains Isolated From Malaysian Foods. Front. Vet. Sci. 7:602280. doi: 10.3389/fvets.2020.602280
Received: 02 September 2020; Accepted: 11 December 2020;
Published: 26 January 2021.
Edited by:
Luisella Verotta, University of Milan, ItalyReviewed by:
Manuel Gonzalez Ronquillo, Universidad Autónoma del Estado de México, MexicoCopyright © 2021 Chang, Foo, Loh, Lim and Abdul Mutalib. This is an open-access article distributed under the terms of the Creative Commons Attribution License (CC BY). The use, distribution or reproduction in other forums is permitted, provided the original author(s) and the copyright owner(s) are credited and that the original publication in this journal is cited, in accordance with accepted academic practice. No use, distribution or reproduction is permitted which does not comply with these terms.
*Correspondence: Hooi Ling Foo, aGxmb29AdXBtLmVkdS5teQ==; Teck Chwen Loh, dGNsb2hAdXBtLmVkdS5teQ==
Disclaimer: All claims expressed in this article are solely those of the authors and do not necessarily represent those of their affiliated organizations, or those of the publisher, the editors and the reviewers. Any product that may be evaluated in this article or claim that may be made by its manufacturer is not guaranteed or endorsed by the publisher.
Research integrity at Frontiers
Learn more about the work of our research integrity team to safeguard the quality of each article we publish.