- 1Laboratory of Poultry Medicine, Seoul National University, Seoul, South Korea
- 2Research Institute for Veterinary Science, College of Veterinary Medicine, BK21 for Veterinary Science, Seoul, South Korea
- 3Laboratory of Avian Diseases, College of Veterinary Medicine, Seoul National University, Seoul, South Korea
- 4Farm Animal Clinical Training and Research Center (FACTRC), GBST, Seoul National University, Seoul, South Korea
Fowl typhoid is caused by Salmonella enterica serovar Gallinarum biovar Gallinarum (SG), and live attenuated, rough vaccine strains have been used. Both humoral and cellular immune responses are involved in protection, but the humoral responses to different forms of SG antigens are unclear. In this study, we compared humoral responses to a killed oil-emulsion (OE) smooth vaccine (SG002) and its rough mutant vaccine (SR2-N6) strains using proteomics techniques. We identified two immunogenic outer membrane proteins (OmpA and OmpX), and the selected linear epitopes were successfully applied in peptide-ELISA. Our peptide- and total OMP-ELISAs were used to compare the temporal humoral responses to various SG antigens: OE SG002 and SR2-N6; live, killed [PBS-suspension (PS) and OE)] and mixed (live and PS) formulations of another rough vaccine strain (SG 9R); and orally challenge with a field strain. Serum antibodies to the linear epitopes of OmpA and OmpX lasted only for the first 2 weeks, but serum antibodies against OMPs increased over time. The rough strain (SR2-N6) and mixed SG 9R induced higher serum antibody titers than the smooth strain (SG002) and single SG 9R (OE, live and PS SG 9R), respectively. Infection with the field strain delayed the serum antibody response by ~2 weeks. Mucosal immunity was not induced by any formulation, except for infection with the field strain after SG 9R vaccination. Thus, our results may be useful to understand humoral immunity against various SG antigens and to improve vaccine programs and serological diagnosis in the field.
Introduction
Salmonella enterica serovar Gallinarum biovar Gallinarum (SG) is a pathogen causing fatal and persistent infection, fowl typhoid (FT) (1, 2). Both humoral and cell-mediated immune responses are required to prevent mortality and achieve bacterial clearance (3). A live vaccine strain, SG 9R, mimics infection of pathogenic field strains, and has been used to prevent FT worldwide (4).
The potent immunostimulatory effect of lipopolysaccharide (LPS) is mediated by O-Ag and lipid A, which induce T cell-independent humoral and TLR4-mediated innate immune responses, respectively (5). Although LPS induces a strong humoral immune response to concomitantly inoculated antigens, LPS on the surface of bacteria may also shield or compete with outer membrane proteins (OMPs), resulting in decreased immunogenicity of OMP (6, 7). Therefore, while SG 9R is a rough strain with defective outer-core and O-antigen regions (O-Ag) of lipopolysaccharide (LPS), it may induce a different humoral immune response from field strains against OMP (8). The protective efficacy of OMP vaccines has already been established, and protective OMPs of S.enterica serovars have been identified for vaccine development (9, 10).
Although SG 9R has been commonly used in the field, it displays potential pathogenicity and may cause mortality and gross lesions in the liver under immunosuppressive conditions (8). Therefore, SG 9R was not recommended for use in chicks under 6 weeks old (w-o) who are most susceptible and may become carriers (4, 11). For this reason, killed vaccines, if possible, need to be considered, but basic data on the differences in humoral immune responses to different forms of SG antigens (oil-emulsion, killed, smooth vs. rough SG; live vs. killed with or without oil adjuvant vs. a mixture of live and killed SG 9R; or field strain) are insufficient. In addition, humoral immunity against natural infection with field strains is unclear. Humoral immunity to live or killed bacteria is the sum of antibodies directed to multiple antigens and their epitopes. Therefore, investigations of a single epitope-specific antibody in the antiserum against different antigens using single peptide epitopes may provide more insights into the kinetics of humoral immunity. In this study, we compared humoral immune responses to smooth and rough SG strains and identified immunogenic OMPs and their linear epitopes. We developed linear epitope-based peptide-ELISAs to compare humoral immune responses to different forms of SG antigens, and the results were compared with data from the OMP-ELISA.
Materials and Methods
Bacteria, Serum Samples, and Experimental Birds
A commercial rough vaccine strain, SR2-N6 (DAE SUNG Microbiological Lab., Uiwang-si, Korea), and its parent strain SG002 were used to compare the effect of LPS on humoral immunity, and a commercial rough vaccine strain, SG 9R, was purchased from the manufacturer (Nobilis; Intervet International, Boxmeer, the Netherlands) (12). SG0197, a virulent strain isolated from commercial chickens in 2001, was used to observe the immune response of challenged chickens (12). The strains were cultured in Luria-Bertani broth (Duchefa Biochemie, Groot Bijgaarden, Belgium) with shaking at 37°C overnight.
One d-o male Hy-Line brown layer chicks without SG vaccination were purchased from a farm (Yangji Farm, Pyeongtaek-si, Korea) and reared for animal experiments to compare humoral immune responses to different forms of SG antigens. Feed and water were supplied ad libitum.
Fifty-six field serum samples obtained for serological tests from 6 layer and breeder farms were used to determine humoral immunity to SG in the field. In detail, L1D included 10 samples from 1-day-old (d-o) layer chicks, L12W included 10 samples from 12-w-o layer chickens vaccinated at 10 w-o, L19W included 10 samples from 19-w-o layer chickens vaccinated twice at 8 and 15 w-o, and L41W included 10 samples from 41-w-o layer chickens vaccinated twice at 7 and 16 w-o. PS18W and PS23W included 10 and 6 samples from 18 and 23-w-o parent stocks, respectively.
2D-Gel Electrophoresis, Immunoblotting, and LC-MS/MS
Total bacterial proteins were extracted via cell lysis with 7 M urea, 2 M thiourea, 4% CHAPS (3-[(3-cholamidopropyl)dimethylammonio]-1-propanesulfonate), and 2.5% dithiothreitol (DTT) and quantified with the Bradford protein assay. OMPs were extracted using the previously described sodium lauroyl sarcosine (SLS) method (13), with some modifications. Briefly, the cultured bacteria were centrifuged and the pellet was washed with 50 mM Tris-HCl. After centrifugation, lysis buffer (50 mM Tris-HCl and 150 mM NaCl) was added, and the cells were lysed by ultrasonication. The supernatant was ultracentrifuged at 100,000 × g for 1 h. Pellets were resuspended in 2% SLS and 50 mM Tris-HCl, and then incubated at room temperature for 40 min. After another ultracentrifugation step, pellets were stored by adding 1% Triton X-100 to the lysis buffer.
2D-gel electrophoresis of total proteins or OMPs was performed using isoelectric focusing (pH 3-10 for whole bacteria or pH 4-7 for OMPs) and 14% SDS-PAGE gels, and separated proteins were electrotransferred to nitrocellulose membranes for western blotting (ProteomeTech, Seoul, Korea) (14). Membranes were incubated with anti-SR-N6 (1:10,000 dilution) and anti-SG002 (1:5,000 dilution) serum samples. LC-MS/MS was performed as described below. The analysis was performed using a nano ACQUITY UPLC and LTQ-Orbitrap-mass spectrometer (Thermo Electron, San Jose, CA). One of the mobile phases for LC separation was 0.1% formic acid in deionized water, and the other was 0.1% formic acid in acetonitrile. The flow rate was 0.5 μl/min, and the transfer tube temperature was set to 160°C. The MS/MS data were interpreted using SEQUEST software (Thermo Quest, San Jose, CA, USA), and the generated peak lists were compared using the MASCOT program (Matrix Science Ltd., London, UK).
B Cell Epitope Prediction and Peptide Synthesis
B cell epitopes were predicted by the IEDB B cell epitope prediction program (http://tools.iedb.org/bcell/), and they were located on the 3D structure files of corresponding proteins generated with PyMOL 2.2 (Schrodinger, New York, USA). Selected peptides were synthesized with a modification of the N-terminus by adding aminocaproic acid for better performance of the peptide-ELISA (Cosmogenetech, Seoul, Korea).
ELISA
Synthesized peptides (1 μl/ml) or SG 9R OMP extracts (105 ng/ml) in 100 mM sodium bicarbonate/carbonate coating buffer (pH 9.6) were used to coat an immunoplate (SPL Life Science, Pocheon-si, Korea) at 4°C overnight. Antigen-coated wells were washed twice with PBST (PBS containing 0.5% Tween 20) and blocked with 1% bovine serum albumin (BSA) (GenDEPOT, Katy, USA) at room temperature for 2 h. After washing the plates as described above, the primary antibody, which was serum or bile juice (1:300 in PBST containing 1% BSA), was added, incubated for 30 min, and then the plate was washed 4 times with PBST. The secondary antibody, an HRP-conjugated goat-anti chicken IgG or IgA antibody (Bethyl, Laboratories, Montgomery, USA; diluted 1:10,000 in PBST containing 1% BSA), was added for 30 min, and the plate was washed as described above. TMB substrate (SurModics, Eden Prairie, USA) was added for 10 min, and the OD was measured at 450 nm after the addition of stop solution. We used a commercial Salmonella D group ELISA kit to test the anti-O-Ag antibody according to the manufacturer's recommendation (BioChek BV., Reeuwijk, the Netherlands).
Inactivation and Preparation of Oil-Emulsion (OE) SG
Cultured bacteria were centrifuged and washed once with PBS. Bacteria were inactivated at 65°C for 2 h in a water bath and cooled gradually to room temperature. The inactivation was confirmed by culture on Mueller Hinton Agar (Duchefa Biochemie, Groot Bijgaarden, Belgium). The live and heat-inactivated bacteria were diluted to 1 × 107 cfu/100 μl and 1 × 109 cfu/100 μl in PBS, respectively. The live and killed mixture was prepared by mixing the same volume of both preparations of bacteria to obtain 200 μl. The OE bacteria were prepared by emulsifying heat-inactivated bacteria with oil adjuvant (Montanide ISA 70, Seppic Co., Courbevoie, France) at a ratio of 3 to 7 (~3.3 × 108 cfu/100 μl of OE) (Table 1).
Animal Experiments
Fifteen (5 chickens in each group) 3-w-o male brown layer chickens were divided into SR2-N6, SG002, and negative control groups to compare the humoral immune responses to smooth (SG002) and rough (SR2-N6) strains, respectively. OE SR2-N6 and OE SG002 were inoculated via the intramuscular route (100 μl/chicken), and serum samples were collected weekly for up to 3 weeks postinoculation (wpi). Bile juice samples were collected from the gall bladder at 3 wpi with a 1 ml syringe. Specific antibodies in serum (IgG) and bile juice (IgA) samples were measured using the ELISA.
Forty 3-w-o male brown layer chickens were assigned to OE SG 9R (10), live SG 9R (10), mixed SG 9R (10), PS (PBS –suspension) SG 9R (5), and negative control groups (5) to compare the humoral immune responses to different forms of SG 9R. All groups were inoculated via the intramuscular route (100 μl/chicken), and serum samples were collected weekly for up to 3 wpi. Bile juice samples were collected at 3 wpi as described above. Specific antibodies in serum and bile juice samples were measured using the ELISA.
Fifteen 4-w-o chickens were infected with the field strain (SG0197, 1 × 106 cfu/0.1 ml/chicken) per os, and serum samples were collected from the surviving chickens weekly for 4 wpi. Bile juice samples were collected after 3 days of starvation at 4 wpi, as described above. Specific antibodies in serum and bile juice samples were measured using the ELISA.
Twenty 6-w-o chickens were divided into SG 9R vaccine and no vaccine groups to compare the mucosal immune responses. The SG 9R vaccine group was vaccinated with SG 9R (1 × 107 cfu/100 μl/chicken) via the intramuscular route, and both groups were challenged with SG0197 at 2 wpi (8 w-o). After 2 wpi, SG0197 bile juice samples were collected as described above, and specific antibodies were measured using the ELISA.
All animal experiments were approved by the Institutional Animal Care and Use Committee of BioPOA Co. (permission number BP-2019-C31-1).
Statistical Analysis
Analyses were performed with SPSS Statistics version 26.0 (SPSS, Chicago, IL, USA). One-way ANOVA was used to analyze significant differences between the groups, followed by the Bonferroni post-hoc test (Figure 2C 1 wpi and 3 wpi, Figure 2D 1 wpi, Figures 5A,C). When unequal variance was observed, the Welch test was used for the analysis, and the Games-Howel test was performed as the post hoc test (Figure 2B 2 wpi, Figure 2C 2 wpi, Figure 2D 2 wpi and 3wpi, Figure 3A 2 wpi, Figure 3B 2 wpi, Figure 3C 1 wpi, Figure 3D 1-3 wpi). Data with a non-normal distribution were subjected to the Kruskal-Wallis H-test, and the Bonferroni correction was used as the post-hoc test (Figure 2A 1-3 wpi, Figure 2B 1 wpi and 3 wpi, Figure 3A 1 wpi and 3 wpi, Figure 3B 1 wpi and 3 wpi, Figure 3C 2 wpi and 3 wpi, Figures 4, 5B,D, 6). If only two groups were analyzed, the significance was determined with the t-test for data with a normal distribution (Figures 5E–G), and the Mann-Whitney U-test was used for data with a non-normal distribution (Figures 5H,I). Statistical significance was considered when the p < 0.05.
Results
Comparison of Humoral Immune Responses to Smooth (SG002) and Rough (SR2-N6) Strains
In contrast to anti-SR2-N6 serum samples, anti-SG002 serum samples showed a strong antibody reaction to O-Ag (at least two distinct ladders at different isoelectric points) (Figure 1A, black dotted rectangle). The different spots recognized by the anti-SR2-N6 serum sample were analyzed with LC-MS/MS. Interestingly, most of the spots were not OMP and included translation elongation factor G, GroEL, phosphoglycerate kinase, elongation factor Tu, electron transfer flavoprotein subunit beta, etc. (Supplementary Table 1). To identify immunogenic OMPs, we performed 2D-gel electrophoresis and immunoblotting with OMPs of SR2-N6 and anti-SR2-N6 serum samples to identify major antigens. The three major antigen spots were identified to be OmpA (spots a and b) and OmpX (spot c) (Figure 1C, Table 2). We selected candidate peptides for the peptide-ELISA according to the amino acid sequences of OmpA (CAR36850) and OmpX (CAR36706) (Table 3).
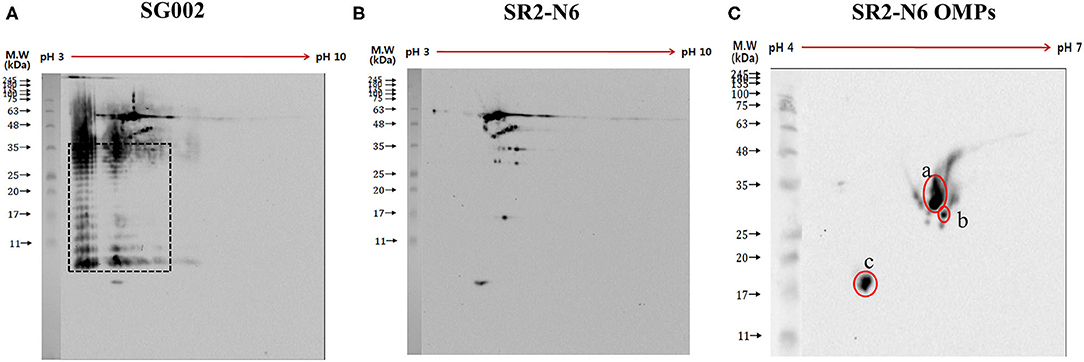
Figure 1. Antigens of SG002 were tested against antiserum of SG002 (A) and SR2-N6 (B) using 2DE western blotting. Different blots obtained in (A,B) were analyzed using LC-MS/MS (Supplementary Table 1). Distinct ladders at different isoelectric points represented the O antigen (black dotted rectangle). Omp extracts of SR2-N6 were also tested against the antiserum of SR2-N6 (C) using 2DE western blotting. Three spots (a, b, and c) were analyzed with LC-MS/MS (Table 2).
A pilot study with the synthesized peptides (OmpA-N-L1, OmpA-N-L2, OmpA-N-L3, OmpA-N-L4, OmpX-L1, and OmpX-L2) and anti-SG002 and anti-SR2-N6 serum samples revealed that the reactivity of OmpA-N-L1, OmpA-N-L2, and OmpX-L1 was too low to differentiate responses from anti-SG002 and anti-SR2-N6 serum samples. We selected OmpA-N-L3, OmpA-N-L4, and OmpX-L2 for the peptide-ELISA. According to the results, the anti-SR2-N6 antibody titer was significantly higher than the anti-SG002 antibody titer in the OmpX-L2 and OMP-ELISA at 1 week postinoculation (wpi) (Figures 2C,D). All the anti-SR2-N6 and anti-SG002 serum samples showed significantly higher OD values than the negative control only for the first 2 weeks using peptide-ELISAs, except for OmpX_L2. However, in the OMP-ELISA, significantly higher OD values were observed than the negative control, with a gradual increase during the observation period.
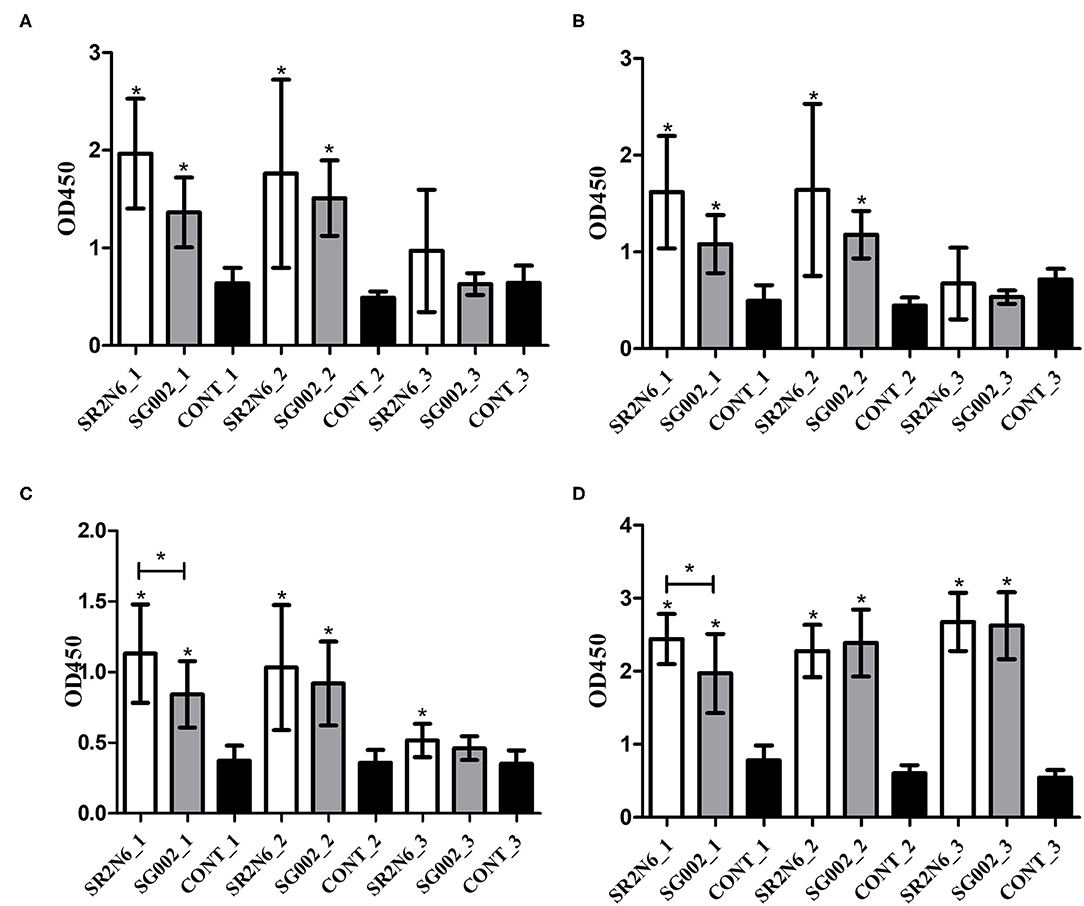
Figure 2. Humoral immune responses to the smooth strain (SG002) and rough strain (SR2-N6) measured using ELISAs (mean with SD): OmpA-N-L3 (A), OmpA-N-L4 (B), OmpX-L2 (C), and OMP ELISAs (D). *Indicates a significant difference [P-values - (A) SR2-N6 vs. CONT at 1 wpi (0.000) and 2 wpi (0.001); SG002 vs. CONT at 1 wpi (0.008) and 2 wpi (0.000); (B) SR2-N6 vs. CONT at 1 wpi (0.000) and 2 wpi (0.005); SG002 vs. CONT at 1 wpi (0.01) and 2 wpi (0.000); (C) SR2-N6 vs. SG002 at 1 wpi (0.046); SR2-N6 vs. CONT at 1 wpi (0.000), 2 wpi (0.002), and 3 wpi (0.003); SG002 vs. CONT at 1 wpi (0.001) and 2 wpi (0.000); (D) SR2-N6 vs. SG002 at 1 wpi (0.035); SR2-N6 vs. CONT at 1 wpi (0.000), 2 wpi (0.000), and 3 wpi (0.000), SG002 vs. CONT at 1 wpi (0.000), 2 wpi (0.000), and 3 wpi (0.000)].
Comparison of Humoral Immune Responses to Live, Killed and Mixture of Live, and Killed Rough Vaccine Strains (SG 9R)
The OD values of anti-OE SG 9R, anti-live SG 9R, and anti-mixed SG 9R serum samples were not significantly different from each other, and produced higher OD values than the anti-PS SG 9R serum samples and negative control samples at 1 and 2 wpi in the peptide-ELISAs (Figure 3). Interestingly, anti-mixed SG 9R showed significantly higher OD values than the negative control in the OmpA-N-L3 and OmpA-N-L4 peptide-ELISAs at 3 wpi. According to the results of the OMP-ELISA, anti-OE SG 9R, anti-SG 9R, and anti-mixed SG 9R serum samples showed significantly higher OD values than the negative control samples with a gradual increase over time. Anti-PS SG 9R serum samples did not show significantly higher OD values than the negative control in either peptide- or OMP-ELISAs.
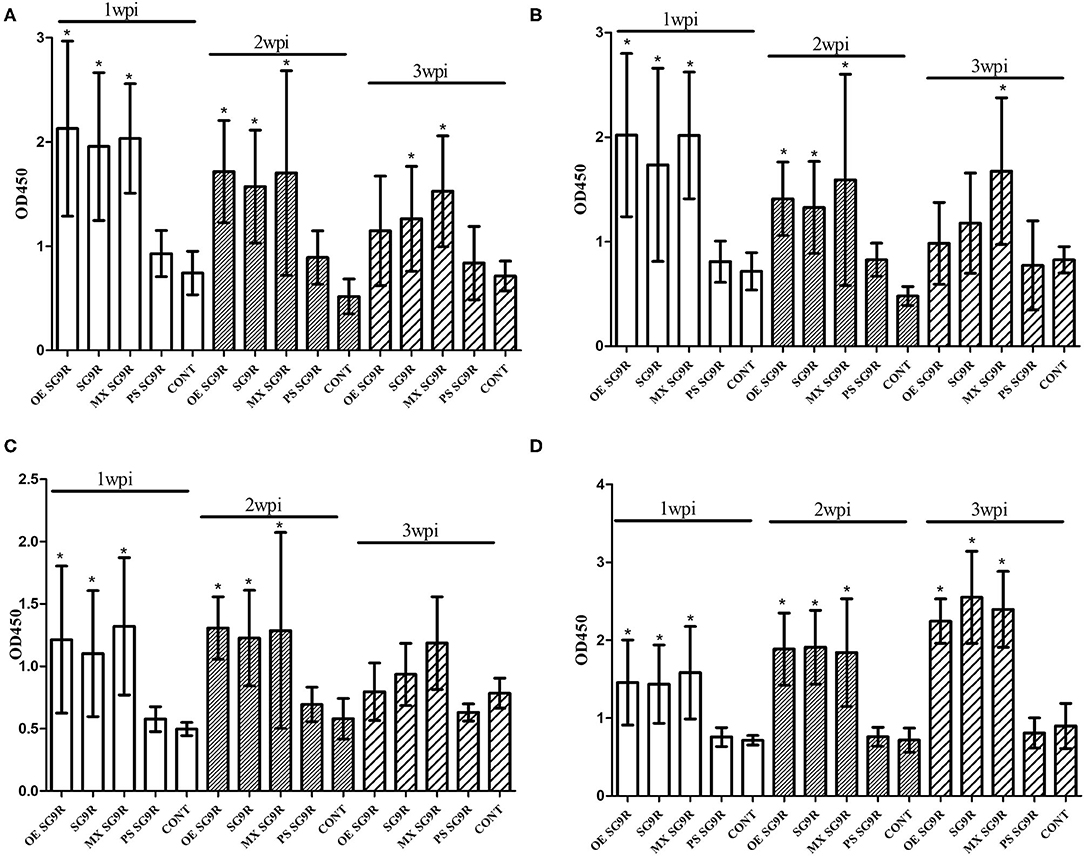
Figure 3. Humoral immune responses measured using ELISAs under various conditions (mean with SD): OmpA-N-L3 (A), OmpA-N-L4 (B), OmpX-L2 (C), and OMP-ELISA (D). SG 9R was inoculated as killed adjuvanted (OE), live, mixed with live and killed (MX), or killed bacteria in a PBS suspension (PS) and compared with the negative control (CONT). *Indicates a significant difference compared with CONT [P-values - (A) OE SG 9R at 1 wpi (0.000) and 2 wpi (0.000); SG 9R at 1 wpi (0.000), 2 wpi (0.000), and 3 wpi (0.048); MX SG 9R at 1 wpi (0.000), 2 wpi (0.000), and 3 wpi (0.000); (B) OE SG 9R at 1 wpi (0.001) and 2 wpi (0.000); SG 9R at 1 wpi (0.019) and 2 wpi (0.000); MX SG 9R at 1 wpi (0.000), 2 wpi (0.001), and 3 wpi (0.022); (C) OE SG 9R at 1 wpi (0.000) and 2 wpi (0.000); SG 9R at 1 wpi (0.000) and 2 wpi (0.000); MX SG 9R at 1 wpi (0.000) and 2 wpi (0.006); (D) OE SG 9R at 1 wpi (0.013), 2 wpi (0.000), and 3 wpi (0.000); SG 9R at 1 wpi (0.009), 2 wpi (0.000), 3 wpi (0.000); MX SG 9R at 1 wpi (0.008), 2 wpi (0.004), and 3 wpi (0.000)].
We tested two additional peptides (OmpA-C-L1 and OmpA-C-L2) in the C-terminal domain of OmpA using the peptide-ELISA (Table 3). The anti-OE SG 9R serum samples showed significantly higher OD values than the negative control at 1 and 2 wpi, but not at 3 wpi (Supplementary Figure 1).
Humoral Immunity Against Natural Infection With a Field Strain (SG0197)
SG0197 infection caused 86.7% (13/15) mortality within 4 weeks (7/15 at 2 wpi, 5/15 at 3 wpi and 1/15 at 4 wpi); therefore, the numbers of serum samples were 8 at 2 wpi, 3 at 3 wpi, and 2 at 4 wpi as the number of surviving chickens decreased. The serum samples from the challenged group only showed significantly higher OD values than the negative control group at 3 wpi using peptide-ELISAs (Figure 4C) but significantly higher OD values at 3 and 4 wpi than the negative control group using the OMP-ELISAs (Figure 4D).
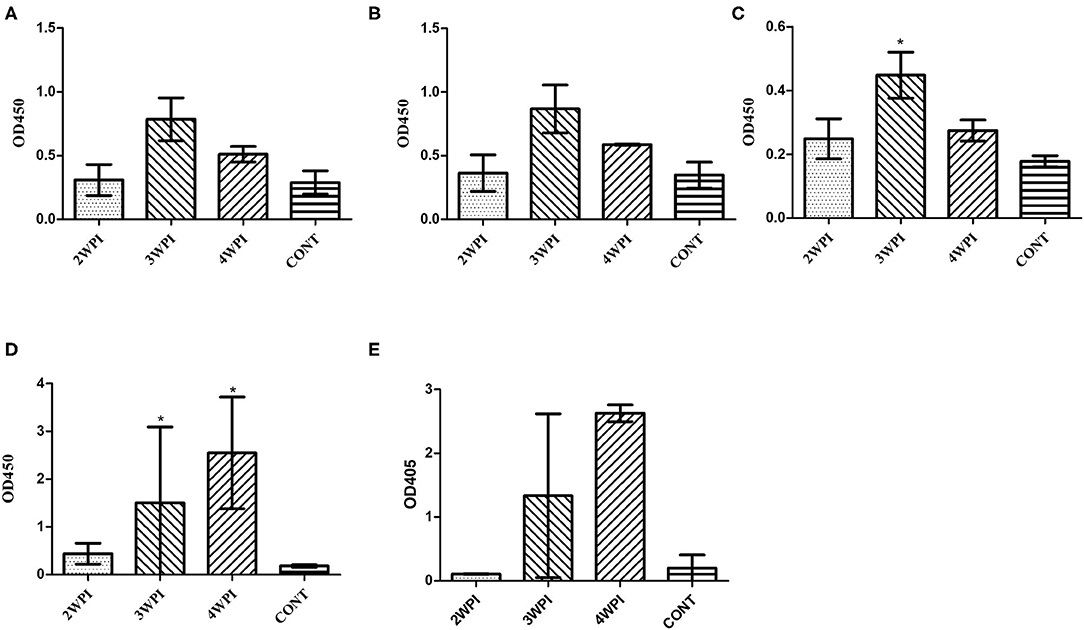
Figure 4. Evaluation of humoral immunity in response to challenge with a field strain (mean with SD) using the OmpA-N-L3 (A), OmpA-N-L4 (B), OmpX-L2 (C), OMP-ELISA (D), and Salmonella D group O-Ag ELISA (E). The field strain SG0197 was infected per os at 4 weeks of age, and serum samples were collected weekly for 4 weeks. *Indicates a significant difference compared with CONT [P-values - (C) 3 wpi (0.016); (D) 3 wpi (0.036) and 4 wpi (0.017)].
Comparison of Mucosal Immunity Against Various SG Antigens
The anti-OE SG 9R, anti-SG 9R, anti-mixed SG 9R, anti-PS SG 9R, anti-OE SR2-N6, and anti-OE SG002 IgA levels in bile juice samples were not significantly different from the negative control using peptide- and OMP-ELISAs (Figures 5A–D).
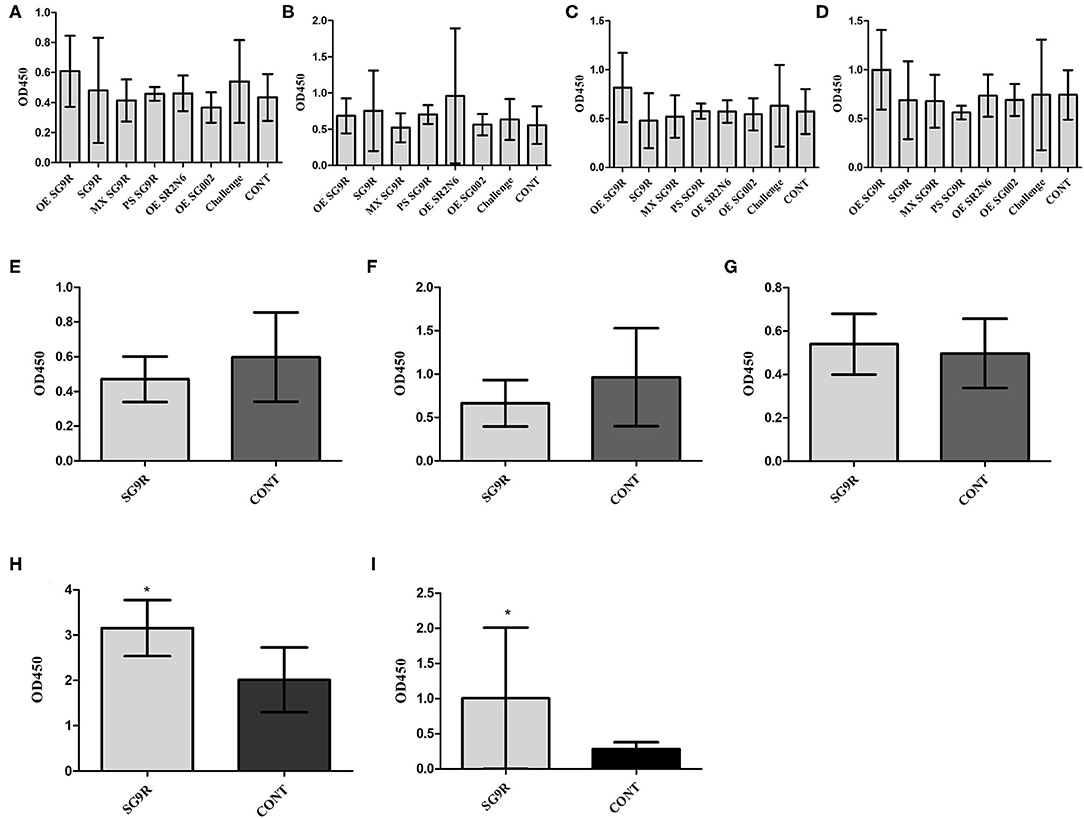
Figure 5. The mucosal immunity of bile was measured with IgA secondary antibody with the same ELISA (mean with SD): OmpA-N-L3 (A,E), OmpA-N-L4 (B,F), OmpX-L2 (C,G), OMPs (D,H), and O-Ag IgA ELISA (I). Birds were inoculated with the vaccine at 3 weeks of age, and bile juice was collected at 3 wpi (A–D). Eight-week-old brown layer chickens with or without SG 9R vaccination were challenged with SG0197 at 6 weeks of age, and bile juice samples were collected at 10 weeks of age (E–I). *Indicates a significant difference compared with CONT [P-values - (H) (0.001) and (I) (0.001)].
The inoculation of SG0197 in 8-w-o male brown layer chickens did not cause mortality in either the SG 9R vaccine or no vaccine (CONT) group at 2 wpi. The SG 9R vaccine and no vaccine groups did not display different OD values for the peptide-ELISA (Figures 5E–G). However, the SG 9R vaccine group showed significantly higher OD values than the no vaccine group using the OMP-ELISA (P < 0.05) and O-Ag-ELISA (Figures 5H,I).
Humoral Immunity Against SG in the Field
When the field serum samples were tested using peptide-ELISAs, L12W showed higher OD values than the other samples, although the differences were not significant. The OMP-ELISA revealed significantly higher OD values in the L12W, L19W, and L41W groups than in the negative control (Figure 6D).
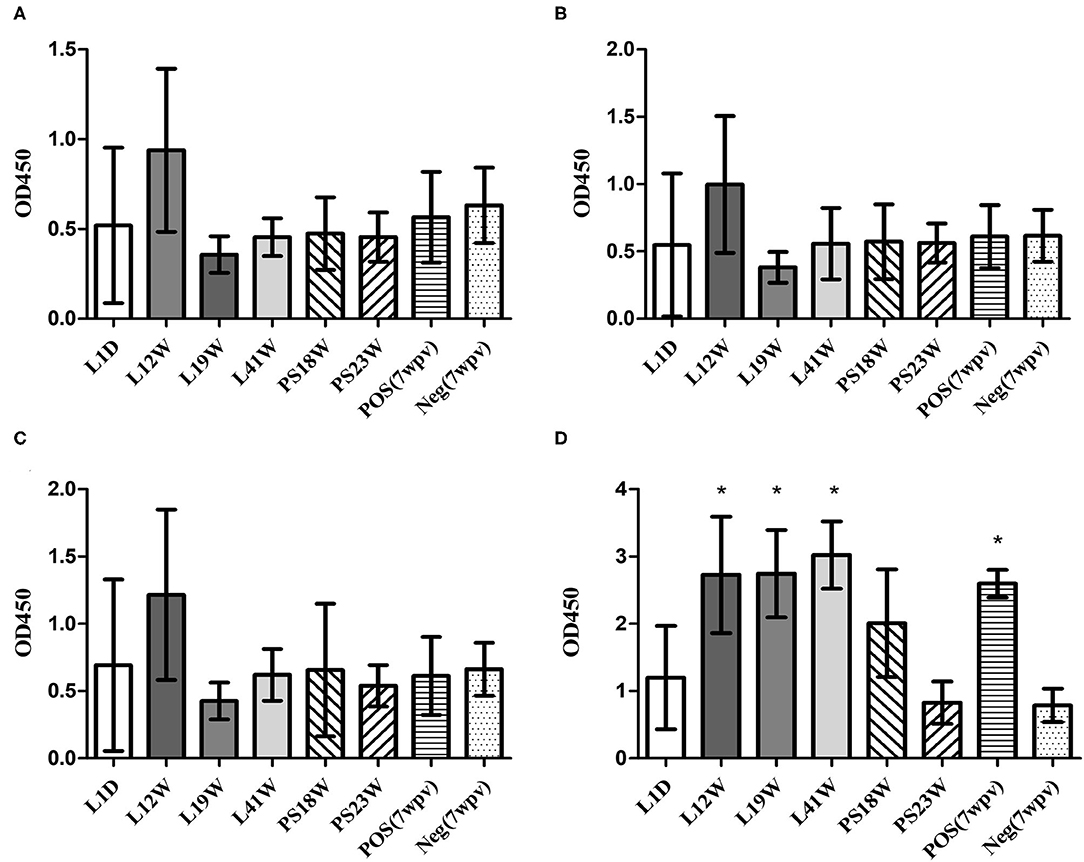
Figure 6. Humoral immunity against SG in the field (mean with SD): OmpA-N-L3 (A), OmpA-N-L4 (B), OmpX-L2 (C), and OMP-ELISA (D). The field serum samples from the L1D (1 d-o), L12W (12 w-o, vaccination at 10 weeks of age), L19W (19 w-o, vaccination at 8 and 15 weeks of age), and L41W (41 w-o, vaccination at 7 and 16 weeks of age) groups were collected from laying hens, and the PS18W (18 w-o) and PS23W (23 w-o) samples were collected from parent stocks. *Indicates a significant difference compared with Neg. [P-values - (D) L12W (0.002), L19W (0.002), L41W (0.000), and POS (0.028)].
Discussion
Fowl typhoid vaccines are categorized into live attenuated and inactivated vaccines, and live attenuated vaccine strains are subdivided into rough and smooth strains (15–17). As O-Ag of LPS hides OMPs and induces strong activation of specific B cells, the immunogenicity of OMPs of smooth strains may be less than rough strains (18). Our western blotting results from 2D-gel electrophoresis with whole bacterial lysates revealed predominant humoral immunity to O-Ag, and the results of the peptide- and OMP-ELISAs of SG002 and SR2-N6 supported the hypothesis that OMPs of the rough strain are more immunogenic than OMPs of the smooth strain (Figures 1, 2). The increased immunogenicity of OMPs may be due to unrestricted exposure to B cells without shielding by O-Ag and the absence of a competing strong immunogen monopolizing most of the resources of humoral immunity. Considering the already improved protective efficacy of OMP vaccines and antigenic conservation among Gram-negative bacteria, the potential value of rough strains to become universal vaccines needs to be demonstrated in future studies (19).
SG 9R has been used worldwide due to its better protection efficacy, but the humoral immune responses to live, killed and a mixture of live, and killed SG 9R have never been compared. The significantly lower immunogenicity of PS SG 9R than SG 9R was unexpected because a killed rough strain of Salmonella serovar Typhimurium generated a higher antibody titer than the live rough strain (18). We killed the bacteria at 65°C for 2 h, while the authors of the previous used 100°C for 30 min and subsequent treatment with 1% human serum albumin and 0.16% formaldehyde. Therefore, the additional treatment with albumin and formaldehyde may have resulted in different results. Interestingly, a synergistic effect of PS SG 9R and live SG 9R was apparent and may reflect cooperative stimulation of humoral immunity by dead and live bacteria. Therefore, this new formulation without the use of the carcinogen formaldehyde may be useful to improve the protective efficacy of conventional live vaccines against infection with virulence variants recently detected in the field (20).
The oil adjuvant significantly increased the immunogenicity of OE SG 9R compared with PS SG 9R and generated similar serum antibody titers to SG 9R. Because of its potential pathogenicity, SG 9R vaccination of chickens aged <6 w-o is not recommended (8, 21, 22). Because the highest susceptibility and likelihood of infection are observed during the prevaccination ages (1 d-o to 6 w-o or age before vaccination), clinical measures, including early inoculation with adjuvanted killed vaccines, can be considered to protect chicks from vertical and/or horizontal transmission of SG.
As invasive Salmonella enterica serovars penetrate the cell as an intracellular pathogen, researchers assumed that live bacteria might not produce sufficiently high titers of specific antibodies in the bloodstream. SG did not stimulate the initial immune response via proinflammatory cytokines or chemokines due to the absence of flagella, and the ability of SG to evade the immune system was very remarkable, even when the systemic infection had progressed (23, 24). Consistent with previous reports, infection with a field strain might delay humoral immunity by ~2 weeks compared with SG 9R inoculation (Figure 4) and might result in insignificant mucosal antibody levels compared with the negative control (Figure 5). However, we should consider their different routes of infections. The observation that surviving chickens mounted antibodies against OmpX peptides and OMPs may support the importance of humoral immunity in survival. Additionally, a significant increase in the IgA titer in the bile juice of SG 9R-vaccinated chickens may support the importance of humoral immunity induced by SG 9R vaccination (Figure 5). Because most commercial layer farms inoculate animals with SG 9R vaccines, testing IgA levels in bile juice, intestinal washes and feces may be useful for the differential diagnosis of FT and an estimation of the risk of SG exposure.
Considering temporary increases in OD values obtained from peptide-ELISAs during the first 2 weeks after SG vaccine inoculations, the higher OD value of L12W may be related to the SG 9R vaccination at 10 weeks of age (Figure 6). Thus, the peptide-ELISAs were able to detect specific antibodies induced by recent SG 9R vaccination under both experimental and field conditions. However, the OMP-ELISA revealed significantly higher antibody levels in vaccinated flocks than in unvaccinated flocks. All field samples were tested using the O-Ag ELISA, and no positive sample indicating a field strain or SE infection was examined (data not shown). Therefore, these assays may be useful to verify the efficacy of the inoculated vaccine and monitor unlawful vaccination with parent stocks in the field in combination with the O-Ag ELISA.
The immunogenic OMPs of Salmonella enterica have been reported, and OmpA and OmpX are known to be protective antigens (25–27). Although we selected OmpA and OmpX due to their immunodominance, the rapid but short-lived antibody responses induced by these antigens were unexpected and have not been reported. Additionally, our study is the first to investigate the kinetics of the production of specific antibodies against linear epitopes of OmpA and OmpX compared with OMPs. In summary, with the gradual increase in the titers of antibodies against OMPs over time, there may be other OMPs inducing long-lasting antibody responses. Although the immune dominance of the C-terminus of OmpA was reported previously, we could not find any difference between the results of C- and N-terminal peptide-ELISAs (Supplementary Figure 1) (28).
In conclusion, rough strains are better than smooth strains in terms of the immunogenicity of OMPs, and a mixture of a live and killed rough vaccine strains may potentiate the efficacy of the conventional live vaccine. The evasion of humoral immunity by the field strain was demonstrated again, but SG 9R may be useful to prime mucosal immunity against infection with a field strain. Additionally, combined serological tests with peptide, OMP, and O-Ag ELISAs may be useful for the differential diagnosis of FT in the field.
Data Availability Statement
The original contributions presented in the study are included in the article/Supplementary Materials, further inquiries can be directed to the corresponding author/s.
Ethics Statement
The animal study was reviewed and approved by Institutional Animal Care and Use Committee of BioPOA Co.
Author Contributions
N-HK, H-JK, and K-SC substantially contributed to conceptualization, data curation, and analysis of the study. H-JK supervised all surveillance components. E-JH and D-SK contributed to analysis of data. N-HK prepared the initial draft, figures, and tables. N-HK and H-JK contributed to the writing and editing of the manuscript. All authors contributed to the article and approved the submitted version.
Conflict of Interest
The authors declare that the research was conducted in the absence of any commercial or financial relationships that could be construed as a potential conflict of interest.
Supplementary Material
The Supplementary Material for this article can be found online at: https://www.frontiersin.org/articles/10.3389/fvets.2020.598610/full#supplementary-material
References
2. Shivaprasad H. Fowl typhoid and pullorum disease. Rev Sci Tech. (2000) 19:405–16. doi: 10.20506/rst.19.2.1222
3. Mastroeni P, Simmons C, Fowler R, Hormaeche C, Dougan G. Igh-6–/–(B-Cell-Deficient) mice fail to mount solid acquired resistance to oral challenge with virulent Salmonella enterica serovar typhimurium and show impaired Th1 T-cell responses tosalmonella antigens. Infect Immun. (2000) 68:46–53. doi: 10.1128/IAI.68.1.46-53.2000
4. Lee YJ, Mo IP, Kang MS. Protective efficacy of live salmonella gallinarum 9R vaccine in commercial layer flocks. Avian Pathol. (2007) 36:495–8. doi: 10.1080/03079450701691278
5. Poltorak A, He X, Smirnova I, Liu M-Y, Van Huffel C, Du X, et al. Defective LPS signaling in C3H/HeJ and C57BL/10ScCr mice: mutations in Tlr4 gene. Science. (1998) 282:2085–8. doi: 10.1126/science.282.5396.2085
6. Johnson AG, Gaines S, Landy M. Studies on the O antigen of Salmonella typhosa. V. Enhancement of antibody response to protein antigens by the purified lipopolysaccharide. J Exp Med. (1956) 103:225–46. doi: 10.1084/jem.103.2.225
7. Seppala IJ, Makela O. Adjuvant effect of bacterial LPS and/or alum precipitation in responses to polysaccharide and protein antigens. Immunology. (1984) 53:827–36.
8. Kwon H-J, Cho S-H. Pathogenicity of SG 9R, a rough vaccine strain against fowl typhoid. Vaccine. (2011) 29:1311–8. doi: 10.1016/j.vaccine.2010.11.067
9. Gil-Cruz C, Bobat S, Marshall JL, Kingsley RA, Ross EA, Henderson IR, et al. The porin OmpD from nontyphoidal Salmonella is a key target for a protective B1b cell antibody response. Proc Natl Acad Sci USA. (2009) 106:9803–8. doi: 10.1073/pnas.0812431106
10. Yang F, Gu J, Zou J, Lei L, Jing H, Zhang J, et al. PA0833 is an OmpA C-like protein that confers protection against pseudomonas aeruginosa infection. Front Microbiol. (2018) 9:1062. doi: 10.3389/fmicb.2018.01062
11. Feberwee A, De Vries T, Hartman E, De Wit J, Elbers A, De Jong W. Vaccination against Salmonella enteritidis in Dutch commercial layer flocks with a vaccine based on a live Salmonella gallinarum 9R strain: evaluation of efficacy, safety, and performance of serologic Salmonella tests. Avian Dis. (2001) 45:83–91. doi: 10.2307/1593015
12. Cho S-H, Ahn Y-J, Kim T-E, Kim S-J, Huh W, Moon Y-S, et al. Establishment of a live vaccine strain against fowl typhoid and paratyphoid. Korean J Vet Res. (2015) 55:241–6. doi: 10.14405/kjvr.2015.55.4.241
13. Baik S-C, Kim K-M, Song S-M, Kim D-S, Jun J-S, Lee S-G, et al. Proteomic analysis of the sarcosine-insoluble outer membrane fraction of Helicobacter pylori strain 26695. J Bacteriol. (2004) 186:949-55. doi: 10.1128/JB.186.4.949-955.2004
14. Park YD, Kim SY, Jang HS, Seo EY, Namkung JH, Park HS, et al. Towards a proteomic analysis of atopic dermatitis: a two-dimensional-polyacrylamide gel electrophoresis/mass spectrometric analysis of cultured patient-derived fibroblasts. Proteomics. (2004) 4:3446–55. doi: 10.1002/pmic.200400998
15. de Paiva J, Penha Filho R, Argüello Y, da Silva M, Gardin Y, Resende F, et al. Efficacy of several salmonella vaccination programs against experimental challenge with salmonella gallinarum in commercial brown layer and broiler breeder hens. Braz J Poultry Sci. (2009) 11:65–72. doi: 10.1590/S1516-635X2009000100010
16. Barrow P. Immunity to experimental fowl typhoid in chickens induced by a virulence plasmid-cured derivative of salmonella gallinarum. Infect Immun. (1990) 58:2283–8. doi: 10.1128/IAI.58.7.2283-2288.1990
17. Chaudhari AA, Jawale CV, Kim SW, Lee JH. Construction of a salmonella gallinarum ghost as a novel inactivated vaccine candidate and its protective efficacy against fowl typhoid in chickens. Vet Res. (2012) 43:44. doi: 10.1186/1297-9716-43-44
18. Muotiala A, Hovi M, Mäkelä PH. Protective immunity in mouse salmonellosis: comparison of smooth and rough live and killed vaccines. Microbial Pathogen. (1989) 6:51–60. doi: 10.1016/0882-4010(89)90007-7
19. Liu Q, Liu Q, Zhao X, Liu T, Yi J, Liang K, et al. Immunogenicity and cross-protective efficacy induced by outer membrane proteins from salmonella typhimurium mutants with truncated LPS in mice. Int J Mol Sci. (2016) 17:416. doi: 10.3390/ijms17030416
20. Kim NH, Ha EJ, Ko DS, Lee CY, Kim JH, Kwon HJ. Molecular evolution of Salmonella enterica subsp. Enterica serovar gallinarum biovar gallinarum in the field. Vet Microbiol. (2019) 235:63–70. doi: 10.1016/j.vetmic.2019.05.019
21. Van Immerseel F, Studholme DJ, Eeckhaut V, Heyndrickx M, Dewulf J, Dewaele I, et al. salmonella gallinarum field isolates from laying hens are related to the vaccine strain SG9R. Vaccine. (2013) 31:4940–5. doi: 10.1016/j.vaccine.2013.08.033
22. Wigley P, Hulme S, Powers C, Beal R, Smith A, Barrow P. Oral infection with the Salmonella enterica serovar gallinarum 9R attenuated live vaccine as a model to characterise immunity to fowl typhoid in the chicken. BMC Vet Res. (2005) 1:2. doi: 10.1186/1746-6148-1-2
23. de Freitas Neto OC, Setta A, Imre A, Bukovinski A, Elazomi A, Kaiser P, et al. A flagellated motile salmonella gallinarum mutant (SG Fla+) elicits a pro-inflammatory response from avian epithelial cells and macrophages and is less virulent to chickens. Vet Microbiol. (2013) 165:425–33. doi: 10.1016/j.vetmic.2013.04.015
24. Seo K-H, Holt PS, Brackett RE, Gast RK, Stone HD. Mucosal humoral immunity to experimental salmonella enteritidis infection in the chicken crop. Avian Dis. (2002) 46:1015–20. doi: 10.1637/0005-2086.2002.046[1015:MHITES]2.0.CO;2
25. Pore D, Mahata N, Pal A, Chakrabarti MK. Outer membrane protein A (OmpA) of shigella flexneri 2a, induces protective immune response in a mouse model. PLoS ONE. (2011) 6:e22663. doi: 10.1371/journal.pone.0022663
26. Erova TE, Rosenzweig JA, Sha J, Suarez G, Sierra JC, Kirtley ML, et al. Evaluation of protective potential of yersinia pestis outer membrane protein antigens as possible candidates for a new-generation recombinant plague vaccine. Clin Vaccine Immunol. (2013) 20:227–38. doi: 10.1128/CVI.00597-12
27. Song J-r, Fu Y-w, Li P, Du T, Du X-j, Wang S. Protective effect of recombinant proteins of Cronobacter sakazakii during pregnancy on the offspring. Front Cell Infect Microbiol. (2020) 10. doi: 10.3389/fcimb.2020.00015
Keywords: Salmonella serovar Gallinarum biovar Gallinarum, humoral immunity, vaccines, natural infection, Peptide-ELISA
Citation: Kim N-H, Ha E-J, Ko D-S, Choi K-S and Kwon H-J (2020) Comparison of Humoral Immune Responses to Different Forms of Salmonella enterica Serovar Gallinarum Biovar Gallinarum. Front. Vet. Sci. 7:598610. doi: 10.3389/fvets.2020.598610
Received: 25 August 2020; Accepted: 19 October 2020;
Published: 06 November 2020.
Edited by:
Constantinos S. Kyriakis, Auburn University, United StatesReviewed by:
Antonio Camarda, University of Bari Aldo Moro, ItalyAneesh Thakur, University of Copenhagen, Denmark
Copyright © 2020 Kim, Ha, Ko, Choi and Kwon. This is an open-access article distributed under the terms of the Creative Commons Attribution License (CC BY). The use, distribution or reproduction in other forums is permitted, provided the original author(s) and the copyright owner(s) are credited and that the original publication in this journal is cited, in accordance with accepted academic practice. No use, distribution or reproduction is permitted which does not comply with these terms.
*Correspondence: Hyuk-Joon Kwon, a3dvbmhqMDEmI3gwMDA0MDtzbnUuYWMua3I=