- 1Department of Animal Science, Iowa State University, Ames, IA, United States
- 2Department of Veterinary Pathology, Iowa State University, Ames, IA, United States
- 3Department of Veterinary Diagnostic and Production Animal Medicine, Iowa State University, Ames, IA, United States
Swine dysentery (SD) induced by Brachyspira hyodysenteriae manifests as mucohemorrhagic diarrhea in pigs, but little is known about the changes that occur to the gastrointestinal tract during this disease. It is thought that dietary fibers alter disease pathogenesis, although the mechanisms of action are unclear. Thus, the objectives of this study were to characterize intestinal integrity, metabolism, and function in pigs during SD and determine if replacing insoluble fiber with fermentable fibers mitigates disease. Thirty-six B. hyodysenteriae-negative gilts [24.3 ± 3.6 kg body weight (BW)] were assigned to one of three treatment groups: (1) B. hyodysenteriae negative, control diet (NC); (2) B. hyodysenteriae challenged, control diet (PC); and (3) B. hyodysenteriae challenged, highly fermentable fiber diet (RS). The NC and PC pigs were fed the same control diet, containing 20% corn distillers dried grains with solubles (DDGS). The RS pigs were fed a diet formulated with 5% sugar beet pulp and 5% resistant potato starch. On days post inoculation (dpi) 0 and 1, pigs were inoculated with B. hyodysenteriae or sham. Pigs were euthanized for sample collection after onset of SD. The challenge had high morbidity, with 100% of PC and 75% of RS pigs developing clinical SD. The timing of onset of clinical SD differed due to treatment, with RS pigs having a delayed onset (dpi 9) of clinical SD compared with dpi 7 for PC pigs. Colon transepithelial resistance was increased and macromolecule permeability was reduced in PC pigs compared with NC pigs (P < 0.01). Minimal changes in ileal permeability, mitochondrial function, or volatile fatty acids (VFAs) were observed. Total VFA concentrations were lower in the colon and cecum in both PC and RS pigs compared to NC pigs (both P < 0.05), but iso-acids were higher (both P < 0.05). Total tract digestibility of dry matter (DM), organic matter (OM), nitrogen (N), and gross energy (GE) was lower in PC pigs compared with both NC and RS pigs (both P < 0.001). These data indicate that SD reduces digestive function but does not reduce ex vivo intestinal integrity. Further, replacement of insoluble fiber with highly fermentable fibers mitigated and delayed the onset of SD.
Introduction
Swine dysentery (SD) is an economically significant disease of growing pigs characterized by mucohemorragic diarrhea, or watery feces containing large amounts of mucus and flecks of blood (1). Pigs clinically affected with SD become anorexic and dehydrated, resulting in rapid weight loss (2). The causative agents of SD include strongly hemolytic Brachyspira spp., such as Brachyspira hyodysenteriae and Brachyspira hampsonii (2). Upon association with the colonic epithelium, B. hyodysenteriae causes marked inflammation, hemorrhage, superficial epithelial necrosis, and excessive production of mucus (3, 4). Further, the mucus layer becomes thickened and disorganized, potentially providing B. hyodysenteriae, a favorable niche for binding and proliferation (4).
Diarrhea during B. hyodysenteriae infection is driven by complete abolition of water absorption (5) and reduces apparent total tract digestibility of nutrients and energy, which contributes to attenuated growth performance (6). Additionally, inflammation at the colonic epithelium may alter intestinal metabolism to favor glycolysis, exacerbating dysbiosis (7). Few studies have evaluated intestinal barrier permeability, digestive function, and intestinal metabolism in B. hyodysenteriae-challenged pigs. Further, as B. hyodysenteriae is a large intestinal pathogen, investigations have been primarily limited to the cecum and colon (5, 8, 9). However, as the small intestine is the primary site of nutrient digestion and absorption (10), understanding if B. hyodysenteriae infection alters small intestinal integrity and function is highly relevant and applicable to developing mitigation strategies.
Although there is discord in the literature, dietary fiber manipulation is known to affect the clinical presentation of SD upon experimental infection (11, 12). Most of these studies examining dietary influences on SD development have utilized base ingredients such as white rice, wheat, barley, and triticale, which are more prominently used in European and Australasian pig production regions. In the US, recent research has shown that high dietary inclusion of corn distillers dried grains with solubles (DDGS), an ingredient high in insoluble fiber, increased SD disease severity in growing pigs (13). It is speculated that by limiting fermentation in the hindgut, SD severity decreases (11, 14), but certain highly fermentable fiber types may increase or decrease disease depending on fiber type and the base diet utilized (11, 12, 15). Inclusion or exclusion of certain dietary fibers may alter disease pathogenesis by changing the physiochemical environment of the large intestine or by modulating microbial communities and microbial fermentation products to either increase or decrease the ability of B. hyodysenteriae to associate with the intestinal epithelium and cause disease. However, these mechanisms of action have not been fully explored.
Thus, the objective of the study herein was to characterize changes to small and large intestinal integrity, metabolism, and function in grower pigs experiencing SD after B. hyodysenteriae infection. Further, we aimed to determine if replacement of dietary insoluble fiber with highly fermentable fibers would mitigate the development and severity of SD.
Materials and Methods
All animal procedures were approved by the Iowa State University Institutional Animal Care and Use Committee (IACUC protocol #19-170) and adhered to the guidelines for ethical and humane use of animals for research.
Animals, Diets, and Experimental Design
A total of 36 gilts from a herd with no history of SD [24.3 ± 3.6 kg body weight (BW); Camborough (1050) X 337 Hendersonville, TN] were randomly selected for this experiment. Pigs were individually confirmed negative for B. hyodysenteriae via selective culture of fecal swabs, allocated to individual pens, and assigned treatment groups. Pigs were allotted across three treatment groups: (1) B. hyodysenteriae negative, fed the control diet (NC, n = 12); (2) B. hyodysenteriae challenged, fed the control diet (PC, n = 12); and (3) B. hyodysenteriae challenged, fed a highly fermentable fiber diet (RS, n = 12). Pigs were housed across two rooms in the same barn, in which NC pigs were housed in a separate room to prevent pathogen spread. The two rooms had identical pen sizes, feeders, flooring, heating, cooling, and water supply, but separate manure pits.
The NC and PC pigs were fed the same corn-soybean meal control diet, formulated with 20% corn DDGS. The RS pigs were fed a corn-soybean meal diet formulated with 5% sugar beet pulp and 5% resistant potato starch added at the expense of DDGS. Diets were ad libitum fed throughout the experiment and met or exceeded all National Research Council (16) requirements and contained no antibiotics (Table 1). Both diets were formulated to be isocaloric (3,300 kcal/kg metabolizable energy) and isonitrogenous (18% crude protein) and to have the same level of standardized ileal digestible lysine (0.98%). Pigs were started on dietary treatments 3 weeks prior to initiation of the B. hyodysenteriae challenge to allow for microbial adaptation.
On days post inoculation (dpi) 0 and 1, pigs were inoculated with B. hyodysenteriae or sham. The B. hyodysenteriae (B204) strain used was originally recovered from a clinical case of SD in 1972 and was obtained from the culture collection at the Iowa State University Veterinary Diagnostic Laboratory (ISU VDL). Inoculum was prepped according to Burrough et al. (17). Briefly, the isolate was thawed, plated onto several tryptic soy agar plates, and anaerobically incubated. Inoculum was prepared by removing the agar, placing it into a bag, and homogenizing the agar by hand until a slurry of uniform consistency was achieved. A small sample of each day's slurry was retained for determination of inoculum concentration by standard plate counting. The B. hyodysenteriae-challenged pigs received two doses of inoculum (60 ml/dose, containing approximately 3.4 × 106 and 3.9 × 106 colony-forming units/ml) administered as an agar slurry via gastric gavage as previously described (17). The sham-inoculated pigs received two 60-ml doses of agar slurry via gastric gavage. Inoculations were performed 24 h apart with each administration preceded by a 12–18-h fast. Throughout the study period, fecal consistency was evaluated each morning by the same individual who was blinded to dietary treatment. Fecal consistency was scored based on the following system: 0 if normal, 1 if soft but formed, 2 if semisolid, and 3 if liquid to watery. An additional 0.5 point was added each for the presence of discernible mucus and/or blood for a total maximum score of 4. Pigs were considered to have clinical SD upon scoring a 4 (liquid to watery diarrhea with presence of blood and mucus). At dpi −14, 0, 4, 8, and 12 and at necropsy, individual pig BW and individual pig feed disappearance were recorded to calculate performance parameters of average daily gain (ADG), average daily feed intake (ADFI), and feed efficiency [gain:feed (G:F)].
Pigs were euthanized within 72 h of the initial observation of clinical SD or at the end of the study on dpi 16 (between dpi 10 and 16). Pigs were euthanized in repetitions of 6–8 pigs per repetition, with at least two NC pigs included in each repetition. Pigs were euthanized by captive bolt followed by exsanguination, and tissues were collected for analysis. Sections from the ileum, collected 30 cm orad from the ileal-cecal junction, and the apex of the spiral colon were placed in continuously aerated bottles containing Krebs buffer (25 mM NaHCO3, 120 mM NaCl, 1 mM MgSO4, 6.3 mM KCl, 2 mM CaCl2, and 0.32 mM NaH2PO4) and for transport to the laboratory for analysis. Adjacent sections of the ileum and spiral colon were rinsed in Krebs buffer, frozen in liquid nitrogen, and then stored at −80°C until analysis. An additional section of the spiral colon apex was aseptically collected and placed on ice until selective anaerobic culture for B. hyodysenteriae using routine methodology at the ISU VDL.
Contents from the ileum, cecum, and spiral colon were collected into 50-ml conical tubes. A portable pH probe (Thermo Fisher Scientific, Waltham, MA) was utilized to measure pH of the contents, after which contents were frozen and stored at −80°C until further analysis.
Ex vivo Barrier Function and Integrity
Freshly isolated ileum and colon sections were mounted on modified Using chambers (Physiologic Instruments Inc., San Diego, CA) within ~1 to 1.5 h of euthanasia. Upon receipt to the laboratory, the serosal layer of intestinal tissues was removed, lumens were opened, and the mucosa was visually assessed for intact and undisturbed adhered mucus and epithelial layers. Explants were selected from tissue regions that remained undisturbed. Modified Using chambers were assembled, and electrophysiological and fluorescein isothiocyanate-dextran 4 kDa (FD4) macromolecule permeability measurements were collected as previously described (18). Estimates of nutrient transport for ileal samples were calculated as the change in current (μA) after glucose and glutamine addition. A fluorescent plate reader (Cytation 5 Hybrid Multi-Mode Reader, BioTek Instruments Inc., Winooski, VT) was used to determine mucosal to serosal flux changes in relative fluorescence of FD4 in the serosal samples from 0 to 60 min after FD4 addition at 485 and 520 nm excitation and emission wavelengths, respectively. Apparent permeability coefficients for FD4 flux were calculated as described by Pearce et al. (19).
Mitochondrial Isolation, Reactive Oxygen Species Production, and Oxygen Consumption
Efficient energy metabolism is critical to feed efficiency and health of growing pigs. As mitochondria provide the intestine with the vast majority of ATP needed for proper functioning, disruptions to their activity are important with regard to energy generation. To understand intestinal mitochondrial function during B. hyodysenteriae challenge, live mitochondria were isolated from freshly excised ileum and colon tissue via differential centrifugation. Tissues were collected, placed in aerated bottles containing Krebs buffer, and transported on ice to the laboratory within 1 to 1.5 h of euthanasia. The serosal layer was removed, then approximately 10 g of tissue was weighed out, diced, and gently homogenized in 35 ml of mitochondrial isolation buffer [220 mM mannitol, 70 mM sucrose, 2 mM 4-(2-hydroxyethyl)-1-piperazineethanesulfonic acid (HEPES), 1 mM ethylene glycol-bis(β-aminoethyl ether)-N,N,N′,N′-tetraacetic acid (EGTA), and 0.5 mg/ml fatty acid-free bovine serum albumin (BSA), pH 7.4]. Homogenates were centrifuged (twice at 600 × g for 10 min at 4°C), the supernatant was strained through cheesecloth after each centrifugation, and the pellet containing cellular debris was discarded. Mitochondria were then pelleted by centrifugation 7,750 × g for 20 min at 4°C. The supernatant was decanted, and pellets were washed three times with 10 ml mitochondrial wash buffer (220 mM mannitol, 70 mM sucrose, 2 mM HEPES, and 0.5 mg/ml fatty acid-free BSA, pH 7.4). Washed mitochondria were resuspended in 3 ml mitochondrial wash buffer, and mitochondrial protein concentrations were determined via bicinchoninic acid (BCA) assay (Thermo Fisher Scientific, Waltham, MA). Mitochondria were diluted to a protein concentration of 2 mg/ml with mitochondrial wash buffer and stored at 4°C until use.
Mitochondrial reactive oxygen species (ROS) production was determined in isolated mitochondria using a 2′,7′-dichlorofluorescin diacetate (DCFH) assay described previously (20, 21). Fluorescence of DCFH was detected at an excitation/emission wavelength of 480/530 nm using a Cytation Hybrid Multi-Mode Reader using Gen 5 software (BioTek Instruments Inc., Winooski, VT). Mitochondrial hydrogen peroxide production was calculated from a hydrogen peroxide standard curve based on changes in relative fluorescence of DCFH. Samples were plated in triplicate using a black 96-well plate. Twenty units of superoxide dismutase (Sigma-Aldrich, St. Louis, MO) were added to each sample well to convert any superoxide produced into hydrogen peroxide. Either hydrogen peroxide standards or 90 μg of mitochondrial protein was added to each well after which 45 μl of an assay buffer (145 mM KCl, 30 mM HEPES, 5 mM KH2PO4, 3 mM MgCl2, 0.1 mM EGTA, 51 μM DCFH, 8 μM glutamate) was added. Plates were incubated at 37°C and read at 0, 5, 10, 15, and 20 min after adding the energy substrate. Readings were used to calculate the rate of hydrogen peroxide production per minute, expressed as mM hydrogen peroxide produced/min/mg mitochondrial protein.
Mitochondrial oxygen consumption was evaluated using a Seahorse XFe24 Extracellular Flux Analyzer (Seahorse Bioscience, North Billerica, MA) as previously described (22, 23). All reagents were made fresh daily from either new or frozen chemical stocks. Optimum concentrations of reagents to be injected were determined via preliminary titration trials. Mitochondria (40 μg) were plated in duplicate into a V7 XFe24 Tissue Culture Plate, and then diluted to 50 μl with mitochondrial assay buffer (220 mM mannitol, 70 mM sucrose, 5 mM KH2PO4, 5 mM MgCl2, 2 mM HEPES, 1 mM EGTA, 0.5 mg/ml BSA, pH 7.4). Blank wells contained 50 μl mitochondrial assay buffer. The plate was centrifuged at 2,000 × g for 10 min at 4°C to adhere mitochondria to the bottom of the plate. After centrifugation, 450 μl mitochondrial assay buffer + 5.5 mM glutamate + 5.5 mM malate (pH 7.4) were gently added to each well to provide mitochondria with a substrate. The plate was incubated in a non-CO2 incubator at 37°C for 8–10 min and then transferred to the XFe24 instrument for initiation of the experiment.
The XFe24 analyzer examines mitochondrial respiration under several states. First, mitochondria were measured in a coupled state with only substrate present (basal respiration). This was followed by the addition of 2 mM ADP (State 3; phosphorylating respiration), 2 μM oligomycin (State 4o; non-phosphorylating respiration, proton leak), 4 μM carbonyl cyanide 4-(trifluoromethoxy) phenylhydrazone (FCCP; State 3u; maximal uncoupler-stimulated respiration), and 4 μM antimycin A (loading control, blocks mitochondrial oxygen consumption). Efficient mitochondria should function by maintaining a low basal respiratory state and low proton leak, while being able to respond in the presence of ADP or FCCP to respire and consume oxygen quickly (22). A schematic of the assay is described in Figure 1A. From these measurements, respiratory control ratios (RCRs; State 3u/State 4o) were calculated, of which healthy mitochondria should have a high respiratory control (22). Mitochondrial respiration data were expressed as pmole O2 consumed per min.
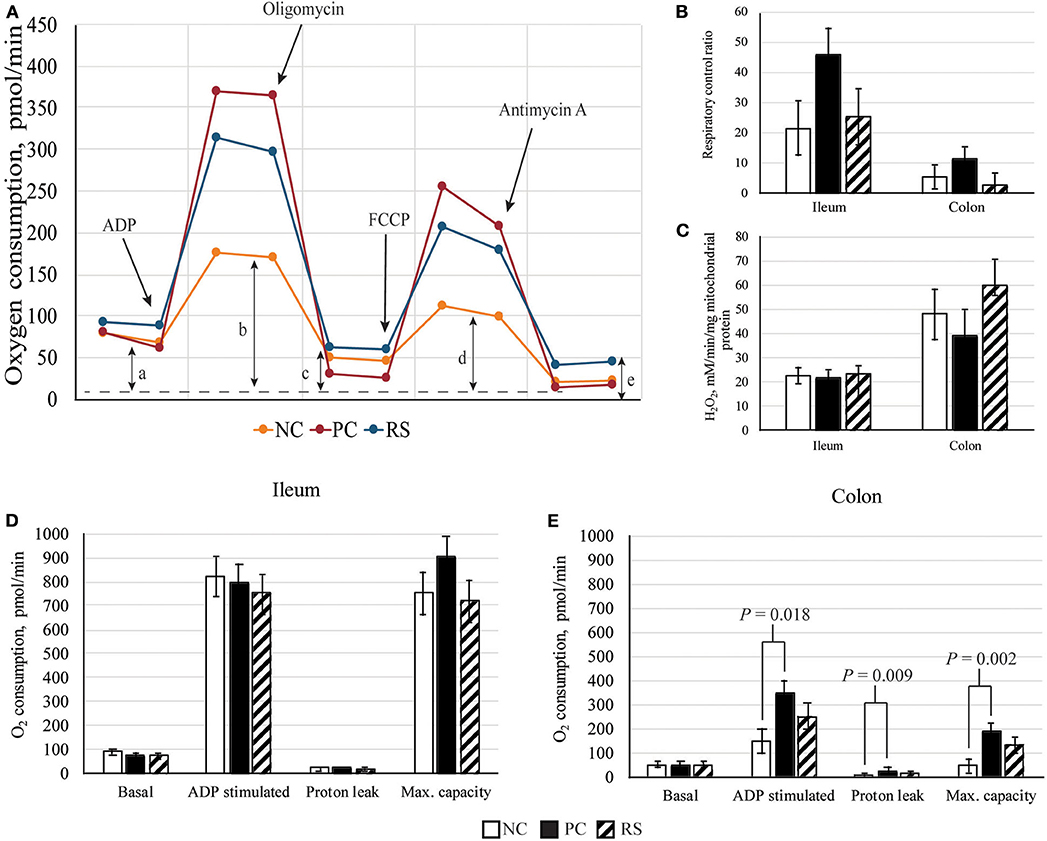
Figure 1. Mitochondrial parameters of sham-inoculated pigs (NC), Brachyspira hyodysenteriae-inoculated pigs (PC), and Brachyspira hyodysenteriae-inoculated pigs fed a diet containing 5% resistant starch and 5% sugar beet pulp (RS). (A) Schematic representation of graph obtained from Seahorse XFe24 Extracellular Flux analysis. Non-mitochondrial respiration (e) was subtracted from all values: (a) state 4, basal respiration; (b) state 3, ADP induced respiration; (c) state 4o, proton leak; and (d) state 3μ, FCCP induced maximal respiratory capacity (max. capacity). (B) Respiratory control ratios (State 3μ/4o) of ileal and colonic mitochondria. (C) Reactive oxygen species (ROS) production by isolated mitochondria. (D,E) Respiration of (D) ileal and (E) colonic mitochondria. Error bars represent lsmeans ± SEM, and P-values represent contrasts between either NC and PC or PC and RS pigs.
Dietary Analysis and Apparent Total Tract Digestibility
A representative feed sample from each complete diet was obtained for analysis. Fecal samples were collected for 3 consecutive days prior to each pig's individual necropsy date (dpi 10–16). Thus, fecal samples were collected to match similar stages of disease progression for all pigs. All freshly collected fecal samples were stored at −20°C until proximate analysis was conducted. For proximate analysis, fecal samples were thawed and homogenized within pig and then dried in a mechanical convection oven at 100°C.
Feed samples were ground through a 1-mm screen (Model ZM1; Retsch Inc., Newton, PA), and fecal samples were ground with a mortar and pestle prior to analysis. Proximate analysis was performed on feed and feces as previously described to determine apparent total tract digestibility (24). Dietary acid detergent fiber, neutral detergent fiber, insoluble fiber, and total dietary fiber analyses were conducted by the University of Missouri Experimental Station Chemical Laboratories (Columbia, MO). Dietary resistant starch and total starch concentrations were determined using the Megazyme Resistant Starch Assay Kit according to the manufacturer's instructions (Megazyme Ltd., Bray, Ireland).
All dietary and fecal samples were analyzed for dry matter (DM; AOAC method 930.15), titanium dioxide as described by Leone (25), nitrogen (N) using TruMac N (Leco Corporation, St. Joseph, MO), and gross energy (GE) using bomb calorimetry (Oxygen Bomb Calorimetry 6200; Parr Instruments, Moline, IL). Organic matter (OM) was determined using the ashing method and calculated as previously described (26). For each of the three treatments, apparent total tract digestibility (ATTD) coefficients for DM, OM, N, and GE were calculated using the index method (27).
Ileal, Cecal, and Colonic Volatile Fatty Acid Concentrations
Ileal, cecal, and colonic volatile fatty acid (VFA) concentrations were determined as previously described (28). Briefly, contents were thawed for 1 h in a water bath at 55°C and then mixed thoroughly. Approximately 1.0 g of cecal and colonic digesta or 2.0 g of ileal digesta from each pig were weighed into a 15-ml polypropylene centrifuge tube. Cecal and colonic digesta were then diluted with 5 ml water and mixed overnight on a digital rocker. All samples were then centrifuged at 20,000 × g for 20 min at 4°C. For ileal samples, approximately 1 ml of supernatant was removed and placed in a new 15-ml polypropylene tube, to which 100 μl o-phosphoric acid was added to achieve a pH of 2.0–2.5. Ileal samples were then centrifuged at 4,000 × g for 10 min at 4°C. Exactly 1 ml of this supernatant was transferred to a 20-ml gas chromatography vial containing 0.3 g of NaCl. For cecal and colonic samples, after centrifugation at 20,000 × g, exactly 1 ml supernatant was removed and transferred to a 20-ml gas chromatography vial containing 0.3 g NaCl, to which 100 μl o-phosphoric acid was added to achieve a pH of 2.0–2.5. Samples were then analyzed via gas chromatography (Agilent 7890A Gas Chromatograph, Agilent Technologies Inc., Wilmington, DE). Samples were analyzed in duplicate, and values were multiplied to adjust for dilution. Concentrations are expressed as mM VFA per gram wet digesta. Furthermore, molar proportions (%) of the top 3 VFAs (acetate, propionate, butyrate) were calculated as the individual VFA/total VFA concentration × 100.
Statistical Analysis
Statistical analysis of all data was performed in SAS 9.4 (SAS Institute, Cary, NC). The following mixed model was fitted to quantitative parameters:
wherein Yij = the phenotype measured on animal j; Trti = effect of treatment (fixed effect; NC, PC, RS); and eij = error term of animal j subjected to treatment i, eij ~ N(0, ). Least square means were determined using the LS means statement, and data are presented as least squares means with a pooled standard error. Contrast statements were used to determine the effect of either B. hyodysenteriae (NC vs. PC) or dietary treatment (PC vs. RS). Fisher's exact tests in the FREQ procedure were used to assess if treatment contributed to the number of pigs positive for B. hyodysenteriae via colon swab culture at necropsy or the number of pigs that had clinical SD at any point during the study. Log rank tests in the LIFETEST procedure were used to determine if diet contributed to the number of days it took for PC and RS pigs to develop clinical SD. For all analyses, differences were considered significant when P < 0.05 and a tendency when 0.05 ≤ P ≤ 0.10.
Results
Confirmation of Disease and Growth Performance
Colon swabs collected from all pigs at necropsy and selectively cultured for B. hyodysenteriae confirmed that sham-inoculated pigs were culture negative and that the B. hyodysenteriae-inoculated PC and RS pigs were all culture positive at the time of necropsy (Table 2). Soft to semisolid feces began to appear at dpi 6, and clinical SD (watery feces containing blood and mucus) was first observed at dpi 7 and 9 for PC and RS pigs, respectively. The timing of onset of clinical SD differed due to dietary treatment, with RS pigs tending to have a delayed onset (dpi 9) of clinical SD compared with dpi 7 for PC pigs (P = 0.083; Table 2). Overall, 100% of PC pigs and 75% of RS pigs developed clinical SD. The three RS pigs that had not developed SD at the end of the study (dpi 16) had soft to semisolid feces at necropsy, suggesting the possibility either of a mild form of disease or that clinical SD might manifest in several days' time.

Table 2. Confirmation of infection and clinical disease incidence of sham-inoculated pigs (NC), Brachyspira hyodysenteriae-inoculated pigs (PC), and Brachyspira hyodysenteriae-inoculated pigs fed a diet containing 5% resistant starch and 5% sugar beet pulp in replacement of 10% DDGS (RS).
Pig performance was recorded in the 14 days prior to challenge. In this pre-challenge period, average daily gains differed, as RS pigs had greater ADG than PC pigs (P < 0.004; Table 3). Performance differences between NC and PC pigs were not observed in the pre-challenge period. Average daily feed intake during this time did not differ, but RS pigs had greater G:F than PC pigs (P = 0.023). However, despite differences in growth during the pre-challenge period, BW at dpi 0 did not differ between treatments (P ≥ 0.360; Table 3).
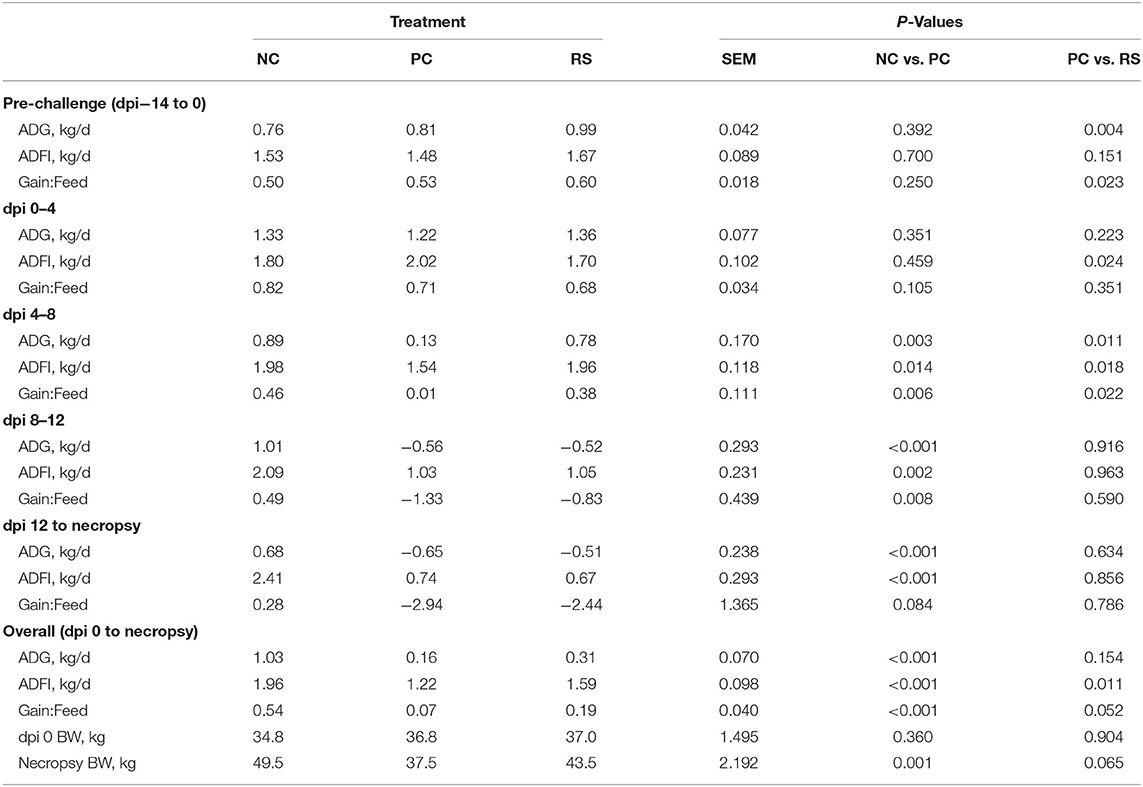
Table 3. Average daily gain (ADG), average daily feed intake (ADFI), and feed efficiency (Gain:Feed) of sham-inoculated pigs (NC), Brachyspira hyodysenteriae-inoculated pigs (PC), and Brachyspira hyodysenteriae-inoculated pigs fed a diet containing 5% resistant starch and 5% sugar beet pulp in replacement of 10% DDGS (RS).
After B. hyodysenteriae inoculation, growth, feed intake, and feed efficiency were all negatively impacted in B. hyodysenteriae-inoculated pigs (Table 3). In the first 4 dpi, ADG and G:F did not differ, but ADFI was reduced in RS pigs compared with PC pigs (P = 0.024). From dpi 4 to 8, ADG was reduced in PC pigs compared with NC and RS pigs 85 and 83%, respectively (P < 0.05 for both comparisons). Feed intake and G:F were also reduced in PC pigs compared with NC pigs in this period (P < 0.05 for both analyses). From dpi 8 to 12, ADG, ADFI, and G:F were all reduced in PC pigs when compared with NC pigs (P ≤ 0.008 for all analyses), while PC and RS pigs did not differ from each other. Similarly, from dpi 12 until the end of the study, ADG and ADFI were reduced in PC pigs compared with NC pigs (P < 0.001 for both analyses) and did not differ between PC and RS pigs. However, G:F only tended to be reduced in PC pigs compared with NC pigs (P = 0.084).
Overall, ADG in the post-challenge period was reduced in both PC and RS pigs compared with NC pigs (P < 0.001; Table 3), and ADG did not differ between PC and RS pigs. Average daily feed intake was reduced 38% in PC pigs compared with NC pigs (P < 0.001) and was reduced 23% in PC pigs when compared with RS pigs (P = 0.011). Overall, G:F was lower in PC pigs compared with NC pigs (P < 0.001) and tended to be lower in PC pigs compared with RS pigs (P = 0.052).
Ex vivo Intestinal Integrity and Nutrient Transport
Ex vivo markers of intestinal integrity and active nutrient transport at necropsy are presented in Table 4. In the ileum, intestinal integrity markers of transepithelial resistance and FD4 macromolecule permeability did not differ (P > 0.10). Active transport of glucose tended to be greater in PC pigs compared with NC pigs (P = 0.060), while active transport of glutamine did not differ (P > 0.10). In the colon, transepithelial resistance was increased in PC pigs compared with NC pigs (P = 0.001) and was increased in PC pigs compared with RS pigs (P = 0.045). Further, colon FD4 permeability was reduced in PC pigs compared with NC pigs (P = 0.025) and did not differ between PC and RS pigs.
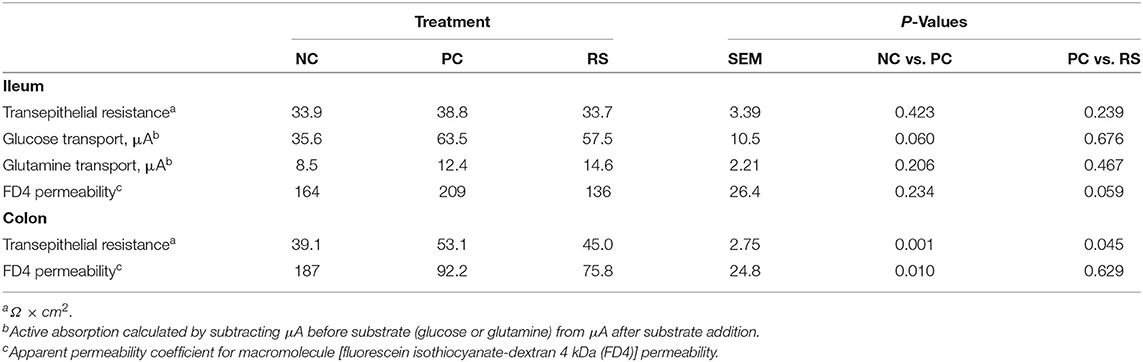
Table 4. Ex vivo intestinal integrity and function parameters of sham-inoculated pigs (NC), Brachyspira hyodysenteriae-inoculated pigs (PC), and Brachyspira hyodysenteriae-inoculated pigs fed a diet containing 5% resistant starch and 5% sugar beet pulp (RS).
Mitochondrial Function
Mitochondrial respiration measurements and mitochondrial ROS production are presented in Figure 1. In the ileum, basal respiration, ADP-stimulated respiration, oligomycin-induced proton leak, and FCCP-induced maximal capacity were not different among treatments (P > 0.10; Figure 1D); however, respiratory control ratios tended to be increased in PC pigs compared with RS pigs (P = 0.086; Figure 1B). Ileal mitochondrial ROS production did not differ (P > 0.10; Figure 1C).
In the colon, basal respiration did not differ. However, ADP-stimulated respiration (P = 0.018), proton leak (P = 0.009), and FCCP-induced maximal capacity (P = 0.002) were all elevated in PC pigs compared with NC pigs (Figure 1E). For these measurements, PC and RS pigs did not differ from one another. Mitochondrial respiratory control ratios and mitochondrial ROS production did not differ in the colon (P < 0.10 for all contrasts).
Digesta pH and Volatile Fatty Acid Concentrations
In the ileum, there were no differences in digesta pH (P ≥ 0.223; Table 5). Furthermore, there were no differences in total VFA concentrations in the ileum (P ≥ 0.579; Table 5) or in individual concentrations of any of the VFAs, except for butyrate and isocaproate. Butyrate concentrations were greater in NC pigs compared with PC pigs (P = 0.045) and did not differ between PC and RS pigs. Isocaproate concentrations were greater in NC pigs compared with PC pigs (P = 0.033) and did not differ between PC and RS pigs. Molar proportions (%) of acetate and propionate did not differ in the ileum, but the molar proportion of butyrate was greater in PC pigs compared with NC pigs (P = 0.032) and did not differ between PC and RS pigs.
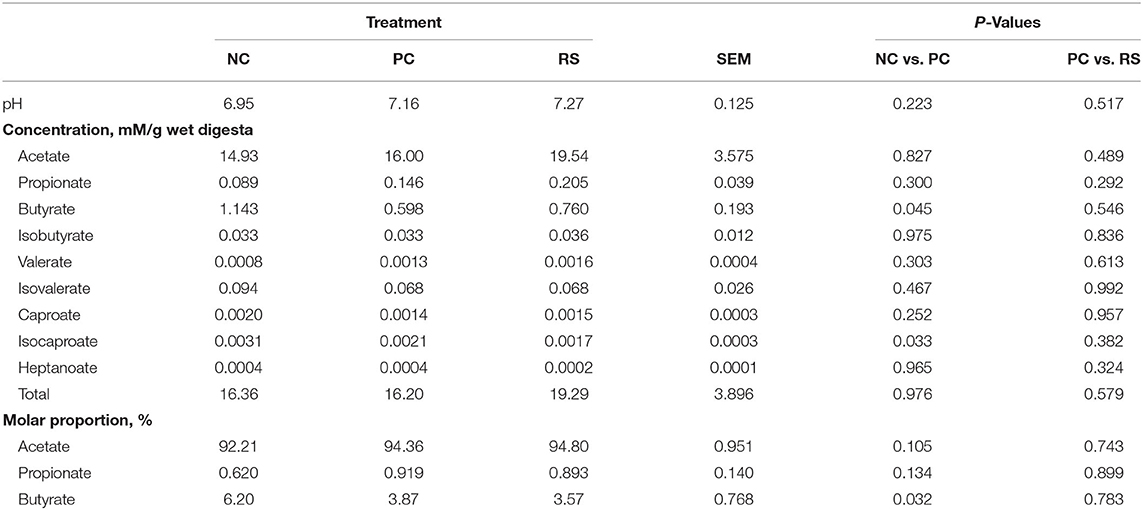
Table 5. Ileum digesta pH, volatile fatty acid (VFA) concentrations, and molar proportions of sham-inoculated pigs (NC), Brachyspira hyodysenteriae-inoculated pigs (PC), and Brachyspira hyodysenteriae-inoculated pigs fed a diet containing 5% resistant starch and 5% sugar beet pulp (RS).
In the cecum, pH values were higher in PC pigs compared with NC pigs (P < 0.001; Table 6) and did not differ between PC and RS pigs. Total and individual VFA concentrations were also different among treatments. Concentrations of acetate, propionate, butyrate, and total VFA concentrations were all lesser in PC pigs compared with NC pigs (P < 0.05 for all analyses). Conversely, concentrations of isobutyrate, valerate, isovalerate, and heptanoate were greater in both PC pigs compared with NC pigs (P < 0.05 for all analyses). Concentrations of isobutyrate and isovalerate tended to be greater in PC pigs compared with RS pigs (P = 0.067 and P = 0.057, respectively). Concentrations of caproate and isocaproate tended to be greater in PC pigs compared with NC pigs (P = 0.055 for both), and concentrations of caproate were greater in PC pigs compared with RS pigs (P = 0.031). Molar proportions of VFAs in the cecum did not differ among treatments.
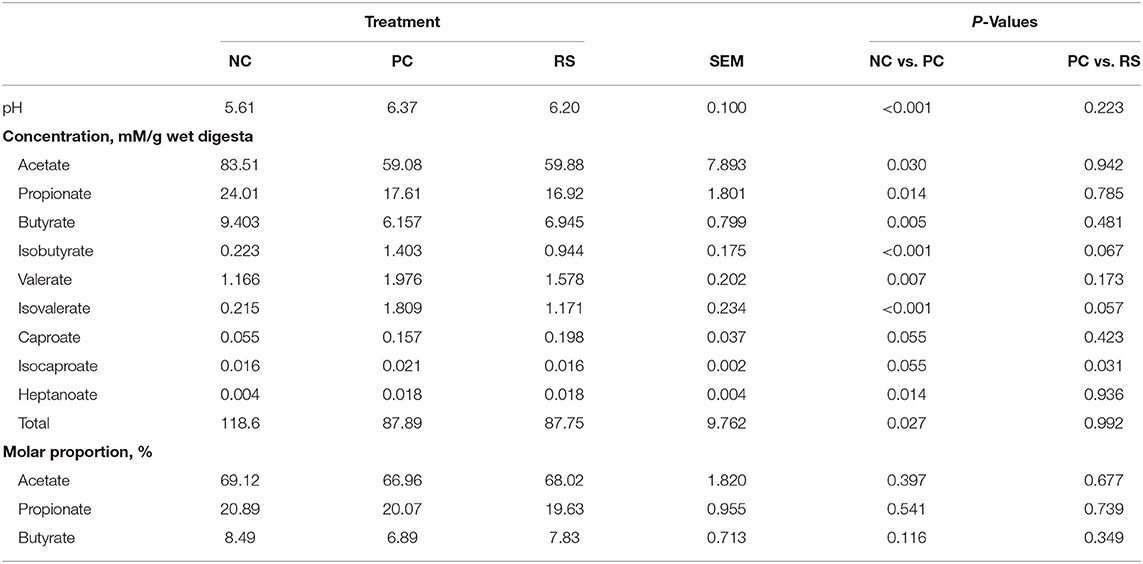
Table 6. Cecum digesta pH, volatile fatty acid (VFA) concentrations, and molar proportions of sham-inoculated pigs (NC), Brachyspira hyodysenteriae-inoculated pigs (PC), and Brachyspira hyodysenteriae-inoculated pigs fed a diet containing 5% resistant starch and 5% sugar beet pulp (RS).
In the colon, pH values were higher in PC pigs compared with NC pigs (P = 0.007; Table 7) and did not differ between PC and RS pigs. Concentrations of acetate tended to be greater in the colon digesta of NC pigs compared with PC pigs (P = 0.053; Table 7). Concentrations of isobutyrate, isovalerate, and isocaproate were increased in PC pigs compared with NC pigs (P < 0.050 for all analyses). Concentrations of caproate were lower in PC pigs compared with NC pigs (P = 0.043). Total VFA concentrations were reduced in PC pigs compared with NC pigs (P = 0.019). Molar proportions of acetate and propionate did not differ among treatments; however, molar proportions of butyrate were reduced in PC pigs compared with NC pigs (P = 0.011).
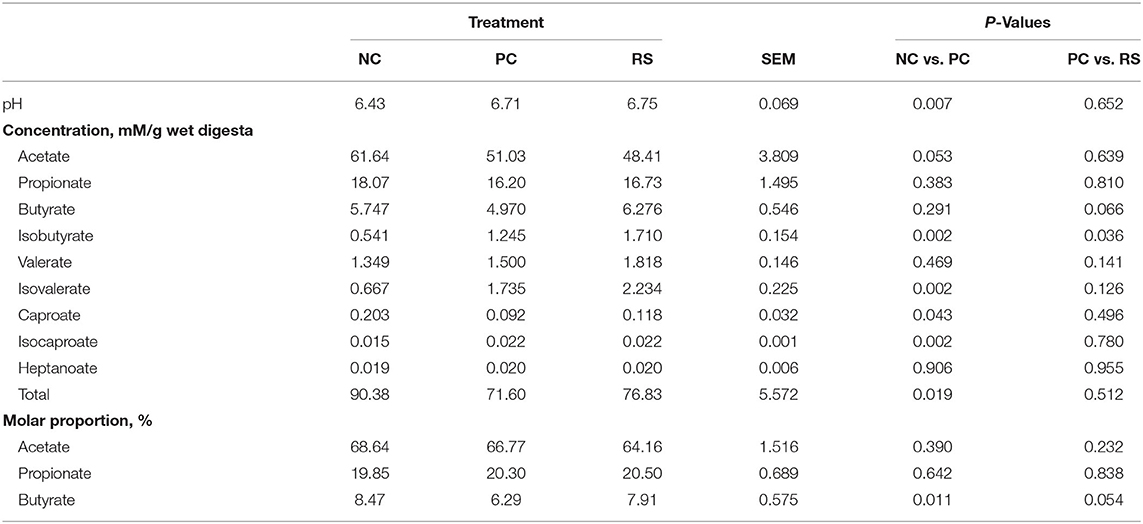
Table 7. Colon digesta pH, volatile fatty acid (VFA) concentrations, and molar proportions of sham-inoculated pigs (NC), Brachyspira hyodysenteriae-inoculated pigs (PC), and Brachyspira hyodysenteriae-inoculated pigs fed a diet containing 5% resistant starch and 5% sugar beet pulp (RS).
Apparent Total Tract Digestibility
Reductions in DM, N, OM, and GE ATTD coefficients were observed in response to B. hyodysenteriae inoculation. Reductions of 20, 72, 22, and 24% were observed in DM, N, OM, and GE digestibility, respectively, in PC pigs compared with NC pigs (P < 0.001 for all analyses; Figure 2). Interestingly, ATTD coefficients of DM, N, OM, and GE were greater in RS pigs compared with PC pigs (P < 0.001 for all analyses).
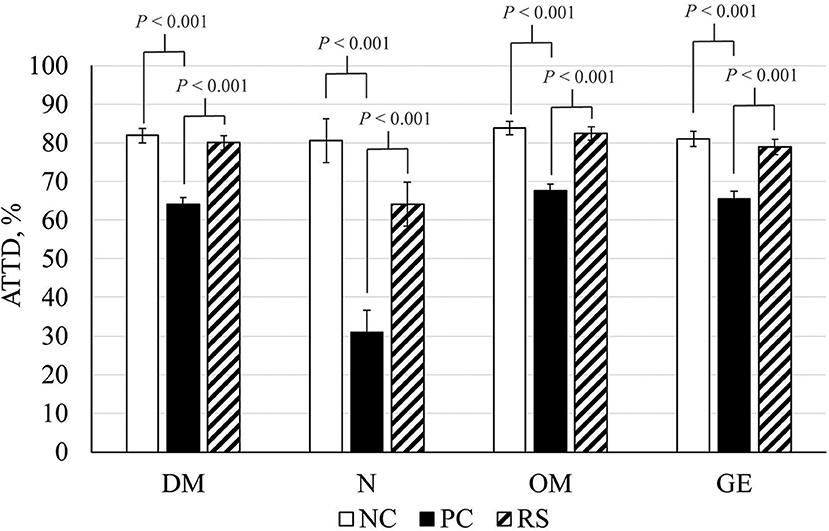
Figure 2. Apparent total tract digestibility (ATTD) coefficients of dry matter (DM), nitrogen (N), organic matter (OM), and gross energy (GE) of sham-inoculated pigs (NC), Brachyspira hyodysenteriae-inoculated pigs (PC), and Brachyspira hyodysenteriae-inoculated pigs fed a diet containing 5% resistant starch and 5% sugar beet pulp (RS). Error bars represent lsmeans ± SEM, and P-values represent contrasts between either NC and PC or PC and RS pigs.
Discussion
In the post-nursery period, pigs clinically affected with SD become anorexic and dehydrated, antagonizing growth and health (2). SD also compromises the health of the intestinal epithelium, inducing mucosal necrosis, neutrophil infiltration, and hemorrhage (29). Intestinal epithelial health comprises an array of physiological and functional elements, including barrier permeability, nutrient digestion and absorption, a stable microbiome, host metabolism and energy generation, mucus layer development, and mucosal immune responses (30). Disruptions to these elements during stress events such as during SD result in intestinal dysbiosis, reducing animal well-being, growth, and feed efficiency. Our group has previously demonstrated that there are differences in how gastrointestinal physiology is affected under various bacterial (31) and viral (32–34) pathogen challenges, highlighting the importance of characterizing the differential changes to physiology that may occur during different disease states. However, the extent to which B. hyodysenteriae and its associated disease directly modulates intestinal function and integrity in grower pigs, and how these relate to reduced performance, has been poorly defined. Further, dietary fiber is known to alter SD development and severity; however, the physiological mechanisms underlying these phenomena are unclear. Thus, the objectives of this study were to (1) evaluate intestinal barrier permeability, digestive function, and metabolism during a B. hyodysenteriae challenge and (2) determine if replacement of dietary insoluble fiber with highly fermentable fiber sources would mitigate SD by improving parameters of intestinal health.
Growth performance of pigs during the development and onset of SD is variable and has been poorly characterized due to factors such as pig age, study duration, disease severity, and researchers failing to include naive controls or failing to report growth performance entirely. In general, B. hyodysenteriae challenge has been associated with anywhere from a 25 to 75% reduction in ADG compared with healthy control pigs (13, 35, 36), reductions which may or may not be mitigated by dietary factors. Wilberts et al. (13) found that pigs inoculated with B. hyodysenteriae had reductions in growth regardless of whether they were fed a control diet or fed an SD-exacerbating diet containing high levels of lowly fermentable insoluble fiber. Other researchers have reported reductions in ADG to be mitigated with supplementation of conjugated linoleic acid (35). Further, several researchers have documented that pigs fed highly fermentable inulin grow faster than those fed either a control diet or a diet high in lowly fermentable fibers following B. hyodysenteriae challenge (12, 36). Herein, we reported reductions in growth to be significantly reduced as early as 8 dpi and continuing until the end of the study. Highly fermentable fiber delayed the reductions observed in ADG; however, these pigs still had dramatic reductions in growth. In fact, by dpi 12, pigs in both B. hyodysenteriae-challenged groups were losing BW. Much of this is likely attributed to a loss of water due to dehydration, as 87% of B. hyodysenteriae-challenged pigs developed watery, bloody, and mucoid feces prior to the end of the study. In general, diarrheic pigs lose body water and non-aqueous tissue mass in an 80/20 ratio, thus ~20% of this dramatic decline in BWs can be attributed to losses in tissue mass (37). Although the pigs herein were not given time to recover, it is likely that these dramatic losses in BW during peak infection would not be fully recompensated by the pig and would increase time to market BW. Further, growth performance and feed efficiency reductions support alterations to homeostasis and a basis to evaluate disruptions to intestinal health.
One key aspect of intestinal health and function is barrier permeability, primarily regulated by the tight junction complexes forming a seal between epithelial cells (38). Increased barrier permeability is documented during enteric challenges such as porcine epidemic diarrhea virus (33) and increases the pig's susceptibility to further challenge by luminal toxins or bacteria. To the authors' knowledge, this is the first published report examining ileum permeability in B. hyodysenteriae-challenged pigs. Although B. hyodysenteriae is a large intestinal pathogen and does not directly impact the small intestinal epithelium, reductions in feed intake alone can increase barrier permeability and active nutrient transport (18, 39). Further, Kaleczyc et al. (40) found increases in ileal tissue concentrations of certain neuropeptides and changes to percentages of some lymphocyte populations in ileal Peyer's patches of pigs with clinical SD, highlighting neuro-immune cross-talk between intestinal segments during SD. However, we observed very few changes in ileal ex vivo integrity, active nutrient transport, or mitochondrial metabolism. Additionally, limited changes to ileal VFA concentrations and proportions were observed. Limited alterations to ileal physiology are supported by observations by Argenzio (41), who demonstrated that pigs in the early stages of SD do not have any changes to small intestinal absorption of glucose or fluid. Additionally, Schweer et al. (6) observed no reduction in apparent ileal digestibility coefficients of DM, N, total amino acids, or GE despite reductions in apparent total tract digestibility of these same parameters, suggesting ileal digestive function is unhindered. This suggests that despite severe colonic disease, ileal function and integrity remain largely unchanged during SD.
B. hyodysenteriae induces neutrophilic inflammation in the colon (42), which may lead to internalization of tight junction proteins and increased barrier permeability (43), rendering the pig susceptible to further insult from other pathogens or toxins. However, previous researchers have found no reduction in colon transepithelial resistance (8) and no difference in macromolecule (mannitol or polyethylene glycol-400) permeability (5) in pigs with SD. In agreement with these reports, we did not see any reduction in colon integrity; in fact, transepithelial resistance was increased and FD4 permeability decreased in pigs challenged with B. hyodysenteriae. Although a necrotic section of tissue was not specifically selected for these permeability measures, macroscopic lesions and inflammation were widely present around the apex of the spiral colon where explants were removed, thus the explant chosen was representative of a lesioned area. Regardless, the reductions in ex vivo permeability observed can likely be attributed to a thickened mucus layer, as mucus hypersecretion is a classic response to B. hyodysenteriae infection (4). Mucin production increases 5-fold and the mucus layer is visibly thicker in experimentally infected pigs compared with healthy controls (4). A thicker mucus layer would cause both an elevation in electrical resistance and a reduction in permeability to macromolecules (44), as what we observed. However, during SD, the mucus layer loses its parallel striations and becomes disorganized, so it may be unstable and less beneficial to the pig in preventing bacteria from reaching the epithelium (4). Additionally, these permeability measures, when skewed by mucus thickness, do not indicate if tight junction complex integrity itself is affected. Further work would be needed to determine if this is the case.
In addition to forming a selectively permeable seal against the luminal environment, the intestinal epithelium is also responsible for digestion and absorption of water and dietary nutrients. Reductions in digestive function reduce nutrients available for growth and lean accretion. To evaluate digestive function during SD, ATTD of nitrogen and energy was evaluated. Herein, PC pigs had reductions in DM, N, OM, and GE ATTD coefficients of 20, 72, 22, and 24%, respectively, compared with NC pigs. The incredibly low nitrogen ATTD observed in PC pigs is likely attributed to excessive mucus secretion, which would increase endogenous losses of N, thus decreasing digestibility. In support of this, increased hindgut N appearance is observed in B. hyodysenteriae-infected pigs (6). Reductions in all ATTD coefficients are consistent with previous reports in B. hyodysenteriae-infected pigs by Schweer et al. (6), although far more dramatic. However, fecal samples from Schweer et al. (6) were collected at the same dpi (9–11) for all pigs, whereas fecal samples from the current study were collected immediately prior to each pig's individual necropsy date, thus ensuring nearly all pigs were clinically afflicted at the time of collection. Further, differences in clinical severity may also explain the greater reductions in the current experiment. Diarrhea reduces passage time through the gut, leading to poor breakdown of feedstuffs and limiting absorption (45, 46). As such, greater severity of diarrhea would lead to greater reductions in ATTD coefficients and would exacerbate the attenuated growth already occurring due to reduced feed intake. Interestingly, ATTD coefficients were greater in RS pigs compared with PC pigs. This suggests that the highly fermentable diet was able to prevent B. hyodysenteriae-induced digestive dysfunction, which could mitigate performance losses in a less severe challenge.
Maintaining a stable microbiota is critical to ensure pig well-being. Not only do commensal microbes prevent against pathogenic bacterial colonization, their metabolic end products can be used by the pig for energy generation. VFAs, the endpoints of microbial fermentation, are often used as a marker of microbial metabolism (47). The most widely produced VFAs include acetate, propionate, and butyrate. Of these, butyrate has been studied the most extensively with regards to its impact on colon metabolism (48). Health status is known to alter microbial populations, and thus their metabolic end products. Nursery pigs challenged with an F18 E. coli had reduced total VFA concentrations when compared with healthy controls (49). Similarly, in the current experiment total concentrations of VFAs were reduced in both the cecum and colon of pigs challenged with B. hyodysenteriae, accompanied by an increase in luminal pH. An increase in pH and reduction in VFA concentrations is not surprising, considering pigs had watery, malabsorptive diarrhea, and thus diluted digestive contents. Further, reduced feed intake would lower available substrates, reducing microbial fermentation and thus products of their metabolism. While concentrations of many VFAs were reduced in the cecum and colon, an increase in concentrations of the iso-acids, or branched-chain fatty acids, was observed in B. hyodysenteriae-challenged pigs. Branched-chain fatty acids are produced exclusively via branched-chain amino acid metabolism (50), indicating increased protein fermentation in B. hyodysenteriae-challenged pigs. This could be due to fermentation of mucus, which is of abundance in clinically affected pigs, or increased dietary protein reaching the large intestine due to reduced transit time in the ileum. In addition to branched-chain fatty acids, other products of protein fermentation include ammonia, hydrogen sulfide, p-cresol, and phenols, which may have toxic effects on the colonic epithelium (51).
The intestine must be able to maintain barrier integrity and perform nutrient absorption with minimal energy usage, so efficient metabolism is critical for intestinal health. Colonocytes preferentially use butyrate as fuel, sparing glucose for the rest of the body (52). In the healthy colon, butyrate is metabolized via mitochondrial β-oxidation and energy generated through oxidative phosphorylation, maintaining epithelial hypoxia (7). Toxic by-products, such as those produced through protein fermentation (including ammonia, hydrogen sulfide, p-cresol, and phenols), or stress events may alter these processes (53). Particularly, hydrogen sulfide is known to inhibit cytochrome c oxidase, the terminal oxidase of the mitochondrial electron transport chain, which catalyzes reduction of dioxygen to water and harnesses the free energy of that reaction to catalyze phosphorylation of ADP to ATP (53, 54). Human colonic HT-29 GLC–/+ cells treated with hydrogen sulfide had reduced maximal respiratory capacity and increased oxygen consumption in the presence of oligomycin, suggesting mitochondrial inefficiency and increased proton leak (55). However, in the current study, PC pigs had increased mitochondrial maximal respiratory capacity, suggesting that energy generation may be more efficient in PC pigs, potentially as an adaptive mechanism or due to infiltrating immune cells, which are highly metabolic. However, PC pigs also had increased oxygen consumption in the presence of F0/F1 ATPase inhibitor oligomycin, indicating enhanced proton leak (55). Although this may be a by-product of overall greater mitochondrial metabolic rates, excessive proton leak leads to the formation of ROS, which can damage the epithelium. Piglets challenged with lipopolysaccharide (LPS) had increased jejunal mitochondrial ROS production, which was associated with increased mitophagy, adding to intestinal dysbiosis and acting as a source of inefficiency (56). In the current experiment, mitochondrial ROS production was not different among treatments, suggesting the evidence of enhanced proton leak was not leading to oxidative stress and acting as a detriment to intestinal metabolism. Taken together, this B. hyodysenteriae challenge appeared to impact intestinal health via reducing digestibility of nutrients, reducing microbial metabolism and inducing mucus hypersecretion, which led to a shift toward protein fermentation in the large intestine. Thus, our next objective was to determine if dietary manipulation could mitigate SD via improving these parameters of intestinal health.
Dietary manipulation has been explored extensively as a method to prevent SD. However, there is much discord in the literature with regard to which diet components are beneficial or detrimental when pigs are at risk for B. hyodysenteriae infection. Addition of 30% corn DDGS to diets based on corn and soybean meal leads to pigs shedding bacteria and developing SD sooner than those without DDGS, suggesting that increasing insoluble fibers may be detrimental with regard to developing SD (13). Additionally, several studies have found that feeding pigs highly digestible diets based on cooked rice (14, 57) or cornstarch (6) reduces the incidence of SD upon experimental challenge. Cooked white rice diets were also associated with increased digesta pH and reduced VFA concentrations, suggesting that limiting microbial fermentation in the large intestine hinders B. hyodysenteriae colonization (14, 57). Adding a highly fermentable fiber to a cooked white rice diet resulted in greater disease incidence, further implicating microbial fermentation as a risk factor for developing SD (11). However, attempts to reproduce these results with cooked white rice by using parboiled rice did not reduce the incidence of SD (58, 59). The reason behind these differential reports may lie in rice processing, as cooked rice gelatinizes and is rapidly digested by the body, while parboiled rice retrogrades and acts as a resistant starch, resistant to small intestinal digestion and rapidly fermented by microbes in the large intestine (60).
In contrast to work by Pluske et al. (57) and Siba et al. (14), several researchers have found that dietary addition of fermentable carbohydrates to pig diets mitigates SD. Specifically, Thomsen et al. (36) found that a diet containing highly fermentable dried chicory roots (fructans) and lupins (galactans) completely protected pigs from SD upon experimental challenge. A series of experiments by Hansen et al. (12, 15) further examined the protective effects of fermentable carbohydrates in pigs fed triticale- and barley-based diets. This work demonstrated that diets containing the fermentable fiber source inulin, but not lupins, were able to prevent the development of SD. However, diets containing both inulin and lupins were able to delay disease onset and increase ADG during the study period (12). Furthermore, a high concentration of inulin (greater than 40–80 g/kg diet) was necessary to provide this protective effect (15). Interestingly, increased dietary inulin was associated with increased VFAs, suggesting an increase in microbial fermentation (15). However, this may be confounded with differences in disease severity, as pigs with lesser disease incidence would have greater digesta dry matter and thus greater VFA concentrations by default, as watery intestinal contents would dilute VFA concentrations (15).
Although differences in study design, base diet, pig age, adaptation period, etc., make it difficult to compare between these studies, some connections can be made. In general, in the case of diets based on highly digestible carbohydrates, increasing fibers, even those that are highly fermentable, increase disease (11, 57). Conversely, when diets are formulated from ingredients that are less highly digestible (barley, triticale), addition of fermentable fiber is beneficial (12, 15). In the case of US swine producers, feeding high levels of highly digestible ingredients such as cooked white rice is not common, as most diets are based on ground corn and soybean meal. Thus, the addition of highly fermentable fibers may prove beneficial. Indeed, in the current study, addition of fermentable fibers in the form of resistant starch and sugar beet pulp, while not protective, delayed the onset of clinical SD and reductions in ADG, as ADG was greater in RS pigs than in PC pigs from dpi 4 to 8. Pigs fed resistant starch also had greater ATTD coefficients than PC pigs, suggesting disease mitigation. However, the current study does not make it possible to evaluate how pigs on the RS diet may perform in the absence of B. hyodysenteriae challenge, thus disease mitigation and diet effects cannot be fully differentiated. Further, as nearly all pigs in the current study developed SD and were euthanized at peak infection, disease mitigation is difficult to determine.
Taken together, these data indicate that SD induced by B. hyodysenteriae does not appear to reduce ex vivo small intestinal integrity and in fact appears to increase ex vivo colon integrity likely due to the high production of epithelial surface mucus by the colon. Further, reductions in ATTD and changes to digesta pH and digesta VFA concentrations all support the pathogenesis of malabsorptive diarrhea and increased mucus production. Finally, replacement of insoluble fiber with highly fermentable fibers delayed the onset of and appeared to mitigate SD; however, a longitudinal study or study with lesser disease severity is needed to confirm these findings.
Data Availability Statement
The raw data supporting the conclusions of this article will be made available by the authors, without undue reservation.
Ethics Statement
The animal study was reviewed and approved by Iowa State University Institutional Animal Care and Use Committee.
Author Contributions
All authors contributed to the design of the study, interpretation of the results, and assisted with manuscript editing. Performed live animal experiments: EH and SL. Performed laboratory experiments: EH. Wrote the manuscript: EH.
Funding
Funding for this research was provided by the USDA National Institute of Food and Agriculture, Agriculture and Food Research Initiative Competitive Grant #2019-67015-29486 and the National Needs Fellowship program (support of EH's graduate studies). The funders were not involved in study design, data collection, analysis, and interpretation of data, writing the manuscript, or decision to submit the article for publication.
Conflict of Interest
The authors declare that the research was conducted in the absence of any commercial or financial relationships that could be construed as a potential conflict of interest.
Acknowledgments
The authors would like to thank the ISU VDL for preparation of inoculum and B. hyodysenteriae diagnostic culture and Shari Steadham for her technical assistance with volatile fatty acid analysis.
References
2. Hampson DJ, Burrough ER. Swine Dysentery and Brachyspiral Colitis. In: Zimmerman JJ, Karriker L, Ramirez A, Schwartz KJ, Stevenson GW, Zhang J, editors. Diseases of Swine. 11th ed. Hoboken, NJ: Wiley and Blackwell (2019). p. 951–70.
3. Albassam MA, Olander HJ, Thacker HL, Turek JJ. Ultrastructural characterization of colonic lesions in pigs inoculated with Treponema hyodysenteriae. Can J Comp Med. (1985) 49:384–90.
4. Quintana-Hayashi MP, Mahu M, De Pauw N, Boyen F, Pasmans F, Martel A, et al. The levels of Brachyspira hyodysenteriae binding to porcine colonic mucins differ between individuals, and binding is increased to mucins from infected pigs with de novo MUC5AC synthesis. Infect Immun. (2015) 83:1610–9. doi: 10.1128/IAI.03073-14
5. Argenzio RA, Whipp SC, Glock RD. Pathophysiology of swine dysentery: colonic transport and permeability studies. J Infect Dis. (1980) 142:676–84. doi: 10.1093/infdis/142.5.676
6. Schweer WP, Burrough ER, Patience JF, Kerr BJ, Gabler NK. Impact of Brachyspira hyodysenteriae on intestinal amino acid digestibility and endogenous amino acid losses in pigs. J Anim Sci. (2019) 97:257–68. doi: 10.1093/jas/sky393
7. Litvak Y, Byndloss MX, Baumler AJ. Colonocyte metabolism shapes the gut microbiota. Science. (2018) 362:eaat9076. doi: 10.1126/science.aat9076
8. Enns CB, Harding JCS, Loewen ME. Decreased electrogenic anionic secretory response in the porcine colon following in vivo challenge with Brachyspira spp. supports an altered mucin environment. Am J Physiol Gastrointest Liver Physiol. (2019) 316:G495–508. doi: 10.1152/ajpgi.00348.2018
9. Enns CB, Keith BA, Challa N, Harding JCS, Loewen ME. Impairment of electroneutral Na+ transport and associated downregulation of NHE3 contributes to the development of diarrhea following in vivo challenge with Brachyspira spp. Am J Physiol Gastrointest Liver Physiol. (2019) 318:G288–97. doi: 10.1152/ajpgi.00011.2019
10. Kiela PR, Ghishan FK. Physiology of intestinal absorption and secretion. Best Pract Res Clin Gastroenterol. (2016) 30:145–59. doi: 10.1016/j.bpg.2016.02.007
11. Pluske JR, Durmic Z, Pethick DW, Mullan BP, Hampson DJ. Confirmation of the role of rapidly fermentable carbohydrates in the expression of swine dysentery in pigs after experimental infection. J Nutr. (1998) 128:1737–44. doi: 10.1093/jn/128.10.1737
12. Hansen CF, Phillips ND, La T, Hernandez A, Mansfield J, Kim JC, et al. Diets containing inulin but not lupins help to prevent swine dysentery in experimentally challenged pigs. J Anim Sci. (2010) 88:3327–36. doi: 10.2527/jas.2009-2719
13. Wilberts BL, Arruda PH, Kinyon JM, Frana TS, Wang C, Magstadt DR, et al. Investigation of the impact of increased dietary insoluble fiber through the feeding of distillers dried grains with solubles (DDGS) on the incidence and severity of Brachyspira-associated colitis in pigs. PLoS ONE. (2014) 9:e114741. doi: 10.1371/journal.pone.0114741
14. Siba PM, Pethick DW, Hampson DJ. Pigs experimentally infected with Serpulina hyodysenteriae can be protected from developing swine dysentery by feeding them a highly digestible diet. Epidemiol Infect. (1996) 116:207–16. doi: 10.1017/s0950268800052456
15. Hansen CF, Hernandez A, Mansfield J, Hidalgo A, La T, Phillips ND, et al. A high dietary concentration of inulin is necessary to reduce the incidence of swine dysentery in pigs experimentally challenged with Brachyspira hyodysenteriae. Br J Nutr. (2011) 106:1506–13. doi: 10.1017/S000711451100208X
16. National Research Council. Nutrient Requirements of Swine: Eleventh Revised Edition. Washington, DC: The National Academies Press (2012).
17. Burrough ER, Strait EL, Kinyon JM, Bower LP, Madson DM, Wilberts BL, et al. Comparative virulence of clinical Brachyspira spp. isolates in inoculated pigs. J Vet Diagn Invest. (2012) 24:1025–34. doi: 10.1177/1040638712457927
18. Helm ET, Curry S, Trachsel JM, Schroyen M, Gabler NK. Evaluating nursery pig responses to in-feed sub-therapeutic antibiotics. PLoS ONE. (2019) 14:e0216070. doi: 10.1371/journal.pone.0216070
19. Pearce SC, Mani V, Boddicker RL, Johnson JS, Weber TE, Ross JW, et al. Heat stress reduces intestinal barrier integrity and favors intestinal glucose transport in growing pigs. PLoS ONE. (2013) 8:e70215. doi: 10.1371/journal.pone.0070215
20. Iqbal M, Pumford NR, Tang ZX, Lassiter K, Wing T, Cooper M, et al. Low feed efficient broilers within a single genetic line exhibit higher oxidative stress and protein expression in breast muscle with lower mitochondrial complex activity. Poult Sci. (2004) 83:474–84. doi: 10.1093/ps/83.3.474
21. Grubbs JK, Fritchen AN, Huff-Lonergan E, Dekkers JC, Gabler NK, Lonergan SM. Divergent genetic selection for residual feed intake impacts mitochondria reactive oxygen species production in pigs. J Anim Sci. (2013) 91:2133–40. doi: 10.2527/jas.2012-5894
22. Rogers GW, Brand MD, Petrosyan S, Ashok D, Elorza AA, Ferrick DA, et al. High throughput microplate respiratory measurements using minimal quantities of isolated mitochondria. PLoS ONE. (2011) 6:e21746. doi: 10.1371/journal.pone.0021746
23. Luso A, Repp B, Biagosch C, Terrile C, Prokisch H. Assessing mitochondrial bioenergetics in isolated mitochondria from various mouse tissues using seahorse XF96 analyzer. Methods Mol Biol. (2017) 1567:217–30. doi: 10.1007/978-1-4939-6824-4_13
24. Schweer WP, Schwartz K, Burrough ER, Yoon KJ, Sparks JC, Gabler NK. The effect of porcine reproductive and respiratory syndrome virus and porcine epidemic diarrhea virus challenge on growing pigs I: growth performance and digestibility. J Anim Sci. (2016) 94:514–22. doi: 10.2527/jas.2015-9834
25. Leone JL. Collaborative study of the quantitative determination of titanium dioxide in cheese. J Assoc. (1973) 56:535–7.
26. Faithfull NT. Methods in Agricultural Chemical Analysis: A Practical Handbook. Wallingford, UK: Cabi (2002).
27. Oresanya TF, Beaulieu AD, Patience JF. Investigations of energy metabolism in weanling barrows: the interaction of dietary energy concentration and daily feed (energy) intake. J Anim Sci. (2008) 86:348–63. doi: 10.2527/jas.2007-0009
28. Weber TE, Trabue SL, Ziemer CJ, Kerr BJ. Evaluation of elevated dietary corn fiber from corn germ meal in growing female pigs. J Anim Sci. (2010) 88:192–201. doi: 10.2527/jas.2009-1896
29. Wilcock BP, Olander HJ. Studies on the pathogenesis of swine dysentery. I Characterization of the lesions in colons and colonic segments inoculated with pure cultures or colonic content containing Treponema hyodysenteriae. Vet Pathol. (1979) 16:450–65. doi: 10.1177/030098587901600409
30. Kogut MH, Arsenault RJ. Gut health: The new paradigm in food animal production. Front Vet Sci. (2016) 3:71. doi: 10.3389/fvets.2016.00071
31. Helm ET, Curry SM, Schwartz KJ, Lonergan SM, Gabler NK. Mycoplasma hyopneumoniae-Lawsonia intracellularis dual challenge modulates intestinal integrity and function1. J Anim Sci. (2019) 97:2376–84. doi: 10.1093/jas/skz112
32. Schweer WP, Pearce SC, Burrough ER, Schwartz K, Yoon KJ, Sparks JC, et al. The effect of porcine reproductive and respiratory syndrome virus and porcine epidemic diarrhea virus challenge on growing pigs II: intestinal integrity and function. J Anim Sci. (2016) 94:523–32. doi: 10.2527/jas.2015-9836
33. Curry SM, Schwartz KJ, Yoon KJ, Gabler NK, Burrough ER. Effects of porcine epidemic diarrhea virus infection on nursery pig intestinal function and barrier integrity. Vet Microbiol. (2017) 211:58–66. doi: 10.1016/j.vetmic.2017.09.021
34. Helm ET, Curry SM, De Mille CM, Schweer WP, Burrough ER, Gabler NK. Impact of viral disease hypophagia on pig jejunal function and integrity. PLoS ONE. (2020) 15:e0227265. doi: 10.1371/journal.pone.0227265
35. Hontecillas R, Wannemeulher MJ, Zimmerman DR, Hutto DL, Wilson JH, Ahn DU, et al. Nutritional regulation of porcine bacterial-induced colitis by conjugated linoleic acid. J Nutr. (2002) 132:2019–27. doi: 10.1093/jn/132.7.2019
36. Thomsen LE, Knudsen KE, Jensen TK, Christensen AS, Moller K, Roepstorff A. The effect of fermentable carbohydrates on experimental swine dysentery and whip worm infections in pigs. Vet Microbiol. (2007) 119:152–63. doi: 10.1016/j.vetmic.2006.09.004
37. Balsbaugh RK, Curtis SE, Meyer RC. Body weight, total body water and hematocrit in diarrheic piglets. J Anim Sci. (1986) 62:307–14. doi: 10.2527/jas1986.622307x
38. Suzuki T. Regulation of intestinal epithelial permeability by tight junctions. Cell Mol Life Sci. (2013) 70:631–59. doi: 10.1007/s00018-012-1070-x
39. Spreeuwenberg MA, Verdonk JM, Gaskins HR, Verstegen MW. Small intestine epithelial barrier function is compromised in pigs with low feed intake at weaning. J Nutr. (2001) 131:1520–7. doi: 10.1093/jn/131.5.1520
40. Kaleczyc J, Podlasz P, Winnicka A, Wasowicz W, Sienkiewicz W, Zmudzki J, et al. Characterization of autonomic nerve markers and lymphocyte subsets in the ileal Peyer's patch of pigs infected experimentally with Brachyspira hyodysenteriae. J Comp Pathol. (2010) 143:248–57. doi: 10.1016/j.jcpa.2010.04.003
41. Argenzio RA. Glucose-stimulated fluid absorption in the pig small intestine during the early stage of swine dysentery. Am J Vet Res. (1980) 41:2000–6.
42. Wilberts BL, Arruda PH, Kinyon JM, Madson DM, Frana TS, Burrough ER. Comparison of sesion severity, distribution, and colonic mucin expression in pigs with acute swine dysentery following oral inoculation with “Brachyspira hampsonii” or Brachyspira hyodysenteriae. Vet Pathol. (2014) 51:1096–108. doi: 10.1177/0300985813516646
43. Bruewer M, Luegering A, Kucharzik T, Parkos CA, Madara JL, Hopkins AM, et al. Proinflammatory cytokines disrupt epithelial barrier function by apoptosis-independent mechanisms. J Immunol. (2003) 171:6164–72. doi: 10.4049/jimmunol.171.11.6164
44. Gagnon M, Zihler Berner A, Chervet N, Chassard C, Lacroix C. Comparison of the Caco-2, HT-29 and the mucus-secreting HT29-MTX intestinal cell models to investigate Salmonella adhesion and invasion. J Microbiol Methods. (2013) 94:274–9. doi: 10.1016/j.mimet.2013.06.027
45. Blaxter K, Wood W. Some observations on the biochemical and physiological events associated with diarrhoea in calves. Vet Rec. (1953) 65:889–92.
46. Bush L, Schuh J, Tennille N, Waller G. Effect of dietary fat and minerals on the incidence of diarrhea and rate of passage of diets in the digestive tract of dairy calves. J Dairy Sci. (1963) 46:703–9.
47. Bergman EN. Energy contributions of volatile fatty acids from the gastrointestinal tract in various species. Physiol Rev. (1990) 70:567–90. doi: 10.1152/physrev.1990.70.2.567
48. Ríos-Covián D, Ruas-Madiedo P, Margolles A, Gueimonde M, de los Reyes-Gavilán CG, Salazar N. Intestinal short chain fatty acids and their link with diet and human health. Front. Microbiol. 7:185. doi: 10.3389/fmicb.2016.00185
49. Li Q, Peng X, Burrough ER, Sahin O, Gould SA, Gabler NK, et al. Dietary soluble and insoluble fiber with or without enzymes altered the intestinal microbiota in weaned pigs challenged with enterotoxigenic E. coli F18. Front Microbiol. (2020) 11:1110. doi: 10.3389/fmicb.2020.01110
50. Diether NE, Willing BP. Microbial fermentation of dietary protein: an important factor in diet-microbe-host interaction. Microorganisms. (2019) 7:19. doi: 10.3390/microorganisms7010019
51. Windey K, De Preter V, Verbeke K. Relevance of protein fermentation to gut health. Mol Nutr Food Res. (2012) 56:184–96. doi: 10.1002/mnfr.201100542
52. Pryde SE, Duncan SH, Hold GL, Stewart CS, Flint HJ. The microbiology of butyrate formation in the human colon. FEMS Microbiol Lett. (2002) 217:133–9. doi: 10.1111/j.1574-6968.2002.tb11467.x
53. Blachier F, de Sá Resende A, da Silva Fogaça Leite G, Vasques da Costa A, Lancha Junior AH. Colon epithelial cells luminal environment and physiopathological consequences: impact of nutrition and exercise. Nutrire. (2018) 43:2. doi: 10.1186/s41110-018-0061-6
54. Yoshikawa S, Shinzawa-Itoh K, Tsukihara T. X-ray structure and the reaction mechanism of bovine heart cytochrome c oxidase. J Inorg Biochem. (2000) 82:1–7. doi: 10.1016/S0162-0134(00)00137-9
55. Leschelle X, Goubern M, Andriamihaja M, Blottière HM, Couplan E, Petit C, et al. Adaptative metabolic response of human colonic epithelial cells to the adverse effects of the luminal compound sulfide. Biochim Biophys Acta. (2005) 1725:201–12. doi: 10.1016/j.bbagen.2005.06.002
56. Cao S, Zhang Q, Wang C, Wu H, Jiao L, Hong Q, et al. LPS challenge increased intestinal permeability, disrupted mitochondrial function and triggered mitophagy of piglets. Innate Immun. (2018) 24:221–30. doi: 10.1177/1753425918769372
57. Pluske JR, Siba PM, Pethick DW, Durmic Z, Mullan BP, Hampson DJ. The incidence of swine dysentery in pigs can be reduced by feeding diets that limit the amount of fermentable substrate entering the large intestine. J Nutr. (1996) 126:2920–33. doi: 10.1093/jn/126.11.2920
58. Kirkwood RN, Huang SX, McFall M, Aherne FX. Dietary factors do not influence the clinical expression of swine dysentery. J Swine Health Prod. (2000) 8:73–6.
59. Lindecrona RH, Jensen TK, Jensen BB, Leser TD, Jiufeng W, Moller K. The influence of diet on the development of swine dysentery upon experimental infection. Anim Sci. (2003) 76:81–7. doi: 10.1017/S1357729800053340
Keywords: pig, Brachyspira hyodysenteriae, intestinal integrity, digestibility, nutrition, fermentable fiber, insoluble fiber
Citation: Helm ET, Lin SJ, Gabler NK and Burrough ER (2020) Brachyspira hyodysenteriae Infection Reduces Digestive Function but Not Intestinal Integrity in Growing Pigs While Disease Onset Can Be Mitigated by Reducing Insoluble Fiber. Front. Vet. Sci. 7:587926. doi: 10.3389/fvets.2020.587926
Received: 27 July 2020; Accepted: 11 September 2020;
Published: 26 October 2020.
Edited by:
Rohana P. Dassanayake, National Animal Disease Center (USDA ARS), United StatesCopyright © 2020 Helm, Lin, Gabler and Burrough. This is an open-access article distributed under the terms of the Creative Commons Attribution License (CC BY). The use, distribution or reproduction in other forums is permitted, provided the original author(s) and the copyright owner(s) are credited and that the original publication in this journal is cited, in accordance with accepted academic practice. No use, distribution or reproduction is permitted which does not comply with these terms.
*Correspondence: Eric R. Burrough, YnVycm91Z2gmI3gwMDA0MDtpYXN0YXRlLmVkdQ==