- 1Escuela de Biología, Universidad de Costa Rica, San Pedro, Costa Rica
- 2Posgrado Regional en Ciencias Veterinarias Tropicales, Universidad Nacional de Costa Rica, San Pedro, Costa Rica
- 3Instituto Internacional de Conservación y Manejo de Vida Silvestre, Universidad Nacional de Costa Rica, San Pedro, Costa Rica
- 4Department of Microbiology, College of Arts and Sciences, University of Tennessee, Knoxville, Knoxville, TN, United States
- 5Centro de Investigación en Enfermedades Tropicales, Facultad de Microbiología, Universidad de Costa Rica, San Pedro, Costa Rica
- 6Departamento de Etología, Fauna Silvestre y Animales de Laboratorio, Facultad de Medicina Veterinaria y Zootecnia, Universidad Nacional Autónoma de México, Mexico City, Mexico
- 7Departamento de Ecología Humana, Centro de Investigaciones y de Estudios de Avanzados del IPN (Cinvestav), Unidad Mérida, Mérida, Mexico
- 8Department of Medicine and Epidemiology, School of Veterinary Medicine, University of California, Davis, Davis, CA, United States
The apicomplexan parasite Toxoplasma gondii (T. gondii) has been found in more than 350 species of homoeothermic vertebrates in diverse climates and geographic areas. In most animals, T. gondii produces mild or asymptomatic infection. However, acute and hyperacute toxoplasmosis is associated with high mortality rates observed in Neotropical primates (NP) in captivity. These primates are distributed in 20 countries across the Americas, and although infection has been reported in certain countries and species, toxoplasmosis in the wild and its impact on NP population survival is unknown. Differences among species in exposure rates and disease susceptibility may be due in part to differences in host behavior and ecology. Four species of NP are found in Costa Rica, i.e., howler (Alouatta palliata), spider (Ateles geoffroyi), capuchin (Cebus imitator), and squirrel monkeys (Saimiri oerstedii). This study reports NP exposure to T. gondii using the modified agglutination test in 245 serum samples of NP (198 wild and 47 from captivity) from Costa Rica. Associations of serostatus with environmental (forest cover, annual mean temperature), anthropogenic (human population density), and biological (sex) variables in howler and capuchin monkeys were evaluated. The seroprevalence among wild NP was 11.6% (95% CI = 7.7–17.34), compared with 60% in captive monkeys (95% CI = 44.27–73.63), with significant differences between species (X2 = 20.072; df = 3, p = 0.000164), suggesting an effect of behavior and ecology. In general, antibody titers were low for wild NP (<1:128) and high for captive NP (>1:8192), suggesting higher exposure due to management factors and increased life span in captivity. Seropositivity in howler monkeys was positively related to forest cover and inversely related to annual rainfall. For capuchins, annual rainfall was inversely related to seropositivity. Surveillance of T. gondii exposure in NP in captivity and in the wild is required to understand drivers of the infection and develop novel strategies to protect them.
Introduction
Toxoplasma gondii (T. gondii) is an obligate intracellular protozoan parasite that can infect more than 350 species of mammals and birds worldwide (1–3). It is the single species within its genus, in the phylum Apicomplexa (4). The parasite (5) undergoes sexual reproduction to produce oocysts in most domestic and wild felids, which function as definitive hosts and excrete oocysts in their feces. Oocysts are the infective stage for intermediate hosts, and thus, felid latrines are a source of contamination for intermediate hosts (6). T. gondii can also be transmitted via ingestion of encysted organisms in tissue (7), allowing the parasite to bio-accumulate in intermediate hosts such as carnivores and scavengers. In addition, several species of cockroaches, earthworms, and beetles function as mechanical hosts (1, 8).
Seroprevalence of T. gondii antibodies in hosts varies spatially (9) and temporally, and is influenced by climate (9, 10). For example, higher seroprevalence has been observed during years with high temperatures or high rainfall in humans (11), rabbits (12), wild ruminants (13), and domestic cats (9, 14, 15). Infection risk is often moderated through impacts on the distribution of domestic and wild cats, which are influenced by human factors such as the presence of domestic and peridomestic rodents, intra or inter specific territorial interactions, environmental stress, vegetation, and landscape characteristics (16).
Host-specific factors also influence susceptibility to T. gondii, with higher prevalence sometimes detected in larger rodents and lagomorphs than smaller ones (17), and in species with a longer life expectancy (18). This may be due to greater exposure to oocysts in species with larger home ranges, longer life expectancies, and higher energy requirements, which are related to body size (19). Males with larger body masses may also consume more and larger prey than females (20), reflecting on seroprevalence in cats (17). In French Guiana, burrowing, granivorous, and insectivorous mammals had much higher prevalences than arboreal mammals (21). Mammals in terrestrial or mixed terrestrial/arboreal habits were more exposed to oocysts than strictly arboreal ones (18, 22).
Among Neotropical primates (NP), the pathology of T. gondii varies among infected species. Such contrast in susceptibility to toxoplasmosis could have evolved due to differences in ecology and behavior (23). Toxoplasmosis in the Callitrichinae NP subfamily (Saguinus, Leontopithecus, Callithrix) may cause almost 100% mortality, resulting in very low seroprevalence and contributing to difficulty in making ante-mortem diagnosis, particularly in free-ranging populations. The Saimiri and Aotus genera of Cebidae family and Ateles and Alouatta in Atelidae family may experience acute and severe toxoplasmosis signs, with mortality from 20 to 80%, allowing for seroprevalence of 15–66% (23). In contrast, signs among Cebus sp. NP are usually subacute and moderate, with a very low mortality rate that generates high and persistent immunoglobin G (IgG) titers (24). Reports in captivity range from 28 to 79% of infected Cebus sp. monkeys, compared with 30.2% of animals in the wild (24–26). Cebus sp. NP may have evolved greater resistance than other NP species after frequent exposure to T. gondii (23). Cebus sp. NP commonly forage for insects on the ground and drink water from puddles and water holes, where they may encounter oocysts (27). In addition, although most of the Cebus diet protein comes from invertebrates, they may consume a variety of vertebrates weighing up to 1/3 of their body weight and constituting up to 3% of their feeding time (27).
In Costa Rica four species of NP have been found. While the Central American white-faced capuchin (Cebus imitator) and the Mantled howler monkey (Alouatta palliata) are considered at low risk (least concern) according to the International Union for Conservation of Nature (IUCN) Red List, Geoffroy's spider monkey (Ateles geoffroyi) and both subspecies of the Central American squirrel monkey (Saimiri oerstedii oerstedii and S. o. citrinellus) are endangered mainly due to habitat loss and fragmentation (28–32). Some species are still captured for the illegal pet trade, and are, thus, protected from international trade under Appendix I of the Convention on International Trade in Endangered Species (33). However, the population size for these NP species is unknown and has never been estimated. Although Costa Rica has protected areas that cover 25% of the country (34), other conservation actions include a system of incentives to farmers known as payments of environmental services (PES) since 1997 (35), and a national program of biological corridors (PNCB) to increase connectivity between forest patches, species migration and genetic flow (36, 37). Ex situ actions include keeping some NP in zoos for education purposes and rescue centers across the country focus on rehabilitation and reintroduction of individuals back to protected areas.
The high mortality rates observed in some NP, which may range from 20 to 80% in Alouatta and Ateles, can imperil already at-risk populations, but the causes of mortality and high rates of exposure are poorly understood (23, 24), so it is important to assess the infection status in captive NP. Living in altered environments or in contact with humans (where domestic cats are present) can affect NP behavior (38) and exposure to infectious agents (39, 40). Thus, it is important to evaluate impacts of T. gondii infection in NP in different areas that experience differential contact with human-altered habitat and have differing behaviors and diet (23, 27). The present study analyzed T. gondii exposure in A. geoffroyi, A. palliata, C. imitator and S. oerstedii and the influences of environmental, anthropogenic, and biological variables in wild A. palliata and C. imitator in Costa Rica.
Materials and Methods
Ethics
The animal study was reviewed and approved by the Institutional Committee for the Care and Use of Animals (Comité Institucional para el Cuidado y Uso de los Animales) of the Universidad de Costa Rica, and adhered to the legal requirements of Costa Rica. Collection permit number: MINAET-SINAC-Costa Rica: 042-2012-SINAC.
Study Area
The small tropical country of Costa Rica is located in southern Central America (latitudes 8 02‘26‘ and 11 13‘12‘N, and longitudes 82 33‘48‘ and 85 57‘57‘W) and has an area of 51,100 km2 (with around 5% of the biodiversity in the planet). After having one of the world's highest deforestation rates during the 1970s, laws were changed and today, forested areas represent 52% of the country (41), with 25% being protected (34). Precipitation varies seasonally, being the highest from May to early November, and spatially, with wettest regions in the Caribbean slope receiving 3500 to 7000 mm of rainfall per year, and the driest receiving around 900 mm in Guanacaste province, in the Pacific slope (42).
Sampling
An observational study was conducted and 245 serum samples were opportunistically collected from four species of native Neotropical primates (NP), C. imitator, A. palliata, A. geoffroyi, and S. oerstedii, collected throughout Costa Rica in 2000–2015 as part of the project “Epidemiological, genetic, ethological and habitat studies in Costa Rica's monkeys” (Resolution 27-2013- National System of Conservation Areas, Figure 1A). No sample size calculation was previously conducted. Out of the 245 sampled NP, 198 were wild and 47 were in captivity. Most samples from captive animals came from three rescue centers, although 12 different sites were sampled. NP were anesthesized using IM injection of 3.3–11 mg/kg of Zoletil 50® or 5–20 mg/kg ketamine with 0.5–2 mg/kg xylazine (43–45), which for wild NP was loaded into darts (PneuDart. Inc, Type P, 1cc) and delivered using a compressed gas rifle (PneuDart. Inc, model X-Caliber Gauged CO2) targeting the lateral triceps and quadriceps femoris (43). After an individual was anesthetized, blood was sampled from the femoral vein on a plastic tube without anticoagulant, and placed into cooler at 4°C. Once in the laboratory, samples were centrifuged at 2000 RCF for 5 min to separate serum, which was transferred to sterile 1.5 ml tubes at −20°C until processed. Animals underwent a physical examination and were released upon awakening from anesthesia.
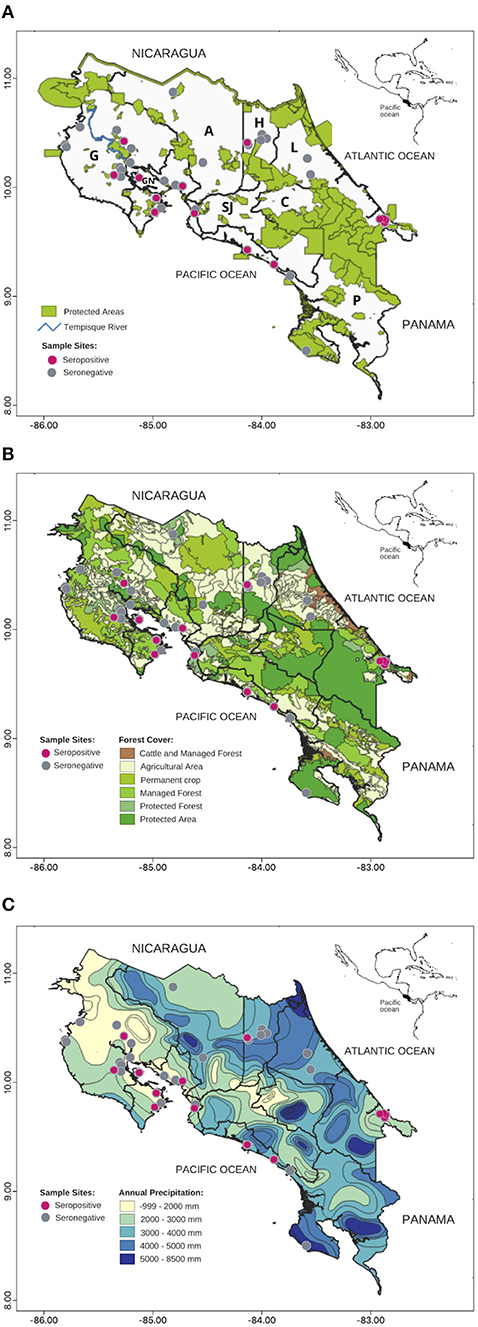
Figure 1. Map of Toxoplasma gondii seropositive wild Neotropical primates (NP) from Costa Rica from 2000 to 2015: (A) Protected areas, (B) Forest Cover, (C) Precipitation. Gray circles: seronegative NP, pink circles: seropositive NP. Gulf of Nicoya (GN), Provinces: Alajuela (A), Cartago (C), Guanacaste (G), Heredia (H), Limón (L), San José (SJ), Puntarenas (P).
Serological Analysis
A modified agglutination test (MAT) was used to detect IgG specific for T. gondii as previously described by Desmonts and Remington (46), and Dubey and Desmonts (47). Formalin-fixed tachyzoites were used as antigen (2 × 108 tachyzoites/mL from KeraFAST Inc., Boston, MA, USA. Catalog No. EH2002). Sera were diluted (1:4) in buffered saline solution (1X PBS) and 50 ul were added to the wells in the first column of 96-well U-bottom microplates. Using a multichannel pipette 25 ul PBS was added to the rest of the wells (columns 2–12) and sera from the first column were serially diluted to 1:8192. Finally, 25 ul of antigen mixture (2.5 ml alkaline buffer, 35 ul 2-mercaptoethanol, 50 ul Evans blue dye (2 mg/ml in H2O) and 150 ul T. gondii MAT antigen) were mixed by pipetting and carefully added to each well. The microplates were covered with sealing tape and incubated at 37°C for 24 h and subsequently for 4 h at 4°C. A positive reaction was characterized by a complete “mesh” of agglutination and a clear bottom, while sedimentation of antigen suspension in the form of a blue pellet at the bottom of the well was considered to be a negative reaction. Sera from mice and/or humans were included as positive (titers of 1:200 or greater) and negative controls in each group of processed sera. All antibody titers equal or higher than 1:16 were reported as positive. Titer values from captive vs. wild NP were analyzed using a Mann-Whitney two-tailed test to know if there were significant differences (at a 5% significance level). Pearson's chi-squared test was used to examine differences in individuals within species, sex and serostatus for each group of NP (captive and wild). Small samples (n < 6) were assessed with Fisher's exact test. A p < 0.05 value was used as significance level, and 95% confidence intervals (CI 95%) were included.
Biological, Environmental, and Anthropogenic Drivers
Due to insufficient sample size, data for A. geoffroyi and S. oerstedii were not included for further statistical analyses. Seven independent variables were explored for their association with exposure to T. gondii in A. palliata and C. imitator. Distance to villages, human population size, and human population density in the nearest village were considered indirect indicators of proximal domestic cats. The percentage of forest cover, annual average temperature, and annual precipitation were used as variables that affect oocyst survival. NP sex was used as an intrinsic factor for oocyst exposure (Table 1).
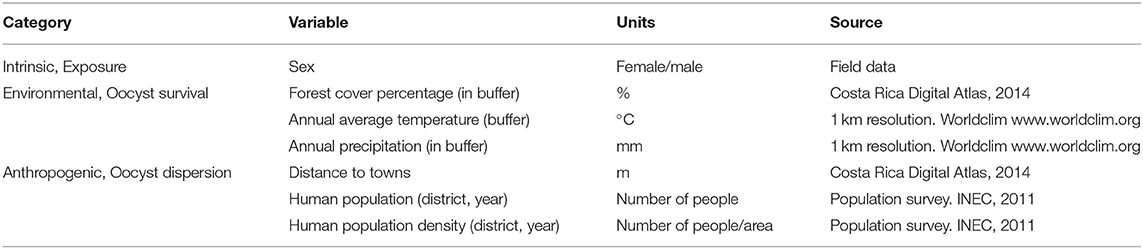
Table 1. Independent variables chosen for Generalized Linear Models (GLM) Toxoplasma gondii exposure in wild Alouatta palliata and Cebus imitator.
Forest cover, annual average temperature, and annual precipitation were measured within a 1.54 km2 circular area (700 m radius) that was constructed around each sample's geolocation point (QGIS software, version 2.14). Data for these variables were obtained from Costa Rica Digital Atlas (2014) and WorldClim (48). The buffer size aimed to include both species' home ranges and adjacent areas (49).
Generalized linear models (GLM) with binomial distribution and logit link were run in R V3.4.2 (50) to explore associations between predictor variables and T. gondii seropositivity. The original predictors (forest cover and precipitation) were centered by subtracting their means and scaled by dividing by their standard deviations. The most parsimonious model was chosen by selecting the one with the lowest Akaike information criterion (AIC). Akaike weights (wi) were calculated to determine the evidence in favor of each model and estimate the relative importance of variables (Table 2).
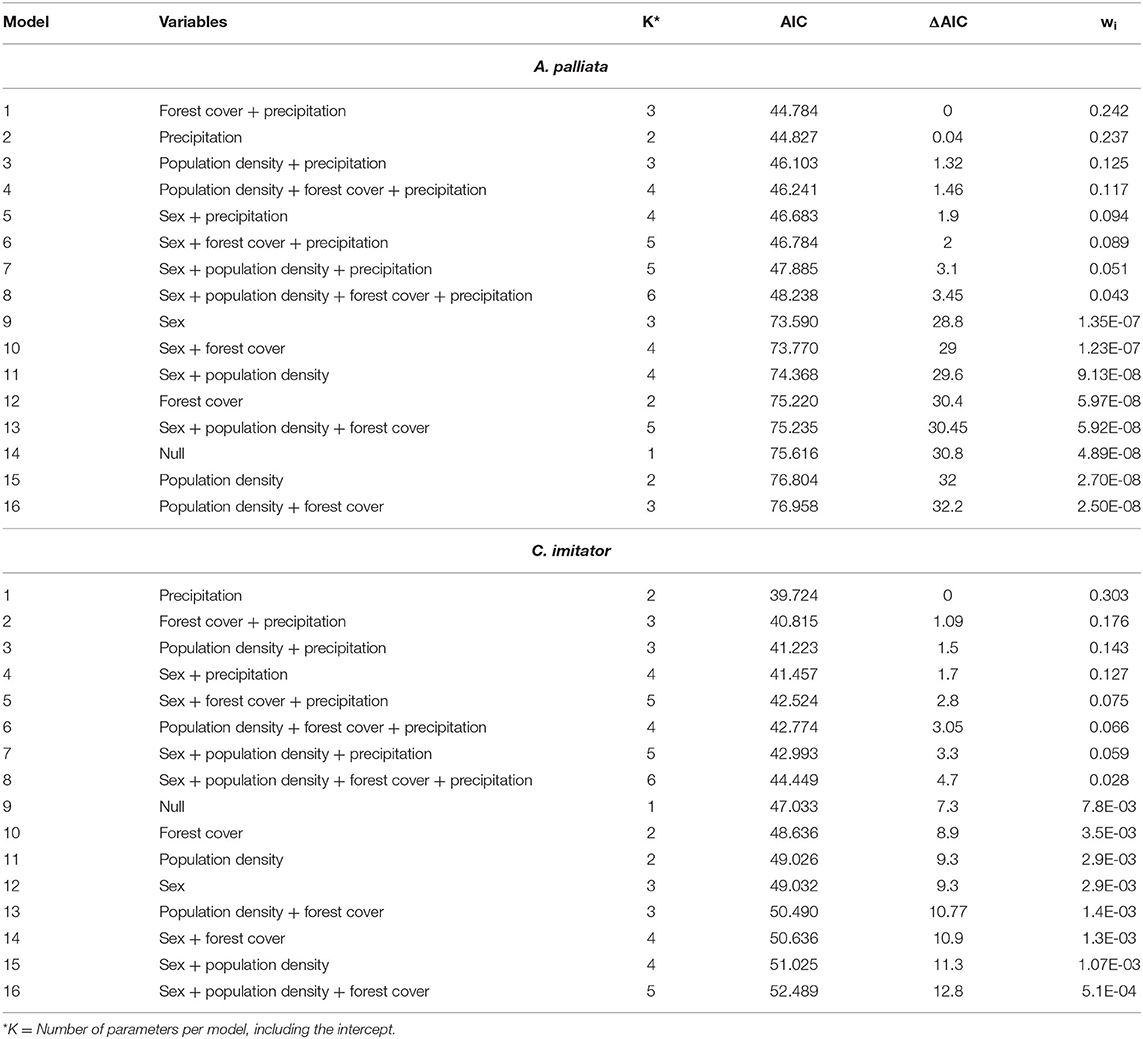
Table 2. Generalized Linear Models that explain intrinsic, environmental, and anthropogenic attributes on Toxoplasma gondii seroprevalence in Alouatta palliata and Cebus imitator.
Each NP species was modeled separately. Multicollinearity among the seven variables was evaluated by means of the variance inflation factor (VIF). When analyzing all the proposed variables, a very high VIF value (>10) was obtained suggesting multicollinearity, therefore the model was reduced to four variables (sex, forest cover, annual precipitation, and human population density). With these models, none of the variables obtained a VIF value <10 (Table 2).
Results
Toxoplasma gondii-specific IgG antibodies were found in 59.6% (28/47, 95% CI = 44.27–73.63) of captive NP and 11.6% (23/198, 95% CI = 7.7–17.34) of wild NP. In captive NP, seroprevalence was high for both species: 59.1% (26/44, 95% CI = 43.25–73.66) of A. geoffroyi and 66.7% (2/3, 95% CI = 9.43–99.16) of C. imitator. There were no significant differences in species or sex with 58.6% (17/29, 95% CI = 38.94–76.47) of A. geoffroyi females and 60.0% (9/15, 95% CI = 32.29–83.66) males being positive, while for C. imitator, 50.0% (1/2, 95% CI = 1.26–98.74) females and 100% (1/1, 95% CI = 2.5–100) male presented positive results (Tables 3, 4).
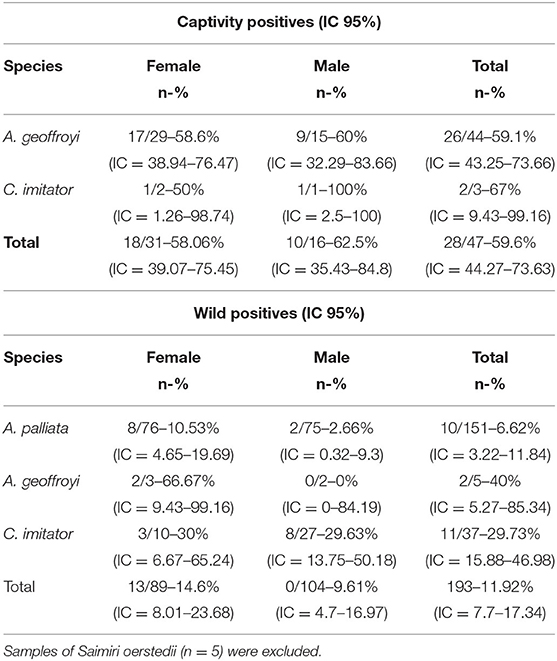
Table 3. Prevalence of Toxoplasma gondii antibodies in captive and wild Neotropical primates in Costa Rica according to species and sex.
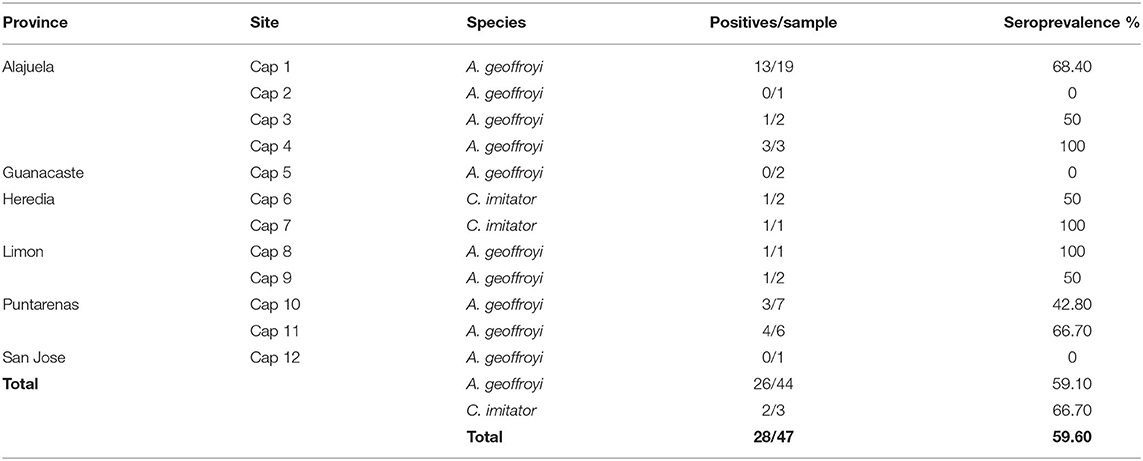
Table 4. Seroprevalence of Toxoplasma gondii in captive Neotropical Primates from Costa Rica according to site and species.
In contrast, there was a significant difference in seroprevalence among wild NP species (X2 = 20.072; df = 3; p = 0.000164): A. geoffroyi had the highest seroprevalence with 40% (2/5, 95% CI = 5.27–85.34), followed by C. imitator with 30% (11/37, 95% CI = 15.88–46.98) and A. palliata with 6.6% (10/151, 95% CI = 3.22–11.84), while no S. oerstedii were positive (0/5). No significant difference was found regarding sex for any of the species in the wild group; however, greater seroprevalence was observed for females in the three positive species (Tables 3, 5).
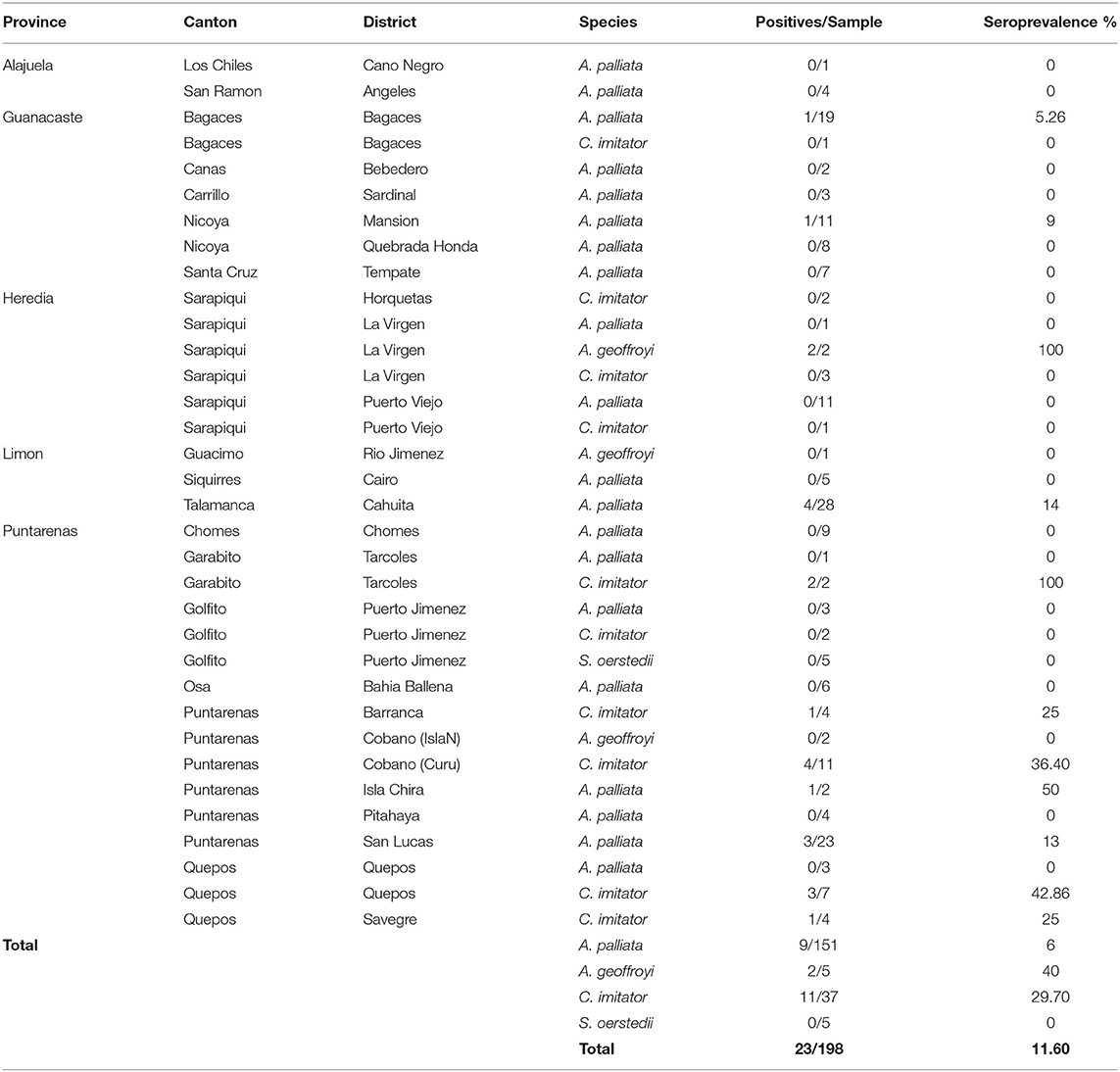
Table 5. Seroprevalence of Toxoplasma gondii in wild Neotropical Primates from Costa Rica according to sample site and species.
Antibody titers tended to be relatively low (Median = 8) in wild NP (<1:128), with the exception of four individuals: one A. geoffroyi and two C. imitator with antibody titers of 1:262,144 and one A. geoffroyi with a titer of 1:1,048,576). In contrast, antibody titers were considerably higher (Median = 262,144) in captive NP, from 1:8,192 to 1:33,554,000 (Figure 2). These two groups of NP were statistically different in their titer values (p < 0.00001).
The model that best accounted for seropositivity among A. palliata (minimizing AIC) was forest cover and annual precipitation (Figure 1B and Table 2), with a positive relationship between forest cover and seropositivity and inverse relationship with annual precipitation. However, evidence weights (wi) for the second and third best-supported models were 1.02 and 1.94 times lower than model 1 respectively, which suggests that no one model is strongly supported as the best candidate. The second best model was annual precipitation (ΔAIC = 0.04) and the third was for human population density and annual precipitation (ΔAIC = 1.32). Notably, the precipitation parameter appeared among the top eight models.
For C. imitator, annual precipitation alone best accounted for seropositivity with a significant inverse relationship (Figures 1C, 2 and Table 2). The next best models were forest cover and annual precipitation (ΔAIC = 1.09) and human population density and precipitation (ΔAIC = 1.5), with evidence weights 1.73 and 2.12 times lower than model 1, respectively. Again, the effect of precipitation on seropositivity was important for C. imitator when comparing the sum of the weights of Akaike (wi) in the models that contained this variable (0.98) with respect to the others, such as forest cover (0.35), population density (0.3), and sex (0.29).
Discussion
The present survey documented widespread exposure and species-specific risks of T. gondii infection. The seroprevalence obtained for captive NP is similar to other studies in South America, such as 30.8 (26) and 76% (51) using MAT in captive Cebus apella, and 79% in Cebus sp. and 57% in Ateles sp. from zoos in São Paulo, Brazil (24). The high prevalence of NP in captivity may be due to management practices, including improperly washed fruits or vegetables, and raw or undercooked meat (51, 52), proximity of wild or domestic cats, and invasion of enclosures by infected birds and rodents that might be ingested by NP (51, 52). These sources of infection would be similar for male and female NP in captivity, consistent with our findings.
The 11.6% seroprevalence in wild Costa Rican NP was lower than the 26.6% (16/60) reported by Garcia et al. (25) using MAT in Brazil, and the prevalence we found for A. palliata (6.6%) is lower than documented for Alouatta caraya by Garcia et al. (25) in Paraná (17%, 3/17) and Molina et al. (53) in São Paulo, Brazil (75%, 15/20). However, it is higher than findings by de Thoisy et al. (54), who found only 4% (2/50) seropositive Alouatta senilicus in French Guiana, but similar in the observed proportion of positive females. The present data did not indicate a significant difference between sexes for any of the species, although this should be studied further given the sex bias observed in French Guiana and among domestic cats (17).
In the case of C. imitator, the prevalence is similar to that reported by Garcia et al. (25) in Cebus spp. with 30.2% (13/43). In fact, several studies have identified that carnivorous diet is a risk factor for T. gondii infection (55, 56). On the other hand, the prevalence found in A. geoffroyi (40%, 2/5) appears to be high, which is surprising considering its arboreal and herbivorous (frugivorous) behavior, characteristics that minimize its exposure to the parasite. No prevalence reports were found for wild Ateles sp. to compare this finding to. However, sample sizes for both A. geoffroyi and S. oerstedii were small, so these findings should be interpreted with caution. The difference in seropositivity observed between howler (A. palliata) and capuchin monkeys (C. imitator) coincides with Garcia et al. (25) and corresponds to behavioral characteristics between species. Howlers are predominantly folivorous, supplementing their diet with fruits, flowers and seeds, and obtaining most of the water they need from their food [reviewed by (49)]. However, they can drink water accumulated in branches, trunks or bromeliads (57), or search for it on the ground (58). The source of infection for howlers would be water bodies infected with oocysts. Meanwhile, capuchins are the most omnivorous NP, feeding on various sources like fruits, insects and small vertebrates such as birds, rodents, squirrels, coatis, bats, frogs, and lizards [reviewed by (23, 49)]. They frequently go down to the undergrowth and ground while foraging and traveling (59). In addition, Cebus spp. drink water directly from puddles (27). These characteristics give capuchins greater exposure to oocysts in the soil, water, in invertebrates (transport hosts), and to tissue cysts present in infected vertebrates.
The low antibody titers obtained for the wild NP (1:16 and 1:32) coincide with those reported by Garcia et al. (25), who found mostly 1:16 and 1:32 for a single individual. While A. palliata had low antibody titers, two A. geoffroyi and two C. imitator had very high antibody titers (1:262,144–1:1,048,576). Molina et al. (53) reported values of 1:25 for Callithrix penicillata (a very susceptible species) and higher titers (up to 1:1,600) for A. caraya, arguing that differences in prevalence and titers could respond to differences in host susceptibility, contact rates or post-exposure time. Indeed, Alouatta's susceptibility in comparison to Cebus could be reflected in serological differences, since the probability of post-infection survival and, therefore, developing an immune response is naturally lower for howlers. Furthermore, if these individuals die, they would be excluded from the population and the sample, reducing the number of individuals captured with high titers. In contrast, titers of captive NP were high in this study. Leite et al. (26) reported antibody titers of 1:8,000 by MAT for Cebus sp. in captivity, while Ekanayake et al. (40) reported antibody titers >1:256 (up to 1:4,096) in 3 of 21 positive free-ranging but urban macaques in Sri Lanka. Management factors along with the increased life expectancy for Cebus sp. and Ateles sp. in captivity could explain the chance of infection and high titers observed in this group.
It is worth highlighting the large number of positive samples from the Gulf of Nicoya (Figure 1A). Tempisque River is one of the most important basins in the country, draining 10.6% of the territory and flowing into the Gulf of Nicoya (60). Areas close to bodies of water could represent sources of infection for NP, because water can be contaminated at any point, transport oocysts long distances, and favor their survival (2). Samples from protected areas with high levels of human contact also showed high prevalence and high titers in the two wild C. imitator mentioned before. In certain national parks and private reserves, feeding wild monkeys is a common practice, and capuchin and squirrel monkeys sometimes exhibit agonistic behaviors that include taking food directly from humans and coming down to the ground. Such opportunistic behavior (61) may increase exposure to infectious agents (39, 40).
Besides identifying specific areas where there could be elevated risk of infection, this data indicates that environmental variables such as forest cover and precipitation could be associated with exposure risk. Seropositivity in A. palliata was higher when there was a higher percentage of forest cover and less annual precipitation. Forest cover can protect oocysts from sunlight, allowing them to remain viable for 1–1.5 years, when protected (62, 63). Smith and Frenkel (64) and Almería et al. (12) found greater seroprevalence in hares and other mammals sampled in forested areas vs. more arid grasslands, arguing that shadow and relative humidity provided by forest cover act on oocyst conservation by decreasing the evaporation rate and desiccation of oocysts.
Wet seasons tend to increase oocyst survival (63). In fact, T. gondii seroprevalence in cats (15) and humans (11) has been associated with rainy and warm episodes (North Atlantic Oscillation), and in wild ruminants (13) with humid areas. Contrary to expectations, in this study precipitation was inversely related with seropositivity in both NP species. Costa Rica is a tropical country with high relative humidity and stable temperature overall. It is possible that increased precipitation in Costa Rica results in greater runoff, transporting oocysts toward the coasts and away from the animals. In recent past years, low rainfall due to the El Niño phenomenon has generated severe droughts and forest fires in some areas of Costa Rica. Among many other animals, A. palliata were severely affected by water and food shortages, with high mortality due to dehydration and starvation, as well as injuries due to troops fighting for food. Because behavior change driven by droughts can increase exposure to parasites present in the scarce sources of water, the risk of disease increases especially for animals weakened by starvation and dehydration.
In the present study, the source of exposure of wild NP could be wild and not domestic cats, which might explain the low effect of human population density on seropositivity. Contact with humans has been associated with high seropositivity in macaques (40), which can become infected by ingesting human food from the ground in areas frequented by domestic cats (3). Domestic cat population estimates as well as sampling in areas where humans feed and interact with wildlife should be included in future studies.
Additionally, Toxoplasma genotypes produce different degrees of virulence in humans and mice (65), and given the high diversity recently found in Central and South America (Ajzenberg et al., 2004; Lehmann et al., 2004) (66–69) the observed differences in seroprevalence between wild and captive NP might be due to different genotypes. Little is known about the genotypes circulating in wildlife, and associations between strain type, lesion patterns, and clinical outcome have not been reported in wildlife frequently. Thus, future studies that focus on genotyping and virulence of T. gondii isolates in wildlife and domestic animals from wild and anthropized environments would be of great value.
Antibody titer of 1:25 is often considered as evidence of exposure to T. gondii in many mammals (70) and 1:5 in birds (71). However, there is no antibody titer that is considered specific for primates to maximize sensitivity of detection given the high susceptibility and low seroprevalence in some NP species, we reported all antibody titers of 1:16 or higher (25).
This study documented widespread T. gondii infection in NP and species-specific risks of infection for the first time in Costa Rica (Figure 3). The high seroprevalence and titers found in captive capuchin and spider monkeys may be due to management practices, the proximity of cats or intermediate hosts, and the increased life expectancy in captivity for these species. Because the number of samples was small, especially in the case of C. imitator, further studies should assess these findings. The low seroprevalence and titers in wild NP varied between species. The difference in seropositivity observed between wild howler (A. palliata) and capuchin monkeys (C. imitator) in this study agrees with behavioral and dietary characteristics, in which capuchins are more exposed to oocysts while foraging on the ground, and by ingesting invertebrates (transport hosts) and vertebrates (tissue cysts). However, Alouatta's susceptibility compared to Cebus might also explain the observed serological differences, due to decreased survival. These data indicated that specific areas could represent an elevated risk of infection (i.e., water runoff and human interaction), and environmental variables such as abundant forest cover and low precipitation could be associated with higher exposure risk in wild NP. Surveillance of T. gondii in NP is required to better understand the infection status, genotypes, and drivers involved in wild and captive NP, including individuals in the process of reintroduction, so that biosecurity measures are improved, avoiding the release of infected individuals, and developing novel strategies to protect wild populations.
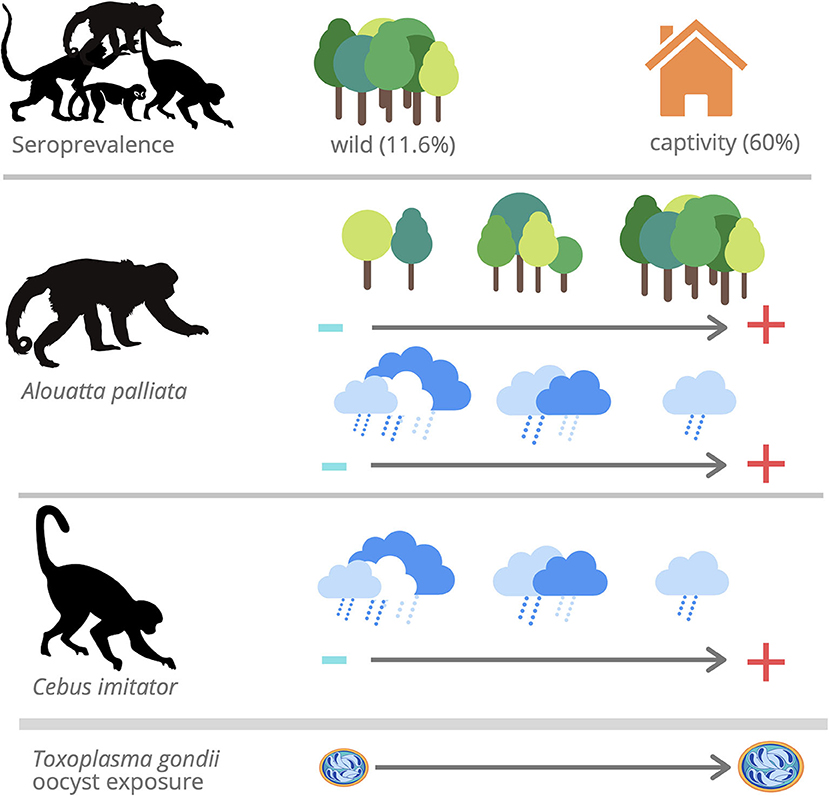
Figure 3. Toxoplasma gondii IgG antibodies were found in 59.6% (28/47) of captive NP and 11.6% (23/198) of wild NP. The model that best accounted for seropositivity among Aloutta palliata with a positive relationship between forest cover and seropositivity and inverse relationship with annual precipitation. The model that best accounted for seropositivity among Cebus imitator with an inverse relationship with annual precipitation.
Data Availability Statement
The raw data supporting the conclusions of this article will be made available by the authors, without undue reservation.
Ethics Statement
The animal study was reviewed and approved by the Institutional Committee for the Care and Use of Animals (Comité Institucional para el Cuidado y Uso de los Animales) of the Universidad de Costa Rica, and adhered to the legal requirements of Costa Rica. Collection permit number: MINAET116 SINAC-Costa Rica: 042-2012-SINAC.
Author's Note
Toxoplasma gondii is a widespread zoonotic protozoan that has a wide host range, which includes birds and mammals. It uses different routes and modes of transmission to move from their definitive hosts (domestic and wild felids) to the intermediate hosts. Even if the T. gondii infections usually produce low or any symptoms, in Neotropical primates it is a severe disease with a high rate of mortality. However, there are scarce studies for these species of monkeys, and the factors associated their infection in wildlife. The oocysts are the infective stage for intermediate hosts; they are excreted by felids in their feces, and maintained in the environment waiting for an intermediate host. For that reason it is important to determine the influences of environmental, anthropogenic, and biological variables for the exposure of T. gondii in Neotropical primates. In our study we found that forest cover is associated with the presence of Alouatta palliata seropositives, and inversely related to annual rainfall with Alouatta palliata and Cebus imitator seropositives. The difference in exposure between monkey species reflects influence of ecology and behavior. High antibody titers in captivity suggest increased exposure due to management.
Author Contributions
CN and AC conceived the investigation and wrote the article. AC and GG-E collected samples. CN, NR, and CS conducted the laboratory analysis. CN, MS, CI-C, AC, and OR-C performed the statistical analysis. JF and GS copy reviewed and edited. All authors reviewed the manuscript.
Funding
This research was carried out thanks to funding by the Cleveland Zoological Society and Cleveland Metroparks Zoo.
Conflict of Interest
The authors declare that the research was conducted in the absence of any commercial or financial relationships that could be construed as a potential conflict of interest.
Acknowledgments
We are grateful to all contributors who assisted with sampling endeavors, especially Mauricio Jimenez, Maria Isabel DiMare, Sofia Bernal, Genuar Nuñez, Natalia Valverde, and Mauricio Losilla. We would also like to thank the National System of Conservation Areas (SINAC), and the Ministry of Environment and Energy (MINAE) of Costa Rica. This research was carried out thanks to funding by the Cleveland Zoological Society and Cleveland Metroparks Zoo.
References
1. Dubey JP. Toxoplasmosis of Animals and Humans. 2nd Ed. Boca Raton, FL: CRC Press Taylor and Francis Group (2010). p. 338.
2. Lindsay DS, Dubey JP. Toxoplasmosis in wild and domestic animals. In: Weiss LM, Kim K. editors. Toxoplasma gondii: The Model Apicomplexan. Perspectives and Methods. London: Academic Press. (2007). p. 133–52. doi: 10.1016/B978-012369542-0/50008-8
3. Tenter AM, Heckerothm AR, Weiss LM. Toxoplasma gondii: from animals to humans. Toxoplasma gondii: from animals to humans. Int. J. Parasitol. 30, 1217–58. doi: 10.1016/S0020-7519(00)00124-7
4. Roberts LS, Janovy JR. Phylum Apicomplexa: Gregarines, Coccidia, and related organisms. En Foundations of Parasitology. EUA: The McGraw-Hill Companies, Inc. (2000). p. 117–140.
5. Dubey JP, Miller NL, Frenkel JK. The Toxoplasma gondii oocyst from cat feces. J Exp Med. (1970) 132:636–62. doi: 10.1084/jem.132.4.636
6. VanWormer E, Fritz H, Shapiro K, Mazeta JAK, Conrad PA. Molecules to modeling: Toxoplasma gondii oocysts at the human-animal-environment interface. Comp Immunol Microb. (2013) 36:217–31. doi: 10.1016/j.cimid.2012.10.006
7. Su C, Evans D, Cole RH, Kissinger JC, Ajioka JW, Sibley LD. Recent expansion of Toxoplasma through enhanced oral transmission. Science. (2003) 299:414–6. doi: 10.1126/science.1078035
8. Chinchilla M, Guerrero OM, Castro A, Sabah J. Cockroaches as transport hosts of the protozoan Toxoplasma gondii. Rev Biol Trop. (1994) 42:329–31.
9. Afonso E, Thulliez P, Gilot-Fromont E. Local meteorological conditions, dynamics of seroconversion to Toxoplasma gondii in cats (Felis catus) and oocyst burden in a rural environment. Epidemiol infect. (2010) 138:1105–13. doi: 10.1017/S0950268809991270
10. Salant H, Spira DT. A cross-sectional survey of anti-Toxoplasma gondii antibodies in Jerusalem cats. Vet Parasitol. (2004) 124:167–77. doi: 10.1016/j.vetpar.2004.07.011
11. Hubálek Z. North Atlantic weather oscillation and human infectious diseases in the Czech Republic, 1951–2003. Eur J Epidemiol. (2005) 20:263–70. doi: 10.1007/s10654-004-6518-3
12. Almería S, Calvete C, Pagés A, Gauss CBL, Dubey JP. Factors affecting the seroprevalence of Toxoplasma gondii infection in wild rabbits (Oryctolagus cuniculus) from Spain. Vet Parasitol. (2004) 123:265–70. doi: 10.1016/j.vetpar.2004.06.010
13. Gamarra JA, Cabezón O, Pabón M, Arnal MC, Luco DF, Dubey JP, et al. Prevalence of antibodies against Toxoplasma gondii in roe deer from Spain. Vet Parasitol. (2008) 153:152–6. doi: 10.1016/j.vetpar.2008.01.028
14. Afonso E, Thulliez P, Gilot-Fromont E. Transmission of Toxoplasma gondii in an urban population of domestic cats (Felis catus). Int J Parasitol. (2006) 36:1373–82. doi: 10.1016/j.ijpara.2006.07.010
15. Afonso E, Germain E, Poulle ML, Ruette S, Devillard S, Say L, et al. Environmental determinants of spatial and temporal variations in the transmission of Toxoplasma gondii in its definitive hosts. Int J Parasitol. (2013) 2:278–85. doi: 10.1016/j.ijppaw.2013.09.006
16. Horn JA, Mateus-Pinilla N, Warner RE, Heske EJ. Home range, habitat use, and activity patterns of free-roaming domestic cats. J Wildl Manag. (2011) 75:1177–85. doi: 10.1002/jwmg.145
17. Afonso E, Thulliez P, Pontier D, Gilot-Fromont E. Toxoplasmosis in prey species and consequences for prevalence in feral cats: Not all prey species are equal. Parasitology. (2007) 134:1963–71. doi: 10.1017/S0031182007003320
18. De Thoisy B, Demar M, Aznar C, Carme B. Ecologic correlates of Toxoplasma gondii exposure in free-ranging neotropical mammals. J Wildl Dis. (2003) 39:456–9. doi: 10.7589/0090-3558-39.2.456
19. Ottaviani D, Cairns SC, Oliverio M, Boitani L. Body mass as a predictive variable of homerange size among Italian mammals and birds. J Zool. (2006) 269:317–30. doi: 10.1111/j.1469-7998.2006.00060.x
20. Niewold FJJ. Voedselkeuze, terreingebruik en aantalsregulatie van in het veld operende huiskatten Felis catus L., 1758. Lutra. (1986) 29:145–87.
21. Carme B, Aznar C, Motard A, Demar M, De Thoisy B. Serologic survey of Toxoplasma gondii in noncarnivorous free-ranging neotropical mammals in French Guiana. Vector Borne and Zoonotic Dis. (2002) 2:11–17. doi: 10.1089/153036602760260733
22. Nardoni S, Angelici MC, Mugnaini L, Mancianti F. Prevalence of Toxoplasma gondii infection in Myocastor coypus in a protected Italian wetland. Parasit Vect. (2011) 23:240–3. doi: 10.1186/1756-3305-4-240
23. Catão-Dias JL, Epiphanio S, Kierulff MCM. Neotropical primates and their susceptibility to Toxoplasma gondii: new insights for an old problem. In: Brinkworth JF, Pechenkina K, editors. Primates, Pathogens, and Evolution, Developments in Primatology: Progress and Prospects. New York: Springer (2013). p. 253–89. doi: 10.1007/978-1-4614-7181-3_9
24. Bouer A, Werther K, Machado RZ, Nakaghi AC, Epiphanio S, Catão-Dias JL. Detection of anti-Toxoplasma gondii antibodies in experimentally and naturally infected non-human primates by Indirect Fluorescence Assay (IFA) and indirect ELISA. Revist Brasil Parasitol Vet. (2010) 19:26–31. doi: 10.1590/S1984-29612010000100006
25. Garcia JL, Svoboda WK, Chryssafidis AL, de Souza Malanski L, Shiozawa MM, de Moraes Aguiar L, et al. Sero-epidemiological survey for toxoplasmosis in wild New World monkeys (Cebus spp., Alouatta caraya) at the Paraná river basin, Paraná State, Brazil. Vet Parasitol. (2005) 133:307–11. doi: 10.1016/j.vetpar.2005.06.004
26. Leite TN, Maja T, Ovando TM, Cantadori DT, Schimidt LR, Guercio AC, et al. Occurrence of infection Leishmania spp. and Toxoplasma gondii in monkeys (Cebus apella) from Campo Grande, MS. Revist Brasil Parasitol Vet. (2008) 17:307–10.
27. Fragaszy DM, Visalberghi E, Fedigan LM. The Complete Capuchin—the Biology of the Genus Cebus. Cambridge: Cambridge University Press (2004).
28. Cuarón AD, Shedden A, Rodriguez-Luna E, de Grammont PC, Link A, Palacios E, et al. Alouatta palliata. The IUCN Red List of Threatened Species 2008: e.T39960A10280447 (2008). Available online at: https://dx.doi.org/10.2305/IUCN.UK.2008.RLTS.T39960A10280447.en (accessed August 13, 2020).
29. Cuarón AD, Morales A, Shedden A, Rodriguez-Luna E, de Grammont PC, Cortés-Ortiz L. Ateles geoffroyi. The IUCN Red List of Threatened Species (2008). Available online at: https://dx.doi.org/10.2305/IUCN.UK.2008.RLTS.T2279A9387270.en (accessed August 13, 2020).
30. Cuarón AD, Morales A, Shedden A, Rodriguez-Luna E, de Grammont PC. Cebus capucinus ssp. capucinus. The IUCN Red List of Threatened Species (2008). Available online at: https://dx.doi.org/10.2305/IUCN.UK.2008.RLTS.T43934A10841636.en (accessed August 13, 2020).
31. Cuarón AD, Morales A, Shedden A, Rodriguez-Luna E, de Grammont PC. Saimiri oerstedii ssp. citrinellus. The IUCN Red List of Threatened Species (2008). Available online at: https://dx.doi.org/10.2305/IUCN.UK.2008.RLTS.T19841A9023545.en (accessed August 13, 2020).
32. Cuarón AD, Morales A, Shedden A, Rodriguez-Luna E, de Grammont PC. Saimiri oerstedii ssp. oerstedii. The IUCN Red List of Threatened Species (2008). Available online at: https://dx.doi.org/10.2305/IUCN.UK.2008.RLTS.T19840A9023408.en (accessed August 13, 2020).
33. CITES Appendices I II and III (2019). Available online at: https://cites.org/eng/app/appendices.php (accessed June 3, 2020).
34. González F, Lobo S. Breve descripción del estado de los recursos forestales de Costa Rica. En: Kleinn C y Davis R. Memoria del Taller sobre el programa de evaluación de los recursos forestales en once países latinoamericanos. Turrialba: FAO, SIDA, CATIE (1999). p. 65–81.
35. Pagiola S. Payments for environmental services in Costa Rica. Ecol Econom. (2008) 65:712–24. doi: 10.1016/j.ecolecon.2007.07.033
36. La G. Decreto ejecutivo N° 33-106 MINAE. Poder ejecutivo de Costa Rica. Diario Oficial de Costa Rica, 133 (2006). Available online at: https://www.imprentanacional.go.cr/pub/2006/05/30/COMP_30_05_2006.html
37. SINAC-MANAE (Sistema Nacional de Áreas de Conservación). Guía práctica para el diseño, oficialización y consolidación de corredores biológicos en Costa Rica, San José (2008). p. 53.
38. McKinney T. The effects of provisioning and crop-raiding on the diet and foraging activities of human-commensal white-faced capuchins (Cebus capucinus). Am J Primatol. (2011) 73:439–48. doi: 10.1002/ajp.20919
39. Wolfe ND, Escalante AA, Karesh WB, Kilbourn A, Spielman A, Lal AA. Wild primate populations in emerging infectious disease research: the missing link? Emerg Infect Dis. (1998) 4:149–58. doi: 10.3201/eid0402.980202
40. Ekanayake DK, Rajapakse RP, Dubey JP, Dittus WP. Seroprevalence of Toxoplasma gondii in wild toque macaques (Macaca sinica) at Polonnaruwa, Sri Lanka. J Parasitol. (2004) 90:870–1. doi: 10.1645/GE-291R
41. Minae and Fonafifo. Estudio de cobertura forestal de Costa Rica 2009–2010. Cooperación Financiera entre Alemania y Costa Rica. Proyecto: Programa Forestal Huetar Norte. Contrato de Aporte Financiero N° 1999 66 268. San José (2012). p. 26.
42. Avalos G. Still searching the rich coast: Biodiversity of Costa Rica, numbers, processes, patterns, and challenges. In: Pullaiah T, editor. Global Biodiversity Volume 4: Selected countries in the Americas and Australia. Apple Academic Press: CRC (2019). p. 101–38. doi: 10.1201/9780429433634-4
43. Glander K, Fedigan LM, Fedigan L, Chapman C. Field methods for capture and measurement of three monkey species in Costa Rica. Folia Primatol. (1991) 57:70–82. doi: 10.1159/000156567
44. Varela N. Introducción al estudio de la salud de los primates neotropicales: Módulo 4. Bogotá: Asociación Colombiana de Primatología (2006). p. 1–50.
45. West G, Heard D, Caulkett N. Zoo Animal and Wildlife Immovilization and Anesthesia. Iowa: Blackwell (2007). p. 718. doi: 10.1002/9780470376478
46. Desmonts G, Remington J. Direct agglutination test for diagnosis of Toxoplasma infection: method for increasing sensitivity and specificity. J Clin Microbiol. (1980) 11:562–8. doi: 10.1128/JCM.11.6.562-568
47. Dubey JP, Desmonts G. Serological responses of equids fed Toxoplasma gondii oocysts. Equine Vet J. (1987) 19:337–9. doi: 10.1111/j.2042-3306.1987.tb01426.x
48. Hijmans RJ, Cameron SE, Parra JL, Jones PG, Jarvis A. Very high resolution interpolated climate surfaces for global land areas. Int J Climatol. (2005) 25:1965–78. doi: 10.1002/joc.12760
49. Wainwright M. The Mammals of Costa Rica, A Natural History and Field Guide. Ithaca, NY: Zona Tropical. (2007). p. 488.
50. R Core Team. R: A Language and Environment for Statistical Computing. Vienna, Austria: R Foundation for Statistical Computing. (2013). Available online at: http://www.R-project.org/
51. Pires JS, Ribeiro CT, Carvalho Filho PR, Pissinatti A, Flausino W, Lopes CWG. Infection by Toxoplasma gondii. Pesquisa Vet Brasil. (2012) 32:1041–4. doi: 10.1590/S0100-736X2012001000017
52. Valentini EJG, Caprara A, Souza SLP, Mattaraia VGM, Gennari SM, Rodrigues UP, et al. Investigação sorológica de infecção por Toxoplasma gondii em colônia de macacos da espécie Macaca mulatta. Arquivos do Instituto Biol São Paulo. (2004) 71:507–510.
53. Molina CV, Catão-Dias JL, Ferreira Neto JS, Vasconcellos SA, Gennari SM, do Valle RDR, et al. Sero-epidemiological survey for brucellosis, leptospirosis, and toxoplasmosis in free-ranging Alouatta caraya and Callithrix penicillata from Sao Paulo State, Brazil. J Med Primatol. (2014) 43:197–201. doi: 10.1111/jmp.12112
54. de Thoisy B, Vogel I, Reynes JM, Pouliquen JF, Carme B, Kazanji M, et al. Health evaluation of translocated free-ranging primates in FrenchGuiana. Am J Primatol. (2001) 54:1–16. doi: 10.1002/ajp.1008
55. Cabezón O, García-Bocanegra I, Molina-López R, Marco I, Blanco JM, Höfle U, et al. Seropositivity and risk factors associated with Toxoplasma gondii infection in wild birds from Spain. PLoS ONE. (2011) 6:e29549. doi: 10.1371/journal.pone.0029549
56. Hejlicek K, Literak I, Nezval J. Toxoplasmosis in wild mammals from the Czech Republic. J Wildlife Dis. (1997) 33:480–5. doi: 10.7589/0090-3558-33.3.480
57. Gilbert KA, Stouffer PC. Use of a ground-water source by mantled howler monkeys (Alouatta palliata). Biotropica. (1989) 21:380. doi: 10.2307/2388292
58. Almeida-Silva B, Guedes PG, Boubli JP, Strier KB. Deslocamento terrestre e o comportamento de beber em um grupo de barbados (Alouatta guariba clamitans Cabrera, 1940) em Minas Gerais, Brasil. Neotrop Primates. (2005) 13:1–3. doi: 10.1896/1413-4705.13.1.1
60. Gutiérrez C, Lücke O, Solórzano R. Integración de la Conservación con el Desarrollo en el Proyecto de Riego Arenal-Tempisque. CATIE- IUCN. Turrialba, Costa Rica. Vol I and II (1985). p. 55.
61. Campbell JC. White-Faced Capuchins (Cebus capucinus) of Cahuita National Park. Costa Rica: Human foods and Human Interactions (Master's thesis). Iowa State University, Ames, IA, United States.
62. Ruiz A, Frenkel JK, Cerdas L. Isolation of Toxoplasma from soil. J Parasitol. (1973) 59:204–6. doi: 10.2307/3278604
63. Frenkel JK, Ruiz A, Chinchilla M. Soil survival of Toxoplasma oocysts in Kansas and Costa Rica. Am J Trop Med Hyg. (1975) 24:439–43. doi: 10.4269/ajtmh.1975.24.439
64. Smith DD, Frenkel JK. Prevalence of antibodies to Toxoplasma gondii in wild mammals of Missouri and east central Kansas: biologic and ecologic considerations of trans- mission. J Wildlife Dis. (1995) 31:15–21. doi: 10.7589/0090-3558-31.1.15
65. Xiao J, Yolken RH. Strain hypothesis of Toxoplasma gondii infection on the outcome of human diseases. Acta Physiol. (2015) 213:828–45. doi: 10.1111/apha.12458
66. Rajendran C, Sub C, Dubey JP. Molecular genotyping of Toxoplasma gondii from Central and South America revealed high diversity within and between populations infection. Genet Evol. (2012) 12:359–68. doi: 10.1016/j.meegid.2011.12.010
67. Vitaliano SN, Soares HS, Minervino AHH, Santos ALQ, Werther K, Marvulo MFV, et al. Genetic characterization of Toxoplasma gondii from Brazilian wildlife revealed abundant new genotypes. Int J Parasitol. (2014) 3:276–83. doi: 10.1016/j.ijppaw.2014.09.003
68. Rêgo WMF, Costa JGL, Baraviera RCA, Pinto LV, Bessa GL, Lopes REN, et al. Genetic diversity of Toxoplasma gondii isolates obtained from free-living wild birds rescued in Southeastern Brazil. Int J Parasitol. (2018) 7:432–8. doi: 10.1016/j.ijppaw.2018.11.001
69. Vethencourt MA, Bolaños JE, Chinchilla M, Valerio I, Loaiza GM, López CM, et al. Detection and molecular characterization of three chronic strains of Toxoplasma gondii, isolated in Costa Rica. EC Microbiol. (2019) 15:846–55.
70. Dubey JP, Thulliez P, Weigel RM, Andrews CD, Lind P, Powell EC. Sensitivity and specificity of various serologic tests for detection of Toxoplasma gondii infection in naturally infected sows. Am J Vet Res. (1995) 56:1030–6.
Keywords: serology, Alouatta palliata, Ateles geoffroyi, Cebus imitator, Saimiri oerstedii, endoparasites, Latin America
Citation: Niehaus C, Spínola M, Su C, Rojas N, Rico-Chávez O, Ibarra-Cerdeña CN, Foley J, Suzán G, Gutiérrez-Espeleta GA and Chaves A (2020) Environmental factors associated With Toxoplasma gondii Exposure in Neotropical Primates of Costa Rica. Front. Vet. Sci. 7:583032. doi: 10.3389/fvets.2020.583032
Received: 14 July 2020; Accepted: 31 August 2020;
Published: 22 October 2020.
Edited by:
Anja Joachim, University of Veterinary Medicine Vienna, AustriaReviewed by:
Rodrigo Costa da Silva, University of West Paulista, BrazilChristina Strube, University of Veterinary Medicine Hannover, Germany
Copyright © 2020 Niehaus, Spínola, Su, Rojas, Rico-Chávez, Ibarra-Cerdeña, Foley, Suzán, Gutiérrez-Espeleta and Chaves. This is an open-access article distributed under the terms of the Creative Commons Attribution License (CC BY). The use, distribution or reproduction in other forums is permitted, provided the original author(s) and the copyright owner(s) are credited and that the original publication in this journal is cited, in accordance with accepted academic practice. No use, distribution or reproduction is permitted which does not comply with these terms.
*Correspondence: Andrea Chaves, YW5kcmVhY2hhdmVzLmJpb2wmI3gwMDA0MDtnbWFpbC5jb20=