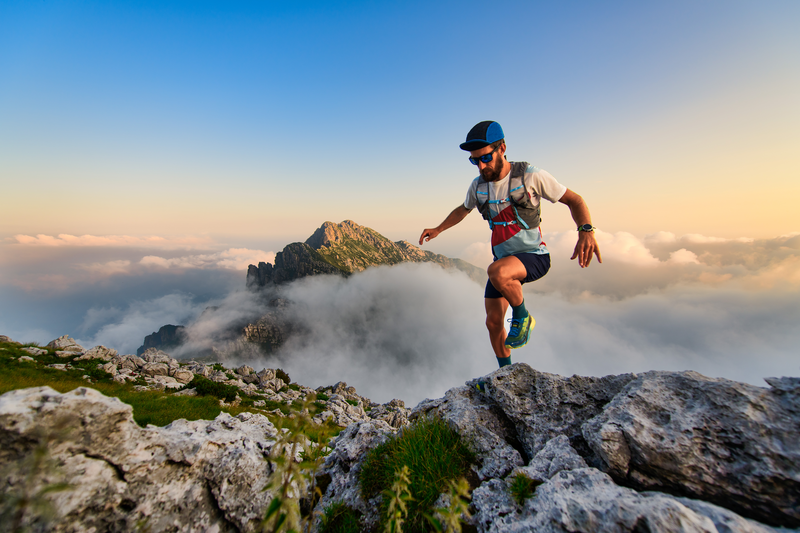
94% of researchers rate our articles as excellent or good
Learn more about the work of our research integrity team to safeguard the quality of each article we publish.
Find out more
ORIGINAL RESEARCH article
Front. Vet. Sci. , 31 July 2020
Sec. Animal Nutrition and Metabolism
Volume 7 - 2020 | https://doi.org/10.3389/fvets.2020.00418
This article is part of the Research Topic Integrated Role of Nutrition and Digestive Physiology for Animal Health View all 14 articles
This study was conducted to evaluate effects of the single and combined use of curcumin (CUR) and piperine (PIP) on performance, intestinal barrier function, and antioxidant capacity of weaned piglets. A total of 50 Wuzhishan piglets weaned at 35 days of age were randomly assigned to five groups receiving a corn–soybean basal diet (CON), the basal diet supplemented with 50 mg/kg piperine, 200 mg/kg curcumin (low-CUR), 200 mg/kg curcumin + 50 mg/kg piperine (PIP + CUR), and 300 mg/kg curcumin (high-CUR), respectively. The results showed that the feed/gain ratio (F/G) and plasma d-lactate and diamine oxidase activity (DAO) of the CUR + PIP and high-CUR groups were lower than those of the CON group (all P < 0.05), while the jejunum and ileum villus height, the villus height/crypt depth ratio, and the messenger RNA (mRNA) expression levels of occludin, claudin-1, and zonula occluden-1 in jejunal and ileal mucosa were higher in the CUR + PIP and high-CUR groups than in the CON group (all P < 0.05). Moreover, the piglets in the CUR + PIP and high-CUR groups had higher serum and intestinal mucosa activity of superoxide dismutase and glutathione peroxidase and lower malonaldehyde concentration than piglets in the CON group (all P < 0.05). The above parameters were not significantly different between the CUR + PIP and high-CUR groups (P > 0.05). In conclusion, the combination of CUR and PIP seemed to be as advantageous as high-CUR to piglets, but it was more effective than the single use of CUR and PIP. These data indicated that the basal diet supplemented with CUR + PIP or high-CUR could improve the intestinal permeability and suppress oxidative stress of weaned Wuzhishan piglets.
After weaning, piglets often suffer from intestinal barrier dysfunction, which contributes to severe diarrhea and decreased performance in piglets (1–3). Antibiotics have been widely used as animal feed additives for many years because of their efficiency in increasing the growth rate, improving feed utilization, and reducing mortality (4). However, their continuous use may lead to the emergence of drug resistance and antibiotic residues in poultry products (5), harming human health and the environment (6). As a result, some countries have banned the addition of antibiotics to livestock as growth promoters (7, 8).
In the past two decades, a large number of materials have been investigated as alternatives to antibiotics added in piglet diets (9). Among them, plant extracts are proven to be useful in relieving the post-weaning syndrome (10). Curcumin, an active natural polyphenol derived from the curry spice turmeric, has been widely used as medicine, dietary additives, and coloring agents (11). It exhibits biological activities as diverse as antioxidant (12), antiviral (13), anticancer (14), antiproliferation (15), antidiabetic (16), and anti-inflammatory properties (17). Particularly, the protective effects of CUR on the intestinal mucosa barrier were also repeatedly demonstrated in rat (18, 19), duck (20), and human intestinal epithelial cells (21, 22). Our previous experiments have also shown that a basal diet supplemented with 300 mg/kg CUR could improve the integrity and morphology of the intestinal mucosal barrier as well as the immunity of weaned pigs challenged with enterotoxigenic Escherichia coli (23).
In spite of an extensive range of pharmacological potentials, the CUR has limited bioavailability when administered orally, which can be explained by its poor absorption, low stability, and fast metabolism and excretion from the body (24, 25). To increase its bioavailability, several attempts have been made. Piperine, a bioactive alkaloid in pepper, has been suggested to inhibit the hepatic and intestinal glucuronidation and improve the bioavailability of CUR (26–28). The combined use of CUR and PIP has been reported to attenuate inflammation (29–31). The addition of PIP to CUR-containing formulations increases intestinal and plasma concentrations of CUR and thus enhances its inflammation-preventing activities (32). In this paper, we hypothesized that the combined use of CUR and PIP might be more effective than the single use of CUR and PIP in preventing diarrhea and poor performance induced by weaning in pigs.
Wuzhishan pig is a miniature pig breed originating from Hainan Island of China. In the previous study, we found that weaning at the 35th day after birth was more beneficial to the intestinal barrier function of Wuzhishan piglets than weaning at the 21st day after birth (33). Therefore, the purpose of this study was to determine the effects of single and combined use of CUR and PIP on growth performance, intestinal barrier function, and antioxidant capacity of 35-day-old weaned Wuzhishan piglets.
Curcumin (90.0%) was purchased from Shi jiazhuang Lv Chuan Bio Technology Co., Ltd (Hebei, China). Piperine was purchased from Shanghai Macklin Biochemical Co., Ltd. (Shanghai China). The determination kits for antioxidant indices, including superoxide dismutase (SOD), glutathione peroxidase (GSH-Px), total antioxidant capacity (T-AOC) and malonaldehyde (MDA), plasma D-lactate, and diamine oxidase (DAO) were all purchased from the Jiancheng Bioengineering Institute of Nanjing (Jiangsu, China). RNAiso Plus and PrimeScript™ RT Reagent Kit were purchased from TakaRa Biotechnology Inc. (Dalian, China).
A total of 50 Wuzhishan piglets weaned at 35 days of age were randomly allocated to one of five treatments (n = 10) for 21 days, with the initial average body weight of 3.54 ± 0.28 kg. The five treatments were CON, CON + 50 mg/kg PIP, CON + 200 mg/kg curcumin (low-CUR), CON + 200 mg/kg curcumin + 50 mg/kg piperine (PIP + CUR), and CON + 300 mg/kg curcumin (high-CUR). Diets were formulated to meet the nutrient requirements suggested by the Wuzhishan pig breeding technology discipline (Table 1). All the piglets were housed in individual pens with room temperature maintained at 25–27°C.
During the 21-day experiment period, piglets were allowed ad libitum to designated diet and water. The piglets were individually weighed at the beginning and end of the trial (days 0 and 21), and feed consumption for each pig was recorded daily. Average daily gain (ADG), average daily feed intake (ADFI), and F/G ratio were calculated.
On day 21, six piglets subjected to each treatment were randomly selected, and a 5-ml blood sample was harvested from the jugular vein of each piglet into a tube with anticoagulant or without anticoagulant. After 20 min standing at room temperature, plasma or serum was obtained, which were centrifuged at 3,000 g for 15 min at 4°C and then stored at −20°C for assays. Plasma samples were used for D-lactate and DAO detection, and serum samples were used for the analysis of antioxidant variables.
After blood sampling, the piglets were sacrificed by injection of sodium pentobarbital solution (50 mg/kg of body weight). The middle sections of the jejunum and ileum were isolated aseptically, flushed with physiological saline, and fixed in 4% paraformaldehyde for 24 h for subsequent histological assays. After mucosa samples from the jejunum and ileum were scraped with a razor, they were frozen in liquid nitrogen immediately and stored at −80°C for further assays.
The levels of D-lactate and DAO in the plasma were detected by using porcine D-lactic acid ELISA kit and DAO assay kit, respectively, according to the manufacturer's instructions (Jiancheng Bioengineering Institute of Nanjing, Nanjing, China).
The intestinal mucosa morphology including the villus height, villus width, and crypt depth were measured as previously described (23). Briefly, the paraformaldehyde-fixed tissue was embedded in paraffin according to standard procedures. Five-micrometer-thick sections were installed on glass slides. After deparaffinization, the slides were stained with hematoxylin and eosin. The villus height, villus width, and crypt depth were measured by using the Axioskop-2 microscope (Olympus) and image processing system (Version 1, Leica Imaging Systems Ltd). Ten well-oriented and intact crypt–villus units of each intestinal cross-section were selected for measurements. The villus height/crypt depth ratio was calculated.
Relative messenger RNA (mRNA) abundance of occludin, ZO-1, claudin-1, interleukin 1β (IL-1β), tumor necrosis factor a (TNF-α), interleukin 6 (IL-6), and interleukin 10 (IL-10) in jejunal and ileal mucosa was determined by real-time PCR as described previously by Wan (34). Briefly, total RNA was extracted using RNAiso Plus (Takara, China) following the manufacturer's guidelines. The RNA samples were reversely transcribed into complementary DNA using RT Reagents (TaKaRa, China) according to the manufacturer's instructions. The primers are listed in Table 2. Quantitative real-time RT-PCR was performed on a PIKO-RT 96 Real-Time PCR Detection System (Thermo Fisher Scientific, America) using SYBR Premix Ex Taq reagent (TaKaRa, China) according to the kit's instructions. Glyceraldehyde-3-phosphate dehydrogenase (GAPDH) was chosen as the reference gene transcript to correct the variances in target gene transcript levels. The reaction was performed in a 10-μl system containing 5 μ SYBR® Premix Ex TaqTMII (Tli RNaseH Plus, 2 ×), 1 μl RT products, 2 μl double distilled water (ddH2O), and 1 μl each of forward and reverse primers at the conditions of 95°C for 7 s followed by 40 cycles of 95°C for 5 s and 60°C for 30 s and a final dissociation step from 60 to 95°C at a heating rate of 0.2°C/s. The 2−ΔΔCT method was used to analyze the relative quantification of gene expression (fold changes), calculated relative to the control group as previously described (23).
Equal amounts of jejunum and ileum mucosa from the same piglet were blended to form a single sample before testing. Antioxidant indexes, including SOD, GSH-Px, T-AOC, and MDA in serum and small intestine mucosa samples were determined using assay kits according to the manufacturer's instructions (Nanjing Jiancheng Bioengineering Institute, Nanjing, China).
Data were analyzed mean by one-way analysis of variance (ANOVA) using SPSS for windows version 18.0 (SPSS, Inc., Chicago, IL, USA). Differences among treatments were detected by Duncan's multiple range tests. The data are presented as mean, and standard error of the mean (SEM) was given. The P-value for significance was set at P < 0.05.
The results of growth performance are given in Table 3. Supplementation of the basal diet with CUR + PIP or high-CUR had no effect on the initial weight, final weight, ADFI, and ADG (P > 0.05), but the F/G ratio of pigs in the CUR + PIP and high-CUR groups was lower than that in the CON, PIP, and low-CUR groups (P < 0.05).
Table 3. Effect of curcumin (CUR) and piperine (PIP) on growth performance of weaned Wuzhishan piglets.
The data for intestinal morphology of piglets are shown in Table 4. The weaned piglets fed with CUR + PIP or high-CUR had significantly higher villus height and villus height/crypt depth ratio in jejunal and ileum mucosa than the CON and PIP pigs (P < 0.05). The villus height/crypt depth ratio in jejunal and ileum mucosa of pigs in the low-CUR group was higher than that in the CON group (P < 0.05), but the villus height was not significantly different between the two groups (P > 0.05). The crypt depth and villus width were not significantly different among the five treatments (P > 0.05).
Table 4. Effect of curcumin (CUR) and piperine (PIP) on intestinal mucosa morphology of weaned Wuzhishan piglets.
The plasma D-lactate and DAO results are shown in Table 5. Compared with the control group, the plasma D-lactate and DAO in CUR + PIP and high-CUR groups were significantly lower (P < 0.05). Supplementation of PIP significantly reduced plasma DAO activities (P < 0.05), but plasma D-lactate was not affected (P > 0.05). Moreover, the plasma D-lactate and DAO were significantly different between low-CUR and control groups (P > 0.05).
Table 5. Effect of curcumin (CUR) and piperine (PIP) on plasma d-lactate and diamine oxidase activity (DAO) in weaned Wuzhishan piglets.
Figure 1 shows the mRNA expression of occludin, claudin-1, and ZO-1 in the jejunal and ileum mucosa of piglets. The expression levels of occludin, claudin-1, and ZO-1 in the jejunal and ileum mucosa of piglets in the CUR + PIP and high-CUR groups were significantly higher than those in the control, PIP, and low-CUR groups (P < 0.05). However, there were no significant differences in the expression of above substances between the PIP and low-CUR groups (P > 0.05).
Figure 1. Effect of curcumin (CUR) and piperine (PIP) on messenger RNA (mRNA) expression of tight junction protein in (A) jejunal mucosa and (B) ileal mucosa of weaned Wuzhishan piglets. Values are expressed as mean ± standard deviations (n = 6). abMeans values within different letters differ significantly (P < 0.05). CON, a corn–soybean basal diet; PIP, low-CUR, PIP + CUR, and high-CUR, the basal diet supplemented with 50 mg/kg piperine, 200 mg/kg curcumin, 200 mg/kg curcumin + 50 mg/kg piperine, and 300 mg/kg curcumin, respectively.
Figure 2 shows the mRNA expression of cytokines in the jejunal and ileum mucosa of piglets. The mRNA levels of IL-1β, TNF-α, IL-6, and IL-10 were not significantly different among the five treatment groups (P > 0.05).
Figure 2. Effect of curcumin (CUR) and piperine (PIP) on messenger RNA (mRNA) expression of cytokines in (A) jejunal and (B) ileal mucosa of weaned Wuzhishan piglets. Values are means (n = 6), and standard deviations represented by vertical bars. CON, a corn–soybean basal diet; PIP, low-CUR, PIP + CUR, and high-CUR, the basal diet supplemented with 50 mg/kg piperine, 200 mg/kg curcumin, 200 mg/kg curcumin + 50 mg/kg piperine, and 300 mg/kg curcumin, respectively. Dietary Rice Improves Growth Performance, Mucosal Enzyme Activities, and Plasma Urea Nitrogen in Weaning Piglets. Effects of Dietary Methionine Supplementation on Growth Performance, Intestinal Morphology, Antioxidant Capacity, and Immune Function in Intra-Uterine Growth-Retarded Suckling Piglets. Comparative Effects of Dietary Supplementations With Sodium Butyrate, Medium-Chain Fatty Acids, and n-3 Polyunsaturated Fatty Acids in Late Pregnancy, and Lactation on the Reproductive Performance of Sows and Growth Performance of Suckling Piglets. Effects of Composite Antimicrobial Peptide on Growth Performance and Health in Weaned Piglets.
As shown in Table 6, pigs in CUR + PIP and high-CUR groups had higher SOD and GSH-Px activities as well as lower MDA concentration in serum and intestinal mucosa than pigs in the CON group (P < 0.05). The PIP piglets had higher GSH-Px activity and lower MDA concentration in the serum and intestinal mucosa than the CON piglets (P < 0.05). The value of T-AOC in serum and intestinal mucosa was not significantly different among the five treatments (P < 0.05).
Table 6. Effect of curcumin (CUR) and piperine (PIP) on antioxidant variables in serum and intestinal mucosa of weaned Wuzhishan piglets.
In recent years, there have been several attempts to demonstrate the use of CUR as a potential feed additive that can replace antimicrobial growth promoters. Diets supplied with 50 and 100 mg/kg CUR increased the growth performance of broilers by improving the antioxidant defense system and enhancing the mitochondrial biogenesis. Ruan et al. (20) found that CUR prevented the decrease in body weight and ADG in ducks fed with corn contaminated by ochratoxin A. Ilsley et al. (35) reported that dietary supplementation with 200 mg/kg CUR had no influence on pig growth performance. This finding was in line with that of our previous study, which suggested that no growth improvement was observed in weaned pigs fed with 200 mg/kg CUR-added diet (23). In the present experiment, supplementation of CUR + PIP or high-CUR significantly improved the growth performance of pigs by reducing F/G, indicating that high-CUR or CUR + PIP had better performance-promoting effects than PIP or low-CUR added alone in piglet diets.
As common indicators for estimating intestinal integrity, the villus height, crypt depth, villus width, and the villus height/crypt depth ratio can reveal some information on gut health in pigs. Increasing the villus height suggest an increased surface area for nutrient absorption (36). The villus crypt is considered as villus factory. The increase in crypt depth indicates fast tissue turnover and high demand for new tissue, which are generally associated with decline in nutrient digestion and absorption capacity (37, 38). Studies have confirmed that weaning is associated with villus atrophy and crypt hyperplasia (3, 39). In the present study, the increase in villus height and villus height/crypt depth ratio in jejunal and ileum mucosa caused by the addition of CUR + PIP or high-CUR was observed. Similar results were achieved in our previous study, which noticed that the addition of 400 mg/kg CUR in the diet increased the villus height and villus height/crypt depth ratio in piglets, demonstrating that both the supplementation with CUR + PIP and the addition of high-CUR could ameliorate the weaning-associated damage to small intestinal morphology, thus correspondingly improving the digestion and absorption of nutrients and promoting the growth performance (23).
The integrity of intestinal mucosa barrier is the basis for the normal function of epithelial cells and defense against the pathogenic bacteria (3). Plasma DAO and D-lactate are used as sensitive circulating indicators of the severity of mucosal injury (40, 41). DAO exists only in the villi of the upper small intestine, and a small amount is normally present in the blood. When the intestinal mucosal function is injured, mucosal permeability increases, promoting the release of more endocellular DAO into the blood (42). Therefore, plasma DAO reflects the integrity of intestinal mucosa. D-Lactate is the end product of intestinal bacterial fermentation. When the intestinal mucosal function is impaired, the D-lactate concentration in blood is increased, which is because D-lactate in mammals cannot be metabolized due to the lack of enzyme systems (43). Studies have shown that early weaning leads to impaired mucosal barrier function and increased intestinal permeability (2, 44). The present results showed that dietary supplementation of CUR + PIP or high-CUR improved intestinal barrier function by reducing plasma DAO and D-lactate. The data were supported by our previous studies, which revealed that dietary addition of 300 or 400 mg/kg CUR reduced plasma DAO and D-lactate in weaned piglets challenged with enterotoxigenic E. coli (23).
Tight junction proteins (occludin, claudin-1, and ZO-1) play an important role in the maintenance of intestinal mucosal barrier integrity. They function as the continuous intercellular barrier against the translocation of intestinal bacteria, antigens, and intraluminal toxins from the lumen into subepithelial tissue and systemic blood circulation (45). It has been established by several studies that CUR promotes the expression of tight junction proteins in intestinal mucosa. Tian et al. (46) found that CUR significantly upregulated the expression of ZO-1 following the intestinal ischemia–reperfusion injury in rats, which might be partly attributed to the TNF-α related pathway. The study of Ruan et al. (20) implied that CUR increased jejunal mucosa occludin and ZO-1 mRNA and protein levels in ducks. In the present study, the upregulation of occludin, claudin-1, and ZO-1 mRNA expression due to the supplementation with CUR + PIP or high-CUR suggested that both treatments might improve the intestinal integrity. The results were consistent with the improved intestinal morphology and decrease in the plasma D-lactate and DAO levels. The molecular mechanism of CUR + PIP in regulation of tight junctions requires further study.
In addition to intestinal integrity, weaning-associated intestinal inflammation was also observed in weaning piglets (39, 47). Weaning causes the upregulation of proinflammatory cytokines, such as TNF-α, IFN-γ, IL-1β, and IL-6. Overproduction of proinflammatory cytokines induces a pathological opening of the intestinal tight junctions and increases intestinal epithelial permeability, resulting in intestinal barrier dysfunction (48, 49). Song et al. (19) noticed that CUR decreased the mRNA expression of IL-1β and TNF-a and increased the mRNA expression of IL-10 in intestinal mucosa of rats and IEC-6 cells. Ruan et al. (20) also observed that CUR decreased the concentrations of TNF-α and IL-1β induced by OTA in jejunal mucosa of ducks. In our previous studies, the mRNA levels of TNF-α, IL-6, and IL-1β were decreased by supplementation with 400 mg/kg CUR in weaned piglets challenged with enterotoxigenic E. coli. However, in the present experiment, the mRNA expression levels of IL-1β, TNF-α, IL-6, and IL-10 were not affected by dietary supplementation. The present study results demonstrated that the transiently upregulated inflammatory cytokines induced by weaning could rapidly return to the preweaning level 9 days after weaning, which might be the reason why dietary CUR supplementation did not affect mRNA expression of inflammatory cytokines 21 days after weaning.
Studies have confirmed that weaning induces oxidative stress and increases free radicals in tissue and blood. Antioxidants can eliminate free radicals and reduce oxidative stress. CUR has the potential in vivo antioxidant activity owing to its ability to scavenge reactive oxygen species (50, 51) and inhibit lipid peroxidation (52). The gastrointestinal tract can remove free radicals and prevent oxidative damage, mainly serving as antioxidant in the human body. Antioxidant enzymes include GSH-Px, SOD, CAT, and MDA (52). MDA is the end product of lipoperoxidation, and the levels of MDA in plasma and tissue are an excellent oxidative stress marker (53). In our study, both CUR + PIP and high-CUR increased the activities of serum and intestinal mucosa antioxidant enzymes (e.g., GSH-Px and SOD) and decreased the lipid peroxidation marker MDA, indicating that the oxidative stress was reduced by dietary CUR or CUR + PIP supplementation. This result was similar with that of the study of Arcaro et al. (54) who reported that the level of MDA was markedly reduced in the plasma of diabetic rats fed with 90 mg/kg curcumin or 90 mg/kg CUR + 20 mg/kg PIP. Oxidative stress caused by weaning is responsible for intestinal mucosal injury (55). Reduced oxidative stress might alleviate the damage of intestinal mucosal barrier, and high-CUR or CUR + PIP supplementation decreased the intestinal permeability subsequently.
In summary, the present study showed that supplementation with PIP + CUR or high-CUR could reduce intestinal permeability, enhance antioxidant capacity, and had beneficial effects on feed utilization rate of the corn–soybean basal diet. The addition of both CUR and PIP appeared to be as advantageous as high-CUR, but it was more effective than low-CUR or PIP alone.
All datasets presented in this study are included in the article/supplementary material.
The animal study was reviewed and approved by Committee on laboratory animal ethics of Tropical Crops Genetic Resources Institute (TCGRI). Written informed consent was obtained from the owners for the participation of their animals in this study.
LS and WX designed the experiments. TC, WP, and HH carried out the feeding experiments. GH analyzed the experimental results. All authors contributed to the article and approved the submitted version.
This study was supported by Central Public-interest Scientific Institution Basal Research Fund for Chinese Academy of Tropical Agricultural Sciences (Nos. 1630032019023 and 1630032017035), Project of Quality and Safety Evaluation of Tropical Feed Resources (2130109), and Natural Science Foundation of Hainan Province (No. 319QN307).
The authors declare that the research was conducted in the absence of any commercial or financial relationships that could be construed as a potential conflict of interest.
The authors also thank the staff of the Key Laboratory of Crop Gene Resources and Germplasm Enhancement in Southern China, Chinese Academy of Tropical Agricultural Sciences. All authors read and approved the final manuscript.
1. Jiao LF, Song ZH, Ke YL, Xiao K, Hu CH, Shi B. Cello-oligosaccharide influences intestinal microflora, mucosal architecture and nutrient transport in weaned pigs. Anim Feed Sci Technol. (2014) 195:85–91. doi: 10.1016/j.anifeedsci.2014.05.014
2. Kim JC, Hansen CF, Mullan BP, Pluske JR. Nutrition and pathology of weaner pigs: nutritional strategies to support barrier function in the gastrointestinal tract. Anim Feed Sci Technol. (2012) 173:3–16. doi: 10.1016/j.anifeedsci.2011.12.022
3. Wijtten PJ, van der Meulen J, Verstegen MW. Intestinal barrier function and absorption in pigs after weaning: a review. Br J Nutr. (2011) 105:967–81. doi: 10.1017/S0007114510005660
4. Vondruskova H, Slamova R, Trckova M, Zraly Z, Pavlik I. Alternatives to antibiotic growth promotersin prevention of diarrhoea in weaned piglets: a review. Vet Med. (2010) 55:199–224. doi: 10.17221/2998-VETMED
5. Schwarz S, Kehrenberg C, Walsh TR. Use of antimicrobial agents in veterinary medicine and food animal production, Int J Antimicrob Agents. (2001) 17:431–7. doi: 10.1016/S0924-8579(01)00297-7
6. Kemper N. Veterinary antibiotics in the aquatic and terrestrial environment. Ecol Indic. (2008) 8:1–13. doi: 10.1016/j.ecolind.2007.06.002
7. Schmidt CW. FDA proposes to ban cephalosporins from livestock feed. Environ Health Perspect. (2012) 120:A106. doi: 10.1289/ehp.120-a106
8. Vidovic N, Vidovic S. Antimicrobial resistance and food animals: influence of livestock environment on the emergence and dissemination of antimicrobial resistance. Antibiotics. (2020) 9:52. doi: 10.3390/antibiotics9020052
9. Thacker PA. Alternatives to antibiotics as growth promoters for use in swine production: a review. J Anim Sci Biotechnol. (2013) 4:35. doi: 10.1186/2049-1891-4-35
10. Windisch W, Schedle K, Plitzner C, Kroismayr A. Use of phytogenic products as feed additives for swine and poultry. J Anim Sci. (2008) 86:140–8. doi: 10.2527/jas.2007-0459
11. Strimpakos AS, Sharma RA. Curcumin: preventive and therapeutic properties in laboratory studies and clinical trials. Antioxid Redox Signal. (2008) 10:511–45. doi: 10.1089/ars.2007.1769
12. Glauert HP, Calfee-Mason K, Stemm DN, Tharappel JC, Spear BT. Dietary antioxidants in the prevention of hepatocarcinogenesis: a review. Mol Nutr Food Res. (2010) 54:875–96. doi: 10.1002/mnfr.200900482
13. Rechtman MM, Har-Noy O, Bar-Yishay I, Fishman S, Adamovich Y, Shaul Y, et al. Curcumin inhibits hepatitis B virus via downregulation of the metabolic coactivator PGC-1α. FEBS Lett. (2010) 584:2485–90. doi: 10.1016/j.febslet.2010.04.067
14. Bar-Sela G, Epelbaum R, Schanffer M. Curcumin as an anti-cancer agent: review of the gap betweebasic and clinical applications. Curr Med Chem. (2010) 17:190–7. doi: 10.2174/092986710790149738
15. Bachmeier BE, Killian P, Pfeffer U, Nerlich AG. Novel aspects for the application of Curcumin in chemoprevention of various cancers. Front Biosci. (2010) 2:697–717. doi: 10.2741/s95
16. Pari L, Murugan P. Effect of tetrahydrocurcumin on blood glucose, plasma insulin and hepatic key enzymes in streptozotocin induced diabetic rats. J Basic Clin Physiol Pharmacol. (2005) 16:257–74. doi: 10.1515/JBCPP.2005.16.4.257
17. Sarker MR, Franks SF. Efficacy of curcumin for age-associated cognitive decline: a narrative review of preclinical and clinical studies. GeroScience. (2018) 40:73–95. doi: 10.1007/s11357-018-0017-z
18. Hou HT, Qiu YM, Zhao HW, Li H, Liu YT, Wang YZ, et al. Effect of curcumin on intestinal mucosal mechanical barrier in rats with non-alcoholic fatty liver disease. Zhonghua Gan Zang Bing Za Zhi. (2017) 25:134–8. doi: 10.3760/cma.j.issn.1007-3418.2017.02.011
19. Song WB, Wang YY, Meng FS, Zhang QH, Zeng J, Xiao LP, et al. Curcumin protects intestinal mucosal barrier function of rat enteritis via activation of MKP-1 and attenuation of p38 and NF-κB activation. PLoS ONE. (2010) 5:e12969. doi: 10.1371/journal.pone.0012969
20. Ruan D, Wang WC, Lin CX, Fouad AM, Chen W, Xia WG, et al. Effects of curcumin on performance, antioxidation, intestinal barrier and mitochondrial function in ducks fed corn contaminated with ochratoxin A. Animal. (2018) 12:1–11. doi: 10.1017/S1751731118000678
21. Wang N, Wang G, Hao J, Ma J, Wang Y, Jiang X, et al. Curcumin ameliorates hydrogen peroxide-induced epithelial barrier disruption by upregulating heme oxygenase-1 expression in human intestinal epithelial cells. Dig Dis Sci. (2012) 57:1792–801. doi: 10.1007/s10620-012-2094-7
22. Wang J, Ghosh SS, Ghosh S. Curcumin improves intestinal barrier function: modulation of intracellular signaling, and organization of tight junctions. Am J Physiol Cell Physiol. (2017) 312:C438–45. doi: 10.1152/ajpcell.00235.2016
23. Xun WJ, Shi LG, Zhou HL, Hou GY, Cao T, Zhao C. Effects of curcumin on growth performance, jejunal mucosal membrane integrity, morphology and immune status in weaned piglets challenged with enterotoxigenic Escherichia coli. Int Immunopharmacol. (2015) 27:46–52. doi: 10.1016/j.intimp.2015.04.038
24. Lopresti AL. The problem of curcumin and its bioavailability: could its gastrointestinal influence contribute to its overall health-enhancing effects? Adv Nutr. (2018) 9:41–50. doi: 10.1093/advances/nmx011
25. Mahmood K, Zia KM, Zuber M, Salman M, Anjum MN, Recent developments in curcumin and curcumin based polymeric materials for biomedical applications: a review. Int J Biol Macromol. (2015) 81:877–90. doi: 10.1016/j.ijbiomac.2015.09.026
26. Jangra A, Kwatra M, Singh T, Pant R, Kushwah P, Sharma Y, et al. Piperine augments the protective effect of curcumin against lipopolysaccharide-induced neurobehavioral and neurochemical deficits in Mice. Inflammation. (2016) 39:1025–38. doi: 10.1007/s10753-016-0332-4
27. Rinwa P, Kuma A. Piperine potentiates the protective effects of curcumin against chronic unpredictable stress-induced cognitive impairment and oxidative damage in mice. Brain Res. (2012) 1488:38–50. doi: 10.1016/j.brainres.2012.10.002
28. Singh S, Jamwal S, Kumar P. Piperine enhances theprotective effect of curcumin against 3-NP induced neurotoxicity: possible neurotransmitters modulation mechanism. Neurochem Res. (2015) 40:1758–66. doi: 10.1007/s11064-015-1658-2
29. Miyazawa T, Nakagawa K, Kim SH, Thomas MJ, Paul L, Zingg JM, et al. Curcumin and piperine supplementation of obese mice under caloric restriction modulates body fat and interleukin-1β. Nutr Metab. (2018) 15:12. doi: 10.1186/s12986-018-0250-6
30. Neyrinck AM, Alligier M, Memvanga PB, Névraumont E, Larondelle Y, Préat V, et al. Curcuma Longa extract associated with white pepper lessens high fat diet-induced inflammation in subcutaneous adipose tissue. PLoS ONE. (2013) 8:e81252. doi: 10.1371/journal.pone.0081252
31. Panahi Y, Hosseini MS, Khalili N, Naimi E, Majeed M, Sahebkar A. Antioxidant and anti-inflammatory effects of curcuminoid-piperine combination in subjects with metabolic syndrome: a randomized controlled trial and an updated meta-analysis. Clin Nutr. (2015) 34:1101–8. doi: 10.1016/j.clnu.2014.12.019
32. Shoba G, Joy D, Joseph T, Majeed M, Rajendran R, Srinivas PS. Influence of piperine on the pharmacokinetics of curcumin in animals and human volunteers. Planta Med. (1998) 64:353–6. doi: 10.1055/s-2006-957450
33. Xun WJ, Shi LG, Zhou HL, Hou GY, Cao T. Effect of weaning age on intestinal mucosal morphology, permeability, gene expression of tight junction proteins, cytokines and secretory IgA in Wuzhishan mini piglets. Ital J Anim Sci. (2018) 17:976–83. doi: 10.1080/1828051X.2018.1426397
34. Wan J, Li Y, Chen DW, Yu B, Chen G, Zheng P, et al. Recombinant plectasin elicits similar improvements in the performance and intestinal mucosa growth and activity in weaned pigs as an antibiotic. Anim Feed Sci Technol. (2016) 211:216–26. doi: 10.1016/j.anifeedsci.2015.12.003
35. Ilsley SE, Miller HM, Kamel C. Effects of dietary quillaja saponin and curcumin on the performance and immune status of weaned piglets. J Anim Sci. (2005) 83:82–8. doi: 10.2527/2005.83182x
36. Caspary WF. Physiology and pathophysiology of intestinal absorption. Am J Clin Nutr. (1992) 55:299–308. doi: 10.1093/ajcn/55.1.299s
37. Ke YL, Jiao LF, Song ZH, Xiao K, Lai TM, Lu JJ, et al. Effects of cetylpyridinium-montmorillonite, as alternative toantibiotic, on the growth performance, intestinal microfloraand mucosal architecture of weaned pigs. Anim Feed Sci Technol. (2014) 198:257–62. doi: 10.1016/j.anifeedsci.2014.10.010
38. Montagne L, Boudry G, Favier C, Le Huërou-Luron I, Lallès JP, Sève B. Main intestinal markers associated with the changes in gut architecture and function in piglets after weaning. Br J Nutr. (2007) 97:45–57. doi: 10.1017/S000711450720580X
39. Hu CH, Xiao K, Luan ZS, Song J. Early weaning increases intestinal permeability, alters expression of cytokine and tight junction proteins, and activates mitogen-activated protein kinases in pigs. J Anim Sci. (2013) 91:1094–101. doi: 10.2527/jas.2012-5796
40. Fukudome I, Kobayashi M, Dabanaka K, Maeda H, Okamoto K, Okabayashi T, et al. Diamineoxidase as a marker of intestinal mucosal injury and the effect of soluble dietary fiber on gastrointestinal tract toxicity after intravenous 5-fluorouraciltreatment in rats. Med Mol Morphol. (2014) 47:100–7. doi: 10.1007/s00795-013-0055-7
41. Hu CH, Gu LY, Luan ZS, Song J, Zhu K. Effects of montmorillonite-zinc oxide hybrid on performance, diarrhea, intestinal permeability and morphology of weanling pigs. Anim Feed Sci Technol. (2012) 177:108–15. doi: 10.1016/j.anifeedsci.2012.07.028
42. Wolvekamp MC, de Bruin RW. Diamine oxidase: an overview of historical, biochemical and functional aspects. Dig Dis. (1994) 12:2–14. doi: 10.1159/000171432
43. Brandt RB, Siegel SA, Waters MG, Bloch MH. Spectrophotometric assay for d-(–)-lactate in plasma. Anal Biochem. (1980) 102:39–46. doi: 10.1016/0003-2697(80)90314-0
44. Smith F, Clark JE, Overman BL, Tozel CC, Huang JH, Rivier JE, et al. Early weaning stress impairs development of mucosal barrier function in the porcine intestine. Am J Physiol Gastrointest Liver Physiol. (2010) 298:352–63. doi: 10.1152/ajpgi.00081.2009
45. Ulluwishewa D, Anderson RC, McNabb WC, Moughan PJ, Wells JM, Roy NC. Regulation of tight junction permeability by intestinal bacteria and dietary components. J Nutr. (2011) 141:769–76. doi: 10.3945/jn.110.135657
46. Tian S, Guo R, Wei S, Kong Y, Wei X, Wang W, et al. Curcumin protects against the intestinal ischemia-reperfusion injury: involvement of the tight junction protein ZO-1 and TNF-α related mechanism. Korean J Physiol Pharmacol. (2016) 20:147–52. doi: 10.4196/kjpp.2016.20.2.147
47. Pié S, Lallès JP, Blazy F, Laffitte J, Sève B, Oswald IP. Weaning is associated with an upregulation of expression of inflammatory cytokines in the intestine of piglets. J Nutr. (2004) 134:641–7. doi: 10.1093/jn/134.3.641
48. Al-Sadi R, Boivin M, Ma T. Mechanism of cytokine modulation of epithelial tight junction barrier. Front Biosci. (2009) 14:2765–78. doi: 10.2741/3413
49. Moeser AJ, Ryan KA, Nighot PK, Blikslager AT. Gastrointestinal dysfunction induced by early weaning is attenuated by delayed weaning and mast cell blockade in pigs. Am J Physiol Gastrointest Liver Physiol. (2007) 293:413–21. doi: 10.1152/ajpgi.00304.2006
50. Ak T, Gülçin I. Antioxidant and radical scavenging properties of curcumin. Chem Biol Interact. (2008) 174:27–37. doi: 10.1016/j.cbi.2008.05.003
51. Barzegar A, Moosavi-Movahedi AA. Intracellular ROS protection efficiency and free radical-scavenging activity of curcumin. PLoS ONE. (2011) 6:e26012. doi: 10.1371/journal.pone.0026012
52. Meghana K, Sanjeev G, Ramesh B. Curcumin prevents streptozotocin-induced islet damage by scavenging free radicals: a prophylactic and protective role. Eur J Pharmacol. (2007) 577:183–91. doi: 10.1016/j.ejphar.2007.09.002
53. Yousef MI, Saad AA, El-Shennawy LK. Protective effect of grape seed proanthocyanidin extract against oxidative stress induced by cisplatin in rats. Food Chem Toxicol. (2009) 47:1176–83. doi: 10.1016/j.fct.2009.02.007
54. Arcaro CA, Gutierres VO, Assis RP, Moreira TF, Costa PI, Baviera AM, et al. Piperine, a natural bioenhancer, nullifies the antidiabetic and antioxidant activities of curcumin in streptozotocin-diabetic rats. PLoS ONE. (2014) 9:e113993. doi: 10.1371/journal.pone.0113993
Keywords: curcumin, piperine, growth performance, intestinal permeability, weaned piglet, antioxidant capacity
Citation: Shi L, Xun W, Peng W, Hu H, Cao T and Hou G (2020) Effect of the Single and Combined Use of Curcumin and Piperine on Growth Performance, Intestinal Barrier Function, and Antioxidant Capacity of Weaned Wuzhishan Piglets. Front. Vet. Sci. 7:418. doi: 10.3389/fvets.2020.00418
Received: 23 April 2020; Accepted: 11 June 2020;
Published: 31 July 2020.
Edited by:
Haoyu Liu, Uppsala University, SwedenReviewed by:
Xihong Zhou, Chinese Academy of Sciences, ChinaCopyright © 2020 Shi, Xun, Peng, Hu, Cao and Hou. This is an open-access article distributed under the terms of the Creative Commons Attribution License (CC BY). The use, distribution or reproduction in other forums is permitted, provided the original author(s) and the copyright owner(s) are credited and that the original publication in this journal is cited, in accordance with accepted academic practice. No use, distribution or reproduction is permitted which does not comply with these terms.
*Correspondence: Guanyu Hou, Z3Vhbnl1aG91QDEyNi5jb20=
Disclaimer: All claims expressed in this article are solely those of the authors and do not necessarily represent those of their affiliated organizations, or those of the publisher, the editors and the reviewers. Any product that may be evaluated in this article or claim that may be made by its manufacturer is not guaranteed or endorsed by the publisher.
Research integrity at Frontiers
Learn more about the work of our research integrity team to safeguard the quality of each article we publish.