- 1Department of Veterinary Surgery, Northeast Agricultural University, Harbin, China
- 2Heilongjiang Key Laboratory for Laboratory Animals and Comparative Medicine, Harbin, China
Bovine laminitis causes substantial economic losses and animal welfare problems in dairy farms worldwide. Previously published studies have reported that the inflammatory response plays a central role in the pathogenesis of the disease. To our knowledge, inflammation associated with bovine laminitis induced by high levels of exposure to oligofructose (OF) has not been reported and characterized. In fact, the disease manifestations in this model closely approximate those of clinical laminitis. The objective of this study was to characterize the inflammatory response in OF-induced bovine laminitis. A total of 12 Chinese Holstein dairy heifers were utilized in this study. The heifers were randomly divided into two groups, treatment (n = 6) and control (n = 6). The treatment group heifers were administered OF solutions via a stomach tube (dose: 17 g/kg of body weight). Upon development of a lameness score of 2 with consecutive positive reactions in the same claw, they would be humanely euthanized. Control heifers were administered deionized water (dose: 2 L/100 kg of body weight) and humanely euthanized at 72 h. Real-time quantitative PCR (qPCR) assays were performed to determine the messenger RNA (mRNA) concentrations of inflammatory mediators in the lamellae. Concentrations of interleukin (IL)-1β, IL-6, IL-8, C-X-C motif chemokine ligand-1 (CXCL-1), macrophage cationic peptide-2 (MCP-2), E-selectin, intercellular adhesion molecule-1 (ICAM-1), cyclooxygenase-2 (COX-2), inducible nitric oxide synthase-1 (iNOS-1), and plasminogen activator inhibitor-1 (PAI-1) were significantly increased (P < 0.05) in the treatment group. No significant difference was found for tumor necrosis factor alpha (TNF-α), IL-10, CXCL-6, and MCP-1. These results demonstrated and characterized the laminar inflammatory response leading to the pathogenesis of bovine laminitis at the early stages.
Introduction
Lameness causes substantial economic losses and animal welfare problems in dairy farms worldwide (1, 2). Bovine laminitis is a disease of the foot that results in lameness (3). Afflicted animals present with sole ulcers, sole hemorrhages, and white line disease in dairy cows (4). The prevalence of these lesions is closely related to breeding, management, and environmental factors (5). In recent years, several studies focused on epidemiology (6), pathophysiology (7), histology (8, 9), and proteomics (10) of disease states. However, due to the inconsistencies in clinical cases and the lack of reproducible experimental models, the exact pathogenesis of bovine laminitis remains unclear (11).
In clinical practice, bovine laminitis typically follows systemic inflammatory diseases, including septic pleuropneumonia, metritis, and ruminal acidosis (12, 13). The oligofructose (OF) overload model most closely mimics this clinical presentation, relative to other existing bovine laminitis models. In the OF model, characteristic pathophysiological and histological changes are observed as in natural clinical cases (8, 14). It also has been utilized by several related studies, such as the biomechanics of claw suspensory tissue (15) and the oxidative response of neutrophils to platelet-activating factors (16). Furthermore, this model is a more reliably induced and representative model than others (starch overload and histamine injection models) (17). Over the last two decades, this model has been intensively used in the study of equine laminitis (18–20), but less frequently in bovine laminitis (21).
A clinically similar but distinct disease called sepsis-related laminitis (SRL) has been described in horses (18), which has similar pathophysiological characteristics to systemic inflammatory response syndrome (SIRS) in humans (22). Inflammatory responses play a central role in laminar injury and subsequent failure in the OF-induced equine laminitis, characterized by markedly increases of inflammatory mediator gene expression and leukocyte influx in lamellae tissue (18, 19, 23). These inflammatory mediators primarily contributed to the inflammatory response, including activation of vessel endothelium, adhesion and emigration of leukocytes, and changes in vessel, which resulted in organ injury and failure (24). However, to our knowledge, no existing studies have characterized the inflammatory response and its role in the pathogenesis of OF-induced bovine laminitis. Thus, the laminar messenger RNA (mRNA) expression of multiple cytokines, chemokines, adhesion molecules, and inflammatory molecules was assessed in the context of the OF-induced bovine laminitis model. The objective of this study was to characterize the inflammatory response in a bovine laminitis model as a foundation for future laminitis studies.
Materials and Methods
Ethics Statement
The experimental protocols describing the management and care of animals were approved by the Animal Ethics Committee (AEC) of the Northeast Agricultural University (Harbin, China) that monitors compliance with the Animal Welfare Act (2001). All heifers were continuously monitored by the investigators (permission number: SRM-13).
Animals
A total of 12 Chinese Holstein dairy heifers were used in this study at an average age of 20.67 ± 3.01 months, an average body weight (BW) of 379.71 ± 19.77 kg, and an average body condition score (25) of 3.00 ± 0.23. Each heifer was clinically healthy, had no history of severe systemic diseases, exhibited normal locomotion and posture, and had no claw disorders (solar ulceration, white line disease, etc.). Animals to be enrolled in the study were purchased from the Wandashan Dairy Farm (Harbin, China).
Model Induction
The claws of heifers were trimmed 10 days prior to initiation of the experiment. For acclimation, heifers were housed in a large animal experimental barn with a concrete floor. Heifers were trained and fed grass hay ad libitum (7.5% total water-soluble sugar content). After acclimation, all heifers could accept clinical examination without any discomfort, could be led to walk and trot by hand, and agreed to lift the distal front limb for foot palpation and hoof testing. The heifers were randomly arranged into two groups, including a treatment group (n = 6) and a control group (n = 6). Each animal in the treatment group was administered OF solutions (Bailong Biotech, Inc., Dezhou, China; purity, 98%; dose, 17 g/kg BW in 2 L/100 kg of BW warm deionized water) into the rumen via gastric tube, and control group heifers were given 2 L/100 kg of BW warm deionized water by the same method, as previously described (14, 17). A 5% OF dose was administered twice daily before the experiment for 3 days.
Orthopedic examinations were performed (14), including locomotion assessment, hoof testing, and weight shifting at −24, 0, 6, 12, 18, 24, 36, 48, 60, and 72 h. In the locomotion assessment, the heifers were led by hand to walk and trot in a straight line and to turn in a small circle on the same surface. Five licensed veterinarians assessed the locomotion scores of each heifer according to previous study (Table 1) in an experimentally blinded manner (26). Heifers receiving a score of ≥2 by all veterinarians were considered to be lame. In hoof testing, the front legs were lifted up, while a hoof tester was applied over the site of the axial sole–bulb junction and the central region of the dorso-abaxial claw wall in all front claws. A suitable pressure was applied to assess fasciculation in the musculus triceps. Animals were scored based on their attempts to withdraw their legs. Reactions to hoof testing were subjectively classified as none, slight, or marked. In weight shifting, heifers were observed whether they shifted their weight to another side during the examination period.
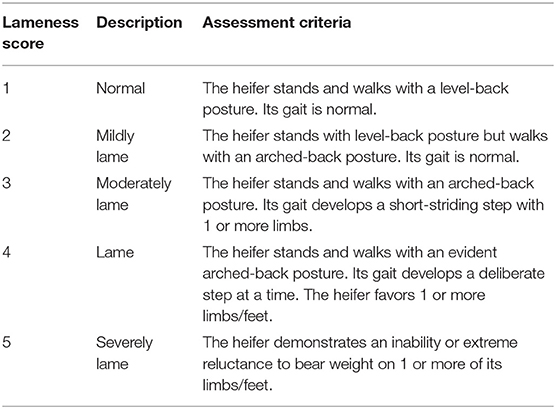
Table 1. Locomotion scoring system adapted from Sprecher et al. (26).
For the consideration of animal welfare, supportive therapy was provided (14). Ringer's acetate (Heping Animal Medicine, Inc., Harbin, China; dose, 15 ml/kg of BW) and sodium bicarbonate (Heping Animal Medicine, Inc., Harbin, China; specification, 84 g/L; dose, 1.5 ml/kg of BW) were administered at 18 and 24 h, and calcium borogluconate (Heping Animal Medicine, Inc., Harbin, China; specification, 14 mg of Ca/ml; dose, 1.4 ml/kg of BW) was administered by jugular infusion at 18 h.
Sample Acquisition
When the treatment group heifers were scored ≥2 and had consecutive positive reactions in the same claw (14, 17), the animals were humanely euthanized. The control heifers were humanely euthanized at 72 h. The front limbs were rapidly removed by disarticulation of the metacarpophalangeal joint. Several tissues (approximately 2.5–3.0 cm2) were excised using a band saw, consisting of the third phalanx, lamellae tissue, and the central part of the dorso-abaxial claw wall (27, 28). Lamellae tissue (~1 cm2) was rapidly dissected from the larger tissue blocks using a lancet. Several dissected lamellae were immediately snap frozen in liquid nitrogen and later stored at −80°C. Remaining lamellae samples were fixed in 10% neutral buffered formalin (Kanning Medicine, Inc., Zhongshan, China) for at least 24 h. The formalin-fixed lamellae was treated with graded alcohol, xylene, and paraffin using an automatic tissue-processing machine (8). Sections (4 μm thick) were cut and stained with Mayers hematoxylin and eosin (H&E) for observation of histological changes within the epidermal and dermal lamellae and periodic acid–Schiff (PAS) staining for basement membrane changes. Veterinary pathologist Prof. Guangxing Li (Northeast Agricultural University) and medical pathologist Prof. Zhao (Harbin Medical University) blindly read the sections in a random order.
RNA Isolation and cDNA Synthesis
Total RNA was extracted from three separate lamellae samples of 12 heifers using Absolutely RNA Miniprep kits (Stratagende, Inc., California, USA). The quantity and purity of extracted RNA were measured using a NanoDropTM One Microvolume spectrophotometry (Thermo Scientific, Massachusetts, USA). The integrity of RNA was determined by 1% agarose gel electrophoresis (Bio-Rad Laboratories, California, USA). One microgram of total RNA was reverse transcribed using the PrimeScriptTM RT reagent kit (Takara, Dalian, China) with genomic DNA (gDNA) eraser according to the manufacturer's instructions. Subsequently, complementary DNA (cDNA) was diluted with DNase/RNase free water (dilution rate, 1:4) (Takara, Dalian, China) and stored at −20°C.
Real-Time Quantitative PCR
The quantitative PCR (qPCR) was conducted using a LightClycler 480 (Roche, Indiana, Germany), and quantification was assessed with SYBR Premix Ex TaqTM II (Takara, Dalian, China). The primers for interleukin (IL)-1β, IL-6, IL-8, IL-10, tumor necrosis factor alpha (TNF-α), C-X-C motif chemokine ligand (CXCL)-1, CXCL-6, macrophage cationic peptide (MCP)-1, MCP-2, E-selectin, intercellular adhesion molecule-1 (ICAM-1), cyclooxygenase-2 (COX-2), inducible nitric oxide synthase-1 (iNOS-1), plasminogen activator inhibitor-1 (PAI-1), and housekeeping genes (β-actin, GAPDH, and UXT) used in this study were the same as previously reported (27–31) and were designed to target bovine-specific sequences (Table 2). The specificity of selected primer sequences was verified using the Basic Local Alignment Search Tool (BLAST) from the National Center for Biotechnology information (NCBI) database (http://blast.ncbi.nlm.nih.gov/). A 20-μl PCR mixture contained 2 μl sample cDNA and 18 μl PCR master mix. The master mix contained 10 μl SYBR green dye, 1.6 μl each primer solution (forward and reverse primers, each 10 μM), and 6.4 μl DNase/RNase free water. The PCR conditions were as follows: 95°C for 1 min (ramp rate, 4.4°C/s); 40 cycles of amplification (quantification analysis model), 95°C for 5 s (ramp rate, 4.4 °C/s) and 60°C for 1 min (ramp rate, 2.2°C/s); 1 cycle of melting (melting curves analysis model), 95°C for 5 s (ramp rate, 4.4°C/s), 60°C for 1 min (ramp rate, 2.2°C/s), increasing to 95°C (ramp rate, 0.11°C/s); 1 cycle of cooling, 50°C for 30 s (ramp rate, 2.2°C/s). All amplified cDNA fragments were confirmed by gel electrophoresis and melting curve analysis. The values of cycle threshold crossing (Ct) were calculated by the Light Cycler 480 software (version 1.5.0, Roche, Germany). Template DNA (10-folded serial dilution) were used to generate standard curves and calculate the efficiency for each PCR reaction. Negative controls consisted of DNase/RNase free water, and each sample was analyzed in triplicate.
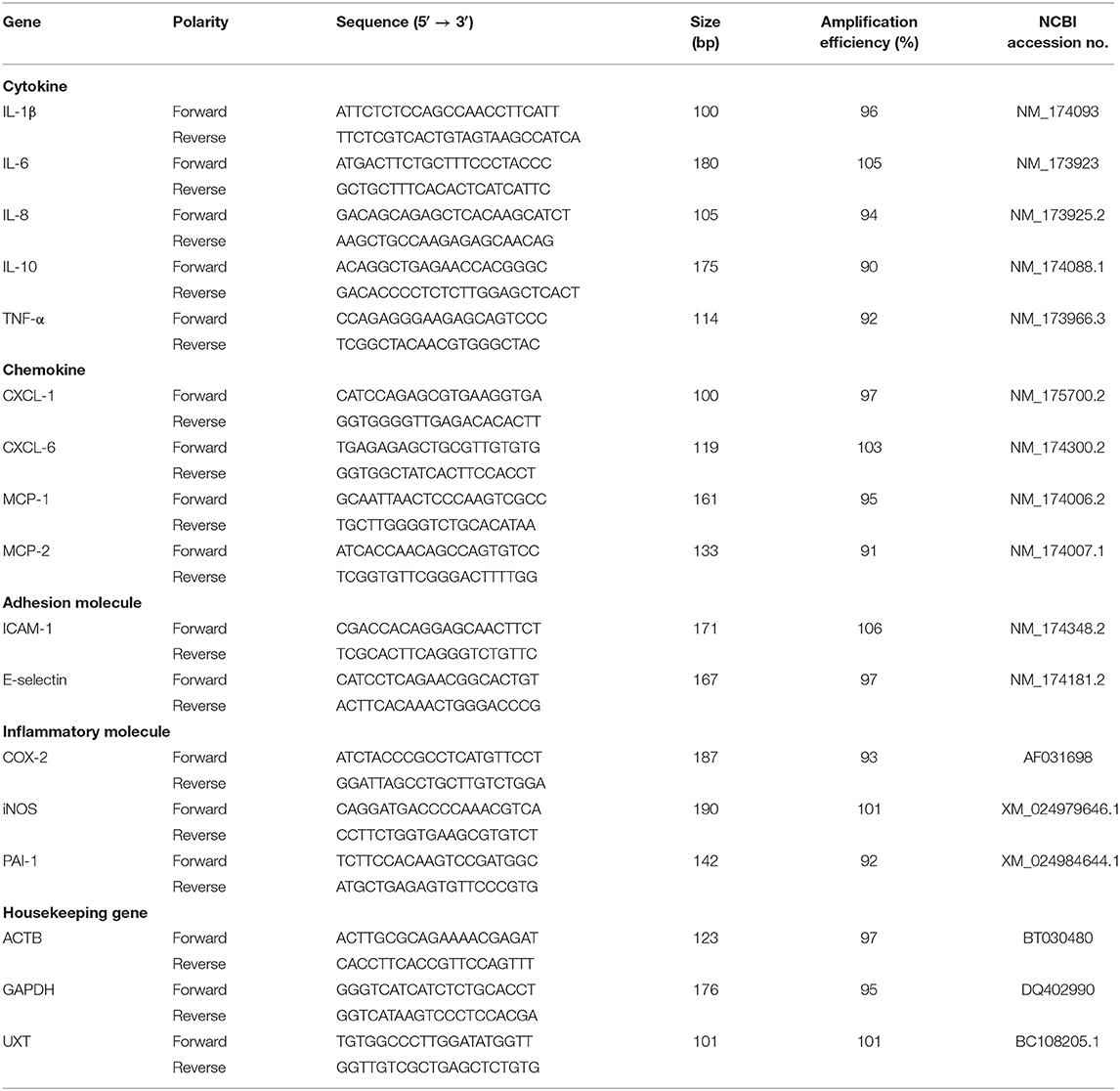
Table 2. Gene, sequence, amplification size, and efficiency of the primers used for quantitative PCR (qPCR).
Data Analysis
The 2−ΔΔCt method was used to calculate changes in relative gene expression. Data analysis was performed using GraphPad Prism (version 7.04, GraphPad Software, Inc., San Diego, USA). The data fulfilled the assumption of a Gaussian distribution, according to a Shapiro–Wilk normality test. The data were analyzed by Student's t-test. P < 0.05 was considered significant. All data are presented as mean ± standard deviation (SD).
Results
Clinical Data
Heifers of the treatment group developed depression, anorexia, watery diarrhea, tachycardia, tachypnea, and pyrexia. The signs of depression, anorexia, and watery diarrhea were observed at 6–12 h, and severe signs occurred at 24–36 h, then gradually recovered. Generally, persistent tachycardia, hypopnea, and pyrexia could be observed from 6 to 72 h and became increasingly severe before 36 h, then gradually recovered. At 36 h, the rate of heart beat reached the maximum mean value (69.83 ± 13.82 beats/min), rectal temperatures also reached the maximum mean value (39.25 ± 0.40°C), and respiratory rate reached the minimum mean value (15.00 ± 1.90 counts/min). These heifers were otherwise graded as normal at −24 and 0 h. The signs of lameness were observed at 36 h. At 72 h, the locomotion scores of them were ≥2. From 36 to 60 h, a variable clinical observation for hoof testing examination was observed in the animals. At 72 h, each of them had a consecutive reaction in the same claw. All heifers developed weight shifting involving both front and hind legs. Weight shifting was first observed in two heifers at 12 h and later in all remaining heifers between 36 and 72 h.
Heifers in the control group failed to develop clinical signs of systemic inflammatory disease. During the assay period, clinical observations were normal, for heart rate (48.92 ± 1.70 beats/min), rectal temperature (38.31 ± 0.22°C), and respiratory rate (23.35 ± 1.11 counts/min). They were graded as normal (locomotion score = 1) and did not exhibit pain reactions during hoof testing examination with no signs of weight shifting.
Laminar Histopathology of Claws
Gross observation of the control group revealed normal lamellae. From histological sections, the epidermal and dermal layers of the lamellae were intimately connected (Figures 1A,B). In the PAS-stained sections, the epidermal lamellae had a rounded tip and was closely connected to the basement membrane. The basement membrane appeared as a consecutive and distinct line. In the HE-stained sections, the basophilic nuclei of basal cells were oval and located away from the basement membrane with regular nuclear polarity shifts. Suprabasal cells were almost parallel to the direction of epidermal lamellae. Hyperemia, hemorrhage, and a few inflammatory cells (mainly lymphocytes and macrophages) were observed, albeit rarely, in the dermal lamellae.
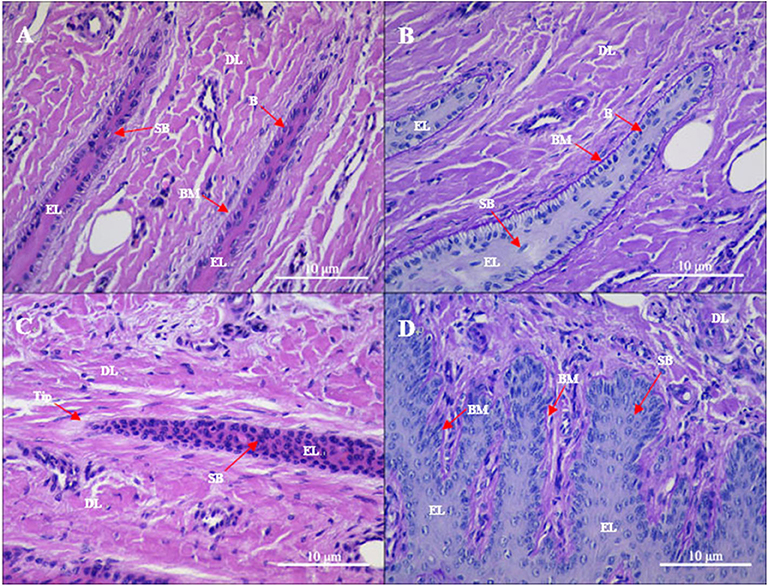
Figure 1. Gross sections of the lamellae layer from (A,B) control and (C,D) oligofructose treated heifers. Labels in the sections: B, basal cells; SB, surprabasal cells; BM, basement membrane; DL, dermal lamellae; EL, epidermal lamellae; WBC, white blood cells; Tip, the tip of the epidermal lamellae). (A) H&E stain. The normal appearance of the dermo-epidermal junction consists of numerous interlocking lamellae. (B) Periodic acid–Schiff (PAS) stain. The tip of the epidermal lamellae is rounded and in a close association with the basement membrane. (C) H&E stain. Stretched epidermal lamellae and increased numbers of suprabasal cells are observed. (D) PAS stain. The basement membrane has an attenuated appearance; the basal cells are enlarged and wider in the dermal lamellae.
Gross observation of the treatment group revealed a patchy distribution of dermal hyperemia in the dorsal and bottom region of the lamellae. In the histological sections, the epidermal lamellae appeared stretched and with a pointed tip (Figures 1C,D). In the PAS-stained sections, the basement membrane had a folded and attenuated appearance, and occasionally separated from the basal cells. Basal cells had rounded and centrally positioned nuclei. Nuclei had a coarse chromatin network. Suprabasal cells appeared hyperplastic. Hyperemia, hemorrhage, and pronounced inflammatory infiltrates (granulocytes and monocytes) were observed in the dermal lamellae.
Laminar Inflammatory Cytokine mRNA Concentration
Laminar mRNA concentrations of the cytokines IL-1β, IL-6, and IL-8 were significantly increased in the treatment group (P < 0.01) relative to the control group. There was no change in laminar mRNA expression of IL-10 and TNF-α (Table 3 and Figure 2).
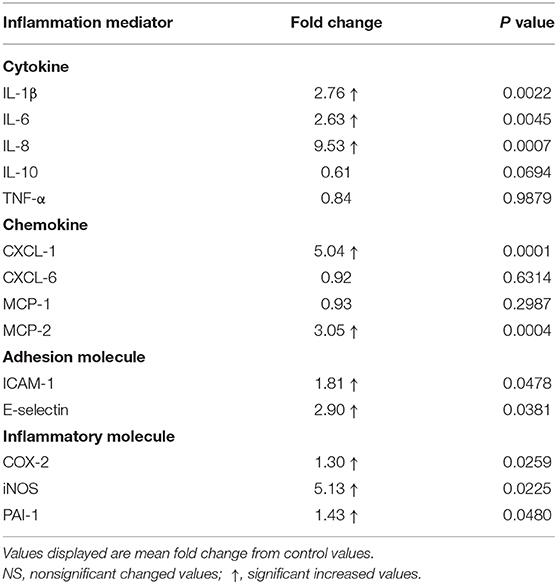
Table 3. Fold changes in laminar gene expression of inflammation mediators after oligofructose administration.
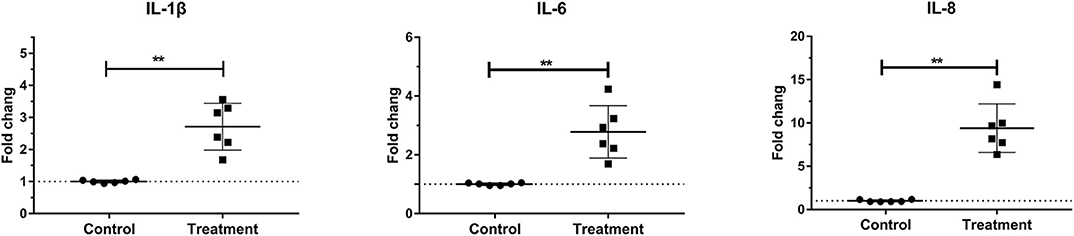
Figure 2. Mean fold changes in interleukin (IL)-1β, IL-6, and IL-10 messenger RNA (mRNA) expression after oligofructose administration in heifers. Significant increases in cytokines relative to the control group are indicated as **P < 0.01.
Laminar Chemokine mRNA Concentration
Laminar mRNA concentrations of chemokine CXCL-1 and MCP-2 were significantly increased in the treatment group (P < 0.01) compared to the control group. There was no change in laminar mRNA expression of chemokine CXCL-6 and MCP-1 (Table 3 and Figure 3).
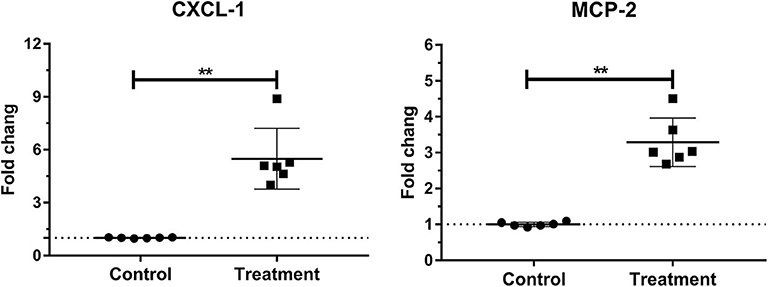
Figure 3. Mean fold changes in CXCL-1 and MCP-2 messenger RNA (mRNA) expression following oligofructose administration in heifers. Significant increases in chemokines relative to the control group are indicated as **P < 0.01.
Laminar mRNA Concentration of Endothelial Adhesion Molecule
Laminar mRNA expressions of the endothelial adhesion molecule E-selectin (P < 0.01) and ICAM-1 (P < 0.05) were significantly increased in the treatment group relative to the control group (Table 3 and Figure 4).
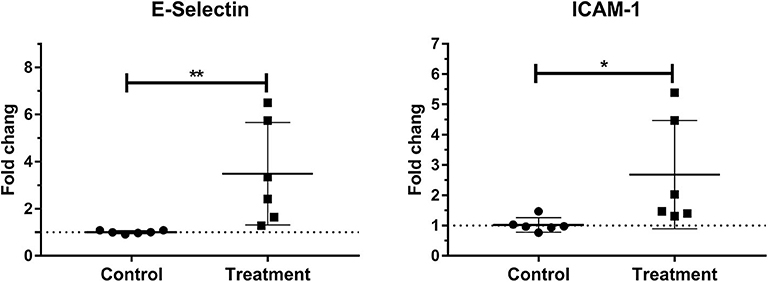
Figure 4. Median fold changes in E-selectin and intercellular adhesion molecule-1 (ICAM-1) messenger RNA (mRNA) expression after oligofructose administration in heifers. Significant increases in adhesion molecules relative to the control group are indicated as *P < 0.05, **P < 0.01.
Laminar mRNA Concentration of Inflammatory Molecule
Laminar mRNA expression of inflammatory molecules COX-2 (P < 0.01), iNOS (P < 0.01), and PAI-1 (P < 0.05) was significantly increased in the treatment group when compared to the control group (Table 3 and Figure 5).
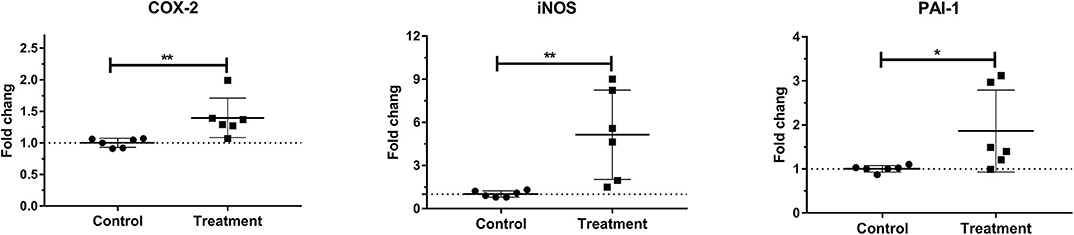
Figure 5. Mean fold changes in cyclooxygenase-2 (COX-2), inducible nitric oxide synthase-1 (iNOS-1), and plasminogen activator inhibitor-1 (PAI-1) messenger RNA (mRNA) expression after oligofructose administration in heifers. Significant increases in inflammatory molecules relative to the control group are indicated as *P < 0.05, **P < 0.01.
Discussion
Bovine laminitis is also referred to as diffuse aseptic pododermatitis, which results in local and systemic clinical signs (32). It is characterized by claw pain and lameness (33). In clinical practices, laminitis is typically secondary to severe systemic inflammatory disease (e.g., septic pleuropneumonia, metritis, ruminal acidosis, etc.).
In the present study, the OF overload model was employed to induce a bovine model of laminitis. This model was selected for the following reasons: (1) the histamine injection model and the starch overload model are not reliable; (2) the histological changes in the OF model are similar to those observed in clinical laminitis cases; and (3) the OF model has been widely used in the studies of bovine and equine laminitis.
The laminitis-inducing mechanism of OF is similar to other carbohydrate overload models. A high intake of nonstructural carbohydrates, which could reduce the rumen pH, results in the death and lysis of Gram-negative bacteria. This bacterial die-off compromises the intestinal barrier functions. This allows lipopolysaccharide (LPS) to translocate from the digestive tract into the peripheral circulation (17, 34, 35). Subsequently, free LPS activates systemic inflammatory responses and leads to multiorgan damage, such as rumenitis (36), synovitis (37), and liver impairment (38).
In the present study, heifers in the treatment group developed characteristic signs of bovine laminitis, and histological changes of their lamellae tissues were consistent with clinical laminitis cases. Those results corroborated those of previous studies, which indicated the successful induction of bovine laminitis (14, 17). This study reported that laminar mRNA expressions of several inflammatory cytokines, chemokines, endothelial adhesion molecules, and inflammatory molecules were increased at the early stage of the OF-induced bovine laminitis. To our knowledge, this is the first study to characterize the inflammatory response in this model. Our findings were similar to equine SRL and human SIRS/sepsis (16, 39), indicating that lamellae failure might be caused by a systemic inflammatory response, as is the case with organ failure in human SIRS/sepsis.
Pathogen-associated molecular pattern molecules (PAMPs) (e.g., LPS) activate leukocytes and other innate immune cells (40, 41). Inflammatory mediators including inflammatory cytokines, chemokines, endothelial adhesion molecules, and inflammatory molecules could be produced by PAMP-activated cells (24). Meanwhile, the activated leukocytes pass through the activated endothelium and migrate into the tissues of target organs (42). Activated tissue macrophages, migratory leukocytes, and multiple inflammatory mediators together constitute the inflammatory response, leading to organ injury and failure resulting from sepsis (43).
In this study, gene expression of inflammatory cytokines IL-1β, IL-6, and IL-8 was increased in the lamellae of the treatment group. Previous studies reported that the laminar expression of IL-1β, IL-6, and IL-8 was related to the degree of lamellar injury (44). Furthermore, these inflammatory cytokines are important components of the innate immune response, which have also been reported to participate in human SIRS/sepsis (22). A previous study found that the increased plasma level of IL-1β was negatively correlated with the progression of organ dysfunction and mortality in septic patients (45). Another study reported that IL-1β commonly underwent an early but transient burst of expression after being exposed to the stimulation of endotoxin (46). Similarly, an increase in IL-1β at the early stage of the development of laminitis was observed here, indicating that it might take part in the productions of other cytokines.
In human sepsis, IL-6 expression was correlated with organ injury and apoptosis (47). Recently, a commercial IL-6 signaling-related drug was used as a potential therapeutic for the treatment of rheumatoid arthritis and sepsis (48, 49). It has been reported that IL-6 signaling-related glycoprotein 130 (gp130) might play an important role in the stretching of epidermal lamellae and separation from the dermal lamellae (50). These changes are also histologically characteristic of clinical laminitis cases, which were also observed in this study. It has been reported that IL-8 primarily acts as a chemokine, promoting migration of neutrophils into the lamellae in OF-induced bovine laminitis (51). It has also been reported to be expressed by endothelial cells following exposure to PAMPs and TNF-α (46).
Tumor necrosis factor-α is a prominent inflammatory cytokine during human SIRS/sepsis. However, a notable lack of TNF-α expression was observed in this study. In OF-induced equine laminitis, the expression of TNF-α was also not increased early on in the lamellae, but the serum concentration of TNF-α was significantly increased (52). These observations are indicative of a systemic cytokine response. An early transient burst of TNF-α occurring in this process may be another possible explanation. A lung sepsis model in baboon reported that the gene expression of TNF-α was also not increased, but that of IL-1β and IL-6 was increased in the target organ (53). Similar observations were made here.
Like TNF-α, IL-10 expression was not increased in this study, indicating that the lamellae did not mount an anti-inflammatory response during the early stage of laminitis. It is well established that IL-10 is an anti-inflammatory cytokine that serves to temper immune responses (42). Increased IL-10 has generally been associated with improved prognostic outcomes in septic patients (54). The expression of IL-10 was not increased, which can be explained by the lack of TNF-α response in this study (18). This speculation is based on previous studies, in which the plasma concentration of IL-10 was decreased by the administration of antimonoclonal TNF-α antibodies in chimpanzees with sepsis (55).
The expression of the chemokines CXCL-1 and MCP-2 were increased in the lamellae, suggesting that the migration of neutrophils and mononuclear cells into lamellae maybe involved in this inflammatory response. In OF-induced equine laminitis, increased gene expression of CXCL-1 and MCP-2 and the migration of neutrophils and mononuclear cells into lamellae are observed (20). A recent study reported that CXCL-1 could induce neutrophils to infiltrate the sites of bacterial infection and was involved in the granulopoiesis and mobilization of neutrophils in sepsis (56). Furthermore, MCP-2 is another important chemokine in inflammation and immunomodulation, which could activate mononuclear cells and direct them into target tissues (57).
The expressions of the adhesion molecules E-selectin and ICAM-1 were increased in lamellae, indicating that endothelial activation occurred in this study. The increased expression of adhesion molecules is a characteristic of endothelial activation, which leads to the adhesion of circulating leukocytes. The expression of E-selectin and ICAM-1 could be explained by the upregulated expression of IL-1β and IL-6, as these proinflammatory cytokines positively induce the transcriptional regulation of E-selectin and ICAM-1 (58).
In this study, the expressions of the inflammatory molecules COX-2, iNOS, and PAI-1 were increased in the lamellae. Similar results were reported in OF-induced equine laminitis, indicating that these inflammatory molecules might play similar roles in the two laminitis models (18). COX-2 is known as a crucial mediator in the inflammatory response, as the various physiological effects of numerous prostanoids (the products downstream of COX-2), including platelet aggregation, vasomotor, and proinflammatory effects (59). As such, COX-2 is a therapeutic target for nonsteroidal anti-inflammatory drugs (NSAIDs). The increased expression of COX-2 could be speculated from the increased proinflammatory cytokine IL-1 in this study, as the expression of COX-2 was mainly driven by the activation of nuclear factor-kappa B (downstream of proinflammatory cytokine signaling) (60). However, the pathological functions of COX-2 in bovine laminitis were still unclear. In equine laminitis, laminar COX-2 expression was observed in multiple laminitis-related cells (61), especially laminar basal epithelial cells (LBEC). Furthermore, LBEC are responsible for maintaining the integrity of the epidermal lamellae and basement membrane. Therefore, it is possible that COX-2 maybe involved in the failure of the lamellae and that COX-2 may serve as a prognostic indicator of laminitis. In endotoxin-induced sepsis, COX-2-deficient mice exhibited increased survival and decreased leukocyte infiltration into kidneys and lungs, when compared to wild-type mice (62).
Expression of the vasoactive substances iNOS and PAI-1 were increased in lamellae. Overexpression of iNOS results in excessive nitric oxide (NO), which causes systemic/local hypotension and organ dysfunction in sepsis (63). Elevated PAI-1 expression was reported to be a crucial regulator of fibrinolysis by inhibiting plasminogen activator, which could lead to disseminated intravascular coagulation and organ dysfunction in septic patients (64). Intravascular coagulation has been observed in bovine lamellae with laminitis (9). Recently, PAI-1 was determined to be a significant predictor of severity through a meta-analysis of human sepsis cases (65).
In conclusion, this study reported the occurrence of laminar inflammatory responses at the early stages of OF-induced bovine laminitis. Expression of multiple mediators of inflammation increased, indicating that the inflammatory injury might play an important role in the pathogenesis of laminitis. Moving forward, more in-depth molecular studies on inflammatory signaling pathways [e.g., nuclear factor kappa B (NF-κB) mitogen-activated protein kinase (MAPK) pathways] are needed to discover effective anti-inflammatory agents for the treatment of bovine laminitis.
Data Availability Statement
The raw data supporting the conclusions of this article will be made available by the authors, without undue reservation, to any qualified researcher.
Ethics Statement
The animal study was reviewed and approved by Animal Ethics Committee (AEC) of Northeast Agricultural University, Harbin, China (permission number: SRM-13).
Author Contributions
JD designed and performed the experiments, analyzed data, and wrote the manuscript. SL, LJ, YL, XZ, QS, and MH performed the animal experiments and contributed to the technical discussions. J-TZ and HW designed the experiments and provided guidance. All authors contributed to the article and approved the submitted version.
Funding
This study was funded by the National Key R & D Program of China (Project No. 2017YFD0502200) and Heilongjiang Provincial Funding for National Subjects (GX18B023).
Conflict of Interest
The authors declare that the research was conducted in the absence of any commercial or financial relationships that could be construed as a potential conflict of interest.
Acknowledgments
We thank Prof. Guangxing Li (NEAU) and Prof. Zhao (HMU) for explaining the histological changes of lamellae tissues, and thank Dr. Qing Tong, Dr. Yansong Ge, Master Dongdong Qi, and Master Mengxin Hu for their assistance in the study. Finally, Assistant Editor Francisco Javier Salguero and reviewers are sincerely thanked for valuable guidance and careful comments to this manuscript.
Supplementary Material
The Supplementary Material for this article can be found online at: https://www.frontiersin.org/articles/10.3389/fvets.2020.00351/full#supplementary-material
Video S1. The treatment group heifers became lamed after oligofructose overload administration.
Video S2. The treatment group heifers refused to move after oligofructose overload administration, although researchers led them with a big strength.
Figure S1. The hoof band of heifers became red and swell after oligofructose overload administration.
References
1. Alvergnas M, Strabel T, Rzewuska K, Sell-Kubiak E. Claw disorders in dairy cattle: effects on production, welfare and farm economics with possible prevention methods. Livest Sci. (2019) 222:54–64. doi: 10.1016/j.livsci.2019.02.011
2. Dolecheck K, Bewley J. Animal board invited review: dairy cow lameness expenditures, losses and total cost. Animal. (2018) 12:1462–74. doi: 10.1017/S1751731118000575
3. Potterton SL, Bell NJ, Whay HR, Berry EA, Atkinson OCD, Dean RS, et al. A descriptive review of the peer and non-peer reviewed literature on the treatment and prevention of foot lameness in cattle published between 2000 and 2011. Vet J. (2012) 193:612–6. doi: 10.1016/j.tvjl.2012.06.040
4. van der Spek D. Genetic Background of Claw Health in Dairy Cattle. Wageningen: Wageningen University (2015).
5. van der Spek D, van Arendonk JAM, Vallee AAA, Bovenhuis H. Genetic parameters for claw disorders and the effect of preselecting cows for trimming. J Dairy Sci. (2013) 96:6070–8. doi: 10.3168/jds.2013-6833
6. Somers J, Frankena K, Noordhuizen-Stassen EN, Metz J. Prevalence of claw disorders in Dutch dairy cows exposed to several floor systems. J Dairy Sci. (2003) 86:2082–93. doi: 10.3168/jds.S0022-0302(03)73797-7
7. Boosman R, Nemeth F, Gruys E. Bovine laminitis: clinical aspects, pathology and pathogenesis with reference to acute equine laminitis. Vet Q. (1991) 13:163–71. doi: 10.1080/01652176.1991.9694302
8. Thoefner MB, Wattle O, Pollitt CC, French KR, Nielsen SS. Histopathology of oligofructose-induced acute laminitis in heifers. J Dairy Sci. (2005) 88:2774–82. doi: 10.3168/jds.S0022-0302(05)72957-X
9. Mendes HM, Casagrande FP, Lima IR, Souza CH, Gontijo LD, Alves GE, et al. Histopathology of dairy cows' hooves with signs of naturally acquired laminitis. Pesquisa Vet Bras. (2013) 33:613–9. doi: 10.1590/S0100-736X2013000500011
10. Dong S-W, Zhang S-D, Wang D-S, Wang H, Shang X-F, Yan P, et al. Comparative proteomics analysis provide novel insight into laminitis in Chinese Holstein cows. BMC Vet Res. (2015) 11:161. doi: 10.1186/s12917-015-0474-x
11. Randall LV, Green MJ, Huxley JN. Use of statistical modelling to investigate the pathogenesis of claw horn disruption lesions in dairy cattle. Vet J. (2018) 238:41–8. doi: 10.1016/j.tvjl.2018.07.002
12. Vermunt J. “Subclinical” laminitis in dairy cattle. New Zealand Vet J. (1992) 40:133–8. doi: 10.1080/00480169.1992.35718
13. Enemark JM. The monitoring, prevention and treatment of sub-acute ruminal acidosis (SARA): a review. Vet J. (2008) 176:32–43. doi: 10.1016/j.tvjl.2007.12.021
14. Danscher AM, Enemark JMD, Telezhenko E, Capion N, Ekstrom CT, Thoefner MB. Oligofructose overload induces lameness in cattle. J Dairy Sci. (2009) 92:607–16. doi: 10.3168/jds.2008-1271
15. Danscher AM, Toelboell TH, Wattle O. Biomechanics and histology of bovine claw suspensory tissue in early acute laminitis. J Dairy Sci. (2010) 93:53–62. doi: 10.3168/jds.2009-2038
16. Concha C, Carretta MD, Alarcon P, Conejeros I, Gallardo D, Hidalgo AI, et al. Oxidative response of neutrophils to platelet-activating factor is altered during acute ruminal acidosis induced by oligofructose in heifers. J Vet Sci. (2014) 15:217–24. doi: 10.4142/jvs.2014.15.2.217
17. Thoefner M, Pollitt C, Van Eps A, Milinovich G, Trott D, Wattle O, et al. Acute bovine laminitis: a new induction model using alimentary oligofructose overload. J Dairy Sci. (2004) 87:2932–40. doi: 10.3168/jds.S0022-0302(04)73424-4
18. Leise BS, Faleiros RR, Watts M, Johnson PJ, Black SJ, Belknap JK. Laminar inflammatory gene expression in the carbohydrate overload model of equine laminitis. Equine Vet J. (2011) 43:54–61. doi: 10.1111/j.2042-3306.2010.00122.x
19. Godman JD, Burns TA, Kelly CS, Watts MR, Leise BS, Schroeder EL, et al. The effect of hypothermia on influx of leukocytes in the digital lamellae of horses with oligofructose-induced laminitis. Vet Immunol Immunop. (2016) 178:22–8. doi: 10.1016/j.vetimm.2016.05.013
20. Dern K, van Eps A, Wittum T, Watts M, Pollitt C, Belknap J. Effect of continuous digital hypothermia on lamellar inflammatory signaling when applied at a clinically-relevant timepoint in the oligofructose laminitis model. J Vet Int Med. (2018) 32:450–8. doi: 10.1111/jvim.15027
21. Huxley JN. Lameness in cattle: an ongoing concern introduction. Vet J. (2012) 193:610–1. doi: 10.1016/j.tvjl.2012.06.039
22. Jaffer U, Wade R, Gourlay T. Cytokines in the systemic inflammatory response syndrome: a review. HSR Proc Intens Care Cardiovasc Anesth. (2010) 2:161. doi: 10.1002/bjs.1800840707
23. Belknap JK, Giguere S, Pettigrew A, Cochran AM, Van Eps AW, Pollitt CC. Lamellar pro-inflammatory cytokine expression patterns in laminitis at the developmental stage and at the onset of lameness: innate vs. adaptive immune response. Equine Vet J. (2007) 39:42–7. doi: 10.2746/042516407X155406
24. de Jong HK, van der Poll T, Wiersinga WJ. The systemic pro-inflammatory response in sepsis. J Innate Immun. (2010) 2:422–30. doi: 10.1159/000316286
25. Edmonson A, Lean I, Weaver L, Farver T, Webster G. A body condition scoring chart for Holstein dairy cows. J Dairy Sci. (1989) 72:68–78. doi: 10.3168/jds.S0022-0302(89)79081-0
26. Sprecher D, Hostetler D, Kaneene J. A lameness scoring system that uses posture and gait to predict dairy cattle reproductive performance. Theriogenology. (1997) 47:1179–87. doi: 10.1016/S0093-691X(97)00098-8
27. Osorio J, Fraser B, Graugnard D, Singh S, Drackley JK, Garrett E, et al. Corium tissue expression of genes associated with inflammation, oxidative stress, and keratin formation in relation to lameness in dairy cows. J Dairy Sci. (2012) 95:6388–96. doi: 10.3168/jds.2011-5143
28. Mills JA, Zarlenga DS, Habecker PL, Dyer RM. Age, segment, and horn disease affect expression of cytokines, growth factors, and receptors in the epidermis and dermis of the bovine claw. J Dairy Sci. (2009) 92:5977–87. doi: 10.3168/jds.2009-2097
29. O'Driscoll K, McCabe M, Earley B. Differences in leukocyte profile, gene expression and metabolite status of dairy cows with or without sole ulcers. J Dairy Sci. (2015) 98:1685–95. doi: 10.3168/jds.2014-8199
30. Bermejo-Álvarez P, Lonergan P, Rath D, Gutiérrez-Adan A, Rizos D. Developmental kinetics and gene expression in male and female bovine embryos produced in vitro with sex-sorted spermatozoa. Reprod Fertil Dev. (2010) 22:426–36. doi: 10.1071/RD09142
31. Abdelmegeid M, Vailati-Riboni M, Alharthi A, Batistel F, Loor JJ. Supplemental methionine, choline, or taurine alter in vitro gene network expression of polymorphonuclear leukocytes from neonatal Holstein calves. J Dairy Sci. (2017) 100:3155–65. doi: 10.3168/jds.2016-12025
32. Nilsson SA. Clinical, morphological, and experimental studies of laminitis in cattle. Acta Vet Scand. (1963) 4(Suppl. 1):297–304.
33. Greenough PR. Bovine Laminitis and Lameness: A Hands on Approach. Philadelphia, PA: Elsevier Health Sciences (2007).
34. Plaizier JC, Khafipour E, Li S, Gozho GN, Krause DO. Subacute ruminal acidosis (SARA), endotoxins and health consequences. Animal Feed Sci Technol. (2012) 172:9–21. doi: 10.1016/j.anifeedsci.2011.12.004
35. Khafipour E, Plaizier JC, Aikman PC, Krause DO. Population structure of rumen Escherichia coli associated with subacute ruminal acidosis (SARA) in dairy cattle. J Dairy Sci. (2011) 94:351–60. doi: 10.3168/jds.2010-3435
36. Zhao C, Liu G, Li X, Guan Y, Wang Y, Yuan X, et al. Inflammatory mechanism of Rumenitis in dairy cows with subacute ruminal acidosis. BMC Vet Res. (2018) 14:135. doi: 10.1186/s12917-018-1463-7
37. Hidalgo AI, Carretta MD, Alarcón P, Manosalva C, Müller A, Navarro M, et al. Pro-inflammatory mediators and neutrophils are increased in synovial fluid from heifers with acute ruminal acidosis. BMC Vet Res. (2019) 15:225. doi: 10.1186/s12917-019-1974-x
38. Guo J, Chang G, Zhang K, Xu L, Jin D, Bilal MS, et al. Rumenderived lipopolysaccharide provoked inflammatory injury in the liver of dairy cows fed a high-concentrate diet. Oncotarget. (2017) 8:46769–80. doi: 10.18632/oncotarget.18151
39. Matsuda N, Hattori Y. Systemic inflammatory response syndrome (SIRS): molecular pathophysiology and gene therapy. J Pharmacol Sci. (2006) 101:189–98. doi: 10.1254/jphs.CRJ06010X
40. Umbro I, Gentile G, Tinti F, Muiesan P, Mitterhofer AP. Recent advances in pathophysiology and biomarkers of sepsis-induced acute kidney injury. J Infect. (2016) 72:131–42. doi: 10.1016/j.jinf.2015.11.008
41. Bianchi ME. DAMPs, PAMPs and alarmins: all we need to know about danger. J Leukocyte Biol. (2007) 81:1–5. doi: 10.1189/jlb.0306164
42. Wiersinga WJ, Leopold SJ, Cranendonk DR, van der Poll T. Host innate immune responses to sepsis. Virulence. (2014) 5:36–44. doi: 10.4161/viru.25436
43. Pugin J. How tissue injury alarms the immune system and causes a systemic inflammatory response syndrome. Ann Intens Care. (2012) 2:27. doi: 10.1186/2110-5820-2-27
44. Dern K, Watts M, Werle B, Van Eps A, Pollitt C, Belknap J. Effect of delayed digital hypothermia on lamellar inflammatory signaling in the Oligofructose laminitis model. J Vet Int Med. (2017) 31:575–81. doi: 10.1111/jvim.14633
45. Costa RT de Araujo OR Brunialti MKC Assuncao MSC Azevedo LCP Freitas F . T helper type cytokines in sepsis: time-shared variance and correlation with organ dysfunction and hospital mortality. Braz J Infect Dis. (2019) 23:79–85. doi: 10.1016/j.bjid.2019.04.008
46. Boontham P, Chandran P, Rowlands B, Eremin O. Surgical sepsis: dysregulation of immune function and therapeutic implications. Surgeon. (2003) 1:187–206. doi: 10.1016/S1479-666X(03)80018-5
47. Lorente L, Martín M, Pérez-Cejas A, Barrios Y, Solé-Violán J, Ferreres J, et al. Association between interleukin-6 promoter polymorphism (-174 G/C), serum interleukin-6 levels and mortality in severe septic patients. Int J Mol Sci. (2016) 17:1861. doi: 10.3390/ijms17111861
48. Tanaka T, Narazaki M, Kishimoto T. Interleukin (IL-6) immunotherapy. Cold Spring Harbor Perspect Biol. (2018) 10:a028456. doi: 10.1101/cshperspect.a028456
49. Tanaka T, Narazaki M, Kishimoto T. Immunotherapeutic implications of IL-6 blockade for cytokine storm. Immunotherapy. (2016) 8:959–70. doi: 10.2217/imt-2016-0020
50. De Laat M, Van Eps A, McGowan C, Sillence M, Pollitt C. Equine laminitis: comparative histopathology 48 hours after experimental induction with insulin or alimentary oligofructose in standardbred horses. J Comp Pathol. (2011) 145:399–409. doi: 10.1016/j.jcpa.2011.02.001
51. Black SJ, Lunn DP, Yin C, Hwang M, Lenz SD, Belknap JK. Leukocyte emigration in the early stages of laminitis. Vet Immunol Immunop. (2006) 109:161–6. doi: 10.1016/j.vetimm.2005.08.017
52. Bailey S, Adair H, Reinemeyer C, Morgan S, Brooks A, Longhofer S, et al. Plasma concentrations of endotoxin and platelet activation in the developmental stage of oligofructose-induced laminitis. Vet Immunol Immunopathol. (2009) 129:167–73. doi: 10.1016/j.vetimm.2008.11.009
53. Zhu H, Tang Y, Ivanciu L, Centola M, Lupu C, Taylor FB, et al. Temporal dynamics of gene expression in the lung in a baboon model of E. coli sepsis. BMC Genom. (2007) 8:58. doi: 10.1186/1471-2164-8-58
54. Miller AC, Rashid RM, Elamin EM. The “T” in trauma: the helper T-cell response and the role of immunomodulation in trauma and burn patients. J Trauma Acute Care Surg. (2007) 63:1407–17. doi: 10.1097/TA.0b013e31815b839e
55. Scumpia PO, Moldawer LL. Biology of interleukin-10 and its regulatory roles in sepsis syndromes. Crit Care Med. (2005) 33:S468–71. doi: 10.1097/01.CCM.0000186268.53799.67
56. Paudel S, Baral P, Ghimire L, Bergeron S, Jin L, DeCorte JA, et al. CXCL1 regulates neutrophil homeostasis in pneumonia-derived sepsis caused by Streptococcus pneumoniae serotype 3. Blood. (2019) 133:1335–45. doi: 10.1182/blood-2018-10-878082
57. Bachmann MF, Kopf M, Marsland BJ. Chemokines: more than just road signs. Nat Rev Immunol. (2006) 6:159. doi: 10.1038/nri1776
58. Van Eps A, Leise B, Watts M, Pollitt C, Belknap J. Digital hypothermia inhibits early lamellar inflammatory signalling in the oligofructose laminitis model. Equine Vet J. (2012) 44:230–7. doi: 10.1111/j.2042-3306.2011.00416.x
59. Ferrer MD, Busquests-Cortes C, Capo X, Tejada S, Sureda A. Cyclooxygenase- 2 inhibitors as a therapeutic target in inflammatory diseases. Curr Med Chem. (2018) 25:3225–41. doi: 10.2174/0929867325666180514112124
60. Chun K-S, Surh Y-J. Signal transduction pathways regulating cyclooxygenase-2 expression: potential molecular targets for chemoprevention. Biochem Pharmacol. (2004) 68:1089–100. doi: 10.1016/j.bcp.2004.05.031
61. Blikslager AT, Yin C, Cochran AM, Wooten JG, Pettigrew A, Belknap JK. Cyclooxygenase expression in the early stages of equine laminitis: a cytologic study. J Vet Int Med. (2006) 20:1191–6. doi: 10.1111/j.1939-1676.2006.tb00721.x
62. Ejima K, Layne MD, Carvajal IM, Kritek PA, Baron RM, Chen Y-H, et al. Cyclooxygenase-2-deficient mice are resistant to endotoxin-induced inflammation and death. FASEB J. (2003) 17:1325–7. doi: 10.1096/fj.02-1078fje
63. Takatani Y, Ono K, Suzuki H, Inaba M, Sawada M, Matsuda N. Inducible nitric oxide synthase during the late phase of sepsis is associated with hypothermia and immune cell migration. Lab Investig. (2018) 98:629. doi: 10.1038/s41374-018-0021-z
64. Zeerleder S, Schroeder V, Hack CE, Kohler HP, Wuillemin WA. TAFI and PAI-1 levels in human sepsis. Thromb Res. (2006) 118:205–12. doi: 10.1016/j.thromres.2005.06.007
Keywords: bovine laminitis, pathogenesis, inflammation, real-time quantitative PCR, oligofructose
Citation: Ding JF, Li SC, Jiang LH, Li YP, Zhang XH, Song QZ, Hayat MA, Zhang JT and Wang HB (2020) Laminar Inflammation Responses in the Oligofructose Overload Induced Model of Bovine Laminitis. Front. Vet. Sci. 7:351. doi: 10.3389/fvets.2020.00351
Received: 06 February 2020; Accepted: 20 May 2020;
Published: 13 July 2020.
Edited by:
Francisco Javier Salguero, Public Health England, United KingdomReviewed by:
Fauna Leah Smith, University of California, Davis, United StatesAlessandra Piersigilli, University of Bern, Switzerland
Valeria Grieco, University of Milan, Italy
Copyright © 2020 Ding, Li, Jiang, Li, Zhang, Song, Hayat, Zhang and Wang. This is an open-access article distributed under the terms of the Creative Commons Attribution License (CC BY). The use, distribution or reproduction in other forums is permitted, provided the original author(s) and the copyright owner(s) are credited and that the original publication in this journal is cited, in accordance with accepted academic practice. No use, distribution or reproduction is permitted which does not comply with these terms.
*Correspondence: Hongbin Wang, hbwang1940@neau.edu.cn