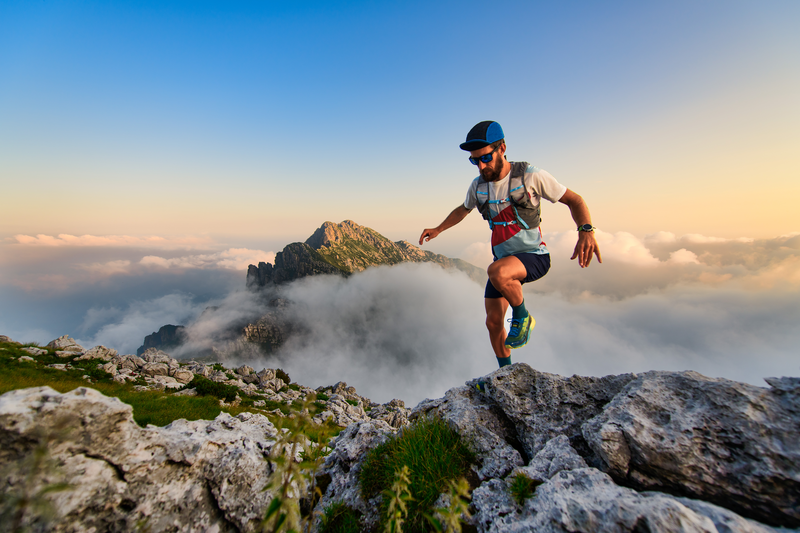
94% of researchers rate our articles as excellent or good
Learn more about the work of our research integrity team to safeguard the quality of each article we publish.
Find out more
ORIGINAL RESEARCH article
Front. Vet. Sci. , 30 April 2020
Sec. Veterinary Infectious Diseases
Volume 7 - 2020 | https://doi.org/10.3389/fvets.2020.00201
Endometritis is one of the main diseases which harm sheep husbandry. Astragalin and chlorogenic acid (CGA) are common active ingredients of traditional Chinese medicine (TCM) with immunoprotective, antioxidant, and anti-inflammatory properties. In the present study, sheep endometrial epithelium cells (SEECs) were successfully purified and identified, and the in vitro inflammation model of SEECs induced by Escherichia coli (E. coli) was successfully established. To explore the effect of astragalin and CGA on the inflammation induced by E. coli and its potential mechanism, six groups were set up, namely, group C, M, astragalin, CGA, BAY, and STR. Cells in group C were incubated with DMEM/F12 for 6 h, while cells in group M, astragalin, CGA, BAY, and STR were incubated with DMEM/F12, astragalin, CGA, BAY, and STR for 3 h, respectively, followed by E. coli infection at a multiplicity of infection (MOI) of 1 E. coli per cell for 3 h. Subsequently, the cells and the supernatant were collected to detect the expression of genes in the toll-like receptor 4 (TLR4)/nuclear factor-kappa B (NF-κB) pathway by ELISA, qPCR, and western blot. The results showed that E. coli could induce inflammation of SEECs in vitro, while astragalin and CGA could alleviate the inflammatory response induced by E. coli via inhibiting the activation of the TLR4/NF-κB signaling pathway, which provides a theoretical and experimental foundation for preventing sheep endometritis clinically.
With the rapid development of the Chinese economy and the improvement of living standards, the breeding industry gets more and more attention including sheep husbandry. However, the perinatal disease of sheep, especially endometritis, restricts the development of sheep husbandry, which results in a substantial economic loss (1). However, not all endometritis can cause injury to the organism; for instance, endometritis induced by breeding is a normal physiological reaction in the mare, and it is a necessary inflammatory response for the effective removal of contaminating bacteria and excess spermatozoa introduced into the uterus. Furthermore, in most cases, the endometritis resolves within 24–48 h, leaving the uterus clean and free of inflammation in time for the arrival of the conceptus as it migrates from the oviduct (2). But many female animals fail to resolve the endometritis and persistent endometritis results, which can cause sub-involution of uterus, reduced milk production, fertilization failure, infertility, and even systemic disease (3, 4). Persistent endometritis results from a response to the introduction of air, urine, semen, bacteria, fungus, and yeast into the uterus (2), with the leading cause being bacterial infection, particularly E. coli, Staphylococcus aureus, and Streptococcus agalactiae (5, 6). Lipopolysaccharide (LPS), a component of the cell wall in gram-negative bacteria, such as E. coli, could activate the toll-like receptor 4 (TLR4)/nuclear factor-kappa B (NF-κB) signaling pathway and lead to a strong inflammatory response. It will result in great damages to the organism if the inflammatory response persists (7). TLR4 mediates several processes in the inflammatory cascade, triggering inflammation through myeloid differentiation primary response gene 88 (MyD88)-dependent and MyD88-independent pathways. TLR4, which was activated by LPS, activates I kappa B kinase (IKKs) via a series of biochemical reactions, which degrades NF-κB inhibitory protein inhibitor kappa B (IκB). Afterward, NF-κB translocates into the nucleus and activates the expression of inflammatory genes (8, 9).
Astragalin (Supplementary Figure 1A) is a flavonoid isolated from persimmon leaves and green tea seeds which has been used for treating many diseases in traditional Chinese medicine (TCM) for many years (10). It has been demonstrated that astragalin could suppress the proliferation of hepatocellular carcinoma cells both in vitro and in vivo, and this result is associated with inhibition of hexokinase 2 through the upregulation of miR-125b and subsequent metabolic reprogramming (11). Astragalin could also alleviate IL-1β-induced inflammatory mediators via activating the peroxisome proliferator-activated receptor (PPAR)-γ, which subsequently inhibited IL-1β-induced NF-κB and mitogen-activated protein kinase (MAPK) activation (12). Chlorogenic acid (CGA) (Supplementary Figure 1B), a phenolic compound that has been identified in coffee, fruits, and vegetables, exerts antioxidant (13), anti-inflammatory (14), anticarcinogenic (15), and antibacterial (16) activities. Gao et al. reported that CGA could be used as a potential therapeutic compound for bovine mastitis due to its anti-inflammatory role by inhibiting NF-κB activation (17). However, there is no evidence of whether astragalin and CGA can alleviate the inflammatory response of endometritis induced by E. coli infection in sheep. Therefore, the present study aimed at creating an in vitro inflammation model of SEECs and evaluating the anti-inflammatory effect and potential mechanism of astragalin and CGA on E. coli-induced inflammation of SEECs.
Sheep uteri were obtained from a slaughterhouse in Zhonghua, Lanzhou, and brought to the lab with 37°C normal saline in 2 h. Endometrium from the cornua of the uterus was collected, which was shredded to a particle size of 1 mm3, followed by digestion with 0.1% collagenase for 3–6 h (18). A 0.15-mm cell strainer was used to remove undigested cell mass, followed by centrifuging at 1,000 rpm for 10 min. The cells were cultured with DMEM/F12 with 20% FBS, and the differential digestion method was used to purify the epithelial cells.
Cytokeratin 18 (CK18) is a characteristic protein of epithelial cells, so the immunofluorescence assay was conducted to detect the expression of CK18 for cell identification. Cells were planted into 24-well plates (4 × 105 cells per well) and were cultured in an incubator at 37°C overnight. The cells were fixed with 4% paraformaldehyde for 30 min and washed twice with PBS, followed by permeabilization with 0.5% Triton X-100 for 10 min and blocking with QuickBlock™ Blocking Buffer for Immunol Staining (Beyotime, Beijing, China) for 30 min. The cells were stained with cytokeratin 18 (1:200) overnight at 4°C, and a secondary antibody against mouse IgG (1:400) was added for 1 h at room temperature in the dark. PBS was used to replace CK18 in the negative control, and the rest remains unchanged. The cells were then stained with DAPI (Coolaber, Beijing, China) for 3 min in the dark and then photographed using a fluorescence inverted microscope (Olympus, Japan).
SEECs were seeded in 96-well culture plates at a density of 1 × 103, 5 × 103, 1 × 104, 5 × 104, 1 × 105, 2.5 × 105, and 5 × 105 cells per well and cultured for 1 h when the cells grew adherently to the wall. A 10-μl cell counting kit-8 (CCK-8) reagent (Solarbio, Beijing, China) was added and incubated at 37°C for 1.5 h. The optical density (OD) was measured at 450 nm using a microplate reader (Bio-Rad, USA). A standard curve was made with OD and cell quantity/well as ordinate and abscissa, respectively. Meanwhile, SEECs were seeded in 96-well culture plates at a density of 103 cells per well and cultured for 1 h when the cells grew adherently to the wall. Ten microliters of CCK-8 reagent was added into five wells and incubated at 37°C for 1.5 h. This process was repeated every 24 h. The OD was measured at 450 nm using a microplate reader. After that, the growth curve was made with OD and cell quantity/well as ordinate and abscissa, respectively.
The astragalin and CGA (Yuanye, Shanghai, China) were dissolved with dimethyl sulfoxide (DMSO) and diluted with DMEM/F12 (astragalin was diluted to 400, 200, 100 50, 25, and 12.5 μg/ml, and CGA was diluted to 200, 100, 50, 25, 12.5, and 6.25 μg/ml), respectively. BAY 11-7085 (MCE, USA), the inhibitor of NF-κB activation and phosphorylation of IκBα, was also dissolved with DMSO and diluted with DMEM/F12 to 200, 100, 50, 20, 10, 5, 1, 0.5, 0.25, and 0.125 μM. SEECs were seeded in 96-well culture plates at a density of 5 × 104 cells per well and cultured for 24 h. Cells were then exposed to a series of concentrations of astragalin, GA, and BAY at 37°C for 24 h. A 10-μl CCK-8 reagent was added and incubated at 37°C for 1.5 h. The OD was measured at 450 nm.
Escherichia coli (ATCC25922) was inoculated in 10-ml LB liquid medium for 18 h at 37°C with shaking at a speed of 180 rpm. SEECs were seeded in six-well culture plates at a density of 4 × 105 cells per well and cultured for 24 h. Cells were then exposed to E. coli at a series of multiplicities of infection (MOIs) (1:10, 1:1, 5:1, 10:1, and 100:1) and incubated at 37°C for 3 h. Changes of cellular morphology of SEECs in each well were analyzed, and apoptosis and necrocytosis of SEECs were tested using YF-Annexin V and PI Apoptosis Kit (US Everbright Inc., Suzhou, China). Total RNA was isolated, and qPCR was performed to detect the expression of NF-κB, IL-1β, and IL-6. The result was a selection criterion of the MOI of E. coli.
To evaluate the protective effect of astragalin and CGA on E. coli infection-induced inflammation of SEECs, six groups were set up, including groups C, M, astragalin, CGA, BAY, and STR. Cells in group C were incubated with DMEM/F12 for 6 h, while cells in groups M, astragalin, CGA, BAY, and STR were incubated with DMEM/F12, astragalin, CGA, BAY, and STR for 3 h, respectively, followed by E. coli infection at an MOI of one E. coli per cell for 3 h in the preexisting medium. Subsequently, the cells and the supernatant were collected to detect the expression of genes in the TLR4/NF-κB pathway by ELISA, qPCR, and western blot. Each in vitro experiment was repeated three times.
The total RNA was isolated from the cells with a TRIpure reagent (Bioteke, Beijing, China) according to the manufacturer's guide. Briefly, the cells were treated with RL lysis buffer and chloroform. After the removal of protein, the total RNA was dissolved in RNase-free water. The concentration of the total RNA was determined using a UV-Vis spectrophotometer Q5000 (Quawell, USA) at 260/280 nm. The first-strand complementary DNA (cDNA) was synthesized from 450 ng of total RNA using the PrimeScript™ RT reagent kit with gDNA Eraser (Takara, Japan). The synthesized cDNA was stored at −80°C until ready for use. qPCR was used to detect gene expression of TLR4, IKKβ, NF-κB, IL-1β, IL-6, IL-8, IL-12α, and TNF-α with the Light Cycler 96 System (Roche, Basel, Switzerland). Reaction mixtures consisted of the following: 10 μl of 2 × TB green premix (Takara, Japan), 0.8 μl of each primer (10 μM), 2 μl of cDNA, and 6.4 μl of RNase-free water. The PCR program for the eight genes and β-actin was one cycle at 95°C for 30 s, 40 cycles at 95°C for 5 s, annealing at 60°C for 30 s, and extension 72°C for 30 s. The primers used in the present study were designed with Primer Premier 5 software, synthesized by Sangon Biotech (Shanghai, China) and are listed in Table 1. The relative mRNA levels were calculated according to the 2−ΔΔCt method accounting for gene-specific efficiencies and were normalized to the mean expression of the reference gene β-actin.
Total protein was extracted with RIPA buffer (high) with PMSF and protein phosphatase inhibitor (all-in-one, 100×) (Solarbio, China). Proteins extracted were boiled for 10 min with loading buffer (Solarbio, China) and subjected to 10% SDS-PAGE under reducing conditions. The separated proteins were then transferred to PVDF membranes for 45 min (β-actin, pIκB, and IκB) or 90 min (TLR4, pNF-κB, and NF-κB) at 200 mA in a transfer apparatus (Bio-Rad, Philadelphia, USA). Membranes were blocked with 5% Bovine Serum Albumin (BSA) for 2 h at room temperature and incubated overnight at 4°C with diluted primary antibodies against TLR4 (1:1,000, Bioss, Beijing, China), IκB (1:1,000, CST), pIκB (1:1,000, CST), NF-κB (1:1,000, CST), and pNF-κB (1:500, Bioss) separately. The samples were then incubated with horseradish peroxidase (HRP)-conjugated secondary antibody against rabbit/mouse IgG (1:1,000, Bioss) for 40 min at room temperature. To verify equal loading of the samples, the membrane was incubated with a monoclonal β-actin antibody (1:1,000, Bioss,), followed by an HRP-conjugated goat anti-mouse IgG (1:1,000, Bioss) secondary antibody. The signal was detected with Amersham Imager 600 (General Electric Company, USA) and quantified by densitometry using ImageJ software. Subsequently, the densitometry of TLR4, pIκB, and pNF-κB was normalized to β-actin, IκB, and NF-κB, respectively.
The protein levels of TLR4 in cells and IL-1β, IL-6, IL-8, and TNF-α in the cell supernatant were measured by ELISA kits (Jingkang, Shanghai, China) according to the manufacturer's instructions. Briefly, when intracellular proteins were detected, cells were digested and made into cell suspension with PBS, followed by multigelation and centrifuging to obtain the supernatant. And when secretory proteins were detected, the culture supernatant was collected. Ten microliters of supernatant and 40-μl sample dilution were added into the 96-well plate, followed by addition of 100-μl HRP-conjugated reagent to each well and incubation at 37°C for 60 min. Fifty microliters of Chromogen Solution A and 50 μl of Chromogen Solution B were added to each well and incubated at 37°C for 15 min after washing the plate five times. Finally, 50 μl of stop solution was added, and the absorbance was detected at 450 nm with a microplate reader (Bio-Rad, USA). These steps follow the manufacturer's instructions strictly.
All statistical analyses were performed using the Statistical Package for the Social Sciences (SPSS) 17.0 software package, and all data were assessed using the one-way analysis of variance (ANOVA). The figures and graphs were prepared with GraphPad Prism version 7.0 for Windows. The differences of one group from the others were considered to be significant when P < 0.05.
The SEECs were successfully isolated and purified with the methods of collagenase and differential digestion, respectively. The passaged cells (Figure 1) were well attached and grew, appearing as oval shapes or pebble shapes with clear boundaries. The cells were further passaged up to the ninth generation, and there was no significant difference in cell morphology, attachment, or growth time between the ninth generation and the primary culture.
Figure 1. Morphology of the SEECs after purification. (A) The SEECs were well attached and grew, appearing as oval shapes or pebble shapes. (B) The SEECs were plump and rounded and closely arranged with clear boundaries.
To determine whether the epithelial marker protein, CK18, can be expressed in established SEECs, an indirect immunofluorescence assay was conducted using mouse monoclonal antibody that targets CK18. The result revealed that the SEECs were reactive with CK18-specific antibody and that the negative group showed no red fluorescence (Figure 2). We concluded from this observation that CK18 can be efficiently expressed in the cells we purified, which strongly confirms that the established cells are epithelial cells.
Figure 2. The identification of SEECs by CK18. (A) Cells incubating with CK18 and secondary antibody. (B) Cells incubating with PBS and secondary antibody (magnification ×200).
To determine the growth properties of the newly established SEECs, the growth curve was carried out at passage 9 of the cell. The standard curve and growth curve of SEECs were shown in Figures 3A,B. In Figure 3A, the R2 of the standard curve is 0.9921. While in Figure 3B, SEECs grew slowly in the first 2 days and achieved the logarithmic phase and plateau phase at the third and fifth days, respectively.
Figure 3. Standard curve and growth curve of SEECs. (A) Standard curve of SEECs. (B) Growth curve of SEECs.
To explore the appropriate concentration of the medicines in the present study, SEECs were treated with different doses of astragalin (12.5, 25, 50, 100, 200, and 400 μg/ml), CGA (6.25, 12.5, 25, 50, 100, and 200 μg/ml), and BAY (0.125, 0.25, 0.5, 1, 5, 10, 20, 50, 100, and 200 μM). In clinical treatment, the purpose of medication is not only to alleviate the symptoms of the disease but also to promote the recovery of the lesion, so that the drug effect will be better. Hence, the results indicate that the dose of 100 μg/ml of astragalin and 50 μg/ml of CGA significantly increased (P < 0.05) cell viability (Figure 4). As a result of this assay, we chose the dose of 100 μg/ml of astragalin, 50 μg/ml of CGA, and 5 μM of BAY to incubate SEECs to explore the effect of astragalin and CGA on E. coli-infected SEECs.
Figure 4. The cell viability assay result of astragalin, CGA, and BAY. (A) The cell viability assay result of incubating with astragalin for 24 h. (B) The cell viability assay result of incubating with CGA for 24 h. (C) The cell viability assay result of incubating with BAY for 24 h. Data are represented as the mean ± SD of three independent experiments with mixed SEECs from 15 sheep. Values with different lowercase letters were considered as statistically significant (P < 0.05). The accurate P values of this article were listed in Supplementary Tables 1–4.
The results of the in vitro inflammation model establishment were shown in Figures 5–7. As is shown in Figure 5, changes in cellular morphology gradually appeared as the MOI of E. coli increased, and significant changes of the cellular morphology were observed when the MOI of E. coli was 5:1, 10:1, and 100:1 where vacuolization, shrinkage, transparency, detachment, and even dead cells appeared.
Figure 5. The cell morphology at different MOIs. The SEECs were non-treated or treated with five different MOIs of E. coli for 3 h, and cell morphologic changes were observed. (A,a) The cell morphology of normal SEECs. The cells were orbicular-ovate, with the edge clear and attached well. (B,b) The cell morphology at the MOI of 1:10. The cells showed no significant changes compared with normal SEECs, but with a few cell vacuolization (arrows). (C,c) The cell morphology at the MOI of 1:1. The cells showed no significant changes compared with normal SEECs, but the cells were not smooth and a few cell vacuolization (arrows) were observed. (D,d) The cell morphology at the MOI of 5:1. The cells in red circles in D become flattened with the edge blurring, and obvious cell vacuolization (arrows), transparence (rectangle), and cell shrinkage (triangle) were observed in d. (E,e) The cell morphology at the MOI of 10:1. The cells were apparently shrunken (triangle), transparent (rectangle), and detached (bold arrows). (F,f) The cell morphology at the MOI of 100:1. Abundant cell shrinkage (triangle), transparence (rectangle), and detachment (bold arrows) were observed.
As is shown in Figure 6, the apoptosis and necrosis of SEECs were increased with the MOI of E. coli, and numerous apoptotic and necrotic cells appeared when the MOI of E. coli was 5:1, 10:1, and 100:1. This result was consistent with the result of the morphological changes of SEECs.
Figure 6. Apoptosis and necrosis of SEECs tested by YF-Annexin V and PI Apoptosis Kit. The SEECs were non-treated or treated with five different MOIs of E. coli for 3 h, followed by apoptosis and necrosis assays. Green fluorescence showed the apoptotic cells which were combined with YF-Annexin V, and red fluorescence showed the dead cells which were combined with PI. (A) Apoptosis and necrosis of SEECs in the non-infecting group. (B) Apoptosis and necrosis of SEECs infected by E. coli at the MOI of 1:10. (C) Apoptosis and necrosis of SEECs infected by E. coli at the MOI of 1:1. (D) Apoptosis and necrosis of SEECs infected by E. coli at the MOI of 5:1. (E) Apoptosis and necrosis of SEECs infected by E. coli at the MOI of 10:1. (F) Apoptosis and necrosis of SEECs infected by E. coli at the MOI of 100:1.
The relative mRNA expression of NF-κB, IL-1β, and IL-6 increased with a dose-dependent effect when MOI was below 5:1, and it is decreased gradually when MOI exceeded 5:1 (Figure 7). The relative mRNA expression of NF-κB was significantly increased (P < 0.05) when compared with that in group C at MOIs of 1:1 and 5:1. The relative mRNA expression of IL-1β and IL-6 was significantly increased (P < 0.05) when compared with that in group C at MOIs of 1:1, 5:1, 10:1, and 100:1. These results indicate that the MOI of E. coli at 1:1 was appropriate to establish the inflammation model of SEECs.
Figure 7. The relative mRNA expression of inflammatory cytokines induced by E. coli with different MOIs. The SEECs were non-treated or treated with five different MOIs of E. coli for 3 h, and the total RNA was isolated, followed by qPCR to detect the expression of NF-κB, IL-1β, and IL-6. (A) The relative mRNA expression of NF-κB induced by E. coli with different MOIs. (B) The relative mRNA expression of IL-1β induced by E. coli with different MOIs. (C) The relative mRNA expression of IL-6 induced by E. coli with different MOIs. Data are represented as the mean ± SD of three independent experiments with mixed SEECs from 15 sheep. Values with different lowercase letters were considered as statistically significant (P < 0.05).
As is shown in Figure 8, the mRNA expression of the genes (TLR4, IKKβ, NF-κB, IL-1β, IL-6, IL-8, IL-12α, and TNF-α) in the TLR4/NF-κB signaling pathway was significantly increased (P < 0.05) by about 45, 35, 160, 35, 230, 87, 77, and 36%, respectively, in group M compared with those in group C. Compared to the cells treated with E. coli only, the mRNA expression of IKKβ, NF-κB, IL-1β, IL-6, IL-8, IL-12α, and TNF-α was obviously reduced (P < 0.05) in cells pretreated with astragalin, CGA, and BAY for 3 h ahead of E. coli. But the mRNA expression of TLR4 in group BAY was significantly higher (P < 0.05) than that in groups C, astragalin, and CGA. Meanwhile, almost all the genes detected in group STR were increased (P < 0.05) compared with groups C, astragalin, and CGA.
Figure 8. The relative mRNA expression of genes in the TLR4/NF-κB pathway. The SEECs were pretreated for 3 h with various compounds (astragalin, CGA, BAY, and STR) and then exposed to E. coli for 3 h, and the mRNA expressions of TLR4, IKKβ, NF-κB, IL-1β, IL-6, IL-8, IL-12α, and TNF-α were detected by qPCR. (A) The relative mRNA expression of TLR4. (B) The relative mRNA expression of IKKβ. (C) The relative mRNA expression of NF-κB. (D) The relative mRNA expression of IL-1β. (E) The relative mRNA expression of IL-6. (F) The relative mRNA expression of IL-8. (G) The relative mRNA expression of IL-12α. (H) The relative mRNA expression of TNF-α. Data are represented as the mean ± SD of three independent experiments with mixed SEECs from 15 sheep. Values with different lowercase letters were considered as statistically significant (P < 0.05).
The protein bands of TLR4, pIκBα/IκBα, and pNF-κB/NF-κB, detected by western blot are shown in Figure 9A while the IntDens of TLR4/β-actin, pIκBα/IκBα, and pNF-κB/NF-κB are shown in Figures 9B–D, respectively. The expression of TLR4 in group M was significantly increased (P < 0.05) by about 34% compared with that in group C, and the expression of TLR4 in groups astragalin and CGA were significantly decreased (P < 0.05) compared with that in group M (Figure 9B). The expression of TLR4 in groups BAY and STR was significantly increased (P < 0.05) by about 23% and 21% compared with that in group C but was lower (P < 0.05) by about 8% and 15% than in group M. Also, the expression of TLR4 in groups BAY and STR was significantly higher (P < 0.05) than that in groups astragalin and CGA. The results in Figures 9C,D showed that the ratios of pIκBα/IκBα and pNF-κB/NF-κB in group M were greatly increased (P < 0.05) compared with that in group C by about 20 and 35%, respectively. Meanwhile, the ratios of pIκBα/IκBα and pNF-κB/NF-κB in groups astragalin, CGA, and BAY were obviously reduced (P < 0.05) compared with those in group M. Interestingly, the expression of TLR4 and the ratios of pIκBα/IκBα and pNF-κB/NF-κB in group astragalin were all remarkably decreased (P < 0.05) compared to group STR.
Figure 9. The relative protein expression of genes in the TLR4/NF-κB pathway. The SEECs were pretreated for 3 h with various medicines (astragalin, CGA, BAY, and STR) and then exposed to E. coli for 3 h, and the protein levels of TLR4, pIκB, IκB, pNF-κB, and NF-κB were determined by western blot analysis. (A) The protein bands of TLR4, pIκB, IκB, pNF-κB, NF-κB, and β-actin detected by western blot. (B) The IntDen of TLR4/β-actin. (C) The IntDen of pIκBα/IκBα. (D) The IntDen of pNF-κB/NF-κB. Data are represented as the mean ± SD of three independent experiments with mixed SEECs from 15 sheep. Values with different lowercase letters were considered as statistically significant (P < 0.05).
As is shown in Figure 10, the protein expression of TLR4 and inflammatory cytokines (IL-1β, IL-6, IL-8, and TNF-α) in the TLR4/NF-κB signaling pathway was significantly increased (P < 0.05) by about 39, 40, 35, 27, and 14%, respectively, in group M compared with those in group C. Compared with that in group M, the protein expression of the genes was obviously reduced (P < 0.05) when cells were pretreated with astragalin and CGA for 3 h. The protein expression of IL-1β, IL-6, IL-8, and TNF-α in group BAY was significantly decreased (P < 0.05) when compared with group M, while the protein expression of TLR4 showed no significant difference (P > 0.05). Meanwhile, the protein levels of TLR4, IL-1β, IL-6, IL-8, and TNF-α in group STR also showed no significant difference (P > 0.05) compared with those in group M but were significantly higher (P < 0.05) than those in group C.
Figure 10. The protein expression of genes in the TLR4/NF-κB pathway. The SEECs were pretreated for 3 h with various medicines (astragalin, CGA, BAY, and STR) and then exposed to E. coli for 3 h, and the protein levels of TLR4, IL-1β, IL-6, IL-8, and TNF-α were determined by ELISA. (A) The protein expression of TLR4. (B) The protein expression of IL-1β. (C) The protein expression of IL-6. (D) The protein expression of IL-8. (E) The protein expression of TNF-α. Data are represented as the mean ± SD of three independent experiments with mixed SEECs from 15 sheep. Values with different lowercase letters were considered as statistically significant (P < 0.05).
Endometritis is characterized by inflammation of the endometrium and has seriously hindered the development of the breeding industry (19). Although the clinical application of antibiotics in the treatment of endometritis has some efficacy, it has led to the emergence of drug-resistant strains (20). Bacteria are the main cause of endometritis, and one of the most common bacteria is E. coli (21, 22). It has been reported that 15–20% of dairy cow endometritis is involved with bacterial infection, indicating that preventing and treating endometritis induced by E. coli are of great significance (23). TCM including compound and monomer keeps getting more attention due to its multiple biological activities, such as anti-inflammation, antioxidant, and immunity enhancement, especially its non-tolerance to bacteria and virus (24, 25). A lot of studies showed that TCM was effective in preventing organisms from a bacterial infection (26). For these reasons, many researchers are focused on the positive effect of TCM on preventing and treating endometritis of dairy cows, but few studies aimed at the preventive and therapeutic effects of TCM on sheep endometritis. In this study, we isolated and purified SEECs and created an in vitro model of sheep endometritis via infecting SEECs with E. coli to explore the protective effect of astragalin and CGA and its possible protective mechanisms. The results showed that astragalin and CGA could inhibit the progress of the inflammatory response of SEECs induced by E. coli infection via a mechanism related to the inhibition of the TLR4/NF-κB signaling pathway.
E. coli is a gram-negative bacterium, which contains the special component LPS in the cytoderm. LPS induces inflammatory response via activating the TLR4/NF-κB signaling pathway (27). Mammalian TLR4 is the signal-transducing receptor activated by the bacterial LPS, and LPS first binds to the cluster of differentiation-14 (CD14) receptor, which then transfers them to TLR4. In the MyD88-dependent pathway, TLR4 combines with MyD88 via TIR after being activated by LPS. MyD88 recruits IRAK4, thereby allowing the association of IRAK1. Then, IKK is activated after a series of complicated biochemical reactions. On the other hand, Tir domain-containing adaptor inducing interferon-beta (TRIF) and Trif-related adaptor molecule (TRAM) link TLR4 to pathways that lead to the activation of TNF receptor-associated factor 6 (TRAF6) in the MyD88-independent pathway, followed by the activation of IKK. In both the MyD88-dependent and MyD88-independent pathways, the NF-κB, which was inhibited by combining with IκB, is released following the activated IKK phosphorylating IκB. Afterward, the activated NF-κB transfers into the nucleus after phosphorylation binds to specific sites and initiates the transcription of pro-inflammatory factors, including IL-1β, IL-6, IL-10, IL-12, and necrocytosis TNF-α (8, 9, 28, 29). However, E. coli infection at an improper MOI will induce obvious apoptosis (30, 31). Therefore, the in vitro inflammation model of SEECs in this study was created by infecting SEECs with E. coli, and the cellular morphology, apoptosis and necrocytosis, and the mRNA expression of NF-κB, IL-1β, and IL-6 were used as the evaluation criteria to choose the proper MOI of E. coli. As a result, the expressions of NF-κB, IL-1β, and IL-6 in SEECs were increased after infection with E. coli, and there was a dose-dependent effect when the MOI was beneath 5:1. But when the MOI of E. coli achieved 5:1, 10:1, and 100:1, the cell morphology changed remarkably. For instance, the cell became flattened, the edge blurred, and evident cell vacuolization, transparence, cell shrinkage, and detachment were observed. Meanwhile, numerous apoptotic and necrotic cells appeared when the MOI of E. coli was 5:1, 10:1, and 100:1. In conclusion, when the MOI of E. coli achieved 1:1, it induced an inflammatory response without numerous apoptosis and necrocytosis. This result was consistent with the conclusion of a previous study which revealed that an appropriate MOI of E. coli could significantly induce inflammatory response rather than cell death (32).
With the development of TCM, many researchers focus on the mechanism of different natural herbs and different active ingredients including flavonoids (33), phenols (34), alkaloids (35), and polysaccharides (36). Deng et al. (37) have demonstrated that inhibiting the progress of inflammation could effectively alleviate the injury to the animals induced by endometritis. Jiang et al. (20) suggested that natural herbs have remarkable curative effects in the treatment of endometritis via inhibiting the progress of inflammation. It is reported that treatment with astragalin suppressed inflammatory response by regulating NF-κB and mitogen-activated protein kinase (MAPK) signaling pathways in leptospirosis-infected uterine and endometrium epithelial cells of mice (38). Another study also revealed that astragalin exerts anti-inflammatory properties possibly via the inactivation of TLR4-mediated NF-κB and MAPK signaling pathways in LPS-stimulated mMECs (39). In addition, some researchers demonstrated the hepatoprotective nature of CGA by attenuating the pro-inflammatory and apoptotic mediators and improving antioxidant competence in hepatic tissues (40). In the preliminary experiment, we have revealed that astragalin and CGA showed no observable antibacterial activity at the concentrations of 100 and 50 μg/ml, respectively (Supplementary Figure 2) and induced no significant changes (P > 0.05) of inflammatory response compared with untreated cells (Supplementary Figure 3). And in the present study, E. coli infection induced inflammatory response via activating the TLR4/NF-κB signaling pathway, and astragalin and CGA inhibited the inflammatory progress by means of reducing the expression of TLR4 (Figure 11). This mechanism was different from the anti-inflammation effect of BAY, which inhibited the inflammatory progress by inhibiting the activation of IκB and NF-κB. However, whether there were other genes in TLR4/NF-κB involved with the anti-inflammation effect of astragalin and CGA still needs further research. Furthermore, although STR could inhibit the E. coli proliferation, it could not alleviate the inflammatory progress at 3 h after E. coli infection.
Figure 11. Scheme summarizing the mechanism of the protective effect of astragalin and CGA on E. coli-induced endometritis. E. coli infection activates the TLR4/NF-κB signaling pathway. Activated NF-κB can be translocated from the cytosol to the nucleus, where it activates the transcription of genes that include IL-1β, IL-6, IL-8, IL-12, and TNF-α. Astragalin and CGA could inhibit the inflammatory response by blocking TLR4 activation.
In summary, our data demonstrated that astragalin (100 μg/ml) and CGA (50 μg/ml) possessed anti-inflammatory effects in E. coli-induced inflammation of SEECs by inhibiting the TLR4-mediated activation of the NF-κB signaling pathway. And the proper mechanism was blocking the activation of TLR4. Accordingly, these findings demonstrated that astragalin and CGA can serve as anti-inflammatory agents combined with antibacterial agents in sheep endometritis induced by E. coli infection.
All datasets generated for this study are included in the article/Supplementary Material.
XH and SY came up with and designed the study. XH, JH, XZ, and TZ performed the experiments. XH, HH, and QL performed the statistical analysis. XH, YX, RN, and MW wrote the manuscript. YP, RN, and YC revised the manuscript.
This research was funded by the National Key Research and Development Project (No. 2017YFD0502200), Industrial support and guidance project of Gansu Provincial Department of Education (No. 2019C-03), and Innovation ability improvement project of Gansu Provincial Department of Education (No. 2019B-081).
The authors declare that the research was conducted in the absence of any commercial or financial relationships that could be construed as a potential conflict of interest.
The Supplementary Material for this article can be found online at: https://www.frontiersin.org/articles/10.3389/fvets.2020.00201/full#supplementary-material
1. Duricic D, Valpotic H, Samardzija M. Prophylaxis and therapeutic potential of ozone in buiatrics: current knowledge. Anim Reprod Sci. (2015) 159:1–7. doi: 10.1016/j.anireprosci.2015.05.017
2. Woodward EM, Troedsson MH. Inflammatory mechanisms of endometritis. Equine Vet J. (2015) 47:384–9. doi: 10.1111/evj.12403
3. Gobikrushanth M, Salehi R, Ambrose DJ, Colazo MG. Categorization of endometritis and its association with ovarian follicular growth and ovulation, reproductive performance, dry matter intake, and milk yield in dairy cattle. Theriogenology. (2016) 86:1842–9. doi: 10.1016/j.theriogenology.2016.06.003
4. Koyama T, Omori R, Koyama K, Matsui Y, Sugimoto M. Optimization of diagnostic methods and criteria of endometritis for various postpartum days to evaluate infertility in dairy cows. Theriogenology. (2018) 119:225–32. doi: 10.1016/j.theriogenology.2018.07.002
5. Sokkar SM, Kubba MA, Al-Augaidy F. Studies on natural and experimental endometritis in ewes. Vet Pathol. (1980) 17:693–8. doi: 10.1177/030098588001700606
6. Han J, Wang M, Pan Y, Hu X, Zhang X, Cui Y, et al. Establishment and evaluation of multiplex PCR for detection of main pathogenic bacteria of endometritis in Tibetan sheep. Chin J Biotech. (2020) 36:1–12. doi: 10.13345/j.cjb.190365
7. Zhang F, Lu S, Jin S, Chen K, Li J, Huang B, et al. Lidanpaidu prescription alleviates lipopolysaccharide-induced acute kidney injury by suppressing the NF-κB signaling pathway. Biomed Pharmacother. (2018) 99:245–52. doi: 10.1016/j.biopha.2018.01.059
8. Barcelos RP, Bresciani G, Rodriguez-Miguelez P, Cuevas MJ, Soares FA, Barbosa NV, et al. Diclofenac pretreatment effects on the toll-like receptor 4/nuclear factor kappa B-mediated inflammatory response to eccentric exercise in rat liver. Life Sci. (2016) 148:247–53. doi: 10.1016/j.lfs.2016.02.006
9. Baek H, Shin HJ, Kim JJ, Shin N, Kim S, Yi MH, et al. Primary cilia modulate TLR4-mediated inflammatory responses in hippocampal neurons. J Neuroinflammation. (2017) 14:189. doi: 10.1186/s12974-017-0958-7
10. Kotani M, Matsumoto M, Fujita A, Higa S, Wang W, Suemura M, et al. Persimmon leaf extract and astragalin inhibit development of dermatitis and IgE elevation in NC/Nga mice. J Allergy Clin Immunol. (2000) 106(1 Pt 1):159–66. doi: 10.1067/mai.2000.107194
11. Li W, Hao J, Zhang L, Cheng Z, Deng X, Shu G. Astragalin reduces hexokinase 2 through increasing miR-125b to inhibit the proliferation of hepatocellular carcinoma cells in vitro and in vivo. J Agric Food Chem. (2017) 65:5961–72. doi: 10.1021/acs.jafc.7b02120
12. Ma Z, Piao T, Wang Y, Liu J. Astragalin inhibits IL-1beta-induced inflammatory mediators production in human osteoarthritis chondrocyte by inhibiting NF-kappaB and MAPK activation. Int Immunopharmacol. (2015) 25:83–7. doi: 10.1016/j.intimp.2015.01.018
13. Rui L, Xie M, Hu B, Zhou L, Saeeduddin M, Zeng X. Enhanced solubility and antioxidant activity of chlorogenic acid-chitosan conjugates due to the conjugation of chitosan with chlorogenic acid. Carbohydr Polym. (2017) 170:206–16. doi: 10.1016/j.carbpol.2017.04.076
14. Liang N, Kitts DD. Role of chlorogenic acids in controlling oxidative and inflammatory stress conditions. Nutrients. (2015) 8:16. doi: 10.3390/nu8010016
15. Yan Y, Liu N, Hou N, Dong L, Li J. Chlorogenic acid inhibits hepatocellular carcinoma in vitro and in vivo. J Nutr Biochem. (2017) 46:68–73. doi: 10.1016/j.jnutbio.2017.04.007
16. Lou Z, Wang H, Zhu S, Ma C, Wang Z. Antibacterial activity and mechanism of action of chlorogenic acid. J Food Sci. (2011) 76:M398–403. doi: 10.1111/j.1750-3841.2011.02213.x
17. Gao R, Yang H, Jing S, Liu B, Wei M, He P, et al. Protective effect of chlorogenic acid on lipopolysaccharide-induced inflammatory response in dairy mammary epithelial cells. Microb Pathog. (2018) 124:178–82. doi: 10.1016/j.micpath.2018.07.030
18. Li X, Li Z, Hou D, Zhao Y, Wang C, Li X. The bovine endometrial epithelial cells promote the differentiation of trophoblast stem-like cells to binucleate trophoblast cells. Cytotechnology. (2016) 68:2687–98. doi: 10.1007/s10616-016-9994-x
19. Gilbert RO, Shin ST, Guard CL, Erb HN, Frajblat M. Prevalence of endometritis and its effects on reproductive performance of dairy cows. Theriogenology. (2005) 64:1879–88. doi: 10.1016/j.theriogenology.2005.04.022
20. Jiang K, Guo S, Yang J, Liu J, Shaukat A, Zhao G, et al. Matrine alleviates Staphylococcus aureus lipoteichoic acid-induced endometritis via suppression of TLR2-mediated NF-kappaB activation. Int Immunopharmacol. (2019) 70:201–7. doi: 10.1016/j.intimp.2019.02.033
21. Shao C, Wang H, Wang X, Jiang S, Sun J, Song H, et al. Characterization of inflammatory responses by cervical cytology, cytokine expression and ultrastructure changes in a goat subclinical endometritis model. J Vet Med Sci. (2017) 79:197–205. doi: 10.1292/jvms.16-0096
22. Socha BM, Łada P, Szczepanska AA, Łupicka M, Korzekwa AJ. The influence of experimentally induced endometritis on the PPAR expression profile in the bovine endometrium. Theriogenology. (2018) 122:74–83. doi: 10.1016/j.theriogenology.2018.09.013
23. Piersanti RL, Zimpel R, Molinari PCC, Dickson MJ, Ma Z, Jeong KC, et al. A model of clinical endometritis in Holstein heifers using pathogenic Escherichia coli and Trueperella pyogenes. J Dairy Sci. (2019) 102:2686–97. doi: 10.3168/jds.2018-15595
24. Li HN, Wang CY, Wang CL, Chou CH, Leu YL, Chen BY. Antimicrobial effects and mechanisms of ethanol extracts of seeds against and methicillin-resistant. Foodborne Pathog Dis. (2019) 16:573–80. doi: 10.1089/fpd.2018.2595
25. Ma Y, Cong W, Huang H, Sun L, Mai AH, Boonen K, et al. Identification of fukinolic acid from Cimicifuga heracleifolia and its derivatives as novel antiviral compounds against enterovirus A71 infection. Int J Antimicrob Agents. (2019) 53:128–36. doi: 10.1016/j.ijantimicag.2018.07.014
26. Zhang Y, Li W, Zou L, Gong Y, Zhang P, Xing S, et al. Metabonomic study of the protective effect of Fukeqianjin formula on multi-pathogen induced pelvic inflammatory disease in rats. Chin Med. (2018) 13:61. doi: 10.1186/s13020-018-0217-6
27. Wu H, Dai A, Chen X, Yang X, Li X, Huang C, et al. Leonurine ameliorates the inflammatory responses in lipopolysaccharide-induced endometritis. International Immunopharmacology. (2018) 61:156–61. doi: 10.1016/j.intimp.2018.06.002
28. Beutler B. Inferences, questions and possibilities in Toll-like receptor signalling. Nature. (2004) 430:257–63. doi: 10.1038/nature02761
29. Li H, Kim WJ, Jiang J, Lee SH, Youn HS, Moon EY, et al. MyD88-dependent Toll-like receptor signaling is required for murine macrophages response to IS2. Int Immunopharmacol. (2011) 11:1578–83. doi: 10.1016/j.intimp.2011.05.017
30. Bielaszewska M, Rüter C, Kunsmann L, Greune L, Bauwens A, Zhang W, et al. Enterohemorrhagic Escherichia coli hemolysin employs outer membrane vesicles to target mitochondria and cause endothelial and epithelial apoptosis. PLoS Pathog. (2013) 9:e1003797. doi: 10.1371/journal.ppat.1003797
31. Priya A, Kaur K, Bhattacharyya S, Chakraborti A, Ghosh S. Cell cycle arrest and apoptosis induced by enteroaggregative Escherichia coli in cultured human intestinal epithelial cells. J Med Microbiol. (2017) 66:217–25. doi: 10.1099/jmm.0.000405
32. Law RJ, Gur-Arie L, Rosenshine I, Finlay BB. In vitro and in vivo model systems for studying enteropathogenic Escherichia coli infections. Cold Spring Harb Perspect Med. (2013) 3:a009977. doi: 10.1101/cshperspect.a009977
33. Xiao J. Dietary flavonoid aglycones and their glycosides: which show better biological significance? Crit Rev Food Sci Nutr. (2017) 57:1874–905. doi: 10.1080/10408398.2015.1032400
34. Serino A, Salazar G. Protective role of polyphenols against vascular inflammation, aging and cardiovascular disease. Nutrients. (2018) 11:53. doi: 10.3390/nu11010053
35. Li N, Xu Q, Liu Q, Pan D, Jiang Y, Liu M, et al. Leonurine attenuates fibroblast-like synoviocyte-mediated synovial inflammation and joint destruction in rheumatoid arthritis. Rheumatology. (2017) 56:1417–27. doi: 10.1093/rheumatology/kex142
36. Hu X, Zhang R, Xie Y, Wang H, Ge M. The protective effects of polysaccharides from Agaricus blazei murill against cadmium-induced oxidant stress and inflammatory damage in chicken livers. Biol Trace Elem Res. (2017) 178:117–26. doi: 10.1007/s12011-016-0905-y
37. Deng C, Liu J, Wang G, Ma L, Xie C, Wang X, et al. A novel small molecule,. (E)-5-(2,4-di-tert-butyl-6-((2,4-dioxothiazolidin-5-ylidene)methyl)phenyl)-5'-methyl-7,7'-dimethoxy-4,4'-bibenzo[d][1,3]dioxole-5,5'-dicarboxylate. (7k), alleviates the development of D-galactosamine/lipopolysaccharide-induced acute liver failure by inhibiting macrophage infiltration and regulating cytokine expression. J Pharmacol Exp Ther. (2012) 341:146–55. doi: 10.1124/jpet.111.189498
38. Zhang W, Lu X, Wang W, Ding Z, Fu Y, Zhou X, et al. Inhibitory effects of emodin, thymol, and astragalin on leptospira interrogans-induced inflammatory response in the uterine and endometrium epithelial cells of mice. Inflammation. (2017) 40:666–75. doi: 10.1007/s10753-017-0513-9
39. Kim MS, Kim SH. Inhibitory effect of astragalin on expression of lipopolysaccharide-induced inflammatory mediators through NF-kappaB in macrophages. Arch Pharm Res. (2011) 34:2101–7. doi: 10.1007/s12272-011-1213-x
Keywords: astragalin, chlorogenic acid, E. coli, endometritis, TLR4/NF-κB
Citation: Hu X, Wang M, Pan Y, Xie Y, Han J, Zhang X, Niayale R, He H, Li Q, Zhao T, Cui Y and Yu S (2020) Anti-inflammatory Effect of Astragalin and Chlorogenic Acid on Escherichia coli-Induced Inflammation of Sheep Endometrial Epithelium Cells. Front. Vet. Sci. 7:201. doi: 10.3389/fvets.2020.00201
Received: 19 December 2019; Accepted: 26 March 2020;
Published: 30 April 2020.
Edited by:
Dirk Werling, Royal Veterinary College (RVC), United KingdomReviewed by:
Pierre Germon, Institut National de la Recherche Agronomique (INRA), FranceCopyright © 2020 Hu, Wang, Pan, Xie, Han, Zhang, Niayale, He, Li, Zhao, Cui and Yu. This is an open-access article distributed under the terms of the Creative Commons Attribution License (CC BY). The use, distribution or reproduction in other forums is permitted, provided the original author(s) and the copyright owner(s) are credited and that the original publication in this journal is cited, in accordance with accepted academic practice. No use, distribution or reproduction is permitted which does not comply with these terms.
*Correspondence: Sijiu Yu, c2lqaXV5QDEyNi5jb20=
Disclaimer: All claims expressed in this article are solely those of the authors and do not necessarily represent those of their affiliated organizations, or those of the publisher, the editors and the reviewers. Any product that may be evaluated in this article or claim that may be made by its manufacturer is not guaranteed or endorsed by the publisher.
Research integrity at Frontiers
Learn more about the work of our research integrity team to safeguard the quality of each article we publish.