- 1College of Animal Science and Technology, Shandong Agricultural University, Taian, China
- 2Sino-German Cooperative Research Centre for Zoonosis of Animal Origin Shandong Province, College of Animal Science and Technology, Shandong Agricultural University, Taian, China
- 3Shandong Provincial Key Laboratory of Animal Biotechnology and Disease Control and Prevention, Shandong Agricultural University, Taian, China
- 4Shandong Provincial Engineering Technology Research Center of Animal Disease Control and Prevention, Shandong Agricultural University, Taian, China
Aspergillus fumigatus is a ubiquitous pathogen in poultry farms, causing aspergillosis in chickens. To study the pathogenicity of A. fumigatus, 14-days-old chickens were infected with fungal conidia (2 × 107 CFU/mL) via thoracic intra-air sacs inoculation. The clinical symptoms, gross and histopathological lesions, and fungal load in the lungs were examined. Additionally, the mRNAs of Toll like receptors (TLR) and pro-inflammatory cytokines were evaluated by quantitative PCR to explore the immune responses induced by A. fumigatus. The results showed that overt depression, ruffled feathers, and dyspnea were observed in the infected chickens as early as 3 days post infection (dpi). Eleven out of 25 infected chickens died from 5 to 9 dpi, and A. fumigatus could also be reisolated from the infected lung. Histopathological examination revealed obvious airsacculitis and pneumonia, characterized by inflammatory cell infiltration (heterophils and macrophages), and granulomatous lesions in the lung. The mRNA expressions of TLR1 and TLR2 were upregulated in the lung and spleen, and most pro-inflammatory cytokines including IL-1β, Cxcl-8, TNF-α, IL-12, and IFN-γ were increased in both the lung and spleen during the tested period, suggesting that the innate immune responses were triggered by A. fumigatus infection, and these cytokines participated in the inflammatory responses against A. fumigatus. These results indicate that A. fumigatus infection by thoracic intra-air sacs inoculation can cause severe respiratory damage in chickens, activate TLR1 and TLR2 mediated immune responses, and elicit large expression of pro-inflammatory cytokines such as IL-1β, Cxcl-8, and IFN-γ. These data will help further understanding of the pathogenesis and immune responses of A. fumigatus infection in the chicken.
Introduction
Avian Aspergillosis is an infectious fungal disease characterized mainly by respiratory symptoms. This disease has been reported worldwide in a large number of wild and domestic birds. Almost all birds are susceptible to aspergillosis, such as chickens (1, 2), turkeys (3), ducks (4, 56), pigeons (5), quails (6), and many wild birds (7, 8). Aspergillus fumigatus is one of the most common etiologic agent of aspergillosis. This filamentous fungus is a ubiquitous, opportunistic pathogen that produces large amounts of small-sized conidia in the air. The clinical signs of susceptible poultry differ from flock to flock, age to age and also to the exposure level. Young birds appear to be more susceptible to acute aspergillosis which is characterized by dyspnoea, gasping, and inappetence, resulting in high morbidity and mortality, thus inducing significant economic losses in poultry (9). The chronic form of aspergillosis is sporadic, which generally occurs in older birds, especially breeders in poultry, and causes lesser mortality (10).
Nowadays, aspergillosis is still prevalent in chickens. Sultana et al. detected a total of 912 sick and dead commercial broilers collected from 20 farms at Chittagong district of Bangladesh in 2013. The overall incidence of aspergillosis was found to be 6.14% (11). Since 2007, outbreaks of chicken airsacculitis have occurred in most areas of China, leading to enormous economic losses, feed-borne A. fumigatus is the main cause for this respiratory disease (12). Additionally, co-infection of A. fumigatus and other pathogens has also been reported clinically (13). Experimental aspergillosis has been studied in chickens for many years (14–16). Recently, Thierry et al. also reported that the conventional JA657 broilers were more susceptible to A. fumigatus than the White Leghorn PA12 layers, indicating the lineage of chicken plays an important role in the pathogenicity of A. fumigatus (17). The immunopathogenesis of A. fumigatus infection in chickens has yet to be fully elucidated.
Host innate immunity is essential for the control of A. fumigatus. Many studies regarding the immune response to A. fumigatus in human beings and mice have been conducted (18–20), but the immune responses of chickens infected with A. fumigatus have not been fully explored. Multiple pattern recognition receptors (PRRs) are involved in recognizing A. fumigatus, especially Toll-like receptors (TLR), and C-type lectin receptors (CLR). Furthermore, different components of fungi cell walls can be sensed by different PRRs. It has been confirmed that TLR2 and TLR4 are involved in sensing fungal DNA and zymosan (21, 22). TLR4-deficient mice have a higher susceptibility to A. fumigatus compared with control mice (23). TLR2 signaling is essential for responses to A. fumigatus in both mouse and human cells (24). C-type lectin receptor, dectin-1, recognizes β-Glucan of A. fumigatus in mice alveolar macrophages, and is required for the induction of alveolar macrophage pro-inflammatory responses to A. fumigatus (25). Upon recognition, the downstream immune responses mediated by PRRs are triggered, and pro-inflammatory cytokines, such as TNF-α, IL-1β, IL-6, and chemokine Cxcl-8, can be induced to participate in the defense against A. fumigatus (26, 27). Conversely, A. fumigatus escapes the host immune via modulation or suppression of the relevant signaling pathways (28, 29). As is well-known, the physiologic and anatomic characteristics of chicken respiratory tract are significantly different from that of mammal, and innate immune system is different, such as chicken TLR21 can sense CpG DNA instead of mammalian TLR9 (30). Thus, the pathogenicity and the immune responses of A. fumigatus in chickens may also be different.
In the present study, the aim is to investigate the pathogenicity and innate immune responses of A. fumigatus in chickens challenged by intra-air sac inoculation. The mortality, clinical signs, gross lesions, and pathological lesions of the infected chickens were observed. Moreover, the expression profiles of innate immune-related genes at 1, 3, and 5 days post infection (dpi) were measured to evaluate the defense against A. fumigatus. These results will provide a better understanding of the pathogenesis of A. fumigatus and type of immune responses induced in the chicken.
Materials and Methods
Strain
Aspergillus fumigatus strain (CCCCMIDA1) was purchased from the Institute of Dermatology and Venereology of the Chinese Academy of Medical Sciences and grown on Potato Dextrose Agar (PDA) medium (Solarbio, Beijing, China) for 5–7 days at 37°C according to other report (31). The cultures were washed using sterile phosphate buffer saline (PBS) to collect conidia, and the suspension was filtered through sterile gauze to remove hyphae. The filtered suspension was transferred to an autoclaved centrifuge tube and centrifuged for 15 min at 1,500 × g at room temperature. A. fumigatus was re-suspended with sterile PBS to a concentration of 2 × 107 colony forming unit (CFU)/mL.
Experimental Design
Ten-days-old specific pathogen free (SPF) White Leghorn chickens were purchased from the Poultry Institute, Shandong Academy of Agricultural Science, and housed in isolators with a 12 h photoperiod and provided sufficient water and feed without antibiotics throughout the experiment. Temperature was maintained between 21 and 25°C and relative humidity was 30–40%. When chickens were 14 days old, they were randomly divided into two groups, with 25 in each group. In the infected group, 0.1 mL (2 × 106 CFU) of conidia suspension was inoculated into the right thoracic air sacs of each chicken as previous study (15). Chickens in the control group were inoculated in the same manner with 0.1 mL of sterile PBS. Clinical signs, gross and microscopic lesions, and mortality were observed. Aside from the dead chickens, three live chickens were randomly selected from each group, and the right tissues samples (right lung and air sacs) were collected for histopathological analysis and detection of innate immune-related genes mRNA at 1, 3, and 5 dpi. The rest of the chickens were observed for clinical signs for 9 days and then euthanized at the end of the experiment.
Lesion Scores of Lungs and Air-Sacs
At necropsy, gross lesions in lungs and air sacs were observed. Lung and spleen were collected and fixed with 4% paraformaldehyde solution to make paraffin sections, which were stained with hematoxylin and eosin for histological examination. The severity of gross lesions and histopathological lesions of infected air sacs and lungs were observed and scored, using the criteria reported elsewhere (12). Briefly, based on the thickness, turbidity, and inflammatory exudate, the severity of gross lesions of air sacs was scored on a scale of 0 to 4: 0, normal, clean, transparent; 1, slightly thickened and turbid or individual local yellow white exudate; 2, yellow white exudate in a few areas of the air sacs; 3, the majority of the air sacs are covered with yellow-white caseous exudate, thick; 4, thick yellow-white exudates are obvious on the thoracic cavity and abdominal cavity. Similarly, gross lesions of the lung were scored on a scale of 0 to 4: 0, normal, faint red; 1, slight edema and hyperaemia; 2, moderate edema, focal necrosis in a few areas of the lung; 3, yellow white necrosis in half areas of the lung; and 4, necrosis in most aeras of the lung, serious congestion.
The scoring criteria for microscopic lesions of the infected lung were as follows. 0, none; 1, edema and hyperaemia of the alveolar wall; 2, inflammatory cell infiltration in the limited areas of the lung; 3, granuloma, large amount of inflammatory cell infiltration, slight necrosis in the lung; and 4, granuloma and severe cell necrosis in the lung.
Aspergillus fumigatus Load in the Lung of the Infected Chickens
A plate count method was adopted to measure the load of A. fumigatus in the lung of the infected chicken. In brief, the infected lungs were collected under sterile condition. The 0.1 g sample was mixed with 900 μL of sterile PBS, and the mixture was ground into homogenate. The homogenate of the lung tissue underwent a 10 times dilution until the concentration reached 10−4. The 100 μL sample of each dilution was selected to add into the PDA medium, and then cultured for 24 h at 37°C. Plates with between 30 and 300 colonies were considered to be effective. The CFU was counted according to the following formula, and each dilution was performed in triplicate.
CFU/g = the number of colonies in each plate × the dilution ratio × 102.
Quantitative Real-Time PCR
Total RNA was extracted from the lung and spleen (0.1 g) using the TRIzol Reagent (Takara, Dalian, China) according to the manufacturer's instructions. The RNA concentration was measured and 1 μg RNA was reverse transcribed with HiScript II QRT SuperMix for qPCR (+gDNA wiper) (Vazyme, Nanjing, China). The synthesized cDNA was stored at −20°C until analysis. Primers (Table 1) required in the study were designed using the Primer 3 online software (http://bioinfo.ut.ee/primer3-0.4.0/) based on the published GenBank sequence or refer to other study (32). Quantitative real-time PCR (qPCR) was prepared in 20 μL according to the operation instruction (Vazyme, Nanjing, China) and performed using Roche LightCycler 96 (Roche, Basel, Switzerland). qPCR was performed at 95°C for 30 s, followed by 40 cycles of 95°C for 10 s, and 60°C for 34 s. The dissociation curves were identified at the final step. All samples were amplified in triplicate.
Statistical Analysis
The relative expression of the target genes in the infected and control groups was calculated with the 2−ΔΔCt method and expressed as the mean fold changes. The data were presented in terms of relative mRNA expressed as means ± standard deviations (SD). One-way ANOVA method with Duncan's multiple range test was used for evaluating data using GraphPad Prism 5 software (GraphPad Software Inc. USA). P < 0.05 were considered to be significant, and values <0.01 were highly significant.
Results
Clinical Signs and Gross Lesions of the Infected Chickens
In the A. fumigatus-infected group, two chickens died at 5 dpi, three died at 6 and 7 dpi, respectively, two at 8 dpi and one died at 9 dpi. A total of 11 chickens infected with A. fumigatus died at 5–9 dpi (Figure 1). Most infected chickens showed obvious clinical signs, including dyspnea, depression, ruffled feathers, and dyskinesia as early as 3 dpi. No chickens died in the control group throughout the study.
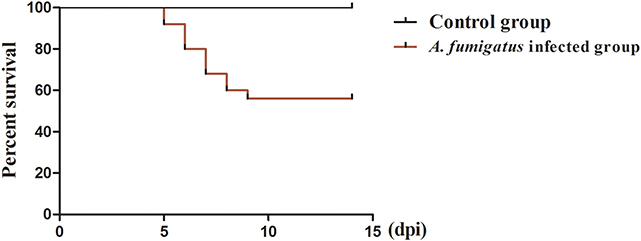
Figure 1. Survival curve after infection with Aspergillus fumigatus. Infected chicken was infected with 2 × 106 CFU condia via intra-right thoracic air sac. Control chicken was inoculated with 0.1 ml PBS via the same route. In the Aspergillus fumigatus infected group, a total of 11 chickens died from 5 to 9 dpi, respectively. No chickens died in the control group throughout the experiment.
The primary organs with gross lesions were the air sacs and lungs, especially the right tissues, the lesions gradually increased with the extension of infection (Table 2). Slightly turbid and local yellow white exudate were observed in two chicken at 1 dpi (Supplementary Figure 1A), and two chickens showed yellow white caseous exudate in a few areas of the air sacs at 3 dpi (Supplementary Figure 1B). However, these lesions increased at 5 dpi. The majority of air sacs were covered with yellow white caseous necrosis exudate in all three infected chickens (Supplementary Figure 1C) compared to that of the control group (Supplementary Figure 1D). For lungs, slight edema, and hyperaemia were observed at 1 dpi (Supplementary Figure 1E), and moderate edema and focal necrosis of the lung were observed at 3 dpi (Supplementary Figure 1F). Diffuse edema and miliary yellow white necrosis were observed in lungs of two infected chickens at 5 dpi (Supplementary Figure 1G). The control lungs were faint red without any lesions (Supplementary Figure 1H). In addition, mild lesions could be observed in other organs including the liver, small intestine, spleen, and skin in the infected group at 3 or 5 dpi.
Histopathological Lesions of Lungs
The lesions scoring of the right lungs of A. fumigatus infected chickens is shown in Table 3. The development of the lesions was gradually becoming more severe from 1 to 5 dpi. The lesions were characterized by inflammatory cell infiltration and granuloma. As shown in Figure 2A, a large number of inflammatory exudates were observed in the parabronchi as early as 1 dpi, including some heterophilic granulocytes; at 3 dpi, the typical granulomatous lesions emerged, characterized by a center of necrotic cells and some fungal elements surrounded by a cliff of heterophils, epithelioid cells, macrophages, multinucleate giant cells, and lymphocytes. Phagocytised fungal elements are observed in the eosinophilic cytoplasm of multinucleated cells (Figure 2B). At 5 dpi, more extensive lesions caused by small granuloma coalescence resulted in parabronchial obliteration with necrotic material, such as degenerated heterophils, and exfoliated epithelial cells (Figure 2C). Normal lung morphology was observed in the control group (Figure 2D).
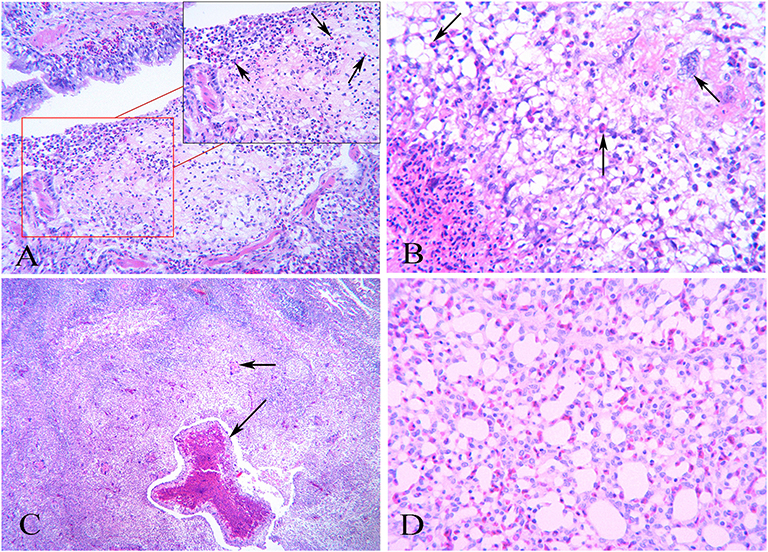
Figure 2. Histopathology of the lungs after infection with Aspergillus fumigatus. Right lungs were sampled in infected and control chickens. (A) At 1 dpi, inflammatory cell infiltration in the parabronchi. Heterophils can be seen (as indicated by the arrow); (B) At 3 dpi, obvious granulomatous inflammation. Arrows indicate inflammatory cells such as heterophils and multinucleated giant cells surrounding the central necrotic area; (C) At 5 dpi, progressive inflammation lesions characterized by small granuloma coalescence in the parabronchi (as indicated by the arrow) and massive inflammatory cell infiltration; (D) The normal histology structure of chicken lungs in the control group.
Aspergillus fumigatus Load in the Lung
To determine whether the lung lesions were resulted from A. fumigatus infection, the right lungs were collected to measure the fungus load, as the chickens were inoculated via the right thoracic air sacs. As shown in Figure 3, the load of fungus was 5.90 × 104 CFU/g as early as 1 dpi, and reached the peak at 3 dpi, with a value as high as 6.75 × 105 CFU/g. Then the load showed a decreasing trend, and reduced to 4.50 × 105 CFU/g at 5 dpi. These results indicated that A. fumigatus was the cause of these lesions.
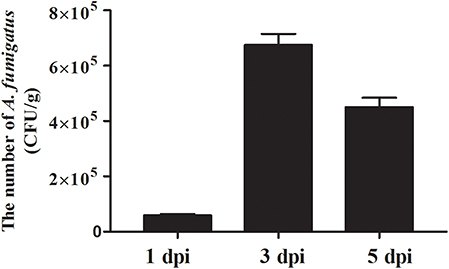
Figure 3. Pathogen load after infection with Aspergillus fumigatus. Right lungs were sampled in infected and control chickens (3 chickens per group). Aspergillus fumigatus load was measured using the plate counting method and expressed as means CFU per gr of tissue ± SD (n = 3).
Expression of TLR Genes in the Lung and Spleen
In order to determine the expression of TLR genes after A. fumigatus infection, the expressions of TLR1, TLR2, TLR4, and TLR21 were detected in the lung and spleen at 1, 3, and 5 dpi. As shown in Figure 4A, in the lung, the expressions of the TLR1 and TLR2 were upregulated at 1 dpi, the expression peaked at 3 dpi, with the fold change of the TLR2 mRNA being the highest (6.83-fold, P < 0.01; Figure 4A), followed by TLR1 (5.25-fold) and TLR4 (1.93-fold). In the spleen, only the expression of TLR1 upregulated compared to the control group at 1 dpi. TLR1 and TLR2 transcripts were significantly increased by 3.04- and 2.70-fold at 3 dpi, respectively (P < 0.05; Figure 4B). The expressions of TLR4 and TLR21 has no significantly change (Figure 4B). Collectively, these data indicated that TLR genes were modulated by A. fumigatus, especially TLR1 and TLR2, which might be involved in the recognition of A. fumigatus.
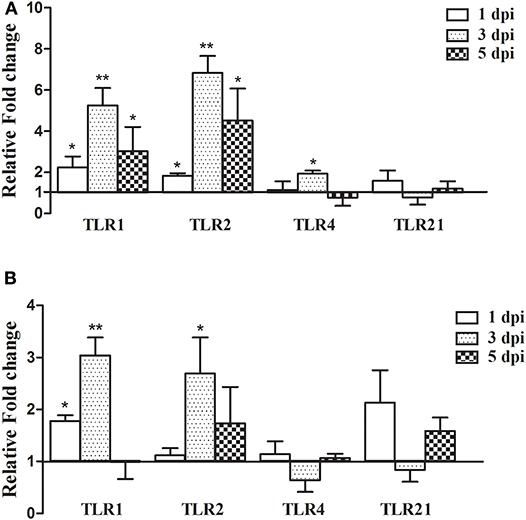
Figure 4. TLR gene expression in the lungs and spleen after infection with Aspergillus fumigatus. Spleen and right lungs were sampled at 1, 3, and 5 dpi. Gene expressions in infected chickens were expressed as fold change compared to the control group [means ± SD (n = 3)]. β-actin and GAPDH were used as reference genes. (A,B) were the expression of TLR gene in the lungs and spleen, respectively. The Y-axis indicates that the relative fold changes of the target gene expression in the Aspergillus fumigatus group vs. those in the control group. *p < 0.05, **p < 0.01.
Expression of Cytokine Genes in the Lung and Spleen
To better understand the expression of the downstream pro-inflammatory cytokines in chickens infected with A. fumigatus, IL-1β, IL-2, IL-6, Cxcl-8, TNF-α, IL-12, IL-18, and IFN-γ were examined. As shown in Figure 5A, the expressions of most cytokines were upregulated in the lung. At 1dpi, IL-1β, IL-6, Cxcl-8, TNF-α, IL-12, and IL-18 increased significantly. In particular at 3 dpi, the fold changes of the IL-1β, Cxcl-8, TNF-α, and IFN-γ mRNA expression were highly significant with highest value (P < 0.01), and the expressions were decreased at 5 dpi. The expression of IL-2 was downregulated at 1 dpi, and then upregulated by 3.32-fold (P < 0.01) at 5 dpi. IL-12 and IL-18 expressions increased significantly by 5.08- and 5.71-fold, respectively at 1 dpi, and then decreased in the following 2 days.
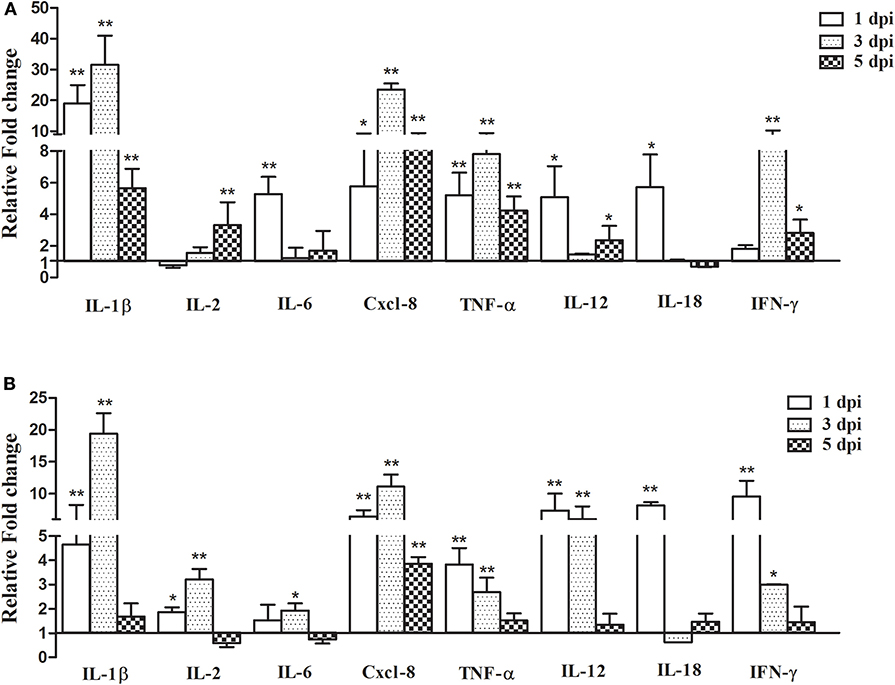
Figure 5. Pro-inflammatory cytokine gene expression in the lungs and spleen after infection with Aspergillus fumigatus. Spleen and right lungs were sampled at 1, 3, and 5 dpi. Gene expressions in infected chickens were expressed as fold change compared to the control group [means ± SD (n = 3)]. β-actin and GAPDH were used as reference genes. (A,B) were the expression of cytokine gene in the lungs and spleen, respectively. The Y-axis indicates that the relative fold changes of the target gene expression in the Aspergillus fumigatus group vs. those in the control group. *p < 0.05, **p < 0.01.
In the spleen, the expressions of IL-1β, IL-2, Cxcl-8, TNF-α, IL-12, and IFN-γ were increased at 1 and 3 dpi, but the upregulation decreased at 7 dpi except for IL-2. Most cytokines transcripts reached the highest value at 1 or 3 dpi (Figure 5B). For example, at 3 dpi, IL-1β and Cxcl-8 increased by 19.39- and 11.12-fold, respectively (P < 0.01), and the expression levels of TNF-α, IL-12, IL-18, and IFN-γ were highest at 1 dpi, increased by 3.83-, 7.36-, 8.16-, and 9.57-fold, respectively.
Discussion
Aspergillus fumigatus, mainly present in mildewed feedstuff and contaminating ambiant air, is an opportunistic pathogen for poultry inducing respiratory diseases and immunosuppression. The conidia (2.0–3.0 μm in diameter) are easily inhaled and deposited in the lower respiratory tract (33). In the current study, right thoracic intra-air sacs injection was adopted as the inoculation route to infect the 14-days-old SPF White Leghorn chickens with A. fumigatus conidia (2 × 106 CFU per chicken). Eleven out of 25 infected chickens died, and most chickens infected with A. fumigatus showed overt clinical signs, such as depression, dyspnea, and ruffled feathers. In contrast, the aerosol-challenged layer chickens belonging to White Leghorn lineage showed resistance to this fungus (17). The mechanisms of induction and the type of immunity are different when pathogens by-pass the upper respiratory tract, which would determine the level of resistance or susceptibility to a pathogen. Considering the different routes of inoculation, aerosol infection can quickly activate the mucosal immunity of chickens' upper respiratory tract and thus inducing resistance fungal infection. However, the intra-air sacs inoculation route bypass the upper airways and their associated defense mechanisms. Consequently, A. fumigatus infection can be established by this route in the chicken. Indeed a large number of A. fumigatus was reisolated from the infected lungs indicated the successful infection. The lesions of the infected chickens induced by this route were mostly compatible with those found in the field cases, that are characterized by pneumonia and airsacculitis (34, 35).
The respiratory tract is an essential infection route of A. fumigatus. In our experiment, the necropsy analysis demonstrated that the severe gross lesions were mainly on the air sacs and lungs, though the small intestine, liver, and spleen were also involved. The apparent yellowish-white caseous exudate and necrosis foci were observed in the air sacs and lungs of the infected chickens, especially the right lungs and air sacs which showed more severe lesions. Further histopathological analysis revealed substantial inflammatory cell infiltration in the lung at 1 dpi. Subsequently, typical granulomas lesions were observed in the lung, with a central core of necrotic cells and fungal elements, and a rim of the epithelioid cells, macrophages, heterophils, and multinucleate giant cells. These gross and microscopic lesions of the infected chickens can also be observed in other birds infected with A. fumigatus, including pigeon (36), gosling (37), and quail (38).
Aspergillus fumigatus infection caused extensive infiltration of inflammatory cells containing heterophils and macrophages as early as 1 dpi in chickens, indicating that substantial conidia can lead to hyperacute infection of aspergillosis and elicit the innate immune responses. In the pulmonary diseases, pulmonary macrophages play a central role in protecting against A. fumigatus and are essential for regulating the pulmonary innate immune response to fungal infection (39). It has been reported that the load of A. fumigatus increased in alveolar macrophage-deficient mice (40). In addition, neutrophils are important cells to defense against various pathogens including Aspergillus in the lung (41). The ability of neutrophils to efficiently kill A. fumigatus hyphae in vivo has been demonstrated by the real-time visualization in the zebrafish (42). These results suggested that macrophages and heterophils are required for A. fumigatus infection in chickens.
To better understand the pathogenesis of A. fumigatus in chickens, it is necessary to explore the interaction between host and pathogen. Since the host innate immune response is essential for defending against A. fumigatus in the early infection, the mRNA expressions of TLR and pro-inflammatory cytokines were measured in this study. Multiple PRRs are involved in sensing fungi during infection. TLR including TLR1, TLR2, TLR4, and TLR9 are crucial for the recognition of fungal cell wall components and the resultant antifungal responses (28, 43, 44). Moreover, it is demonstrated that the TLR associated MyD88 signal pathway is required for controlling fungi in mice (45). In the present study, the expressions of TLR1 and TLR2 were significantly upregulated in the chicken infected lungs and spleen, especially the TLR2 mRNA, which was increased by 6.83-fold in the lungs at 3 dpi, which may indicate that both were involved in the recognition of A. fumigatus in chickens (46, 47). The activation of PRRs can trigger the production of a large number of downstream pro-inflammatory cytokines (48). Th1-type cytokines, such as IL-1, IL-12, TNF-α, IFN-γ, and some chemokines including MIP-1 and Cxcl-8 play an important role in antifungal infection (27). In this study, the expressions of most pro-inflammatory cytokines, such as IL-1β, Cxcl-8, IL-12, TNF-α, and IFN-γ were increased, meaning they likely facilitated the inflammatory response to A. fumigatus in chickens. Recently, Li et al. reported that A. fumigatus infection induced inflammatory responses characterized by the increased production of IL-1 and IL-12 in chicken macrophage cell line HD-11, and exposure of A. fumigatus-infected macrophages to T-2 toxin further upregulated the expression of IL-1L, IL-6, IL-12, and IL-18 (49). The upregulated expression of IL-1L and IL-12 in infected chickens was compatible with the A. fumigatus-induced production of these cytokines in mouse alveolar macrophage (50), but IL-18 change was unconsistent with that in mouse lung tissue (51). It is known that macrophages not only phagocytize pathogens, but they can also regulate the immune response and secrete cytokines, such as TNF-α and IFN-γ. It has been reported that A. fumigatus extract differentially regulated CD8+ T cells expansion accompanied by differentiation into IFN-γ-producing cytotoxic cells to promote host immunity, but had no effect on CD4+ T cells response (52). In the current study, the production of TNF-α and IFN-γ was significantly upregulated in infected chickens, it may act as a stimulator to induce classically macrophages and heterophils, destruct intracellular A. fumigatus and further promote a local Th1 environment (53). Cxcl-8 recruits neutrophils to the sites of inflammation and mediates the release of antimicrobial peptides (10). In A. fumigatus-infected chickens, Cxcl-8 expression reached the maximum value at 3 dpi, since the resistance to aspergillosis is dependent on heterophils (45), these data suggested that Cxcl-8 could recruit heterophils to fight against A. fumigatus infection in chickens, which is consistent with histopathological examination of a large number of heterophils in the lungs. However, heterophils functions as a double-edged sword, on the one hand, they are essential in acute inflammatory responses of A. fumigatus infection, but on the other hand, the excessive release of oxidants and proteases from heterophils may result in tissues injury.
On the whole, although the immune responses to A. fumigatus has been activated in chickens, they may not efficiently prevent the early establishment of infection in this study. As a result, many chickens died from A. fumigatus infection in this study. Part of the reason may be the inoculation method and dose, and part may be due to the fact that conidia are cytotoxic to macrophages (54), and the number of fungal conidia exceed the killing capacity of the host immunity, leading to intracellular germination, colonization, and disease, moreover excessive pathogen replication may further exacerbate deleterious inflammation. Additionally, considering the roles played by humoral factors in the host response to Aspergillus (55), further studies will explore the adaptive immune response to A. fumigatus.
Conclusions
In summary, the typical clinical symptoms and histopathological lesions were reproduced after A. fumigatus infection by thoracic intra-air sacs injection in chickens, with active recruitment of macrophages and heterophils. A. fumigatus can trigger TLR mediated innate immune responses, leading to the massive production of IL-1e, TNF-α, Cxcl-8, and IFN-γ etc. pro-inflammatory cytokines in chickens.
Data Availability Statement
All datasets generated for this study are included in the article/Supplementary Material.
Ethics Statement
These animal experiments were approved by the Shandong Agricultural University Animal Care and Use Committee (SDAUA-2015-012) and performed according to the approved guidelines.
Author Contributions
ZC and ML carried out the experiments and wrote the manuscript. YW performed the experiments and analyzed the data. TC designed the experiments. YC and NL designed the experiments, reviewed the manuscript, and approved the submission.
Funding
This work was funded by Shandong Double Tops Program; and the Project of Natural Science Foundation of Shandong Province (ZR2011HM032); Shandong Province Major Application of Agricultural Technology Innovation Projects Research and Integrated Application of Comprehensive Control Technology for Major Animal Diseases and Zoonoses affecting Animal Health and Safety (SD2019XM006).
Conflict of Interest
The authors declare that the research was conducted in the absence of any commercial or financial relationships that could be construed as a potential conflict of interest.
Supplementary Material
The Supplementary Material for this article can be found online at: https://www.frontiersin.org/articles/10.3389/fvets.2020.00143/full#supplementary-material
Supplementary Figure 1. Gross lesions of air sacs and lungs infected with Aspergillus fumigatus. (A) At 1 dpi, slight thickness, turbidity and a small amount of yellow white exudate in air sac; (B) At 3 dpi, yellow white exudate increased in air sac; (C) At 5 dpi, a large amount of yellow white caseous exudate in air sac; (D) Clean and transparent in normal air sac; (E) At 1 dpi, focal edema and hemorrhage in the lung; (F) At 3 dpi, the lesions size increased, with edema and hemorrhage in the lung; (G) At 5 dpi, yellow white necrosis in the majority of lung; (H) Normal lung.
References
1. Akan M, Haziroglu R, Ilhan Z, Sareyyüpoglu B, Tunca R. A case of aspergillosis in a broiler breeder flock. Avian Dis. (2002) 46:497–501. doi: 10.1637/0005-2086(2002)046[0497:ACOAIA]2.0.CO;2
2. Martin MP, Pecelunas Bouck K, Helm J, Dykstra MJ, Wages DP, Barnes HJ. Disseminated aspergillus flavus infection in broiler breeder pullets. Avian Dis. (2007) 51:626–31. doi: 10.1637/0005-2086(2007)51[626:DAFIIB]2.0.CO;2
3. Okwara N. Aspergillosis in turkeys: a review. IOSR J Agr Vet Sci. (2016) 9:40–1. doi: 10.9790/2380-0906024041
4. Dyar P, Fletcher O, Page R. Aspergillosis in turkeys associated with use of contaminated litter. Avian Dis. (1984) 28:250–5. doi: 10.2307/1590149
5. Beernaert LA, Pasmans F, Haesebrouck F, Martel A. Modelling Aspergillus fumigatus infections in racing pigeons (Columba livia domestica). Avian Pathol. (2008) 37:545–9. doi: 10.1080/03079450802382280
6. Ahamad DB, Selvaraj J, Prasath NB, Sasikala M. Pathomorphology of aspergillosis in a Japanese quail. Indian Vet J. (2017) 94:85–6.
7. Pazhanivel N, Saahithya R, Thangapandiyan M, Venkata G, Rao S, Sridhar K. Pulmonary aspergillosis in a seventeen-day old ostrich chick (Struthio camelus). J Entomol Zool Stud. (2018) 6:553–5.
8. Gulcubuk A, Erdogan-Bamac O, Metiner K, Ozturk GY, Ozgur Y, Haktanir D. A case of pulmonary aspergillosis in white storks. J Hell Vet Med Soc. (2018) 69:1004–9. doi: 10.12681/jhvms.18021
9. Sajid M, Khan I, Rauf U. Aspergillus fumigatus in commercial poultry flocks, a serious threat to poultry industry in Pakistan. J Anim Pl Sci. (2006) 16:79–81.
10. Femenia F, Fontaine JJ, Lair-Fulleringer S, Berkova N, Huet D, Towanou N, et al. Clinical, mycological and pathological findings in turkeys experimentally infected by Aspergillus fumigatus. Avian Pathol. (2007) 36:213–9. doi: 10.1080/03079450701332337
11. Sultana S, Rashid SMH, Islam MN, Ali MH, Islam MM, Azam MG. Pathological investigation of avian aspergillosis in commercial broiler chicken at Chittagong district. Int J Innov Appl Stud. (2015) 10:366–76.
12. Chu J, Zhang Q, Zuo ZH, Elashram S, Guo YX, Zhao P, et al. Co-infection of chlamydia psittaci with H9N2, ORT and Aspergillus fumigatus contributes to severe pneumonia and high mortality in SPF chickens. Sci Rep. (2017) 7:13997. doi: 10.1038/s41598-017-14519-1
13. Cai LM, Wang YM, Wang GH, Cai YM, Cheng ZQ. Identification and characterization of natural concurrent avian leukosis virus subgroup J and Aspergillus flavus infection in commercial layer chickens. Vet Arhiv. (2014) 84:279–289.
14. O'Meara DC, Chute H. Aspergillosis experimentally produced in hatching chicks. Avian Dis. (1959) 3:404–6. doi: 10.2307/1587580
15. Van JC. Antifungal activity of enilconazole on experimental Aspergillosis in chickens. Avian Dis. (1983) 27:36–42. doi: 10.2307/1590369
16. Fadl EA, Abu EA, el Mubarak AK. Experimental aspergillosis in young chicks. Rev Elev Med Vet Pays Trop. (1984) 37:437–41.
17. Thierry S, Durand B, Melloul E, Tafani JP, Wang DY, Deville M, et al. Assessment of Aspergillus fumigatus pathogenicity in aerosol-challenged chickens (Gallus gallus) belonging to two lineages. Comp Immunol Microbiol Infect Dis. (2013) 36:379–85. doi: 10.1016/j.cimid.2013.01.003
18. Zhang YT, Wu JY, Xin ZT, Wu XY. Aspergillus fumigatus triggers innate immune response via NOD1 signaling in human corneal epithelial cells. Exp Eye Res. (2014) 127:170–8. doi: 10.1016/j.exer.2014.07.025
19. Lemieszek MK, Dutkiewicz J, Golec M, Chilosi M, Skórska C, Huaux F, et al. Age influence on mice lung tissue response to Aspergillus fumigatus chronic exposure. Ann Agric Environ Med. (2015) 22:69–75. doi: 10.5604/12321966.1141371
20. Stanzani M, Orciuolo E, Lewis R, Kontoyiannis DP, Martins SLR, John LSS, et al. Aspergillus fumigatus suppresses the human cellular immune response via gliotoxin-mediated apoptosis of monocytes. Blood. (2005) 105:2258–65. doi: 10.1182/blood-2004-09-3421
21. van de Veerdonk FL, Kullberg BJ, van der Meer JW, Gow NA, Netea MG. Host-microbe interactions: innate pattern recognition of fungal pathogens. Curr Opin Microbiol. (2008) 11:305–12. doi: 10.1016/j.mib.2008.06.002
22. Romani L. Immunity to fungal infections. Nat Rev Immunol. (2011) 11:275–88. doi: 10.1038/nri2939
23. Bellocchio S, Montagnoli C, Bozza S, Gaziano R, Rossi G, Mambula SS, et al. The contribution of the Toll-like/IL-1 receptor superfamily to innate and adaptive immunity to fungal pathogens in vivo. J Immunol. (2004) 172:3059–69. doi: 10.4049/jimmunol.172.5.3059
24. Mambula SS, Sau K, Henneke P, Golenbock DT, Levitz SM. Toll-like receptor (TLR) signaling in response to Aspergillus fumigatus. J Biol Chem. (2002) 277:39320–26. doi: 10.1074/jbc.M201683200
25. Steele C, Rapaka RR, Metz A, Pop SM, Williams DL, Gordon S, et al. The beta-glucan receptor dectin-1 recognizes specific morphologies of Aspergillus fumigatus. PLoS Pathog. (2005) 1:e42. doi: 10.1371/journal.ppat.0010042
26. Stojanovic I, Mirkov I, Kataranovski M, Glamoclija J, Stosic-Grujicic S. A role for macrophage migration inhibitory factor in protective immunity against Aspergillus fumigatus. Immunobiology. (2011) 216:1018–27. doi: 10.1016/j.imbio.2011.03.005
27. Grazziutti ML, Rex JH, Cowart RE, Anaissie EJ, Ford A, Savary CA. Aspergillus fumigatus conidia induce a Th1-Type cytokine response. J Infect Dis. (1997) 176:1579–83. doi: 10.1086/514157
28. Chai LY, Kullberg BJ, Vonk AG, Warris A, Cambi A, Latgé JP, et al. Modulation of Toll-like receptor 2 (TLR2) and TLR4 responses by Aspergillus fumigatus. Infect Immun. (2009) 77:2184–92. doi: 10.1128/IAI.01455-08
29. Chai LY, Vonk AG, Kullberg BJ, Verweij PE, Verschueren I, van Der Meer JW, et al. Aspergillus fumigatus cell wall components differentially modulate host TLR2 and TLR4 responses. Microbes Infect. (2011) 13:151–9. doi: 10.1016/j.micinf.2010.10.005
30. Keestra AM, de Zoete MR, Bouwman LI, van Putten JP. Chicken TLR21 is an innate CpG DNA receptor distinct from mammalian TLR9. J Immunol. (2010) 185:460–7. doi: 10.4049/jimmunol.0901921
31. Khoufache K, Puel O, Loiseau N, Delaforge M, Rivollet D, Coste A, et al. Verruculogen associated with Aspergillus fumigatus hyphae and conidia modifies the electrophysiological properties of human nasal epithelial cells. BMC Microbiol. (2007) 7:5. doi: 10.1186/1471-2180-7-5
32. Rohde F, Schusser B, Hron T, Farkašová H, Plachý J, Härtle S, et al. Characterization of chicken tumor necrosis factor-α, a long missed cytokine in birds. Front Immunol. (2018) 9:605. doi: 10.3389/fimmu.2018.00605
33. Munir MT, Rehman ZU, Shah MA, Umar S. Interactions of Aspergillus fumigatus with the respiratory system in poultry. Worlds Poult Sci J. (2017) 73:321–36. doi: 10.1017/S0043933917000022
34. Kunkle RA, Rimler RB. Pathology of acute aspergillosis in turkeys. Avian Dis. (1996) 40:875–86. doi: 10.2307/1592312
35. Kunkle RA, Sacco RE. Susceptibility of convalescent turkeys to pulmonary aspergillosis. Avian Dis. (1998) 42:787–790. doi: 10.2307/1592716
36. Tokarzewski S, Ziolkowska G, Lopuszynski W, Nozdryn-Plotnicki Z. Aspergillus fumigatus infection in a pigeon flock. Bull Vet Inst Pulawy. (2007) 51:563–7.
37. Beytut E, Ozcan K, Erginsoy S. Immunohistochemical detection of fungal elements in the tissues of goslings with pulmonary and systemic aspergillosis. Acta Vet Hung. (2004) 52:71–84. doi: 10.1556/AVet.52.2004.1.8
38. Gümüşsoy K, Uyanik F, Atasever A, Çam Y. Experimental Aspergillus fumigatus infection in quails and results of treatment with itraconazole. J Vet Med. (2004) 51:34–8. doi: 10.1046/j.1439-0450.2003.00720.x
39. Murthy S, Larson-Casey JL, Ryan AJ, He C, Kobzik L, Carter AB. Alternative activation of macrophages and pulmonary fibrosis are modulated by scavenger receptor, macrophage receptor with collagenous structure. FASEB J. (2015) 29:3527–36. doi: 10.1096/fj.15-271304
40. Bhatia S, Fei M, Yarlagadda M, Qi Z, Akira S, Saijo S, et al. Rapid host defense against Aspergillus fumigatus. involves alveolar macrophages with a predominance of alternatively activated phenotype. PLoS ONE. (2011) 6:e15943. doi: 10.1371/journal.pone.0015943
41. Park SJ, Mehrad B. Innate immunity to Aspergillus species. Clin Microbiol Rev. (2009) 22:535–51. doi: 10.1128/CMR.00014-09
42. Knox BP, Huttenlocher A, Keller NP. Real-time visualization of immune cell clearance of Aspergillus fumigatus spores and hyphae. Fungal Genet Biol. (2017) 105:52–4. doi: 10.1016/j.fgb.2017.05.005
43. Rubino I, Coste A, Roy DL, Roger T, Jaton K, Boeckh M, et al. Species-specific recognition of Aspergillus fumigatus by toll-like receptor 1 and toll-like receptor 6. J Infect Dis. (2012) 205:944–54. doi: 10.1093/infdis/jir882
44. Ramirezortiz ZG, Specht CA, Wang JP, Lee CK, Bartholomeu DC, Gazzinelli RT, et al. Toll-like receptor 9-dependent immune activation by unmethylated CpG motifs in Aspergillus fumigatus DNA. Infect Immun. (2008) 76:2123–9. doi: 10.1128/IAI.00047-08
45. Bretz C, Gersuk G, Knoblaugh S, Chaudhary N, Randolphhabecker J, Hackman RC, et al. MyD88 signaling contributes to early pulmonary responses to Aspergillus fumigatus. Infect Immun. (2008) 76:952–8. doi: 10.1128/IAI.00927-07
46. Meier A, Kirschning CJ, Nikolaus T, Wagner H, Heesemann J, Ebel F. Toll-like receptor (TLR) 2 and TLR4 are essential for Aspergillus-induced activation of murine macrophages. Cell Microbiol. (2010) 5:561–70. doi: 10.1046/j.1462-5822.2003.00301.x
47. Bellocchio S, Moretti S, Perruccio K, Fallarino F, Bozza S, Montagnoli C, et al. TLRs govern neutrophil activity in aspergillosis. J Immunol. (2004) 173:7406–15. doi: 10.4049/jimmunol.173.12.7406
48. Akira S, Uematsu S, Takeuchi O. Pathogen recognition and innate immunity. Cell. (2006) 124:783–801. doi: 10.1016/j.cell.2006.02.015
49. Li SJ, Pasmans F, Croubels S, Verbrugghe E, Van WL, Yang Z, et al. T-2 toxin impairs antifungal activities of chicken macrophages against Aspergillus fumigatus conidia but promotes the pro-inflammatory responses. Avian Pathol. (2013) 42:457–63. doi: 10.1080/03079457.2013.822958
50. Taramelli D, Malabarba M, Sala G, Basilico N, Cocuzza G. Production of cytokines by alveolar and peritoneal macrophages stimulated by Aspergillus fumigatus conidia or hyphae. J Med Vet Mycol. (1996) 34:49–56. doi: 10.1080/02681219680000081
51. Brieland JK, Jackson C, Menzel F, Loebenberg D, Cacciapuoti A, Halpern J, et al. Cytokine networking in lungs of immunocompetent mice in response to inhaled Aspergillus fumigatus. Infect Immun. (2001) 69:1554–60. doi: 10.1128/IAI.69.3.1554-1560.2001
52. Tao J, Segal BH, Eppolito C, Li Q, Dennis CG, Youn R, et al. Aspergillus fumigatus extract differentially regulates antigen-specific CD4+ and CD8+ T cell responses to promote host immunity. J Leukoc Biol. (2006) 80:529–37. doi: 10.1189/jlb.0106026
53. Byrne AJ, Mathie SA, Gregory LG, Lloyd CM. Pulmonary macrophages: key players in the innate defence of the airways. Thorax. (2015) 70:1189–96. doi: 10.1136/thoraxjnl-2015-207020
54. Van Waeyenberghe L, Pasmans F, D'Herde K, Ducatelle R, Favoreel H, Li SJ, et al. Germination of Aspergillus fumigatus inside avian respiratory macrophages is associated with cytotoxicity. Vet Res. (2012) 43:32. doi: 10.1186/1297-9716-43-32
55. Shoham S, Levitz SM. The immune response to fungal infections. Br J Haematol. (2005) 129:569–582. doi: 10.1111/j.1365-2141.2005.05397.x
Keywords: Aspergillus fumigatus, chicken, pathogenicity, TLR signaling, pro-inflammatory cytokines
Citation: Cheng Z, Li M, Wang Y, Chai T, Cai Y and Li N (2020) Pathogenicity and Immune Responses of Aspergillus fumigatus Infection in Chickens. Front. Vet. Sci. 7:143. doi: 10.3389/fvets.2020.00143
Received: 01 November 2019; Accepted: 25 February 2020;
Published: 11 March 2020.
Edited by:
Jasim Muhammad Uddin, Bangladesh Agricultural University, BangladeshReviewed by:
Christi Swaggerty, United States Department of Agriculture (USDA), United StatesPascale Quéré, Institut National de la Recherche Agronomique, France
Copyright © 2020 Cheng, Li, Wang, Chai, Cai and Li. This is an open-access article distributed under the terms of the Creative Commons Attribution License (CC BY). The use, distribution or reproduction in other forums is permitted, provided the original author(s) and the copyright owner(s) are credited and that the original publication in this journal is cited, in accordance with accepted academic practice. No use, distribution or reproduction is permitted which does not comply with these terms.
*Correspondence: Yumei Cai, caiyum@163.com; Ning Li, sdtaianlining@126.com