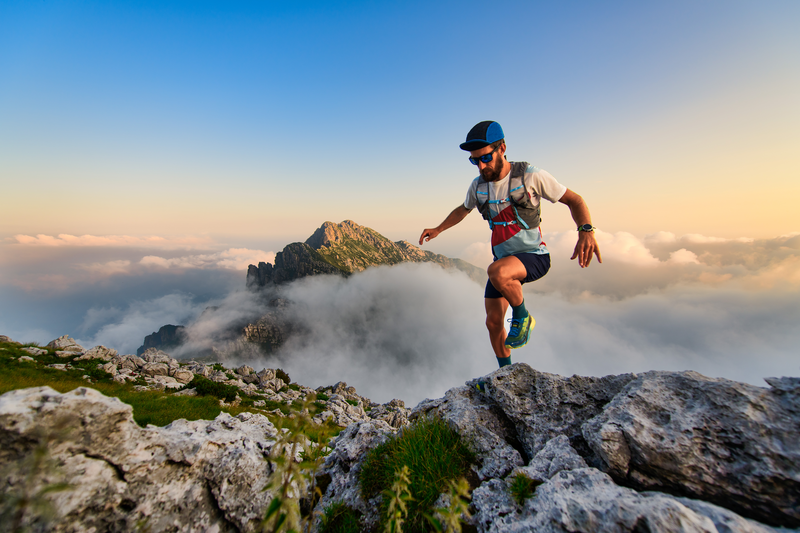
94% of researchers rate our articles as excellent or good
Learn more about the work of our research integrity team to safeguard the quality of each article we publish.
Find out more
ORIGINAL RESEARCH article
Front. Vet. Sci. , 21 February 2020
Sec. Veterinary Infectious Diseases
Volume 7 - 2020 | https://doi.org/10.3389/fvets.2020.00083
This article is part of the Research Topic Plant Derived Products to Combat Bacterial, Fungal and Parasitic Pathogens View all 5 articles
The fish parasites Saprolegnia spp. (Oomycota) and Amyloodinium ocellatum (Dinophyceae) cause important losses in freshwater and marine aquaculture industry, respectively. The possible adverse effects of compounds used to control these parasites in aquaculture resulted in increased interest on the search for natural products with antiparasitic activity. In this work, eighteen plant-derived compounds (2′,4′-Dihydroxychalcone; 7-Hydroxyflavone; Artemisinin; Camphor (1R); Diallyl sulfide; Esculetin; Eucalyptol; Garlicin 80%; Harmalol hydrochloride dihydrate; Palmatine chloride; Piperine; Plumbagin; Resveratrol; Rosmarinic acid; Sclareolide; Tomatine, Umbelliferone, and Usnic Acid) have been tested in vitro. Sixteen of these were used to determine their effects on the gill cell line G1B (ATCC®CRL-2536™) and on the motility of viable dinospores of Amyloodinium ocellatum, and thirteen were screened for inhibitory activity against Saprolegnia spp. The cytotoxicity results on G1B cells determined that only two compounds (2′,4′-Dihydroxychalcone and Tomatine) exhibited dose-dependent toxic effects. The highest surveyed concentrations (0.1 and 0.01 mM) reduced cell viability by 80%. Upon lowering the compound concentration the percentage of dead cells was lower than 20%. The same two compounds revealed to be potential antiparasitics by reducing in a dose-dependent manner the motility of A. ocellatum dinospores up to 100%. With respect to Saprolegnia, a Minimum Inhibitory Concentration was found for Tomatine (0.1 mM), Piperine and Plumbagin (0.25 mM), while 2′,4′-Dihydroxychalcone considerably slowed down mycelial growth for 24 h at a concentration of 0.1 mM. Therefore, this research allowed to identify two compounds, Tomatine and 2′,4′-Dihydroxychalcone, effective against both parasites. These compounds could represent promising candidates for the treatment of amyloodiniosis and saprolegniosis in aquaculture. Nevertheless, further in vitro and in vivo tests are required in order to determine concentrations that are effective against the considered pathogens but at the same time safe for hosts, environment and consumers.
Saprolegnia spp. Nees, 1823 (Oomycota–Straminopila supergroup) and Amyloodinium ocellatum Brown, 1931 (Dinophyceae–Alveolata) are considered dangerous parasites in the management and the economy of freshwater and marine aquaculture industry, as they parasitize generally the skin and gills of fish (1, 2) and, concerning Saprolegnia, also the eggs (3), causing significant losses.
Amyloodinium ocellatum is an ectoparasite dinoflagellate of brackish and marine warm water fish worldwide. Amyloodiniosis can be a major threat for land-based and lagoon-type rearing sites causing a parasitic branchitis associated with high morbidity, mortality and significant economic losses (1, 4, 5).
Oomycetes of the genus Saprolegnia, in particular S. parasitica, are the causative agents of Saprolegniosis, a disease characterized by the presence of visible white or gray patches of filamentous mycelium on the skin of freshwater fish and their eggs, with high mortality rates in freshwater fish culture. Besides being a problem for the fish farming industry, S. parasitica has also been implicated in wild salmon population decline around the world (6, 7).
The control of these parasites is problematic and a variety of treatments has been investigated over time (8–13).
In the past, Saprolegniosis was controlled with Malachite Green (MG). Following the discovery of its potential carcinogenicity, teratogenicity and mutagenicity in humans, and its environmental impact, the use of MG in the production of fish destined to human consumption is not authorized in the European Union (EU) (14). Nevertheless, EFSA established that food contaminated with MG or its metabolic product leucomalachite green (LMG) at or below the reference point for action (RPA) of 2 μg/kg is unlikely to represent a public health concern (15).
Copper sulfate is the most widely used compound for the control of Amyloodiniosis epidemics, usually administered at 0.12–0.15 mg/L for 10–14 days, although its therapeutic doses are also toxic to most invertebrates and algae (1). Copper sulfate was also described as effective against Saprolegnia, both in vitro (16) and on fish eggs in flow-through systems (17). However, although not banned, this compound is not registered for treatment against Saprolegnia or A. ocellatum in European Union. High copper concentration in water is associated with acute toxicity in the gill, with loss of the structural integrity of the epithelium and of branchial ionoregulatory functions (18). Moreover, the use of copper sulfate in aquaculture can contribute to increased accumulation of copper in the soil, where it already tends to increase due to its use in agriculture, which poses a significant risk to human health (19).
Another commonly used compound for the treatment of both Saprolegnia and A. ocellatum is formalin, a 37% solution of formaldehyde. Fajer-Ávila et al. (20), surveying the antiparasitic properties of the compound against ectoparasites (among which A. ocellatum) of bullseye puffer fish (Sphoeroides annulatus), reported 4 mg/L as the dose at which the compound had less adverse effects on fish in long-term exposure and reduced the parasite burden on skin and gills after 7 h of treatment. With respect to Saprolegnia, formalin has been effectively employed for egg disinfection in aquaculture to both treat and prevent infection in fish eggs (21). These authors observed that it is capable of inhibiting Saprolegnia cyst germination at a concentration of 250 mg/L. Formalin is currently not licensed as a veterinary medicine for the treatment of live fish in most of the EU countries. From the 1st of January 2016, formaldehyde has been classified as a category 1B carcinogen (22), thus its use should be restricted, due to possible hazardous effects to both exposed workers and environment.
Therefore, the use of chemicals in aquaculture may generate problems of environmental contamination: particularly, effluents from aquaculture facilities have become the subject of major concern regarding pollution, with adverse effects on the treated organisms and the environment. For these reasons, research has been increasingly focused on the selection of natural products with antimicrobial activity, in order to reduce the impacts of chemical and synthetic compounds on the environment, and with the perspective to avoid the possible development of drug resistance in parasites.
The purpose of this comparative study was to investigate the in vitro effectiveness of different biological compounds, of proven antimicrobial, antifungal and antiparasitic activity, against the oomycete species Saprolegnia parasitica and S. delica and against A. ocellatum dinospores.
According to the Consortium of the ParaFishControl Horizon2020 project, ZF-screens (ZF-S; Leiden, the Netherlands) provided a library from which 18 natural compounds were selected based on their recognized antiparasitic and antimicrobial activity. The compounds were supplied dissolved in Dimethyl Sulfoxide (DMSO) (10 mM) and stored at −20°C until use. Table 1 lists the natural compounds tested in the different experiments: 16 for cytotoxicity and activity against A. ocellatum, 13 for activity against Saprolegnia spp.
Pre-trial tests were performed on the G1B gill cell line (ATCC® CRL-2356™) in order to define the work compound concentrations and exclude the toxic ones. Cells were cultivated in sterile conditions in L-15 complete medium (L-15 containing 2 mM L-glutamine, 10% FCS, penicillin 100 U/ml, streptomycin 100 μg/ml) at 25°C. Upon reaching 100% confluence, cells were enzymatically digested with 0.25% Trypsin EDTA, counted by using the Trypan blue exclusion method and a Thoma chamber (23) and adjusted to a concentration of 1.1 × 106/ml. The cells where then transferred into sterile 96-well tissue culture plates and incubated in L-15 complete medium in sterile conditions at 25°C for 24 h to let them adhere to the wells bottom. After this period, the supernatant was removed and replaced with the diluted plant compounds.
Sixteen compounds (Table 1) were tested three times in triplicate wells in sterile 96-well tissue culture plates (Sarstedt) at 10-fold serial dilutions (ranging from 0.1 to 0.000001 mM). Compounds were diluted in L-15 complete medium starting from a concentration at which the toxicity due to DMSO solvent was negligible. Then 225 μl of the diluted compounds were added to the wells with seeded cells and incubated for 24 h at 25°C. Control wells were always included and represented by cells incubated with only L-15 complete medium. After the incubation period, the cytotoxicity effects of the compounds were verified by the absorbance-based Neutral Red test as described by Taju et al. (24).
In vitro motility test was performed in two subsequent rounds: in the first round, AOd hatched from non-preserved tomonts were used and in the second round tomonts hibernated for 3 months before use, as described hereafter. Trophonts were collected from the gills of the same naturally infested European sea bass (Dicentrarchus labrax) following the protocol of Beraldo et al. (25). Then, purified early tomonts (before the first division) were aliquoted and part of them incubated at 24°C for immediate use, while some aliquots were maintained in a hibernation status (16°C) and used 3 months later, after transferring them to 24°C for AOd hatching (25, 26). The reproductive process of both non-hibernated and hibernated tomonts was constantly monitored under an inverted microscope to check for dinospore hatching capacity. No differences in the hatching rate of tomonts and in the morphology, vitality and motility of dinospores were seen.
Dinospores were counted by adapting Dehority's (27) protocol. Briefly, 500 μl aliquots of the dinospore suspensions were stained with Lugol's iodine solution and counted using a counting cell chamber (S50 Sedgewick Rafter Cell, Pyser–SGI). Parasites were adjusted to a concentration of 5,200 dinospores/ml in HBSS/IO2 medium [H8264, Sigma Aldrich (28)].
Sixteen natural compounds (Table 1) were tested twice in duplicate wells in sterile 96-well plates (Sarstedt), at 2-fold serial dilutions (ranging from 50 to 6.25 μg/ml; 100 μl/well). Then, the experiment was repeated only for the compounds with evident inhibitory properties; in this case the dilutions ranged from 50 to 0.39 μg/ml. The compounds were diluted in HBSS/IO2 medium starting from a concentration at which the toxicity due to DMSO solvent was negligible and from the cytotoxicity results on the G1B gill cell line.
Dinospores (100 μl) were then added to each well; those incubated in culture medium without compound were used as negative controls. Copper sulfate chelated with citric acid (100 μl/well) at a final concentration of 1 μg/ml and 100 μl/well of formalin (4 μg/ml) were included in some wells of the plates as inhibitory positive controls. Plates, covered with lids, were maintained at room temperature for the whole duration of the experiment (24 h).
For the motility tests, AOd behavior was observed under an inverted microscope. At 1, 6, and 24 h after the beginning of the incubation with the compounds, aliquots of 50 μl were taken from each well and transferred into urinary deposit chambers (Vacutest Kima precision cell) in order to facilitate the visualization and counting of the dinospores. Aliquots were inspected under light microscopy. Only non-motile dinospores and those whose flagella did not vibrate (dead dinospores) were counted. Based on the number of non-motile dinospores, the percentage of motile dinospores was calculated for every compound concentration and at the different times of evaluation. Motility percentages were then compared to the motility of dinospores detected in the negative control, to which a motility value of 100% was assigned.
Tests were carried out on three Saprolegnia strains: one reference strain of Saprolegnia parasitica (CBS 223.65 provided by CSIC-RJB Madrid, Spain) isolated in Holland from northern pike (Esox lucius), one field strain of S. parasitica (ITT 320/15/20) isolated in Italy from brown trout (Salmo trutta fario), and one field strain of Saprolegnia delica (ITT 290/15/15) isolated in Italy from rainbow trout (Oncorhynchus mykiss). Saprolegnia spp. strains were maintained with periodic subcultures on glucose-yeast (GY) agar medium (5 g D-(+)-glucose, 1 g yeast extract, 12 g agar in 1 L deionized water) supplemented with 6 mg/L of penicillin and 10 mg/L of oxolinic acid (GY + P + Ox) (29) and kept at 18°C. For the in vitro trials, subcultures of the strains employed were incubated at 18°C until growth covered the full diameter of the dish (48–72 h). Inocula were obtained from the outer 10 mm of the culture, using a sterile 5 mm diameter glass cannula (protocol I) or cutting a 4 × 4 mm piece with a sterile scalpel.
Thirteen compounds were tested (Table 1) following protocol I according to Alderman (30). Ten-fold serial dilutions of the compound were added to sterile GY agar maintained liquid at a temperature of 49°C to obtain final concentrations of 0.00001; 0.0001; 0.001; 0.01; 0.1; and 0.25 mM. Mixtures were then distributed in six-well plates (Ø 35 mm) (allowing to test 5 different concentrations and one negative control) and were let solidify overnight. A 5 mm diameter well was then obtained in the center of the agar, using a sterile glass cannula, and was filled with a standard 5 mm inoculum, culture surface facing upwards. Each strain was tested in triplicate per each concentration. 2′,4′-Dihydroxychalcone, 7-Hydroxyflavone, Eucalyptol, Esculetin, Palmatine Chloride and Tomatine were not tested at the concentration of 0.25 mM, since they were already slightly toxic at 0.1 mM, according to the results of cytotoxicity tests on G1B cells. Pure DMSO was also screened at the same amount to exclude any inhibitory or lethal effect. Plates were incubated at 18°C and checked after 24, 48, 72 h and 6 days, determining the colony diameter of the growing mycelium as average of two axes measured at 90° from each other. Mycelial growth was then expressed as mean value of the replicates. The minimum inhibitory concentration (MIC) was defined as the lowest concentration inhibiting completely the growth of the mycelium after 6 days of incubation.
Cytotoxicity results demonstrated that 14 out of 16 compounds (7-Hydroxyflavone; Artemisinin; Camphor (1R); Diallyl sulfide; Esculetin; Eucalyptol; Garlicin 80%; Harmalol hydrochloride dihydrate; Palmatine chloride; Piperine; Resveratrol; Rosmarinic acid; Sclareolide and Umbelliferone) displayed no or slightly toxic effects on G1B cells (with <20% of dead cells). On the other hand, 2′,4′-Dihydroxychalcone and Tomatine exhibited dose-dependent toxic effects. At the highest concentrations investigated (0.1 and 0.01 mM) only 20% of the cells was still viable. However, the percentage of dead cells considerably decreased at the third concentration (0.001 mM) with more than 80% of viable cells, while the lowest concentrations displayed a negligible toxicity.
In the present investigation, the 16 plant compounds were initially tested twice on dinospore motility at concentrations ranging from 50 to 6.25 μg/ml. For these tests it was decided to express the concentration as μg/ml in order to study their properties at the same concentration of active compound. The range of tested concentrations derived from literature consultation and was applied in parallel studies to evaluate the possible immunomodulatory activities of the same compounds on European sea bass head kidney leukocytes by respiratory burst tests (31). Fourteen out of 16 compounds (7-Hydroxyflavone; Artemisinin; Camphor (1R); Diallyl sulfide; Esculetin; Eucalyptol; Garlicin 80%; Harmalol hydrochloride dihydrate; Palmatine chloride; Piperine; resveratrol; Rosmarinic acid; Sclareolide; and Umbelliferone) showed no considerable effects on dinospore motility, as described in Figures 1–3. In fact, the percentage of dinospores' movements in the wells with these substances was comparable to those recorded for the negative control.
Figure 1. Motility of dinospores of A. ocellatum incubated at room temperature with different concentrations (6.25–50 μg/ml) of 16 plant derived compounds. The motility is expressed as percentage of motile dinospores on the total number of dinospores present per well after 1 h of incubation. Then, values have been related to the motility observed in the wells assigned to negative control and corresponding to 100%.
Figure 2. Motility of dinospores of A. ocellatum incubated at room temperature with different concentrations (6.25–50 μg/ml) of 16 plant derived compounds. The motility is expressed as percentage of motile dinospores on the total number of dinospores present per well after 6 h of incubation. Then, values have been related to the motility observed in the wells assigned to negative control and corresponding to 100%.
Figure 3. Motility of dinospores of A. ocellatum incubated at room temperature with different concentrations (6.25–50 μg/ml) of 16 plant derived compounds. The motility is expressed as percentage of motile dinospores on the total number of dinospores present per well after 24 h of incubation. Then, values have been related to the motility observed in the wells assigned to negative control and corresponding to 100%.
Instead, 2′,4′-Dihydroxychalcone and Tomatine had an evident inhibitory action on dinospores, for this reason they were subsequently tested extending the range of concentrations from 50 to 0.39 μg/ml, as reported in Figures 4–6. These results showed that 2′,4′-Dihydroxychalcone inhibited the dinospore motility at the highest tested concentrations 50 and 25 μg/ml (0.2 and 0.1 mM) after 1 h of incubation. After 6 h of incubation, only the three highest doses 50, 25, and 12.5 μg/ml (0.2, 0.1, and 0.05 mM) stopped the motility of dinospores, whereas motility inhibition was halved at 6.25 and 3.13 μg/ml (0.025 and 0.013 mM), while the remaining concentrations did not demonstrate a relevant inhibitory activity on dinospore motility. After 24 h of incubation, 2′,4′-Dihydroxychalcone at the concentration range of 50–6.25 μg/ml (0.2–0.025 mM) inhibited the motility of all the dinospores in the wells, while at 3.13 μg/ml (0.025 mM) 4% of dinospores still moved. Conversely, 0.39, 0.78, and 1.56 μg/ml (0.002, 0.003, 0.006 mM) concentrations of this chalcone did not inhibit dinospore motility.
Figure 4. Motility of dinospores of A. ocellatum incubated at room temperature with different concentrations (0.39–50 μg/ml) of 2′,4′-dihydroxychalcone and tomatine. The motility is expressed as percentage of motile dinospores on the total number of dinospores present per well after 1 h of incubation. Then, values have been related to the motility observed in the wells assigned to negative control and corresponding to 100%.
Figure 5. Motility of dinospores of A. ocellatum incubated at room temperature with different concentrations (0.39–50 μg/ml) of 2′,4′-dihydroxychalcone and tomatine. The motility is expressed as percentage of motile dinospores on the total number of dinospores present per well after 6 h of incubation. Then, values have been related to the motility observed in the wells assigned to negative control and corresponding to 100%.
Figure 6. Motility of dinospores of A. ocellatum incubated at room temperature with different concentrations (0.39–50 μg/ml) of 2′-4′-dihydroxychalcone and tomatine. The motility is expressed as percentage of motile dinospores on the total number of dinospores present per well after 24 h of incubation. Then, values have been related to the motility observed in the wells assigned to negative control and corresponding to 100%.
Tomatine displayed more evident inhibitory effects on dinospore motility than 2′,4′-Dihydroxychalcone for the whole duration of the experiment (24 h) in the range doses 6.25–50 μg/ml (0.006–0.05 mM). In fact, after 1 h of incubation, the highest concentrations of this substance ranging from 50 to 6.25 μg/ml (0.05–0.006 mM) completely inhibited the dinospore motility. On the other hand, in the concentrations ranging from 3.13 to 0.39 μg/ml (0.003–0.0004 mM) the percentage of swimming dinospores was 89–95%. Similarly, after 6 h and 24 h of incubation no motility of dinospores was observed in the wells with the highest Tomatine concentrations 50–6.25 μg/ml (0.05–0.006 mM) as noticed after 1 h, whereas the lowest concentrations were less or not effective, and capable of inhibiting dinospore motility up to 9%.
Chelated copper sulfate at 1 μg/ml (0.006 mM) was effective for the whole duration (24 h) of the experiment; similarly, formalin at 4 μg/ml (0.133 mM) concentration showed an inhibiting activity on the dinoflagellate but at a long term exposure. In fact, after 1 h of incubation only 2% of dinospores was immotile. However, at 6 and 24 h no swimming dinospores were observed.
According to the results of in vitro tests against Saprolegnia strains, triplicates were always consistent among each other. As expected, DMSO alone had no detectable effect at tested concentrations.
Minimum Inhibitory Concentrations (MICs) were defined for only three biological compounds, namely Tomatine, Piperine, and Plumbagin (Table 2), albeit with different in vitro behavior. Tomatine at 0.1 mM (99.4 μg/ml) completely inhibited S. delica while, for S. parasitica, at this concentration only a partial reduction of radial mycelial growth was observed (Figure 7). Nevertheless, after 6 days at this concentration, inhibition of aerial mycelium for S. parasitica was observed. The effect of Piperine, showing MIC at 0.25 mM (71.33 μg/ml), was consistent between different strains (Figure 8). Also Plumbagin showed MIC at 0.25 mM (47.045 μg/ml), although a complete inhibition of S. delica was also observed at a concentration of 0.1 mM (18.82 μg/ml) for the first 24 h (Figure 9).
Figure 7. Average diameter (mm) of Saprolegnia parasitica (A) and Saprolegnia delica (B) mycelium with different concentrations of Tomatine.
Figure 8. Average diameter (mm) of all tested strains mycelium with different concentrations of Piperine.
Figure 9. Average diameter (mm) of Saprolegnia parasitica (A) and Saprolegnia delica (B) mycelium with different concentrations of Plumbagin.
For 2′,4′-Dihydroxychalcone no MIC was defined, however mycelial growth was considerably slowed down for 24 h in all tested strains at a concentration 0.01 mM (2.40 μg/ml). Furthermore, the complete inhibition of S. parasitica for 48 h and of S. delica for 24 h at a concentration of 0.1 mM (24.03 μg/ml) was observed; after 6 days, at this concentration mycelial growth was still slower than the control in all tested strains (Figure 10).
Figure 10. Average diameter (mm) of Saprolegnia parasitica (A) and Saprolegnia delica (B) mycelium with different concentrations of 2′,4′-Dihydroxychalcone.
In general, a slower radial mycelial growth of S. parasitica and S. delica strains was recorded at 24 h for all tested compounds at a concentration 0.1 mM, although with different degrees of effectiveness (Figure 11). Percentage radial growth compared with the untreated control is reported in Table 3. Increasing concentrations of 7-Hydroxyflavone, Eucalyptol and Esculetin progressively slowed down mycelial growth only for 24 h, however at 48 h mycelial growth was equal to the control for all tested concentrations. A similar pattern occurred with Camphor and Diallyl Sulfide: increasing concentrations of these compounds progressively slowed down mycelial growth for 24 h at all tested concentrations, and for 48 h at a concentration of 0.25 mM (38.06 and 28.56 μg/ml, respectively). For Piperine and Sclareolide, mycelial growth of S. parasitica never reached the diameter of the control at 0.1 mM (28.53 and 25.38 μg/ml, respectively). The same growth pattern could be observed for Umbelliferone at a concentration of 0.25 mM (40.53 μg/ml). On the contrary, plumbagin was more effective at inhibiting the growth of S. delica at 0.1 mM (18.82 μg/ml) rather than the two tested S. parasitica strains. Moreover, Usnic Acid effectively reduced mycelial growth for 72 h at a concentration of 0.1 mM (86.08 μg/ml). Finally, at 6 days, in five compounds other than Tomatine, the inhibition of aerial mycelium was also observed at different concentrations: Camphor and Diallyl Sulfide at 0.25 mM (30.06 and 20.55 μg/ml, respectively) for all tested strains; Umbelliferone at 0.1 mM (16.21 μg/ml) for S. parasitica and at 0.25 mM (40.53 μg/ml) for S. delica; Sclareolide at 0.1 mM (25.038 μg/ml) for S. parasitica and 0.25 (62.59) for S. delica (Table 2).
Figure 11. Average radial growth (mm) at 24 h of Saprolegnia spp. with different products at a concentration of 0.1 mM.
Table 3. Percentage radial growth of S. delica e S. parasitica compared with the control after 24 h at 0.1 mM.
The use of chemicals is commonly the principal approach for keeping ectoparasites in cultured fish under control. However, as highlighted above, compounds commonly used for the control of parasitic diseases in aquaculture are potentially harmful to fish, humans and the environment. In this context, there is a strong need to search for new strategies to limit the spread of diseases in fish farms, with a particular attention on animal welfare and environmental impact including, among other approaches, the use of natural compounds.
Regarding Saprolegnia, several studies are present in literature about the use of natural compounds. Tampieri et al. (32) evaluated the in vitro effectiveness of 18 essential oils and their isolated chemical constituents against Saprolegnia parasitica, finding that the most effective essential oils showed inhibitory and lethal effects at concentrations of 100 ppm (μg/ml). Moreover, plant extracts have been tested in vitro for their antifungal activity against Saprolegnia ferax isolated from naturally infected carps (Cyprinus carpio) (33), highlighting how a range of extracts displayed strong antifungal activity, at concentrations <500 μg/ml.
Regarding Amyloodinium, several treatments alternative to copper sulfate have been tested in experimental trials in order to manage amyloodiniosis, but inhibitory effects were not determined and, in some cases, neither fully clarified (9, 11). On the other hand, to date there is no available literature information about the in vitro and/or in vivo use of plant derived compounds or medicinal plants as antiparasitic candidates against A. ocellatum.
In the present study, the potential antiparasitic effects of 18 plant derived compounds were investigated. In particular, 13 compounds were screened to test their effect on the hyphal growth of S. parasitica and S. delica, while 16 compounds were tested on viable A. ocellatum dinospores (Table 1). In fact, among different developmental stages of A. ocellatum, only dinospores are susceptible to fish-safe chemical bath treatments (9, 13, 34), while trophonts and tomonts (the parasitic and reproductive stage of the protozoan, respectively) are more resistant. In the tests performed in the present investigation, copper sulfate and formalin were used as inhibitory positive control for A. ocellatum. Copper sulfate was used chelated with citric acid since the free copper ion, that is the active component, is unstable in seawater. Therefore, in order to increase the ion stability the usage of chelated copper compounds is recommended (1, 35). According to Paperna (9) and Bessat and Fadel (13), at a concentration of 1 μg/ml the compound was capable of completely interrupting dinospore motility for the whole duration of the experiment (24 h). Formalin was used at a concentration of 4 μg/ml on the basis of Fajer-Ávila et al. (20) in vivo investigations.
The choice of the compounds, selected from a larger pre-existing panel, was determined by their antiparasitic/anti-invertebrate activity in other contexts. For example, several studies have demonstrated antifungal activity of camphor-rich essential oils against a wide range of phytopathogenic fungi including the oomycetes Phytophthora infestans through alterations in hyphal morphology and cytoplasmic content (36). Garlic (Allium sativum) and its extracts have long been known for their antibacterial, antiviral, antifungal, antiparasitic, and immune modulating properties (37–43) and Buchmann et al. (44) found that garlic extract killed theronts of the ciliate Ichthyophthirius multifiliis. The antimicrobial activity of coumarins, among which Esculetin and Umbelliferone, has been demonstrated in several studies (45–50). Palmatine chloride has been recently screened against the causative agent of malaria, the protozoan parasite Plasmodium falciparum, showing strong anti-malarial effects (51). Flavonoids, such as 7-Hydroxyflavone, are naturally produced in plants with antifungal properties (52–54). Usnic acid, a secondary lichen metabolite, possesses antimicrobial activity against a number of planktonic gram-positive bacteria and seems to be able to inhibit bacterial biofilm formation (55). Piperine is a bioactive alkaloid found in the skin and seeds of black pepper (Piper nigrum) fruits. Earlier work highlighted the antibacterial and antifungal effectiveness of piperine suggesting its use as natural antimicrobial agent [(56, 57)]. Plumbagin is a hydroxyl-naphthoquinone originally extracted from plants belonging to the Plumbaginaceae with renown antifungal (58) and antiparasitic (59) properties.
Nevertheless, the A. ocellatum motility test results obtained in the present study, showed that 14 compounds [7-Hydroxyflavone; Artemisinin; Camphor (1R); Diallyl sulfide; Esculetin; Eucalyptol; Garlicin 80%; Harmalol hydrochloride dihydrate; Palmatine chloride; Piperine; Resveratrol; Rosmarinic acid; Sclareolide and Umbelliferone] had a limited inhibitory activity against the protozoan (up to 14%), while only the glycoalkaloid Tomatine and the chalcone 2′,4′-Dihydroxychalcone considerably reduced dinospores motility. Regarding Saprolegnia spp., even if a slower radial mycelial growth of S. parasitica and S. delica strains was recorded for all the 13 tested compounds, only 2′,4′-Dihydroxychalcone and Usnic Acid completely inhibited radial growth of both Saprolegnia spp. after 24 h at 0.1 mM (Table 3). MICs were determined only for Tomatine, Piperine and Plumbagin. Although no MIC was defined for 2′,4′-Dihydroxychalcone, after 6 days the mycelial growth was still reduced in all tested strains at 0.1 mM (24 μg/ml).
Glycoalkaloids, which are commonly referred to as saponins, are known to possess antimicrobial and antifungal activities that act as plant defenses against pests, pathogens and invasion by neighboring plants (60). Tomato plants (Solanum lycopersicum) produce Tomatine, a tetrasaccharide linked to the 3-OH group of the aglycone tomatidine. Immature green tomatoes contain up to 500 mg of Tomatine/kg of fresh fruit weight, nevertheless the compound is largely degraded as the tomato ripens until it reaches levels in mature red tomatoes of 5 mg/kg of fresh fruit weight (61). Tomatine administrated in vivo at a dose of 2,000 ppm showed anticarcinogenic effects against dibenzo[a,l]pyrene (DBP)-induced liver and stomach tumors in rainbow trout (O. mykiss) without adverse effects on animals (62). More recently, Liu et al. (63) documented inhibitory effects of Tomatine on human and animal pathogenic protozoa. In particular, the reported doses of the compound, which inhibited the growth of the three surveyed trichomonads, are in line with the concentrations tested in the present trial against A. ocellatum, in which Tomatine displayed inhibitory effects for the whole duration of the experiment (24 h) in the dose ranges 6.25–50 μg/ml (0.006–0.05 mM). Conversely, at concentrations lower than 6.25 μg/ml (0.006 mM) this glycoalkaloid was not or less effective. However, further studies will be necessary to understand whether Tomatine displays only an inhibitory effect or a dinosporicide activity against A. ocellatum at the surveyed effective concentrations. Previous research showed that α-tomatine owes its toxic properties to the ability to interact with 3ß-hydroxy sterol (64). Some oomycetes, such as Pythium and Phytophthora species, do not produce 3ß-hydroxy sterol and are relatively tolerant to α-tomatine (65). Nevertheless, Sandrock and VanEtten (66) found inhibitory effect on Phytophthora infestans and Pythium aphanidermatum, where this sterol is lacking, suggesting that α-tomatine may possess additional properties other than binding the 3ß-hydroxy sterol. In the present work, Tomatine showed a MIC of 99.4 μg/ml only against Saprolegnia delica although, after 6 days, at this concentration the inhibition of aerial mycelium for S. parasitica was observed. A similar behavior was also observed for Camphor (at 38 μg/ml), Diallylsulfide (at 28.6 μg/ml), Sclareolide (at 25 μg/ml for S. parasitica and 62.6 μg/ml for S. delica), and Umbelliferone (16.2 μg/ml for S. parasitica and 40.5 for S. delica) (Table 2). The inhibition of aereal mycelium of Saprolegnia spp. was also described, due to other compounds (67). In the past Kaminskyj and Heath (68) hypothesized that this phenomenon could be associated to chemically induced morphological hyphae changes, but the mechanisms of action are not clear and require further study.
The variety of biological properties displayed by chalcones is well-documented in literature. In fact, these aromatic ketones possess anti-inflammatory, antioxidant, antitumoral, antimicrobial, antifungal, anti-leishmanial, anti-malarial, and antiviral activities (69–71). 2′,4′-Dihydroxychalcone, isolated from Zuccagnia punctata Cav. (Fabaceae), has shown antifungal properties against a wide range of plant (72) and human (73) pathogens and has been suggested as promising antifungal agent for use in humans, acting by a different mechanism of action than currently used antifungal drugs, such as azoles or echinocandins (73). Seo et al. (74) tested the inhibitory effects and investigated the mode of action of 2′,4′-Dihydroxychalcone against Aspergillus fumigatus, demonstrating how the compound inhibits the calcinurin signaling pathway, necessary for proper hyphal growth. At 48 h, the MIC50 (MIC that inhibits 50% of growth) of 2′,4′-Dihydroxychalcone was between 64 and 128 μg/mL, while treatment with 256 μg/mL drastically decreased mycelial growth. This compound was tested also against Saprolegnia by Flores et al. (75), showing a 48 h MIC of 6.25 μg/mL. Partially in accord with these authors, our results show that 2′,4′-Dihydroxychalcone completely inhibited S. parasitica for 48 h and S. delica for 24 h at a concentration of 24.03 μg/mL (0.1 mM), however some mycelial growth was observed starting at 72 h. Conversely, the tests performed on A. ocellatum show that 2′,4′-Dihydroxychalcone completely inhibited the dinospore motility at the highest concentrations 50 and 25 μg/ml (0.2 and 0.1 mM) after 1 h of incubation, to then being inhibitory till 3.13 μg/ml (0.013 mM) in long term exposure (24 h). Anyway, other experiments are necessary to clarify if A. ocellatum dinospores may be killed by this compound at higher concentrations or if their motility is only temporarily inhibited in the presence of this substance. However, the results obtained by Flores et al. (75) with a spores germination inhibition test on S. parasitica and S. australis, suggest that 2′,4′-Dihydroxychalcone may display dinosporicide activity at concentrations higher than MIC, whereas at lower concentrations it could inhibit the dinospore movements only for a certain period of time. Nevertheless, since there are no published reports on the use of 2′,4′-Dihydroxychalcone in fish, further researches will be necessary to determine the effects of this compound on fish in the perspective of its application in aquaculture as remedy against fish parasites.
Only for Saprolegnia spp. an inhibiting activity was found also for Piperine and Plumbagin, at 71.3 μg/ml (0.25 mM) and 47 μg/ml (0.25 mM), respectively. In aquaculture, Piperine was also described as effective at 9.0 mg/L against Argulus sp. in Carassius auratus (76) however, to our knowledge, no studies involving Saprolegnia spp., are present in literature. The extract of Plumbago rosea, which contains plumbagin (2-methoxy-5-hydroxy-1-4-napthoquinone) as pharmacologically active component, was used mixed with food as immunostimulant in Catla catla post-challenged with Aeromonas hydrophila (77). Plumbagin was also found effective against various oomycetes (MIC = 5 μg/ml) but was not tested against Saprolegnia sp. (78). Other studies showed its considerable bactericidal activity and overall toxicity to aquatic organisms, and it was proposed for treatment of ship's ballast water (79). Therefore, its possible use in aquaculture should be subjected to further investigations aimed at excluding harmful effects on the environment.
To our knowledge, the plant derived compounds investigated in this study have never been used before on viable dinospores of A. ocellatum and only 2′,4′-Dihydroxychalcone was previously tested for Saprolegnia spp. (75). The present investigation showed that, among the compounds tested, Tomatine and 2′,4′-Dihydroxychalcone have shown to be effective for both parasites. In particular, even if the latter compound does not completely inhibit the mycelial growth of Saprolegnia spp. after 6 days, its possible application in aquaculture should be better investigated in the future. In fact, Flores et al. (75) stated that the use of 2′,4′-Dihydroxychalcone in the hatchery could be cost effective and safe for both workers and environment, representing a good option to replace synthetic compounds in the control of saprolegniosis. Nevertheless, the observations provided by this research are to be considered preliminary. Further in vitro investigations should be performed to better explore the mechanisms of action of these compounds, and in vivo small-scale trials should be arranged in order to select doses which may be effective against A. ocellatum and Saprolegnia spp. but at the same time non-toxic for their marine or freshwater hosts, respectively, the environment and consumer.
The datasets generated for this study are available on request to the corresponding author.
RG and PB conceived the original idea. PT, PB, MM, and DV carried out the in vitro tests. RD prepared and furnished the tested compounds solutions in DMSO. PT with RG, PB, and MM wrote the manuscript. MF supervised the project and all the authors provided critical feedback and the supervision of the manuscript.
This project has received funding from the European Union's Horizon 2020 research and innovation programme under grant agreement No. 634429. This publication reflects the views only of the author, and the European Commission cannot be held responsible for any use, which may be made of the information contained therein.
RD was employed by company Future Genomics Technologies BV.
The remaining authors declare that the research was conducted in the absence of any commercial or financial relationships that could be construed as a potential conflict of interest.
The authors would like to thank CSIC-RJB Madrid, Spain for providing Saprolegnia parasitica reference strain CBS 223.65, and all the fish farmers/staff, veterinarians, and biologists, who collaborated in the Amyloodinium ocellatum trophont collection.
1. Noga EJ. Amyloodinium ocellatum. In: Woo PTK, Buchman K, editors. Fish Parasites Pathobiology and protection. London: CAB International (2012). p. 383.
2. Dighton J, White JF. The Fungal Community: Its Organization and Role in the Ecosystem. 4th ed. Boca Raton, FL: CRC Press (2017), 597 pp.
3. Van Den Berg AH, McLaggan D, Diéguez-Uribeondo J, Van West P. The impact of the water moulds Saprolegnia diclina and Saprolegnia parasitica on natural ecosystems and the aquaculture industry. Fungal Biol Rev. (2013) 27:33–42. doi: 10.1016/j.fbr.2013.05.001
4. Cruz-Lacierda ER, Maeno Y, Pineda AJT, Matey VE. Mass mortality of hatchery-reared milkfish (Chanos chanos) and mangrove red snapper (Lutjanus argentimaculatus) caused by Amyloodinium ocellatum (Dinoflagellida). Aquaculture. (2004) 236:85–94. doi: 10.1016/j.aquaculture.2004.02.012
5. Beraldo P, Byadgi O, Massimo M, Bulfon C, Volpatti D, Galeotti M. Grave episodio di amyloodiniosi in giovanili di branzino (Dicentrarchus labrax): analisi dei determinanti di malattia e rilievi anatomopatologici. In: Conference Proceedings of the XXIII Convegno Nazionale Società Italiana di Patologia Ittica, Lecce (2017). p30.
6. Neitzel DA, Elston RA, Abernethy CS. DOE Report (Contract:DE-AC06-76RL01830). Prevention of Prespawning Mortality: Cause of Salmon Headburns and Cranial lesions. (2004). p. 1–B25. Available online at: https://www.pnnl.gov/main/publications/external/technical_reports/PNNL-14748.pdf
7. Van West P. Saprolegnia parasitica, an oomycete pathogen with a fishy appetite: new challenges for an old problem. Mycologist. (2006) 20:99–104. doi: 10.1016/j.mycol.2006.06.004
8. Alderman DJ, Polglase JL. A comparative investigation of the effects of fungicides on Saprolegnia parasitica and Aphanomyces astaci. Trans Br Mycol Soc. (1984) 83:313–8. doi: 10.1016/S0007-1536(84)80153-9
9. Paperna I. Chemical control of Amyloodinium ocellatum (Brown 1931) (Dinoflagellida) infections: in vitro tests and treatment trials with infected fishes. Aquaculture. (1984) 38:1–18. doi: 10.1016/0044-8486(84)90133-9
10. Oestmann DJ, Lewis DH, Zettler BA. Clearance of Amyloodinium ocellatum dinospores by Artemia salina. J Aquat Anim Health. (1995) 7:257–261. doi: 10.1577/1548-8667(1995)007<0257:CCOAOD>2.3.CO;2
11. Montgomery-Brock D, Sato VT, Brock JA, Tamaru CS. The application of hydrogen peroxide as a treatment for the ectoparasite Amyloodinium ocellatum (Brown 1931) on the Pacific threadfin Polydactylus sexfilis. J World Aquacult Soc. (2001) 32:250–4. doi: 10.1111/j.1749-7345.2001.tb01103.x
12. Rahkonen R, Koski P, Shinn A, Wootten R, Sommerville C. Post malachite green: alternative strategies for fungal infections and white spot disease. Bull Eur Assoc Fish Pat. (2002) 22:152–7.
13. Bessat M, Fadel A. Amyloodiniosis in cultured Dicentrarchus labrax: parasitological and molecular diagnosis, and an improved treatment protocol. Dis Aquat Organ. (2018) 129:41–51. doi: 10.3354/dao03237
14. European Commission. Commission Regulation (EU) No 37/2010 of 22 December 2009 on pharmacologically active substances and their classification regarding maximum residue limits in foodstuffs of animal origin. Off J Eur Union. (2010) 15:1–72.
15. EFSA. Malachite green in food. EFSA panel on contaminants in the food chain (CONTAM). EFSA J. (2016) 14:4530. doi: 10.2903/j.efsa.2016.4530
16. Marking LL, Rach JJ, Schreier TM. American Fisheries Society evaluation of antifungal agents for fish culture. Prog Fish Cult. (1994) 56:225–31. doi: 10.1577/1548-8640(1994)056<0225:AFSEOA>2.3.CO;2
17. Straus DL, Mitchell AJ, Carter RR, Steeby JA. Hatch rate of channel catfish Ictalurus punctatus (Rafinesque 1818) eggs treated with 100 mg/L copper sulphate pentahydrate. Aquac Res. (2012) 43:14–8. doi: 10.1111/j.1365-2109.2010.02791.x
18. Shaw BJ, Al-Bairuty G, Hanfy RD. Effects of waterborne copper nanoparticles and copper sulphate on rainbow trout (Oncorhynchus mykiss): physiology and accumulation. Aquat Toxicol. (2012) 116–117:90–101. doi: 10.1016/j.aquatox.2012.02.032
19. Panagos P, Ballabio C, Lugato E, Jones A, Borrelli P, Scarpa S, et al. Potential sources of anthropogenic copper inputs to European agricultural soils. Sustainability. (2018) 10:2380. doi: 10.3390/su10072380
20. Fajer-Ávila EJ, Abdo-de la Parra I, Aguilar-Zarate G, Contreras-Arce R, Zaldívar-Ramírez J, Betancourt-Lozano M. Toxicity of formalin to bullseye puffer fish (Sphoeroides annulatus Jenyns, 1843) and its effectiveness to control ectoparasites. Aquaculture. (2003) 223:41–50. doi: 10.1016/S0044-8486(03)00166-2
21. Bly JE, Quiniou SMA, Lawson LA, Clem LW. Therapeutic and prophylactic measures for winter saprolegniosis in channel catfish. Dis Aquat Organ. (1996) 24:25–33. doi: 10.3354/dao024025
22. European Commission. Commission Regulation (EU) No. 605/2014 of 5 June 2014 amending, for the purposes of introducing hazard and precautionary statements in the Croatian language and its adaptation to technical and scientific progress, Regulation (EC) No 1272/2008 of the European Parliament and of the Council on classification, labelling and packaging of substances and mixtures. Off J Eur Union. (2014) 167, 36–49.
23. Volpatti D, Bulfon C, Tulli F, Galeotti M. Growth parameters, innate immune response and resistance to Listonella (Vibrio) anguillarum of Dicentrarchus labrax fed carvacrol supplemented diets. Aquac Res. (2014) 45:31–44. doi: 10.1111/j.1365-2109.2012.03202.x
24. Taju G, Abdul Majeed S, Nambi KSN, Farook MA, Vimal S, Sahul Hameed AS. In vitro cytotoxic, genotoxic and oxidative stress of cypermethrin on five fish cell lines. Pest Biochem Physiol. (2014) 113:15–24. doi: 10.1016/j.pestbp.2014.06.006
25. Beraldo P, Massimo M, Galeotti M. SOP for Amyloodinium ocellatum. In: Sitjà-Bobadilla A, Bron JE, Wiegertjes GF, Piazzon MC, editors. Fish Parasites: A Handbook of Protocols for Their Isolation, Culture and Transmission. Sheffield: 5m Publishing (in press).
26. Massimo M. New perspectives in the control of Amyloodinium ocellatum infection in reared sea bass (Dicentrarchus labrax) (Ph.D. dissertation), University of Udine, Udine, Italy (2019).
28. Noga EJ. Culture conditions affecting the in vitro propagation of Amyloodinium ocellatum. Dis Aquat Organ. (1989) 6:137–43. doi: 10.3354/dao006137
29. Alderman DJ, Polglase JL. Aphanomyces astaci: Isolation and culture. J Fish Dis. (1986) 9:367–79. doi: 10.1111/j.1365-2761.1986.tb01030.x
30. Alderman DJ. In vitro testing of fisheries chemotherapeutants. J Fish Dis. (1982) 5:113–23. doi: 10.1111/j.1365-2761.1982.tb00464.x
31. Massimo M, Bulfon C, Beraldo P, Byadgi O, Galeotti M, Volpatti D. Preliminary study on in vitro effects of selected plant compounds on sea bass (D. labrax) head kidney leukocytes. In: Book of Abstracts, p. 385 of the 18th International Conference on Diseases of Fish and Shellfish, European Association of Fish Pathologists (EAFP), Belfast (2017).
32. Tampieri MP, Galuppi R, Carelle MS, Macchioni F, Cioni PL, Morelli I. Effect of selected essential oils and pure compounds on Saprolegnia parasitica. Pharm Biol. (2003) 41:584–91. doi: 10.1080/13880200390501839
33. Huang XL, Liu RJ, Whyte S, Du ZJ, Chen DF, Deng YQ, et al. The in vitro antifungal activity of 30 Chinese herb extracts to Saprolegnia sp. J Appl Ichthyol. (2015) 31:681–6. doi: 10.1111/jai.12773
34. Lawler AR. Studies on Amyloodinium ocellatum (Dinoflagellata) in Mississippi sound: natural and experimental hosts. Gulf Res Rep. (1980) 6:403–13. doi: 10.18785/grr.0604.08
35. Montgomery-Brock D, Sylvester JY, Tamaru CS, Brock J. Hydrogen peroxide treatment for Amyloodinium sp. on mullet (Mugil cephalus) fry. Center for Tropical and Subtropical Aquaculture. Aqua Tips Reg Notes. (2000) 11, 4–6.
36. Soylu EM, Soylu S, Kurt S. Antimicrobial activities of the essential oils of various plants against tomato late blight disease agent Phytophthora infestans. Mycopathologia. (2006) 161:119–28. doi: 10.1007/s11046-005-0206-z
37. Soffar SA, Mokhtar GM. Evaluation of the antiparasitic effect of aqueous garlic (Allium sativum) extract in Hymenolepiasis nana and giardiasis. J Egypt Soc Parasitol. (1991) 21:497–502.
38. Weber ND, Andersen DO, North JA, Murray BK, Lawson LD, Hughes BG. In vitro virucidal effects of Allium sativum (garlic) extract and compounds. Planta Med. (1992) 58:417–23. doi: 10.1055/s-2006-961504
39. Yoshida H, Katsuzaki H, Ohta R, Ishikawa K, Fukuda H, Fujino T, et al. An organosulfur compound isolated from oil-macerated garlic extract, and its antimicrobial effect. Biosci Biotechnol Biochem. (1999) 63:588–90. doi: 10.1271/bbb.63.588
40. Harris JC, Plummer S, Turner MP, Lloyd D. The microaerophilic flagellate Giardia intestinalis: Allium sativum (garlic) is an effective antigiardial. Microbiology. (2000) 146:3119–27. doi: 10.1099/00221287-146-12-3119
41. Behnia M, Haghighi A, Komeilizadeh H, Tabaei SS, Abadi A. In vitro antiamoebic activity of Iranian Allium sativum in comparison with metronidazole against Entamoeba histolytica. Iran J Parasitol. (2008) 3:32–8.
42. Abdel-Salam AM, Ammar N, Abdel-Hamid AZ. Effectiveness of probiotic Labneh supplemented with garlic or onion oil against Schistosoma mansoni in infected mice. Int J Dairy Sci. (2008) 3:97–104. doi: 10.3923/ijds.2008.97.104
43. Moazeni M, Nazer A. In vitro effectiveness of garlic (Allium sativum) extract on scolices of hydatid cyst. World J Surg. (2010) 34:2677–81. doi: 10.1007/s00268-010-0718-7
44. Buchmann K, Jensen PB, Kruse KD. Effects of sodium percarbonate and garlic extract on Ichthyophthirius multifiliis theronts and tomocysts: in vitro experiments. N Am J Aquacult. (2003) 65:21–24. doi: 10.1577/1548-8454(2003)065<0021:EOSPAG>2.0.CO;2
45. Jurd L, King AD Jr, Mihara K. Antimicrobial properties of umbelliferone derivatives. Phytochermstry. (1971) 10:2965–70. doi: 10.1016/S0031-9422(00)97333-3
46. Duncan SH, Leitch EC, Stanley KN, Richardson AJ, Laven RA, Flint HJ, et al. Effects of esculin and esculetin on the survival of Escherichia coli O157 in human faecal slurries, continuous-flow simulations of the rumen and colon and in calves. Br J Nutr. (2004) 91:749–55. doi: 10.1079/BJN20041101
47. Wu L, Wang X, Xu W, Farzaneh F, Xu R. The structure and pharmacological functions of coumarins and their derivatives. Curr Med Chem. (2009) 16:4236–60. doi: 10.2174/092986709789578187
48. Kumar R, Saha A, Saha D. A new antifungal coumarin from Clausena excavata. Fitoterapia. (2012) 83:230–3. doi: 10.1016/j.fitote.2011.11.003
49. Dekic BR, Radulovic NS, Dekic VS, Vukicevic RD, Palic RM. Synthesis and antimicrobial activity of new 4-heteroarylamino coumarin derivatives containing nitrogen and sulfur as heteroatoms. Molecules. (2010) 15:2246–56. doi: 10.3390/molecules15042246
50. Mercer DK, Robertson J, Wright K, Miller L, Smith S, Stewart CS, et al. A prodrug approach to the use of coumarins as potential therapeutics for superficial mycoses. PLoS ONE. (2013) 8:e80760. doi: 10.1371/journal.pone.0080760
51. Nonaka M, Murata Y, Takano R, Han Y, Kabir MHB, Kato K. Screening of a library of traditional Chinese medicines to identify anti-malarial compounds and extracts. Malar J. (2018) 17:244. doi: 10.1186/s12936-018-2392-4
52. O'Neill TM, Mansfield JW. Antifungal activity of hydroxyflavans and other flavonoids. Trans Br Mycol Soc. (1982) 79:229–37. doi: 10.1016/S0007-1536(82)80108-3
53. Weidenbörner M, Hindorf H, Jha HC, Tsotsonos P. Antifungal activity of flavonoids against storage fungi of the genus Aspergillus. Phytochemistry. (1990) 29:1103–5. doi: 10.1016/0031-9422(90)85412-9
54. Weidenbörner M, Jha HC. Antifungal activity of flavonoids and their mixtures against different fungi occurring on grain. Pestic Sci. (1993) 38:347–51. doi: 10.1002/ps.2780380412
55. Francolini I, Norris P, Piozzi A, Donelli G, Stoodley P. Usnic acid, a natural antimicrobial agent able to inhibit bacterial biofilm formation on polymer surfaces. Antimicrob Agents Chemother. (2004) 48:4360–5. doi: 10.1128/AAC.48.11.4360-4365.2004
56. Shiva Rani SK, Saxena N, Udaysree N. Antimicrobial activity of black pepper (Piper nigrum L.). Global J Pharmacol. (2013) 7:87–90. doi: 10.5829/idosi.gjp.2013.7.1.1104
57. Zarai Z, Boujelbene E, Salem NB, Gargouri Y, Sayari A. Antioxidant and antimicrobial activities of various solvent extracts, piperine and piperic acid from Piper nigrum. LWT-Food Sci Technol. (2013) 50:634–41. doi: 10.1016/j.lwt.2012.07.036
58. Dzoyem JP, Tangmouo JG, Lontsi D, Etoa FX, Lohoue PJ. In vitro antifungal activity of extract and plumbagin from the stem bark of Diospyros crassiflora Hiern (Ebenaceae). Phytother Res. (2007) 21:671–4. doi: 10.1002/ptr.2140
59. Saowakon N, Lorsuwannarat N, Changklungmoa N, Wanichanon C, Sobhon P. Paramphistomum cervi: the in vitro effect of plumbagin on motility, survival and tegument structure. Exp Parasitol. (2013) 133:179–86. doi: 10.1016/j.exppara.2012.11.018
60. Medina JM, Fernandes RJC, Moreira OC, Atella G, de Souza W, Barrabin H. Mechanisms of growth inhibition of Phytomonas serpens by the alkaloids tomatine and tomatidine. Mem Inst Oswaldo Cruz. (2015) 110:48–55. doi: 10.1590/0074-02760140097
61. Friedman M. Tomato glycoalkaloids: role in the plant and in the diet. J Agricult Food Chem. (2002) 50:5751–80. doi: 10.1021/jf020560c
62. Friedman M, McQuistan T, Hendricks JD, Pereira C, Bailey GS. Protective effect of dietary tomatine against dibenzo[a,l]pyrene (DBP)-induced liver and stomach tumors in rainbow trout. Mol Nutr Food Res. (2007) 51:1485–91. doi: 10.1002/mnfr.200700176
63. Liu J, Kanetake S, Wu Y-H, Tam C, Cheng LW, Land KM, et al. Antiprotozoal effects of the tomato tetrasaccharide glycoalkaloid tomatine and the aglycone tomatidine on mucosal trichomonads. J Agric Food Chem. (2016) 64:8806–10. doi: 10.1021/acs.jafc.6b04030
64. Schulz G, Sander H. über Cholesterin-Tomatid, eine neue Molekfilverbindung zulr Analyvse und Praiparativen Gewinnung von Steroiden. Z Phyisiol Chem. (1957) 308:122–6. doi: 10.1515/bchm2.1957.308.1.122
65. Steel CC, Drysdale RB. Electrolyte leakage from plant and fungal tissues and disruption of liposome membranes by α-tomatine. Phytochemistry. (1988) 27:1025–30. doi: 10.1016/0031-9422(88)80266-8
66. Sandrock RW, VanEtten HD. Fungal sensitivity to and enzymatic degradation of the phytoanticipin alpha-tomatine. Phytopathology. (1998) 88:137–43. doi: 10.1094/PHYTO.1998.88.2.137
67. Tedesco P, Fioravanti ML, Galuppi R. In vitro activity of chemicals and commercial products against Saprolegnia parasitica and Saprolegnia delica strains. J Fish Dis. (2019) 42:237–48. doi: 10.1111/jfd.12923
68. Kaminskyj SG, Heath IB. Age-dependent differential responses of Saprolegnia hyphal tips to a helical growth-inducing factor in the agar substitute, gellan. Exp Mycol. (1992) 16:230–9. doi: 10.1016/0147-5975(92)90031-L
69. Dimmock JR, Elias DW, Beazely MA, Kandepu NM. Bioactivities of chalcones. Curr Med Chem. (1999) 6:1125–49.
70. Go ML, Wu X, Liu XL. Chalcones: an update on cytotoxic and chemoprotective properties. Curr Med Chem. (2005) 12:483–99. doi: 10.2174/0929867053363153
71. Nowakowska Z. A review of anti-infective and anti-inflammatory chalcones. Eur J Med Chem. (2007) 42:125–37. doi: 10.1016/j.ejmech.2006.09.019
72. Svetaz L, Tapia A, López SN, Furlán RL, Petenatti E, Pioli R, et al. Antifungal chalcones and new caffeic acid esters from Zuccagnia punctata acting against soybean infecting fungi. J Agric Food Chem. (2004) 52:3297–300. doi: 10.1021/jf035213x
73. Svetaz L, Agüero MB, Alvarez S, Luna L, Feresin G, Derita M, et al. Antifungal activity of Zuccagnia punctata Cav.: evidence for the mechanism of action. Planta Med. (2007) 73:1074–80. doi: 10.1055/s-2007-981561
74. Seo YH, Kim SS, Shin KS. In vitro antifungal activity and mode of action of 2′,4′-dihydroxychalcone against Aspergillus fumigatus. Mycobiology. (2015) 43:150–6. doi: 10.5941/MYCO.2015.43.2.150
75. Flores S, Montenegro I, Villena J, Cuellar M, Werner E, Godoy P, et al. Synthesis and evaluation of novel oxyalkylated derivatives of 2′,4′-dihydroxychalcone as anti-oomycete agents against bronopol resistant strains of Saprolegnia sp. Int J Mol Sci. (2016) 17:1–12. doi: 10.3390/ijms17081366
76. Kumar A, Raman RP, Kumar K, Pandey PK, Kumar V, Mohanty S, et al. Antiparasitic efficacy of piperine against Argulus spp. on Carassius auratus (Linn. 1758): in vitro and in vivo study. Parasitol Res. (2012) 111:2071–6. doi: 10.1007/s00436-012-3054-z
77. Innocent BX, Fathima SAM, Sivarajani S. Immune response of Catla catla fed with an oral immunostimulant Plumbago rosea and postchallanged with Aeromanas hydrophila. Int J Appl Biol Pharm Technol. (2011) 2:447–54.
78. Larsen J, Lange L, Olson LW. Mastigomycotina developmental sensitivity to α, β unsaturated carbonyl compounds. J Phytopathol. (1992) 134:336–42. doi: 10.1111/j.1439-0434.1992.tb01242.x
Keywords: Amyloodinium ocellatum, Saprolegnia sp., in vitro test, natural compounds, alternative treatments
Citation: Tedesco P, Beraldo P, Massimo M, Fioravanti ML, Volpatti D, Dirks R and Galuppi R (2020) Comparative Therapeutic Effects of Natural Compounds Against Saprolegnia spp. (Oomycota) and Amyloodinium ocellatum (Dinophyceae). Front. Vet. Sci. 7:83. doi: 10.3389/fvets.2020.00083
Received: 15 October 2019; Accepted: 03 February 2020;
Published: 21 February 2020.
Edited by:
Francesca Mancianti, University of Pisa, ItalyReviewed by:
Jason Kindrachuk, University of Manitoba, CanadaCopyright © 2020 Tedesco, Beraldo, Massimo, Fioravanti, Volpatti, Dirks and Galuppi. This is an open-access article distributed under the terms of the Creative Commons Attribution License (CC BY). The use, distribution or reproduction in other forums is permitted, provided the original author(s) and the copyright owner(s) are credited and that the original publication in this journal is cited, in accordance with accepted academic practice. No use, distribution or reproduction is permitted which does not comply with these terms.
*Correspondence: Roberta Galuppi, cm9iZXJ0YS5nYWx1cHBpQHVuaWJvLml0
Disclaimer: All claims expressed in this article are solely those of the authors and do not necessarily represent those of their affiliated organizations, or those of the publisher, the editors and the reviewers. Any product that may be evaluated in this article or claim that may be made by its manufacturer is not guaranteed or endorsed by the publisher.
Research integrity at Frontiers
Learn more about the work of our research integrity team to safeguard the quality of each article we publish.