- 1Department of Natural Sciences, Issyk-Kul State University, Karakol, Kyrgyzstan
- 2Karakol Anti-plague Department, Republic Center of Quarantine and Dangerous Infections, Karakol, Kyrgyzstan
- 3Republic Center of Quarantine and Dangerous Infections, Bishkek, Kyrgyzstan
- 4Reference-Laboratory, Kazakh Scientific Center of Quarantine and Zoonotic Diseases, Almaty, Kazakhstan
- 5Institute of Evolutionary Sciences, University of Montpellier, Montpellier, France
- 6Department of Microbiology and Immunology, Medical Branch, University of Texas, Galveston, TX, United States
- 7Division of Vector-Borne Diseases, Centers for Disease Control and Prevention, Fort Collins, CO, United States
The main purpose of this study was to clarify the role of gray marmots (Marmota baibacina) in the long-term maintenance of highly virulent strains of Yersinia pestis in two plague endemic foci of the Tien Shan Mountains in Kyrgyzstan. We present data from regular observations of populations of M. baibacina and small rodents cohabiting with marmots in the mountainous grasslands of the Sari-Dzhas (east of Issyk-Kul Lake) and the Upper-Naryn (south of Issyk-Kul Lake) natural foci. During 2012–2017, an abundance of marmots and their ectoparasites (fleas and ticks) was significantly higher in Upper-Naryn comparing to Sari-Dzhas, although there were no differences in a number and diversity of small rodents cohabiting with marmots. The plague bacterium was detected either in marmots or in their ectoparasites collected during 4 of 6 years of observation in Sari-Dzhas and during 2 of 4 years of observation in Upper-Naryn. Plague was found in three sectors situated closely to each other in Sari-Dzhas and in 1 of 8 repeatedly surveyed sectors in Upper-Naryn. During 6 years, we isolated 9 strains of Y. pestis from marmots, two from their fleas Oropsylla silantiewi, one from an unidentified tick, and one from the gray hamster (Cricetulus migratorius). All plague strains isolated from the rodents and their ectoparasites in this study were similar to Antiqua biovar specific for marmots. The results indicate that plague can circulate continuously in the Tien Shan Mountains in populations of gray marmots and their ectoparasites with a facultative involvement of other rodent species after significant changes in rodent communities that happened in Kyrgyzstan during the previous two decades. The simultaneous field survey of two natural foci of plague, Sari-Dzhas, and Upper-Naryn, would be important for further analysis of circulation of Y. pestis strains belonging to Antiqua biovar in the Tien Shan Mountains.
Introduction
One of the most active plague endemic areas in the world is located in Kyrgyzstan, Central Asia. There are two independent foci of plague in the Issyk-Kul province: the Sari-Dzhas (east of Issyk-Kul Lake) and the Upper-Naryn (south of Issyk-Kul Lake) sub-regions of the Tien Shan natural focus of plague (1, 2). Several plague outbreaks involving natural vectors, including the gray marmots (Marmota baibacina) and other rodents, have been registered there since 1907 (3). Most of the 5,000 km2 Sari-Dzhas natural plague focus is located in Kyrgyzstan (4,250 km2), with a part extending to Kazakhstan (750 km2). The 8,000 km2 Upper-Naryn area is located in the Naryn and Issyk-Kul regions of Kyrgyzstan.
The first plague outbreak with high human mortality was recorded in the Kyrgyzstan part of Tien Shan focus in 1907, and the last big outbreak of plague occurred in 1928 in the Bash-Kaindi settlement of Atbashi district resulting in 54 deaths (Upper-Naryn mezofocus) (4). Sporadic human cases were also seen in 1965 and 1982. In 2013, a 15-year-old boy who ate marmot meat died from plague in Kyrgyzstan. The Kyrgyz Ministry of Health established a temporal quarantine in parts of the country's mountainous northeast (5), where the risk of plague is well-known for a century. The endemic areas were investigated previously starting from 1942, and the last activity of plague was reported there in 1983 (4, 6, 7).
Gray marmots (M. baibacina) are well-known hosts of multiple zoonotic diseases, out of which plague presents the greatest danger to people (8–10). The gray marmots prefer grasslands and shrubs and avoid woodlands, including even the forest edges and forest-steppe areas with a tree cover <10% (11). Marmot fleas are actively involved in transmission of plague pathogen between animals (12–14). Marmots can host the following species of fleas: Oropsylla silantiewi, Rhadinopsylla li ventricosa, Ceratophyllus lebedewi, and Pulex irritans; the first two flea species are host-specific for marmots (15, 16).
Human activities, such as agriculture, hunting, recreation, and degradation of natural habitats, may dramatically influence plague manifestations (17). Changes in landscape potentially can lead to altering the rodent and flea communities that in turn affect plague transmission cycle (18). Plague in Asian natural systems is commonly spatially stable and corresponds with distribution of primary rodent hosts that can support a strong association of specific strains of Yersinia pestis in mammalian communities (19). Marmots in mountainous plague foci of Central Asia carry a specific strain of Y. pestis (20). Disturbance of evolutionary relations between plague pathogen and their rodent hosts, such as marmots, may result in unpredictable dynamics of the infection.
The collapse of the Soviet Union and subsequent separation of anti-plague station in Kyrgyzstan from the federal anti-plague system led to dramatic reduction of plague investigations and plague control measures. During the last couple of decades, the density of marmots was significantly reduced as a result of chemical suppression in the 1960s, increased hunting of marmots, habitat destruction, and climate changes (6–8, 21). This raises the question whether the changes in rodent communities in these areas affected marmots playing a leading role in circulation of plague pathogen. Thus, the present study aimed to clarify the role of gray marmots in the long-term maintenance of the highly virulent strains of Y. pestis in two plague foci of the Tien Shan Mountains in Kyrgyzstan. The human case in 2013 attracted attention to the situation that plague is endemic to the territory of Kyrgyzstan and the urgent need to obtain new information about the status of the epidemiological situation in these plague foci.
Materials and Methods
Fieldwork
In June–August 2012–2017, the Karakol Anti-Plague Department (KAPD) of the Republic Center for Quarantine and Dangerous Infections (RCQDI) organized field surveys within the Sari-Dzhas and Upper-Naryn foci. The fieldwork was organized as described by Weaver et al. (22) and Sariyeva et al. (6, 7). All work with wild and laboratory animals and plague strains was conducted in accordance with the regulations and protocols approved by the Ministry of Health of Kyrgyzstan (23) in 2015. The procedures were similar to those described by Aytkuluyev (24) and Ezhlova et al. (25). The animal work in the field was performed according to the Regulations approved by the State Agency for Environmental and Forest Protection of the Kyrgyz Republic (details of permits are presented in Table 1). Each permit was issued for collecting a certain number of animals (400 marmots, 300–400 rodents of other species, and excavating 10 nests of marmots and rodents to look for nest parasites) and used during a fixed period of time (from June 1 to August 30) in specific areas within plague foci.
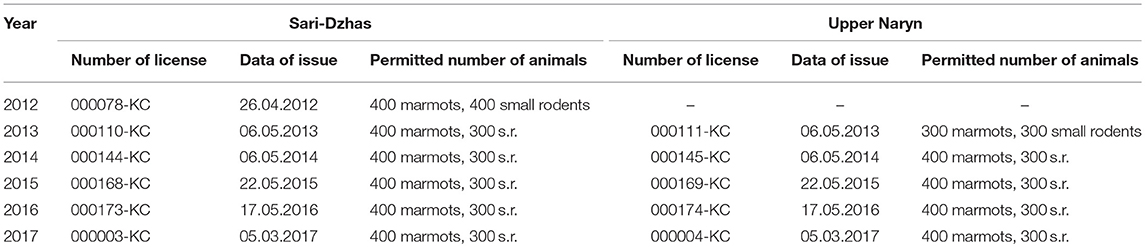
Table 1. Numbers and dates of permits for trapping of animals for epizootological research in 2012–2017.
Trapping Rodents
At the beginning of the annual anti-plague field work, zoologists visually estimated a number of marmots within study sites and accordingly planned trapping efforts in each sector (10 km2). The number of installed traps depends on the average density of marmot population in each sector. Around 8–10 traps were set next to marmot burrows each day at early morning. As the weather in May–June in high-altitude areas of Tien Shan is highly variable with snow, rains, and cold weather, the trapping was conducted during each sunny day. The marmot traps were set at the entrance of burrows and removed by evening. One trap remained at each burrow for half a day. The burrows for trapping were chosen based on external signs such as presence of fresh litter at the entrance, presence of freshly excavated and well-rammed entrances, grass coverage, presence of marmot footprints, and paths around the burrow. The traps used for capturing marmots were 20 cm wide and 8 cm high (Figure 5). The traps were attached to 50-cm-long metal rods with a strong metal chain. The rods are driven into the rocky soil to their full depth to prevent marmots or their predators from dragging the traps away. The metal parts were masked by horse manure to reduce smell of metal.
A number of small rodents, such as mice, voles, and hamsters, were estimated by counting animals captured by handmade snap traps set in transects (1, 25). The snap traps were also sprinkled with soil and baited with dry bread soaked in vegetable oil, vegetables, or fruit. We placed traps in the evening and checked them in the early morning. If there was a captured rodent, we transferred the trap to another spot. The predators were estimated by trapping and visual observations. Each trapped animal was morphologically identified by species. The captured marmots were euthanized by cervical dislocation (25). Then, the animal was wrapped in two linen sacs with label (date, place, number of sector, and name of catcher), the inlet was tightly wrapped so that the ectoparasites could not escape, and then the animal was placed in a plastic bag, in a canvas bag, and finally brought to camp.
Parasitological Analysis
Ectoparasites of marmots were collected by combing the captured animals (“body” ectoparasites) and by collecting “off-host” ectoparasites from rodent burrows and nests (25). Ectoparasites were then identified using entomological keys (26) and placed in labeled glass tubes with ether. Inside burrows, dry grass bedding from the nesting chamber was carefully removed with a wire and was searched for fleas and other ectoparasites. Fleas of one species from a single animal were pooled and triturated in saline solution.
Bacteriological Analysis
The triturated flea suspension was inoculated on Hottinger agar with pH 7.2 (27) (bacteriological approach). The inoculation pool consisted of 20 ectoparasites of one species, collected from the same sector during several days and stored before inoculation in glass tubes without ether. If the animal exhibited any pathological manifestation, the ectoparasites from the pool were inoculated individually. Identification of the isolated bacteria was carried out by standard microbiological microscopy of smears and by Pokrovsky's test for Y. pestis using both pseudo-tuberculosis bacterial phage and bacteriophage for Y. pestis (L-413-C) produced by the Kazakh Scientific Center for Quarantine and Zoonotic Diseases (KSCQZD). Additional diagnostic methods included serological indirect hemagglutination assay with both erythrocyte–immunoglobulin and erythrocyte–antigen diagnostic reagents produced by KSCQZD. The tissues of internal organs of sampled marmots (liver, lung, spleen, lymph nodes, and blood from the heart) were sterilely taken with forceps and immediately placed on an agar plate by directly touch. A Petri dish was divided into three segments to separately plate lungs, livers, and spleens. Each plate was marked and placed in an incubator at 37°C. We used individual and pool methods of inoculation. The individual method was used for ectoparasites collected from carcass of marmot or marmot with visual pathological abnormalities relevant to plague (pathology of inner organs, sick marmots). For inoculation of pooled tissue suspensions, we combined tissue pieces from five marmots collected on the same day from the same sector. Then, the organ suspension was inoculated on agar plate with a bacterial inoculating loop. After inoculation, tissue samples were placed in liquid nitrogen (Dewar flask) and transported to the laboratory in Karakol for further confirmation.
Genetic Analysis
The genetic analysis was performed using two types of material: whole-genome DNA samples and MLVA fragments (Table 2). The 14 studied Y. pestis strains were obtained from the RCQDI collection. The strains were isolated during the last several years in the territory of Sari-Dzhas and Upper-Naryn plague foci (Table 2). The suspension of Y. pestis cultures was heated at 100°C for 20 min and centrifuged at 12,000 rpm for 2 min. This allowed the inactivation of the pathogens and the release of the DNA in the cells. The DNA samples were further used for genotyping. The control MLVA amplification fragments of the reference strains CO92, Pestoides F, KIM10+, and Nepal 516 of Y. pestis were obtained from the University of Texas Medical Branch, Texas, USA. In addition, nucleotide sequences of nine Y. pestis species (GenBank accession numbers: CP010023, CP010247, CP006751, CP009935, AE017042, CP006806, CP000308, CP006794, and CP002956) and three Yersinia pseudotuberculosis strains (GenBank accession numbers: CP009712, CP008943, and CP001048) were used for phylogenetic analysis. The studied strains belonging to the Y. pestis species was confirmed using the “Pest-Quest” PCR assay (“Master-Gene” Limited Liability Partnership, Almaty, Kazakhstan). The genotyping of several Y. pestis strains was performed by the methods of Multi-Locus VNTR Analysis (MLVA) and Melt Analysis of Mismatch Amplification Mutation Assay (Melt-MAMA). For MLVA assay, seven VNTR (Variable Number Tandem Repeats) loci were studied using conventional PCR and agarose gel electrophoresis as described by Le Flèche et al. (28). For Melt-MAMA assay, three sets of PCR primers designed and produced by KSCQZD were used to identify three SNP loci, described by Morelli et al. (29). The selected SNP loci allowed differentiation of four branches of Y. pestis bv. Antiqua (0.ANT1, 0.ANT2, 0.ANT3, and 3.ANT). The Melt-MAMA analysis was performed as described by Birdsell et al. (30). Each primer set consisted of two forward primers (“ancestral” and “derived”) and a universal reverse primer. One of the forward primers was linked to the so-called GC-clamp, three to four repeats of a GGGGC motif, which increased the melting temperature of the corresponding amplicons and made it possible to differentiate the alleles. Phylogenetic analysis of the studied strains was carried out using the PAUP 4.0 software and the UPGMA (Unweighted Pair Group Method with Arithmetic Mean) algorithm.
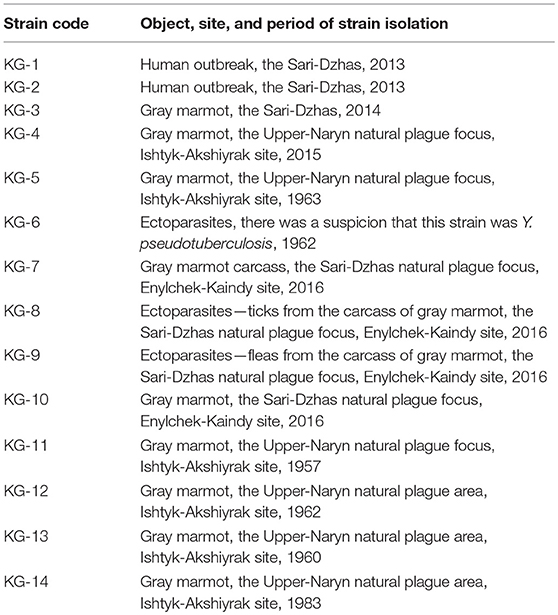
Table 2. Strains of Y. pestis isolated in the Sari-Dzhas and Upper-Naryn plague foci used for DNA analysis.
Mapping
The map of Sari-Dzhas and Upper-Naryn areas of plague was prepared using GIS MapInfo Professional 7.8. For their construction, we used the geographic objects digitized from topographic maps of a scale of 1:100,000, as well as the Digital Elevation Model GDEM2. The maps are constructed in UTM—the Mercater projection (WGS 84). The boundaries of the foci are plotted along the boundaries of the sectors (21).
Results
Sari-Dzhas Plague Focus
Rodents
Overall, in the Sari-Dzhas plague focus for the period 2012 to 2017, we surveyed a total of 3,700 km2, out of which 500 km2 were surveyed repeatedly in 2012, 2013, 2014, and 2016 (Figure 1; Table 4). In total, we trapped and analyzed 972 marmots (M. baibacina), 442 narrow-headed voles (Microtus gregalis), 61 wood mouse (Apodemus uralensis), 40 gray dwarf hamsters (Cricetulus migratorius), and a small number of other mammals: the large-eared pika (Ochotona macrotis), the hare-tolai (Lepus tolai), and the beech marten (Martes foina) (Table 3). Additionally, we found carcasses of six dead rodents.
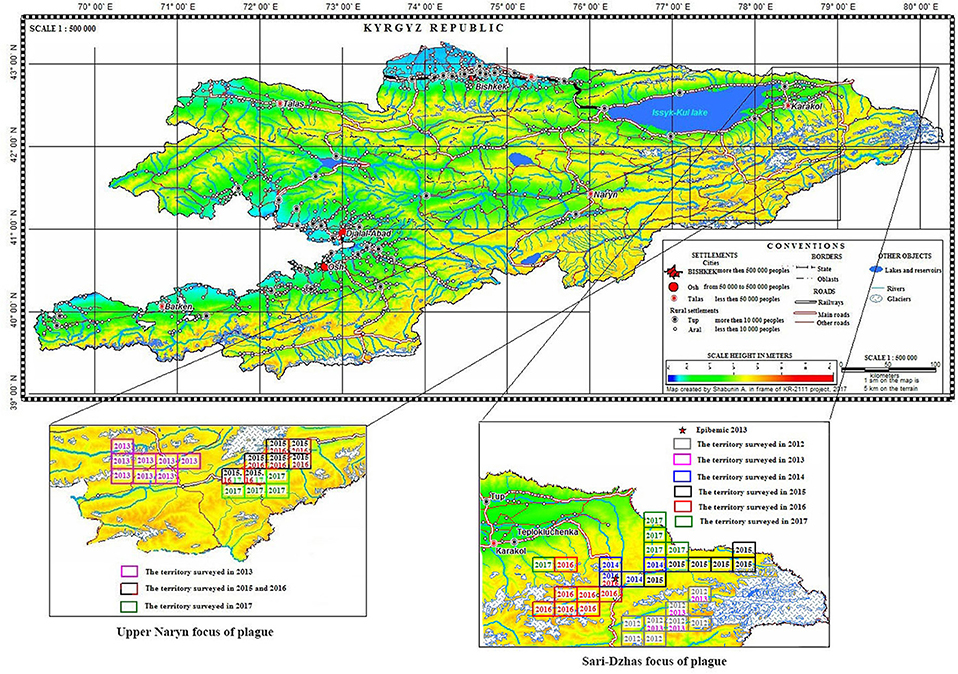
Figure 1. Localization of the Sari-Dzhas and Upper-Naryn natural plague foci and conducted field activities in 2012–2017 (—the territory survived in 2012;
—the territory survived in 2013;
—the territory survived in 2014;
—the territory survived in 2015;
—the territory survived in 2016;
—the territory survived in 2017).
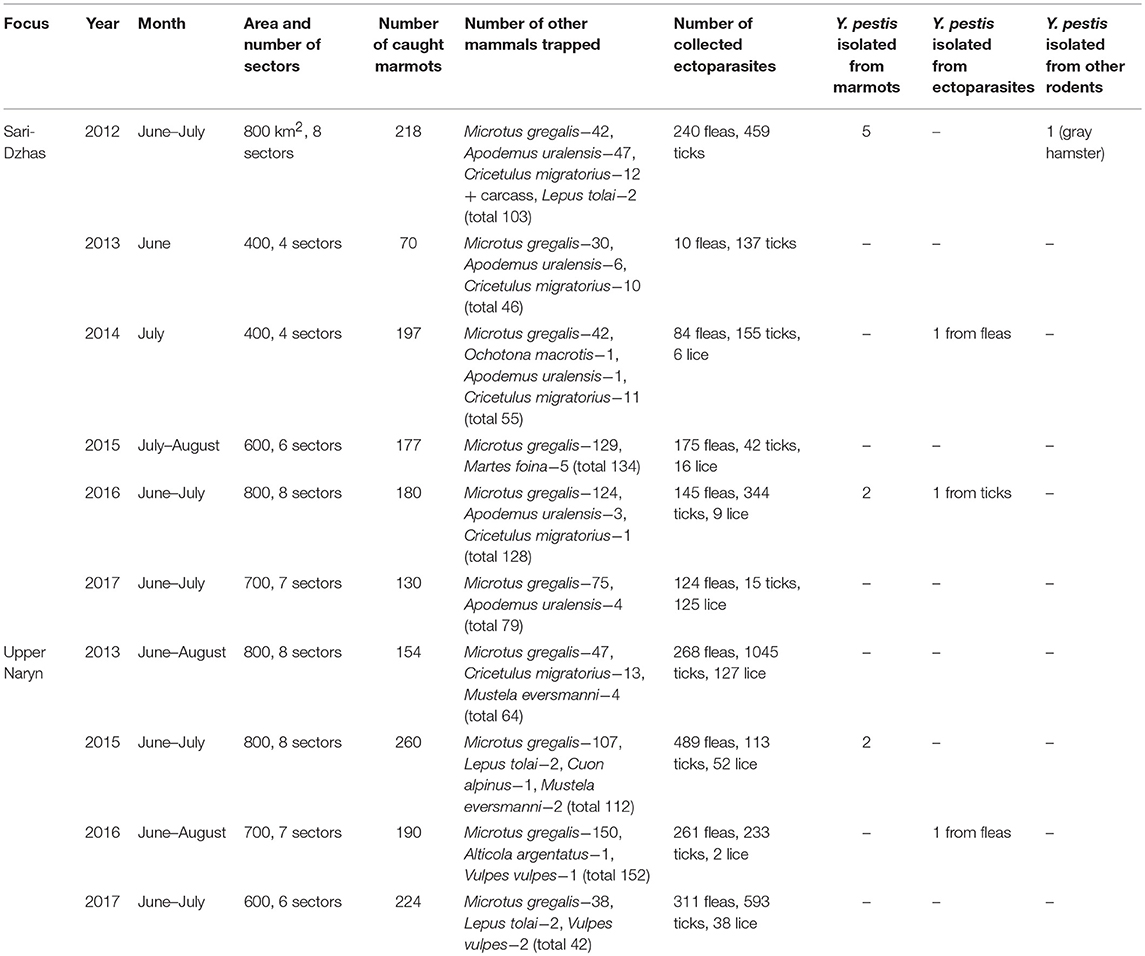
Table 3. Field survey of the Sari-Dzhas and Upper Naryn sites of the Tien Shan high-altitude focus of plague in 2012–2017.
From all sampled marmots, we collected and tested 778 fleas, 1,152 ticks, and 156 lice (Table 3). The average number of sampled marmots ranged from 17 to 27 animals per sector in 2012 and 2013 (Table 4). The highest number of trapped marmots (49 ± 7.62) was recorded in 2014 in a forested area with preferable conditions such as vegetation and humidity. In 2015–2017, the number of collected marmots was similar to 2012. Among small rodents, the most common species was M. gregalis, which is widely distributed across all observed territories (Tables 3, 4). The density of population of M. gregalis and A. uralensis varied significantly. A reverse correlation between abundance of these two species was observed. A high number of M. gregalis was associated with limited or no A. uralensis (Table 4).
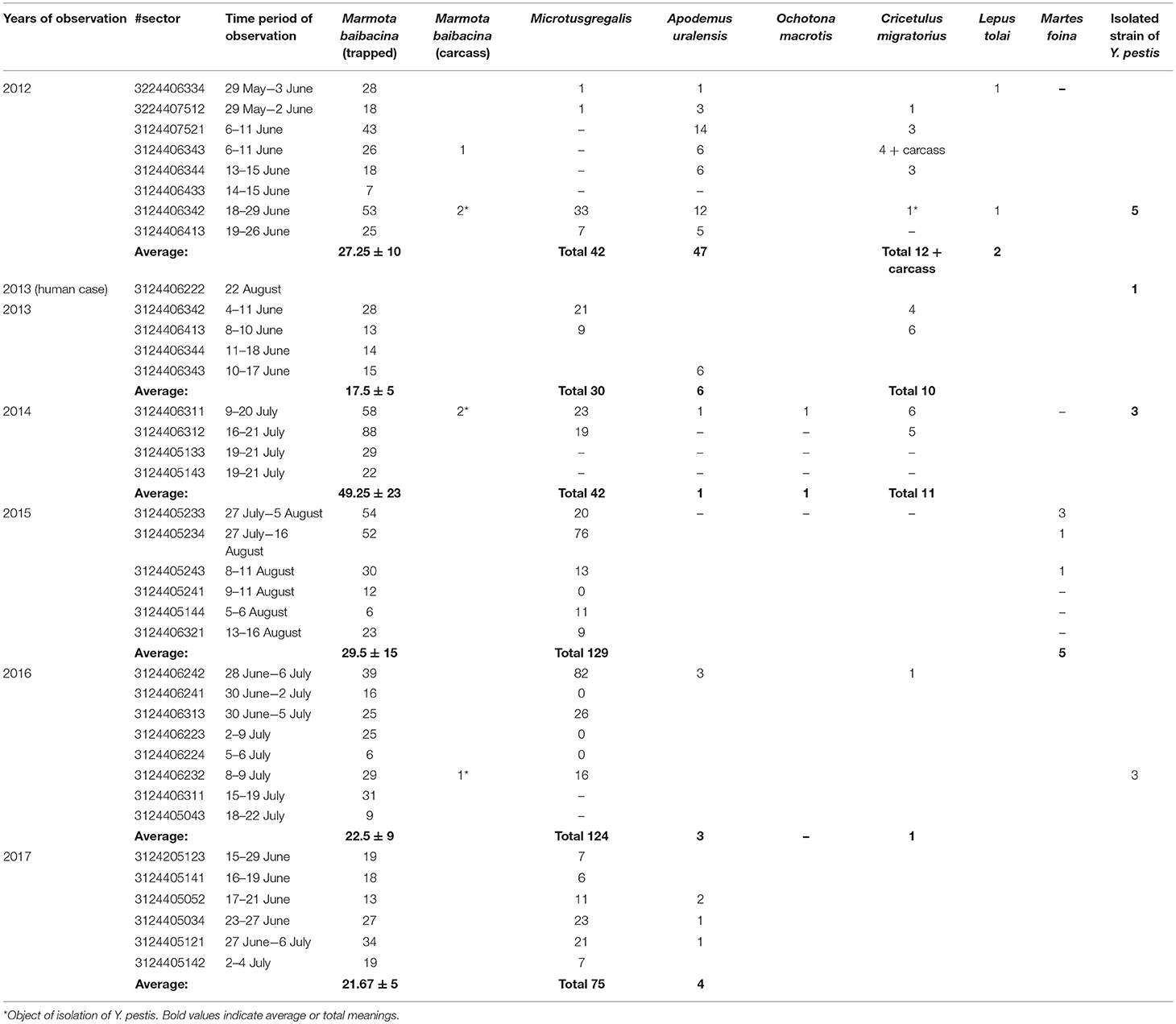
Table 4. The number of trapped mammals in open stations of the Sari-Dzhas natural plague focus in 2012–2017.
Comparing the total number of collected marmots in Sari-Dzhas for 2013 and 2015–2017 with that in Upper-Naryn, the last one has much higher value on the whole area observed in this period (Figure 2). At the same time, there were no differences in the total number of trapped rodents and other mammals in these periods (2013, 2015–2017) between the two plague foci (Figure 2).
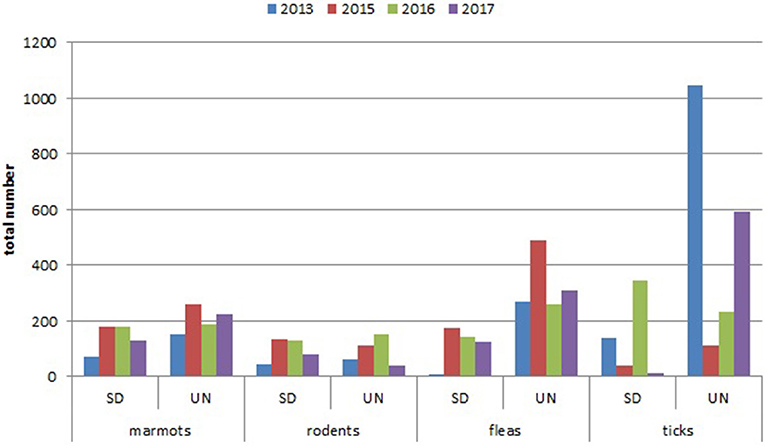
Figure 2. Year dynamics of plague hosts and carriers in Sari-Dzhas and Upper-Naryn plague foci (SD, Sari-Dzhas natural plague focus; UN, Upper-Naryn natural plague focus).
Ectoparasites
The number of ticks collected from marmots was three to five times higher than fleas collected within all sectors of both investigated plague foci (Tables 5, 7; Figure 2). In the Upper-Naryn natural plague area, the average number of fleas and ticks was significantly higher than in the Sari-Dzhas in all years, except ticks in 2016 (Figure 2).
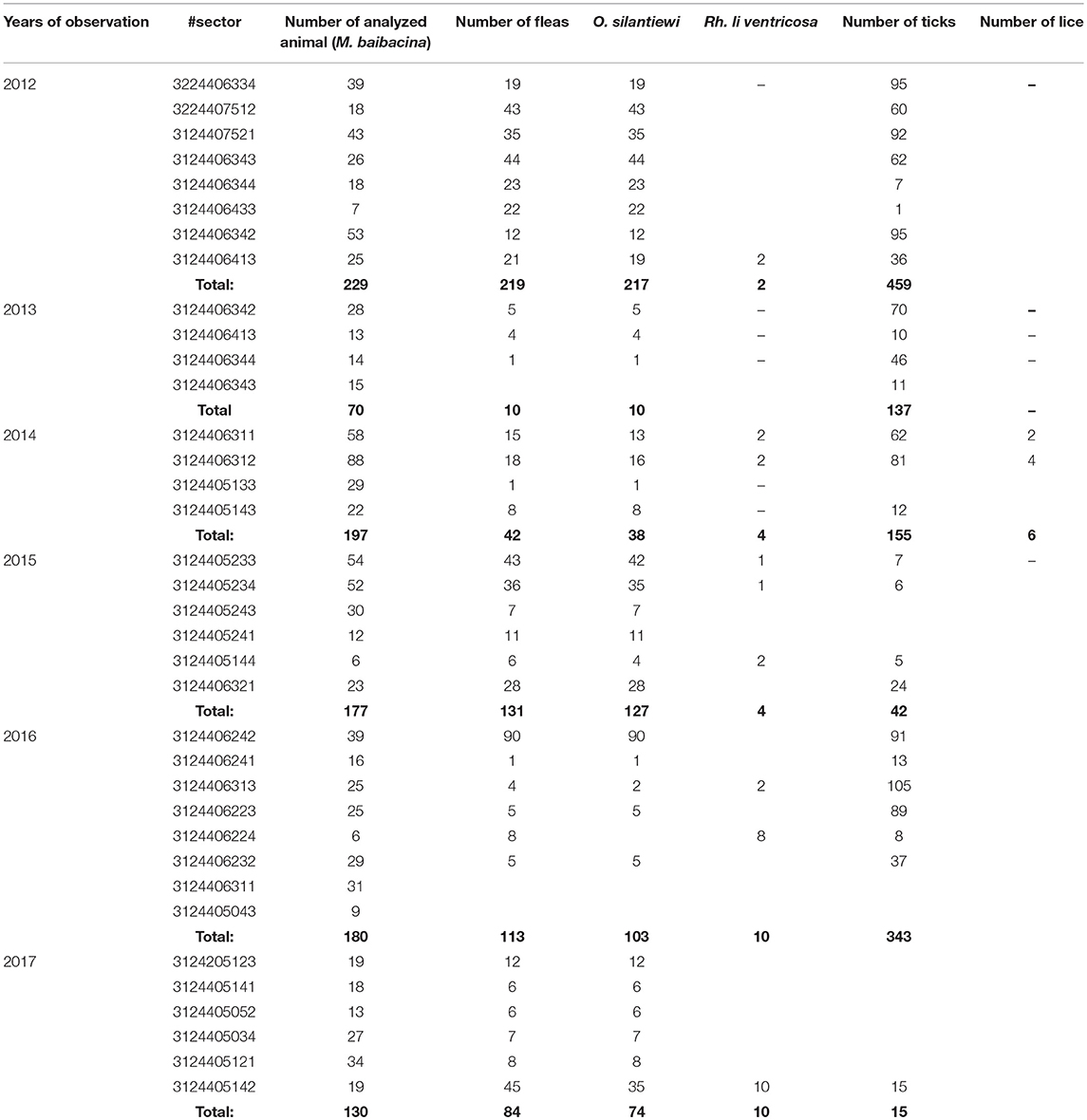
Table 5. The number of “body-ectoparasites” collected from trapped marmots in open stations of the Sari-Dzhas natural plague focus in 2012–2017.
Isolation of Y. pestis
In 2012 after the 29-year period between outbreaks, one observed an acute epizootic of plague in its primary (M. baibacina) and secondary carriers (C. migratorius). In total, five strains of Y. pestis were isolated during the current study: two strains were from found carcasses of marmots, two were from trapped marmots, and one strain was from C. migratorius (Tables 3, 4). All strains were isolated from marmots trapped or found in one sector from eight studied in 2012 within the southeast part of the Sari-Dzhas focus (Figure 4).
In 2013, the human case of plague was registered on the north-west part of the Sari-Dzhas focus, sector #3124406222 (Table 4; Figure 4). In 2014, the plague epizootic in marmots with involved host-specific fleas (O. silantiewi) was in the neighbor sector #3124406311. Three strains of Y. pestis were isolated from found carcasses of marmots and fleas, collected from them, and confirmed serologically.
In 2016, the field study was conducted near the area observed in 2014–2015, with one sector overlapping between surveys (Figures 1, 4). As a result of this investigation, three strains of plague pathogen were isolated—two from M. baibacina (one strain from the pool inoculation of marmots captured in this area and second from a carcass of marmot found in another sector, Table 4). The third strain was isolated from pooled unidentified mites collected from a plague-positive carcass.
In 2013 and 2015, different areas of the Sari-Dzhas plague focus were surveyed with plague-negative result (Figure 1).
Three strains were isolated in the Upper-Naryn: two from marmots in 2015 and one from ectoparasites collected from marmots in 2016. All three strains were isolated in the same sector #3224407344 (Figure 4; Table 6).
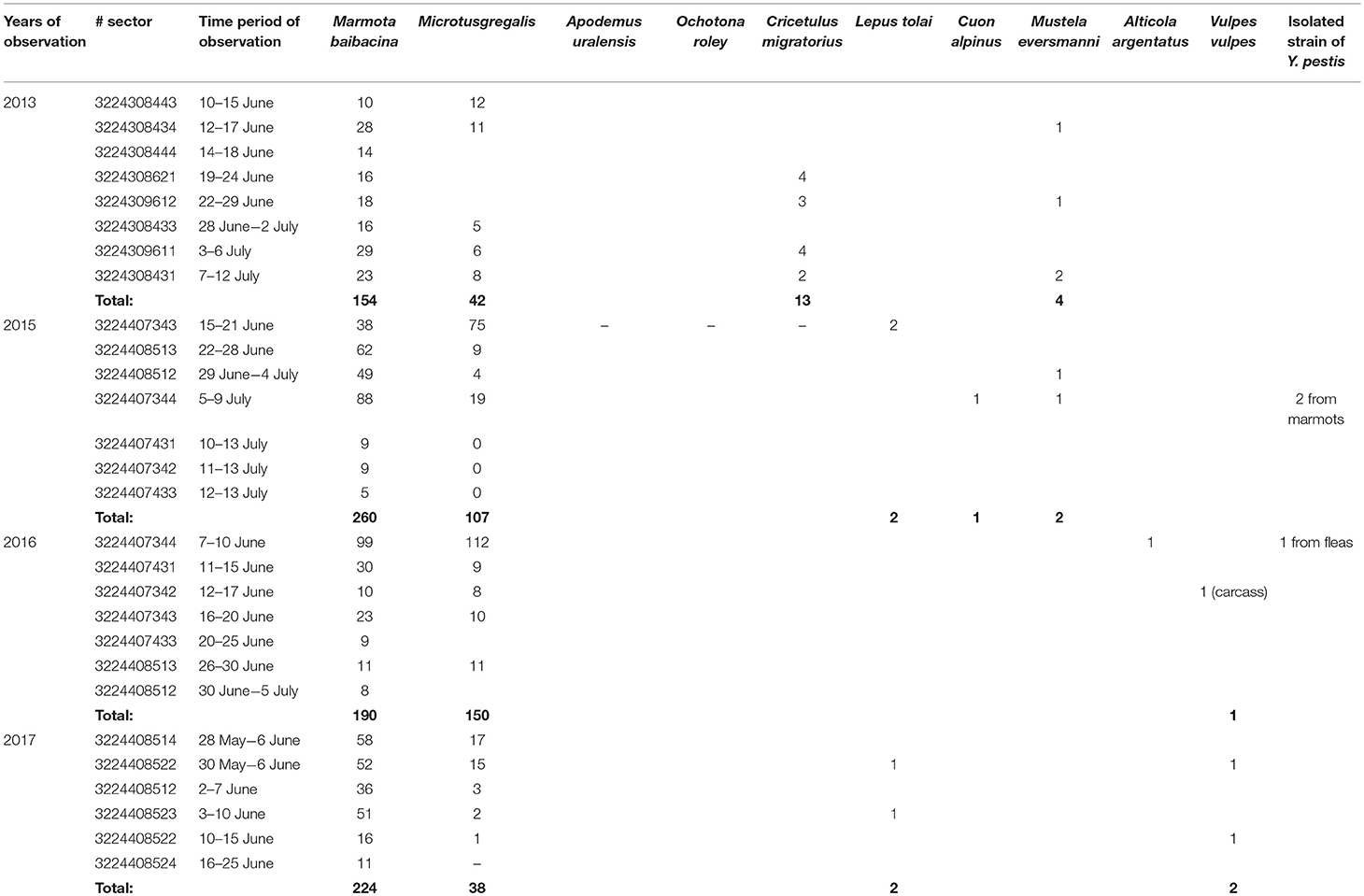
Table 6. The number of trapped mammals in open stations of the Upper Naryn natural plague focus in 2013–2017.
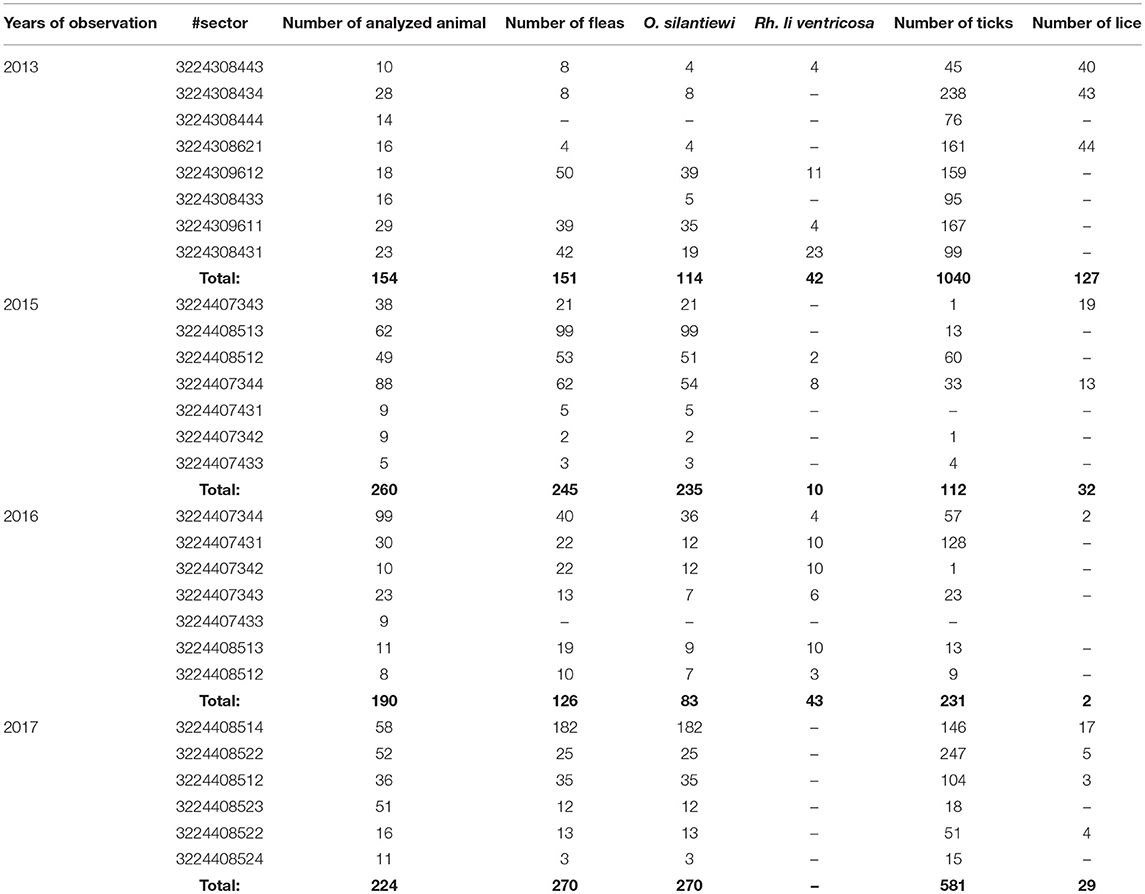
Table 7. The number of “body-ectoparasites” collected from trapped marmots in open stations of the Upper-Naryn natural plague focus in 2013–2017.
The Upper-Naryn Plague Focus
During 2013–2017, a total of 2,900 km2 were investigated in the Upper-Naryn focus, out of which we surveyed 700 km2 repeatedly in 2015, 2016, and 2017 (Figure 1; Table 3). In total, 828 marmots, 342 narrow-headed voles (M. gregalis), 13 gray dwarf hamsters (C. migratorius), and a small number of other mammals: six steppe polecats (Mustela eversmanni), four tolai hares (L. tolai), one red fox (Vulpes vulpes) were trapped and screened (Table 6). Additionally, we found the carcass of a dead fox.
From all sampled marmots, we collected and tested 1,329 fleas, 1,984 ticks, and 219 lice (Table 3). As a result, in 2015–2016, a plague epizootic was recorded in the same sector (Figure 4; Table 6). Y. pestis strains were isolated from M. baibacina and their specific fleas—O. silantiewi.
Results of the Genetic Analysis
The application of the “Pest-Quest” PCR assay confirmed that all the studied strains from Sari-Dzhas plague focus belonged to the Y. pestis species. Based on the results of the MLVA-7 genotyping, all the studied Y. pestis isolates were presented by nine genotypes differing in one or several VNTR loci. Five strains (KG-1–KG-5) shared the same MLVA-7 profile (probably clones of the same strain). The software we used for phylogenetic analysis showed them not as one branch, but as a bunch of sub-branches (Table 8). All 14 strains were assigned to biovar Antiqua that was considered to be typical for marmot strains (Figure 3). According to the Melt-MAMA analysis, most of the strains belonged to the branch 0.ANT2, while one strain (KG-14) was apparently a member of the branch 0.ANT3 (Table 9). These two branches were among those reported by Eroshenko et al. (31) in Kyrgyzstan (along with branches 0.ANT5, 0.PE4t, and 2.MED1).
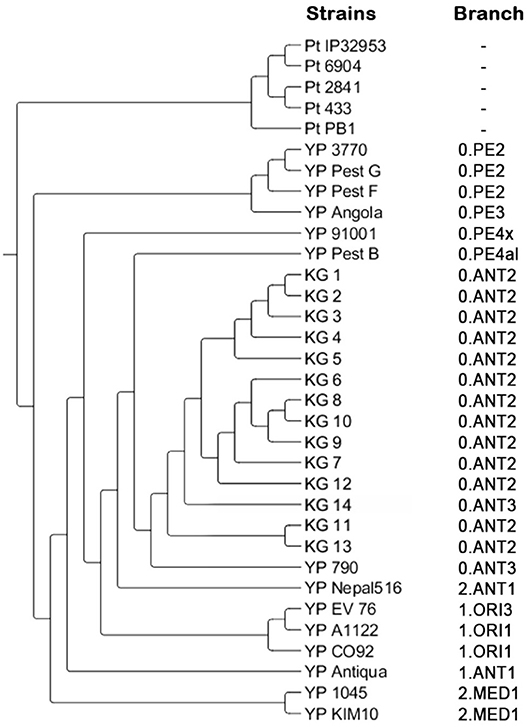
Figure 3. Phylogenetic tree created based on the results of MLVA and SNP analysis. Pt, Y. pseudotuberculosis control strains; YP, Y. pestis control strains; KG, Y. pestis strains isolated on the Kyrgyz territory of Sari-Dzhas and Upper-Naryn plague foci.
Discussion
The greater part of both Sari-Dzhas and Upper-Naryn plague foci were studied simultaneously in 2013 and 2015–2017. If an epizootic of plague was observed once, the area was repeatedly examined in the succeeding years. This allows us to compare two independent plague origin areas for its epizootological status during the same time period. In the Tien Shan mountain focus of plague, the gray marmot is known as the main carrier of plague pathogen, with other rodents serving as secondary hosts (2, 8, 32, 33). The spatial distribution of marmots within plague focus is related mainly to local landscape and climatic conditions, as well as to different human activity–animal grazing, hunting, and tourism that are intensively developing during the last decade, but unequally presented in different sectors (21). Actually, the high-altitude pastures for horses and sheep (so called “syrts”) are more distant from human settlements in the Upper-Naryn area than in Sari-Dzhas. A higher distance to humans may favor the increase in density of marmots in Upper-Naryn compared to Sari-Dzhas where most of the syrts are tightly used for summer seasonal pastures and for international tourism. The disturbance of the environment may affect the marmots leading to a decrease in their population sizes. The number of other small rodents and their variability and density are approximately equal in simultaneously studied years in both plague foci. Prevalence of M. gregalis confirms the role of this species as a potential secondary host of plague pathogen in mixed ecosystems of high-altitude Tien Shan focus. Isolation of one Y. pestis strain from C. migratorius in the Sari-Dzhas focus confirms the role of small mice-like rodents in the epidemiology of plague. Previously, plague strains were isolated in the neighboring Aksay focus from M. gregalis (1968) and C. migratorius (1983–1984) and in the Upper-Naryn focus from Alticola argentatus and C. migratorius (3). Similar observations were reported in the high altitude of Altay and Tuva plague foci of Russia (34) and the North Aral sandy plague focus of Kazakhstan (35). Increasing density of rodents co-inhabiting with the marmots altogether with an increased number of their specific ectoparasites as observed in the Upper-Naryn focus in 2013–2017 could be a sign of potential activity of plague epizootics in this area of Kyrgyzstan. The isolation of 9 of 13 strains from marmots in 2012–2017, 1 strain from other rodents, and 3 strains from their ectoparasites is in favor of this hypothesis. Both independent plague foci (Sari-Dzhas and Upper-Naryn), located on the border of Kyrgyzstan, Kazakhstan, and China, are among the most active natural high-altitude foci of Central Asia. This territory is characterized by special climatic conditions, different relief with many heavily rugged canyons, mountain river valleys, and patches with specific flora and fauna with a high degree of biological diversity (36). Apparently, these factors give optimal conditions for the long-term circulation of Y. pestis biovar Antiqua (0.ANT2, 0.ANT3) in the populations of its natural host (31, 37). In total, 462 strains of Y. pestis were registered in the Sari-Dzhas area from 1944 to 1976 (4, 6, 7). Ectoparasites are actively involved in the epizootic process, in particular O. silantiewi and Rh. li ventricosa fleas specific to M. baibacina, as well as ixodes mites. The Sari-Dzhas area was very active in the 1940s to 1980s, and then mass disinsection of marmot populations with dichlorodiphenyltrichloroethane (DDT) insecticide and the decrease in the number of animals as a result of human activities (special extermination conducted in 1960s, hunting) led to a significant decrease in epizootic activity. Whereas, human activity (agriculture development, tourism, and hunting) changed environment during this period significantly, an obvious activation of epizootic plague activity within the area was observed after 30 years. Overall, the obtained results confirm ongoing epidemiological risk and vulnerability of the territory to plague. In such situation, it is necessary to strengthen ecological and epidemiological monitoring and control over the entire endemic area in order to preserve safety of local populations.
Ethics Statement
All manipulations with wild and laboratory animals and handling plague strains were conducted according to the protocols approved by the Kyrgyz government regulations that are related to guidelines of Russia, Kazakhstan (1, 25). The animal work was performed according to the Regulation of the State Agency for Environmental and Forest Protection Facilities at the Government of the Kyrgyz Republic. Animal work in the field was conducted according to the following protocols: protocol 000144-KC for trapping marmots, other rodents, and analysis of their nests approved on May 6, 2014; protocol # 000168-KC approved on May 22, 2015; protocol #000173-KC approved on May 17, 2016; and protocol #000003-KC approved on March 5, 2017. Each permit to collecting a certain number of animals (400 marmots, 400 rodents of other species, and 10 nests of marmots and rodents) was issued and used during the fixed period of time (from June 1 to August 30).
Author Contributions
GS initiated the study and wrote the first draft. GB organized and conducted the field studies and performed zoological and entomological analysis. RMu performed agreement with the state agencies for animal catching and analysis. SA provided strains for DNA analysis and genotyping. BK and AA performed molecular-genetic analysis of strains. AS performed GIS-mapping and database design. ZS performed bacteriological analysis and spatial epizootical analysis. AD performed the bacteriological analysis. ZA analyzed the environmental effect on population structure. RMa provided consultative assistance. SM provided collaborative efforts. VM edited the draft and provided reference strains. MK edited the draft and provided collaborative efforts. All authors read and approved the final manuscript.
Funding
This study was supported by the International Science and Technology Center (ISTC). The field epizootical surveys and other analyses were funded through regular projects #KR-1784 and #KR-2111 with financial support from the Government of Canada, EU, and USA. The authors are very grateful for the assistance given by both ISTC and international collaborators of the projects.
Conflict of Interest Statement
The authors declare that the research was conducted in the absence of any commercial or financial relationships that could be construed as a potential conflict of interest.
Acknowledgments
We thank Kenneth Gage and Irina Goodrich for the careful reading of the manuscript and constructive comments.
References
1. Aubakirov SA, Serzhanov OS, Fomushkin VM, Khotko II, Rudenchik UV, Pole SB, et al. Guidelines for the Landscape-Epizootic Zoning of Natural Foci of Plague in Central Asia and Kazakhstan. Kazakh Scientific Center of Qurantine and Zoonotic Diseases, Alma-Ata (1990).
2. Atshabar BB, Burdelov LA, Ageyev VS, Aubakirov SA, Dubyansky VM, Grazhdanov AK, et al. Atlas of the Spread of Bacterial and Viral Zoonotic Infections in Kazakhstan. Almaty: Reforma (2010).
3. Ibragimov Abdikarimov ST, Usenbaev NT, Altybaev KI. Some of the results of non-specific prevention of plague foci in the highland of the Kyrgyz Republic. Health Care Kyrgyzstan. (2017) 4:30–3. Available online at: https://elibrary.ru/item.asp?id=32741738
4. Abdikarimov S, Sariyeva G, Sagiyev Z, Bazarkanova G, Shabunin A, Maimulov R, et al. The Database “Epidemiology and Epizootology of the Plague in the Sari-Dzhas Natural Focus of Kyrgyzstan.” Intellectual property—Official Bulletin of the State Service of Intellectual Property and Innovations under the Government of the Kyrgyz Republic (2017) 80–1.
5. Popov NV, Kedrova OV, Toporkov VP. Information Letter “On the Situation of the Plague in the Kyrgyz Republic in August 2013”. Available online at: http://www.microbe.ru/files/infletter_2013_1.pdf
6. Sariyeva G, Abdel Z, Shabunin A, Sagiyev Z, Abdikarimov S, Bazarkanova G, et al. Current status of the Sari-Dzhas natural focus of plague, Kyrgyzstan: epizootic activity and marmot population. Vector Borne Zoonotic Dis. (2018) 18:524–32. doi: 10.1089/vbz.2017.2200
7. Sariyeva GE, Bazarkanova GD, Sagiyev ZA, Shabunin AG, Abdikarimov ST, Maimulov RK, et al. Atlas of the Sari-Dzhas natural focus of plague in Kyrgyzstan: Spatial and temporal characteristics. Bishkek: Global Print (2018), p. 172.
8. Pole SB. Marmots and zoonotic infections in CIS. In: Ramousse R, Allainé D, Le Berre M, editors. Adaptive Strategies and Diversity in Marmots/Stratégies Adaptatives et Diversité chez les Marmottes 2003. Lyon: International Network on Marmots (2003). p. 13–8.
9. Armitage KB. Climate change and the conservation of marmots. Nat Sci. (2013) 5:36–43. doi: 10.4236/ns.2013.55A005
10. Dai R, Wei B, Xiong H, Yang X, Peng Y, He J, et al. Human plague associated with Tibetan sheep originates in marmots. PLoS Negl Trop Dis. (2018) 12:e0006635. doi: 10.1371/journal.pntd.0006635
11. Ricankova V, Riegert J, Semancíkova E, Hais M, Cejkova A, Prach K. Habitat preferences in gray marmots (Marmota baibacina). Acta Theriol. (2014) 59:317–24. doi: 10.1007/s13364-013-0161-x
12. Reijniers J, Begon M, Ageyev VS, Leirs H. Plague epizootic cycles in Central Asia. Biol Lett. (2014) 10:20140302. doi: 10.1098/rsbl.2014.0302
13. Eisen RJ, Borchert JN, Mpanga JT, Atiku LA, MacMillan K, Boegler KA, et al. Flea diversity as an element for persistence of plague bacteria in an East African plague focus. PLoS ONE. (2012) 7:e35598. doi: 10.1371/journal.pone.0035598
15. Vashchenok VS. The role of fleas (Siphonaptera) in the epizootology of plague. Parazitology. (1999) 33:198–209.
16. Suntsov VV, Suntsova NI. Plague. Origin and Evolution of the Epizootic System (Ecological, Geographical and Social Aspects). Moscow: KMK Publishing House (2006). p. 247.
17. Collinge SK, Johnson WC, Ray C, Matchett R, Grensten J, Cully JF, et al. Landscape structure and plague occurrence in black-tailed prairie dogs on grasslands of the Western USA. Landscape Ecol. (2005) 20:941–55. doi: 10.1007/s10980-005-4617-5
18. Gage KL, Kosoy MY. Natural history of plague: perspectives from more than a century of research. Annu Rev Entomol. (2005) 50:505–28. doi: 10.1146/annurev.ento.50.071803.130337
19. Biggins DE, Kosoy M. Influences of introduced plague on North American mammals: implications from ecology of plague in Asia. J Mamm. (2001) 82:906–16. doi: 10.1644/1545-1542(2001)082<0906:IOIPON>2.0.CO;2
20. Lowell JL, Zhansarina A, Yockey B, Meka-Mechenko T, Stybayeva G, Atshabar B, et al. Phenotypic and molecular characterizations of Yersinia pestis isolates from Kazakhstan and adjacent regions. Microbiology. (2007) 153:169–77. doi: 10.1099/mic.0.29059-0
21. Shabunin AG, Sarieva GE, Abdikarimov ST, Maimulov RK, Bazarkanova GD, Dzhaparova AK, et al. Assessment of the vulnerability of the population on Sari-Dzhas autonomous focus of plague, Kyrgyzstan. Acta Biomed Scientifica. (2017) 2:107–14. doi: 10.12737/article_59fad5258950d0.72474236
22. Weaver ML, Sariyeva GE, Kendirbaev DU. Biosafety in the field: an account of a Kyrgyz anti-plague expedition. Appl Biosafety. (2014) 19:74–86. doi: 10.1177/153567601401900203
23. Order No. 207 of 04/27/2015. “On the Improvement of Epidemiological and Epidemiological Surveillance of the Plague in the Kyrgyz Republic”. p. 87–92.
24. Aytkuluyev NS. Instructions on the conducting of anti-epidemic and preventive measures for plague, approved by the order of the ministry of health care of Kyrgyzstan 102 from 24.02.2010. (2010) 12–13.
25. Ezhlova EB, Pakskina ND, Popov VP, Knyazeva TB, Kuznetzov AA, Porshakov AM, et al. Collection, Recording and Preparation for Laboratory Investigation of Blood-Sucking Arthropods in Natural Foci of Dangerous Infectious Diseases: Methodological guidelines. MU 3.1.3012-12. Federal Center for Hygiene and Epidemiology of Russian Surveillance on Consumer Rights Protection and Human Wellbeing (2011) 55:14.
26. Ioff IG, Michelin MA, Skalon OI. Determinant of Fleas of Central Asia and Kazakhstan. Moscow: Medicine (1965). p. 11–21.
27. Suleymenov BM, Abdirasilova AA. The Basis of Individual Biosafety Under Work With Plague Pathogen. Almaty: Evero (2014). p. 200.
28. Le Flèche P, Hauck Y, Onteniente L, Prieur A, Denoeud F, Ramisse V, et al. A tandem repeats database for bacterial genomes: application to the genotyping of Yersinia pestis and Bacillus anthracis. BMC Microbiol. (2001) 1:2–11. doi: 10.1186/1471-2180-1-2
29. Morelli G, Song Y, Mazzoni CJ, Eppinger M, Roumagnac P, Wagner DM, et al. Phylogenetic diversity and historical patterns of pandemic spread of Yersinia pestis. Nat Genet. (2010) 42:1140–43. doi: 10.1038/ng.705
30. Birdsell DN, Pearson T, Price EP, Hornstra HM, Nera RD, Stone N, et al. Melt analysis of mismatch amplification mutation assays (Melt-MAMA): a functional study of a cost-effective SNP genotyping assay in bacterial models. PLoS ONE. (2012) 7:e32866. doi: 10.1371/journal.pone.0032866
31. Eroshenko GA, Nosov NY, Krasnov YM, Oglodin YG, Kukleva LM, Guseva NP, et al. Yersinia pestis strains of ancient phylogenetic branch 0.ANT are widely spread in the high mountain plague foci of Kyrgyzstan. PLoS ONE. (2017) 12:e0187230. doi: 10.1371/journal.pone.0187230
32. Burdelov LA. Modern Ideas About State of the Art of Natural Plague Foci: An Overview. Problems of Quarantine and Zoonotic Infections in Kazakhstan, Alma-Ata: Kazakh Scientific Center of Quarantine and Zoonotic Diseases. (1999). p. 18–23.
33. Bibikov DI. Mountain Marmots of Central Asia and Kazakhstan. Moscow: Moscow University Press (1967). p. 202.
34. Popov NV, Bezsmertny VE, Matrosov AN, Kuznetsov AA, Knyzeva TV, Popov VP, et al. Epizootic activity of natural plague foci in the territory of the Russian Federation in 2011, and prognosis for 2012. Prob Danger Infect. (2012) 111:5–10. doi: 10.21055/0370-1069-2012-1(111)-5-10
35. Varshavskiy BS. Spatial and biocenotic structure of the plague focus on the northern edge of the area of the great gerbil (Northern Aral Sea). (Dissertation thesis) : Astrakhan anti-plague station, Astrakhan, Russia (1985).
36. Mamatkanov DM, Bazhanova LB, Kuzmichenok VA, Romanovsky VV. Influence of Climate Changes on the Mountain Ecosystem of the Tien-Shan (on the Example of the Issyk-Kul-Chui basin). Bishkek: Nur-As Press (2014). p. 273.
Keywords: grey marmot, ectoparasites, plague, rodent, Kyrgyzstan, Yersinia pestis
Citation: Sariyeva G, Bazarkanova G, Maimulov R, Abdikarimov S, Kurmanov B, Abdirassilova A, Shabunin A, Sagiyev Z, Dzhaparova A, Abdel Z, Mussagaliyeva R, Morand S, Motin V and Kosoy M (2019) Marmots and Yersinia pestis Strains in Two Plague Endemic Areas of Tien Shan Mountains. Front. Vet. Sci. 6:207. doi: 10.3389/fvets.2019.00207
Received: 19 December 2018; Accepted: 11 June 2019;
Published: 04 July 2019.
Edited by:
David Modrý, University of Veterinary and Pharmaceutical Sciences Brno, CzechiaCopyright © 2019 Sariyeva, Bazarkanova, Maimulov, Abdikarimov, Kurmanov, Abdirassilova, Shabunin, Sagiyev, Dzhaparova, Abdel, Mussagaliyeva, Morand, Motin and Kosoy. This is an open-access article distributed under the terms of the Creative Commons Attribution License (CC BY). The use, distribution or reproduction in other forums is permitted, provided the original author(s) and the copyright owner(s) are credited and that the original publication in this journal is cited, in accordance with accepted academic practice. No use, distribution or reproduction is permitted which does not comply with these terms.
*Correspondence: Gulmira Sariyeva, c2FyaWV2YS5nJiN4MDAwNDA7aWtzdS5rZw==