- 1Institute of Infection and Global Health, University of Liverpool, Liverpool, United Kingdom
- 2International Livestock Research Institute, Nairobi, Kenya
The zoonotic helminth T. solium is one of the leading causes of acquired epilepsy in endemic countries, resulting in a high burden both in human health and social stigma of affected people (1–3). In 2012 T. solium was highlighted as a priority for control in the World Health Assembly resolution 66.12 (4). Despite a call for validated control strategies by 2015 and a “Tool Kit” of control options being available, relatively few examples of successfully implemented and sustainable control programs are available (5–7). A minimal control strategy focusing solely on the porcine host has also been proposed although the cost-effectiveness of such has yet to be explored (8). Although acknowledgment has been made of the need for initiatives to be sustainable, we are yet to see sufficient consideration of the balance between the provision of public and private goods, and the need for engagement of the people and organizations in the pork value chains within T. solium control strategies. We utilized a food chain risk analysis model to determine the incremental cost-effectiveness ratio (ICER) in terms of $/infective meal avoided, of combining a pharmaceutical intervention in pigs with strengthened meat hygiene services. The addition of a vaccination and treatment protocol, at an additional 10.3% cost, was illustrated to have the potential to improve the ICER of improving meat inspection by 74.6%. The vaccination and treatment protocol also had the potential to reduce the losses borne by the pork industry of condemned meat by 66%, highlighting the potential to leverage private sector investment in T. solium control.
Introduction
Taenia solium is a zoonotic tapeworm which utilizes a porcine intermediate and a human definitive host. It is thought that T. solium has been associated with a hominid definitive host pre-dating the advent of Homo sapiens (9) and has accompanied modern humans as they colonized the globe (10). Humans acquire a T. solium taeniosis infection through consumption of pork containing viable cysticerci and pigs acquire T. solium cysticercosis through the ingestion of infective eggs or proglottids excreted in the feces of infected humans (11). The ingestion of infective eggs by humans due to fecal contamination of food or drinking water, or auto-infection from a tapeworm carrier, can lead to an aberrant intermediate infection, cysticercosis, with larval cysts found in muscle, optical or neural tissue. Infection of the central nervous tissue, neurocysticercosis (NCC) is considered to be a major causes of acquired epilepsy in endemic counties (12), leading to significant reductions in quality of life (13) and making T. solium the foodborne parasite with the greatest global burden (14).
Improvements in pig production, sanitation and meat hygiene have contributed to the decline in T. solium infection pressure in North America and Europe, although pockets of endemnicity exist where a triad of poor sanitation, free-range pigs and lack of food safety governance are found, making it very much a disease of poverty (15). The three major endemic regions are Africa, Asia, and Latin America (16), although there is evidence that the parasite may still have autochthonous transmission within Eastern Europe (17). Even within individual countries in endemic regions the parasite has a varied spatial and temporal distribution depending on local factors influencing the lifecycle (18).
T. solium taeniosis/cysticercosis has traditionally been considered one of the neglected zoonotic diseases (19–22) but increased advocacy and a growing body of literature detailing the prevalence and burden of this parasite has led to its incorporation into the 2012 London Declaration on Neglected Topical Diseases (NTDs) (23), The WHO Roadmap “Accelerating work to overcome the global impact of neglected tropical diseases” (24) and the World Health Assembly resolution WHA66.12 (25).
Despite this high-level commitment we have yet to significantly advance the control of the parasite on a large scale. As a community, we have failed to achieve the 2015 goal set by the WHO Roadmap, to have a “validated strategy for control and elimination of T. solium taeniosis/cysticercosis available” and are unlikely to meet the 2020 goal for scaled up interventions. A “tool kit” of intervention options are available, each of which has the potential to break the tapeworm lifecycle at different points by focusing on either the human or porcine host (7).
Interventions targeting the human host include improving access to clean water and sanitation, preventative chemotherapy (often in the form of mass drug administration) and wider public health education campaigns (26). Control interventions in the porcine host and associated value chain such as the confinement, anthelmintic treatment or vaccination of pigs can be considered as pre-harvest (26). Post-harvest control covers stringent meat inspection with condemnation of infected meat (27), treatment of meat through freezing (28), gamma-radiation (29), and salt-pickling (30) as well as cooking to over 50°C (30, 31) which also assists in the control of other foodborne pathogens (32–34).
An optimal intervention strategy has not yet been demonstrated in the field and importantly the acceptability and sustainability of these strategies has not been evaluated (6, 35–37), with the cost-effectiveness of control evaluated in only two studies to date (38, 39). A “One Health” approach, with interventions in both the human and non-human animal host has generally been regarded to be necessary for the control of zoonoses such as T. solium (40). It has recently been suggested that a “minimal intervention strategy” targeting only the porcine host through vaccination and anthelmintic treatment of pigs between 2 and 7 months of age may be appropriate (8).
The One Health strategy utilizes the TSOL18 vaccine which has demonstrated 100% protection against porcine cysticercosis under field conditions (41) and which is now produced by India Immunologicals Ltd, India as Cysvax® (8). The anthelmintic treatment to be administered is oxfendazole, administered at 30 mg/kg, now available in some African countries as a 10% formulation for pigs (Paranthic) (8). Oxfendazole (30 mg/kg) has also been demonstrated to also have 100% efficacy against the gastrointestinal nematodes Ascaris suum, Oesophagostomum spp., Trichuris suis and Metastrongylus spp., thereby providing additional benefits to productivity for pig farmers (42). A recent trial of this strategy in Nepal demonstrated a significant reduction in porcine cysticercosis, with elimination of infection in those animals assessed by post-mortem (43).
In an integrated control program in Laos PDR, a pig intervention including the TSOL18 vaccine, oxfendazole (30 mg/kg), was combined with T. solium and soil transmitted helminth control in humans through the mass administration of Albendazole (44). An economic analysis of this program from a societal perspective has been conducted and the combined approach was judged to be highly cost-effective at 214 USD/DALY averted against the GDP per capita of Laos PDR of 1,793 USD (38).
As yet all studies utilizing porcine pharmaceutical interventions have been provided to farmers free of charge. Whilst acknowledging the barriers, including lack of access to finance or credit to make capital investments of feed purchases (45), to improve the pork value chain in endemic areas, it is important to provide value chain actors with the responsibility and agency to deliver a safe and quality product to market, if sustainable control is to be achieved. We suggest that a cost-sharing model between the private and public sector may be a suitable direction to take for T. solium control, based upon the delivery of private (e.g., profit) or public (e.g., food safety) goods through the different control interventions.
We hypothesize that farmers may be incentivized to adopt a control strategy through demonstration of “rewards,” such as increased profitability of the pig production system due to improved husbandry practices or the adoption of judicial use of anthelmintic treatment for gastro-intestinal nematode infections in combination with T. solium control. Behavior change may also be encouraged through potential punishments, such as the condemnation of grossly infected meat at inspection.
Despite the low sensitivity of meat inspection for the detection of T. solium (46), highly infected carcasses are likely to be observed if qualified personnel are present at slaughter, well trained and sensitized to the importance of preventing consumption of infected meat. If meat inspection is carried out according to regulatory standards, a trader or butcher who presents a pig to slaughter carries the full burden of risk should that carcass be condemned at meat inspection as no compensation is received for condemned animals (47).
Changes in demand, toward uninfected pigs, may induce losses for small-holder pig farmers until they adopt T. solium control strategies. It could be hypothesized therefore, that the public expenditure of enforcing meat hygiene regulations may therefore “leverage” investment from the private sector in control measures (48). An example of such would be the purchase of vaccines and anthelmintic treatment for pigs directly by farmers, rather than through publicly funded campaigns.
The current study aimed to explore this hypothesis by determining the incremental cost-effectiveness ratio (ICER) in terms of $/infective meal avoided, of the “minimal intervention strategy” of pharmaceutical intervention in pigs in combination with strengthened meat hygiene services in western Kenya. We utilize a food chain risk analysis modified from Thomas et al. (49) parameterized by data relating to western Kenya although the model parameters may be easily adjusted for use in other settings.
Materials and Methods
Study Area
The data used to parameterize the risk model described below was obtained through previously described studies conducted in a mixed crop-livestock farming community in western Kenya, centered around Busia town on the Uganda border (50, 51). Many pig farmers in this area practice extensive forms of pig production, with three systems predominating; full time free range where pigs are left to scavenge for all their food requirements, part-time free-range where pigs scavenge during the dry season, where during planting or growing seasons they may be tethered or confined with supplemental feedstuffs to prevent crop damage, or full time confined systems which may involve tethering or confining pigs in rudimentary structures and providing supplementary feedstuffs (52–54). These systems are similar to those described in other endemic areas (55–60). Within the study site, 16.6% (95% C.I. 13.1–20.5) of homesteads owned pigs, and the majority of farmers sell their pigs to butchers who transport the pigs to rudimentary, but licensed, slaughter premises for slaughter, with a small, but important proportion (4.3%, 95% C.I 2–12%) of pigs undergoing “back-yard” slaughter (52). By law, a meat inspector must inspect each pig, although low staffing levels and poor facilitation in terms of transport, means that many animals are currently slaughtered without inspection.
Food Chain Risk Analysis
A stochastic risk assessment model, built using the @Risk (Palisade, Newfield, NY, USA) add-on for Excel (Microsoft corp., USA) and the initial parameters (P1–P25) are described in detail in Thomas et al. (49). The structure of the model is illustrated in Figure 1 for ease of reference. This model indicates that any one pork meal consumed in western Kenya has a 0.006 (99% Uncertainty Interval (U.I). 0.0002 ± 0.0164) probability of containing at least one viable T. solium cysticercus at the point of consumption and therefore being potentially infectious to humans (49). We adapted this model to investigate the ICER from a societal perspective of enforcing best practice meat inspection at every registered porcine slaughter facility in Busia county with or without adoption of a regime of Cysvax vaccine and Oxfendazole in pigs at 3 and 6 months of age, adapted from the minimal intervention strategy as recommended by Lightowlers and Donadeu (8).
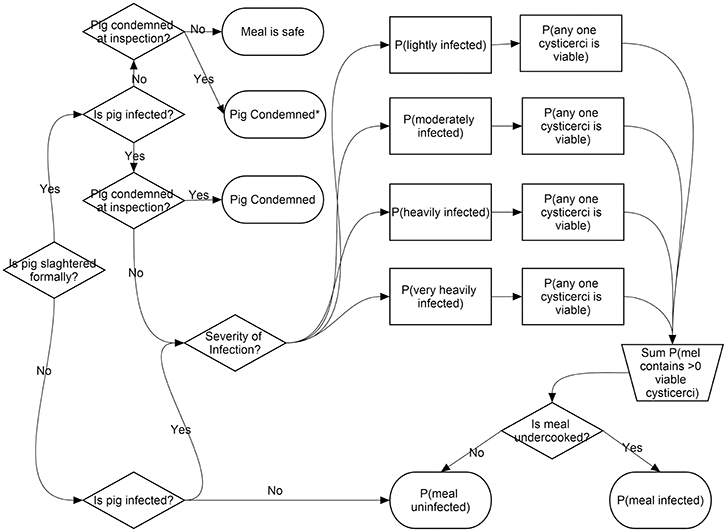
Figure 1. Food chain risk model structure © 2017 Thomas et al. Reproduced from Thomas et al. (49).
The assumptions in this adapted model are as follows:
• Pigs are slaughtered between 7 and 12 months of age
• Adoption of vaccination and treatment protocol was assumed to be 75% (70–80%) of farmers fattening pigs for the “formal” value chain (not those destined for “backyard” slaughter)
• Vaccination and treatment at 3 and 6 months (2 doses) is 100% protective (8, 61), but 1% of treatments may fail through user error
• Vaccination and treatment failure will result in infection profiles equivalent to non-treated pigs (i.e., Proportion of light/medium/heavy infections will be equivalent)
• Meat inspectors will be present at every formal slaughter facility and inspect every pig presented
• The proportion of pigs destined for the informal sector from fattening remains at the baseline level and these farmers do not take up the pharmaceutical intervention.
• Pigs slaughtered in the informal sector obtain only 45% of the price of a formally slaughtered pig (62).
The updated model can be found in Supplementary Material S1. The new model parameters are described in Table 1 and the original model parameters are presented in Thomas et al. (49). Ten additional scenarios were added to the original 15 detailed in the original model (49) and are described as follows:
Scenario 1 = Pig is formally slaughtered/treated/lightly infected/not detected at meat inspection
Scenario 2 = Pig is formally slaughtered/treated/lightly infected/detected at meat inspection/condemned
Scenario 3 = Pig is formally slaughtered/treated/moderately infected/not detected at meat inspection
Scenario 4 = Pig is formally slaughtered/treated/moderately infected/detected at meat inspection/condemned
Scenario 5 = Pig is formally slaughtered/treated/heavily infected/not detected at meat inspection
Scenario 6 = Pig is formally slaughtered/treated/heavily infected/detected at meat inspection/condemned
Scenario 7 = Pig is formally slaughtered/treated/very heavily infected/not detected at meat inspection
Scenario 8 = Pig is formally slaughtered/treated/very heavily infected/detected at meat inspection/condemned
Scenario 9 = Pig is formally slaughtered/treated/uninfected/ not detected at meat inspection
Scenario 10 = Pig is formally slaughtered/treated/ uninfected/ detected at meat inspection (false positive)/condemned
Scenario 11 = Pig is formally slaughtered/Not treated/lightly infected/not detected at meat inspection
Scenario 12 = Pig is formally slaughtered/Not treated/lightly infected/detected at meat inspection/condemned
Scenario 13 = Pig is formally slaughtered/Not treated/ moderately infected/not detected at meat inspection
Scenario 14 = Pig is formally slaughtered/Not treated/moderately infected /not detected at lingual palpation/condemned
Scenario 15 = Pig is formally slaughtered/Not treated/ heavily infected/not detected at meat inspection
Scenario 16 = Pig is formally slaughtered/Not treated/heavily infected/detected at meat inspection/condemned
Scenario 17 = Pig is formally slaughtered/Not treated/very heavily infected/not detected at meat inspection
Scenario 18 = Pig is formally slaughtered/Not treated/very heavily infected/detected at meat inspection/condemned
Scenario 19 = Pig is formally slaughtered/Not treated/uninfected/ not detected at meat inspection
Scenario 20 = Pig is formally slaughtered/Not treated/ uninfected/detected at meat inspection (false positive)/condemned
Scenario 21 = Pig is informally slaughtered/not treated/ lightly infected
Scenario 22 = Pig is informally slaughtered/not treated/ moderately infected
Scenario 23 = Pig is informally slaughtered/not treated/ heavily infected
Scenario 24 = Pig is informally slaughtered/not treated/ v. heavily infected
Scenario 25 = Pig is informally slaughtered/not treated/ uninfected
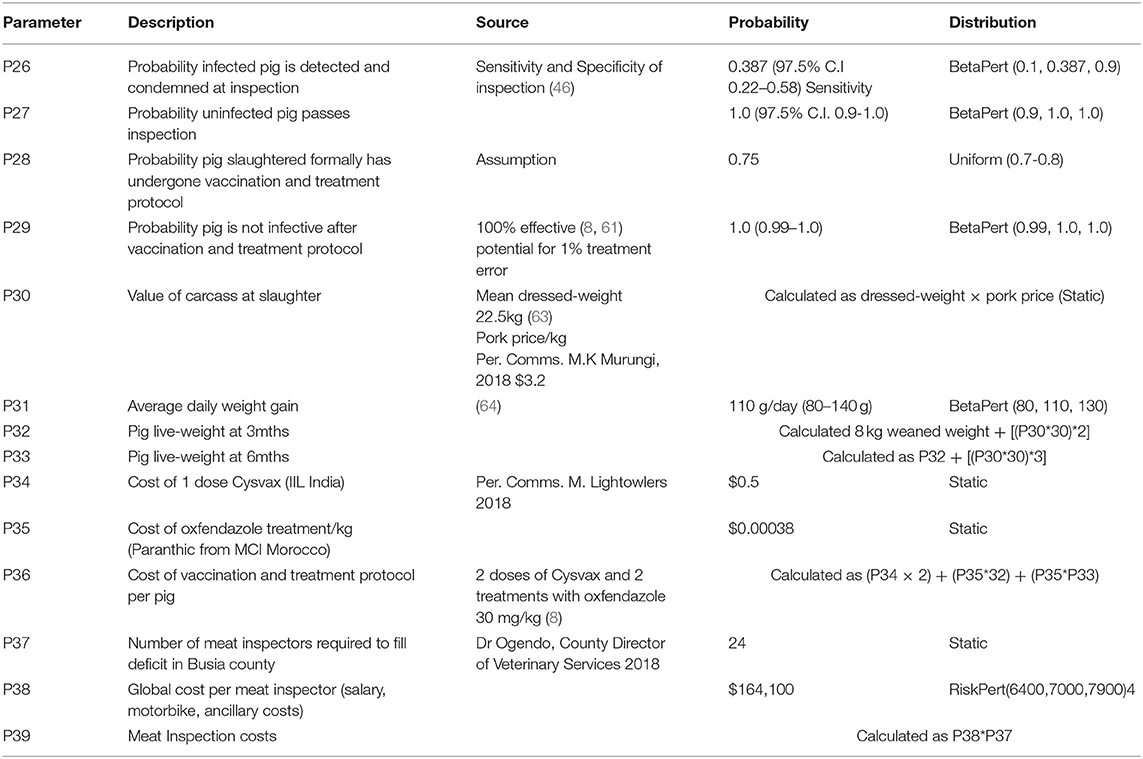
Table 1. New model parameters. Parameters P1–P25 as per original model (49).
The probability of each scenario described is calculated as follows:
And the probability of any one meal being potentially infective at consumption expressed as:
Only partial costs to the pig industry were considered in this analysis, including; the vaccination and treatment protocol, and losses due to carcass condemnation (pork price/kg × carcass weight), feeding and transport of pigs were not included. Costs to the county government considered are the additional cost of staffing all pork slaughter facilities with a qualified meat inspector. The income from meat inspection fees ($1.4 per pig) were not included as these are currently paid for every pig irrespective of the presence of a meat inspector. The interventions are compared through their incremental cost-effectiveness analysis (ICER), calculated according to the equation (65)
Where: Costs are in US$ at 2017 values
A sensitivity analysis was conducted according to the method described previously (49) to determine the most influential parameters on the ICER output.
Results
The models converged after 48,900 iterations, a summary of the results can be found in Table 2. All model inputs and outputs can be found in Supplementary Table 1 (improved meat inspection only) and Supplementary Table 2 (Meat inspection and treatment protocol).
The model suggests that within the context of an improved meat hygiene service, addition of a vaccination and treatment protocol in pigs has the potential to improve the cost-effectiveness of the intervention by 74.6% from $59 (99% U.I $15–$402) to $15 (99% U.I.$ 9.81–$33.75) per infective meal avoided. For farmers, the cost-benefit ratio for adopting the vaccination and treatment protocol is 10.29, due to the resultant reduction in condemnation losses, without considering the potential additional benefits from increase in weight-gain though treatment of gastro-intestinal nematode infections.
Spearmans rank order coefficients (ρ) indicated that the five most influential inputs on the ICER were; the probability of any one cysticercus being viable (ρ = 0.76), the probability that an uninfected pig is correctly passed at meat inspection (ρ = −0.26), the probability that an untreated pig is infected (ρ = 0.22), the probability of a pig being treated (ρ = −0.18) and the mean number of cysts in a heavily infected pig (ρ = 0.17). These five parameters were included in an advanced sensitivity analysis. If all other parameters are fixed, the probability of any one cyst being viable has the largest influence over the ICER, with the mean at 1% of the input value being $11.06 and at 99% of the input value being $22.4.
Discussion
The analysis indicates that from a societal perspective, implementing a vaccination and treatment protocol in pigs has the potential to enhance the incremental cost-effectiveness ratio ($/potential infective event avoided) of a T. solium control intervention based on enforcing meat inspection regulations. It also indicates the potential for public sector investment, in this case in the meat hygiene inspectorate, to leverage private sector investment, e.g., in a vaccination and treatment protocol for pigs, to “insure” the private sector against potential losses due to regulatory standards.
Within the immediate aftermath of tightening meat hygiene regulations it is expected that food producers will incur a degree of financial loss as they adapt to the new regulatory environment (66). Increased costs may relate to carcass or partial carcass condemnation, or from the increased time required for stringent meat inspection to occur. However, it would also be expected that over time these losses would reduce and stabilize as the market adapts to the new environment, with pork traders and butchers seeking pigs from “improved” producers, or pre-screening pigs for infection prior to purchase, in order to reduce their risk. Screening of pigs by pork traders using lingual palpation has already been reported in Tanzania (67) and Zambia (62) and traders in Kenya have expressed an interest in “insurance” against condemnation (47).
Providing small-scale farmers with the responsibility and agency to bring a safe product to market is an important aspect of improving and growing a viable pig industry in T. solium endemic areas. How farmers address the problem of T. solium, alongside other animal health and husbandry issues they may be facing, is essentially an individual decision and the solutions chosen must be relevant to the context in which they are operating. Encouraging farmers to invest in T. solium control interventions may require a “carrot and stick” approach including enforcement of meat hygiene regulations and promotion of the potential profits afforded by producing “safe” pork.
A combination of rewards and punishments, “carrots and sticks,” have been demonstrated to have a stronger effect on eliciting “correct” behavior, than either alone (68). In terms of rewards to the farmer for adopting such pharmaceuticals there are two potential ways in which revenue may be enhanced. The use of oxfendazole also has the potential to improve the profitability of pig farming through the treatment of the gastro-intestinal nematode infections which are prevalent in many small-holder pig systems. An overall gastro-intestinal nematode prevalence of 91% was detected in small-holder pigs in Uganda (69) and of 84.2% in western Kenya (70). Treatment of these infections should lead to improvement in the feed conversion efficiency of these pigs, leading to increased daily weight gain, as has been demonstrated in cattle (71).
Rewards may also come in the form of a price premium, or enhanced market access, for a high quality product, the goal of many private food standards. Willingness-to-pay for pork perceived to be “safe” has been previously demonstrated in China (72), but the ability to pay a “safe pork” premium assumes a level of disposable income which will allow a degree of inelasticity of demand.
In China, where pork is a traditional component of the diet, the price elasticity of pork has been shown to be low (73). In sub-Saharan Africa pork is not a traditional food, though as populations urbanize and incomes rise there is a rapid increase in the volume of pork consumed in the region (74). In Kenya the price elasticity of pork across rural and urban households was also found to be inelastic at 0.96, although closer to the threshold for a “luxury good” than beef, chicken, and goat (75).
Consumption of pork in much of the region is still predominately the domain of those in the upper income brackets. In Kigali, Rwanda for instance pork has been referred to as “Benz” (as in Mercedes Benz) designating it as a high-status product (76), in Uganda the consumption of pork has been shown to be significantly higher among families of higher socio-economic status (77). Within this demographic there may be an ability to pay such a “safe pork premium,” but willingness-to-pay for safe food is not only a product of consumer incomes, but of education, risk perception, cultural food preferences, and access to substitute foodstuffs or food suppliers (72, 78).
Although the model presented here is of course only an approximation of reality, with many assumptions incorporated, it illustrates how providing pig farmers with access to pharmaceutical products such as the Cysvax vaccine and oxfendazole, could substantially reduce exposure of consumers to a dangerous zoonotic infection as well as reduce potential losses to the pork industry from the condemnation of pig carcasses, or through the sale of infected pork through the “informal” sector, assuming that these pigs obtain only 45% of the market value (62).
Field trials have indicated the efficacy of the vaccination and treatment protocol to reduce the prevalence of porcine cysticercosis (43, 61, 79). Studies are now needed to establish farmers' willingness-to-pay for these pharmaceutical products and the likelihood of uptake in the context of different regulatory frameworks. In order to allow smallholder farmers in endemic areas to adopt vaccination and treatment protocols, products must be available through local suppliers of agro-veterinary products, they must be appropriately packaged in appropriate dosages for smallholder famers who own 1–5 pigs and sufficient extension services should be provided to raise awareness of the products.
Protecting the food chain through meat inspection requires that countries formulate and enact appropriate meat hygiene legislation and also that sufficient staff are deployed and facilitated including across potentially inaccessible rural areas. Within the analysis presented here, the total cost of meat inspection services has been allocated to T. solium control, although these services provide goods far beyond this goal. Meat inspection, including ante mortem inspection of the animals arriving for slaughter, provides wider benefits than purely cysticercosis control. By removing diseased animals from the food chain, inspection aims to both reduce zoonotic disease exposure to people and to assist in the detection and control of some livestock diseases thereby providing public goods, which cannot be appropriated by any one individual, to both consumers and the livestock sectors, respectively (80). Meat inspectors, or official veterinarians at meat processing facilities, also have a role in ensuring facility hygiene, a role which provides possibly the most important control on microbial contamination of meat products (81).
Regulatory impact assessments and cost-benefit analysis of meat hygiene regulations would be highly useful for policy makers within endemic counties to enable more efficient allocation of resources within already stretched public budgets. Meat inspectors in Kenya are also trained animal health assistants and their role also incorporates aspects of farm extension and surveillance activities. Ongoing work in western Kenya on the surveillance of zoonotic diseases will enable us to begin quantifying the cost-effectiveness of deploying these professional resources across a range of different surveillance and extension activities. Providing economic data will allow countries to prioritize interventions for the NTDs as they move into the next phase of the roadmap to 2030 (82).
Conclusions
Through the use of a stochastic risk model, we have demonstrated how within the context of enforced meat hygiene legislation, adoption of a porcine vaccination and treatment protocol by farmers may provide a quantifiable economic benefit to the pig industry through a reduction in losses through condemnation. A porcine formulation of oxfendazole (as Paranthic 10%) and TSOL18 (Cysvax) are now in commercial production and licensing is underway in several sub-Saharan African countries, including Kenya. Programmes are now urgently needed to provide access to these products to those who require them, stimulate demand and monitor the uptake and cost-effectiveness of these products if we are to be successful in the global goal to control this important zoonotic parasite.
Author Contributions
LT conceived and produced a first draft of the manuscript. EC, EF, and JR were involved in the development of the ideas presented in the manuscript and assisted in the writing. All authors read and approved the final draft of the manuscript.
Conflict of Interest Statement
The authors declare that the research was conducted in the absence of any commercial or financial relationships that could be construed as a potential conflict of interest.
Acknowledgments
We thank all of the Zoonotic and Emerging Diseases Group (University of Liverpool/ILRI) and our national partners who facilitated the field elements of this study. We are grateful to all our research participants for their willingness to be involved in the research and to the other research teams whose work has informed this model. We also thank the team at Palisade (Newfield, NY, USA) who provided invaluable assistance with the @Risk software and Prof. Bronsvoort and Dr. Handel for their assistance in building the original food chain risk analysis model used in this manuscript. The authors are supported by the Biotechnology and Biological Sciences Research Council, the Department for International Development, the Medical Research Council, the Natural Environment Research Council and the Defense Science and Technology Laboratory, under the Zoonoses and Emerging Livestock Systems (ZELS) programme, grant reference BB/L019019/1, and the CGIAR Agriculture for Nutrition and Health (A4NH) programme, led by the International Food Policy Research Institute (IFPRI). This work was part-funded by the Global Challenges Research Fund (GCRF) One Health Regional Network for the Horn of Africa (HORN) Project, from UK Research and Innovation (UKRI) and Biotechnology and Biological Sciences Research Council (BBSRC) (project number BB/P027954/1). We also acknowledge the CGIAR Fund Donors (hppt://www.cgiar.org/funders/). The funders had no role in the decision to publish or the preparation of this manuscript. Open access publication fees are supported by the University of Liverpool institutional access fund.
Supplementary Material
The Supplementary Material for this article can be found online at: https://www.frontiersin.org/articles/10.3389/fvets.2019.00176/full#supplementary-material
Supplementary Material S1. Adapted food chain risk model. Available online at: https://figshare.com/s/42e6a65deaafc72e1cb6.
Supplementary Table 1. Model inputs and outputs improved meat inspection only.
Supplementary Table 2. Model inputs and outputs improved meat inspection and pharmacological inputs.
References
1. Carabin H, Ndimubanzi PC, Budke CM, Nguyen H, Qian Y, Cowan LD, et al. Clinical manifestations associated with neurocysticercosis: a systematic review. PLoS Negl Trop Dis. (2011) 5:e1152. doi: 10.1371/journal.pntd.0001152
2. Carabin H, Krecek RC, Cowan LD, Michael LM, Foyaca-Sibat H, Nash TE, et al. Estimation of the cost of Taenia solium cysticercosis in Eastern Cape Province, South Africa. Trop Med Int Health. (2006) 11:906–16. doi: 10.1111/j.1365-3156.2006.01627.x
3. Bhattarai R, Budke CM, Carabin H, Proaño JV, Flores-Rivera J, Corona T, et al. Estimating the non-monetary burden of neurocysticercosis in Mexico. PLoS Negl Trop Dis. (2012) 6:e1521. doi: 10.1371/journal.pntd.0001521
4. World Health Organization. Accelerating Work To Overcome The Global Impact of Neglected Tropical Diseases: A Roadmap for Implementation. Geneva: WHO (2012).
5. Okello AL, Thomas LF. Human taeniasis: current insights into prevention and management strategies in endemic countries. Risk Manage Healthcare Policy. (2017) 10:107. doi: 10.2147/RMHP.S116545
6. Carabin H, Traoré AA. Taenia solium taeniasis and cysticercosis control and elimination through community-based interventions. Curr Trop Med Rep. (2014) 1:181–93. doi: 10.1007/s40475-014-0029-4
7. Thomas LF. Landscape analysis: control of Taenia solium. Geneva: World Health Organization (2015).
8. Lightowlers MW, Donadeu M. Designing a minimal intervention strategy to control Taenia solium. Trends Parasitol. (2017) 33:426–34. doi: 10.1016/j.pt.2017.01.011
9. Bobes RJ, Fragoso G, Fleury A, García-Varela M, Sciutto E, Larralde C, et al. Evolution, molecular epidemiology and perspectives on the research of taeniid parasites with special emphasis on Taenia solium. Infect Genet Evol. (2014) 23:150–60. doi: 10.1016/j.meegid.2014.02.005
10. Yanagida T, Carod J-F, Sako Y, Nakao M, Hoberg EP, Ito A. Genetics of the pig tapeworm in Madagascar reveal a history of human dispersal and colonization. PLoS ONE. (2014) 9:e109002. doi: 10.1371/journal.pone.0109002
11. Garcia HH, Gonzalez AE, Evans AWC, Gilman RH. Taenia solium cysticercosis. Lancet. (2003) 362:547–56. doi: 10.1016/S0140-6736(03)14117-7
12. Debacq G, Moyano LM, Garcia HH, Boumediene F, Marin B, Ngoungou EB, et al. Systematic review and meta-analysis estimating association of cysticercosis and neurocysticercosis with epilepsy. PLoS Neglect Trop Dis. (2017) 11:e0005153. doi: 10.1371/journal.pntd.0005153
13. Nau AL, Mwape KE, Wiefek J, Schmidt K, Abatih E, Dorny P, et al. Cognitive impairment and quality of life of people with epilepsy and neurocysticercosis in Zambia. Epilepsy Behav. (2018) 80:354–9. doi: 10.1016/j.yebeh.2017.10.042
14. Torgerson PR, Devleesschauwer B, Praet N, Speybroeck N, Willingham AL, Kasuga F, et al. World health organization estimates of the global and regional disease burden of 11 foodborne parasitic diseases, 2010: a data synthesis. PLoS Med. (2015) 12:e1001920. doi: 10.1371/journal.pmed.1001920
15. Ito A, Yanagida T, Nakao M. Recent advances and perspectives in molecular epidemiology of Taenia solium cysticercosis. Infect Genet Evol. (2015) 40:57–367 doi: 10.1016/j.meegid.2015.06.022
16. Coral-Almeida M, Gabriël S, Abatih EN, Praet N, Benitez W, Dorny P. Taenia solium human cysticercosis: a systematic review of sero-epidemiological data from endemic zones around the world. PLoS Negl Trop Dis. (2015) 9:e0003919. doi: 10.1371/journal.pntd.0003919
17. Devleesschauwer B, Allepuz A, Dermauw V, Johansen MV, Laranjo-González M, Smit SAG, et al. Taenia solium in Europe: still endemic? Acta Trop. (2017) 165:96–9. doi: 10.1016/j.actatropica.2015.08.006
18. Raghava MV, Prabhakaran V, Jayaraman T, Muliyil J, Oommen A, Dorny P, et al. Detecting spatial clusters of Taenia solium infections in a rural block in South India. Trans R Soc Trop Med Hygiene. (2010) 104:601–12. doi: 10.1016/j.trstmh.2010.06.002
19. World Health Organization. Intensifying control of neglected diseases. In: Daurmerie KM, DK, editors. International Workshop on the Intensified Control of Neglected Diseases. Berlin: WHO Communicable Diseases (2003).
20. World Health Organization. The Control of Neglected Zoonotic Diseases: A Route to Poverty Alleviation. Geneva: WHO (2006), p. 65.
21. World Health Organization. Integrated Control of Neglected Diseases in Afric: Applying the “One Health” Concept. Nairobi: WHO, ILRI (2007).
22. World Health Organization. The Control of Neglected Zoonotic Diseases: From Advocacy to Action: Report of the Fourth International Meeting Held at WHO Headquarters, 19-20 November 2014. Geneva: WHO (2015).
23. U. T. C. Diseases NT. London Declaration on Neglected Tropical Diseases. (2012). Available online at: https://unitingtocombatntds.org/london-declaration-neglected-tropical-diseases/.
24. World Health Organization. Accelerating Work to Overcome the Global Impact of Neglected Tropical Diseases: A Roadmap for Implementation: Executive Summary. Geneva: World Health Organization (2012). (accessed October 1, 2018).
25. World Health Assembly. WHA66. 12: Neglected Tropical Diseases. World Health Organization (2013).
26. De Coster T, Van Damme I, Baauw J, Gabriël S. Recent advancements in the control of Taenia solium: a systematic review. Food Waterborne Parasitol. (2018) 13:e00030. doi: 10.1016/j.fawpar.2018.e00030
27. Gemmell M. A critical approach to the concepts of control and eradication of echinococcosis/hydatidosis and taeniasis/cysticercosis. Int J Parasitol. (1987) 17:465–72. doi: 10.1016/0020-7519(87)90122-6
28. Sotelo J, Rosas N, Palencia G. Freezing of infested pork muscle kills cysticerci. JAMA. (1986) 256:893–94. doi: 10.1001/jama.256.7.893
29. Flores-Pérez I, Gonzalez GF, Sciutto E, de Aluja AS. Apoptosis induced by gamma irradiation of Taenia solium metacestodes. Parasitol Res. (2003) 90:203–8. doi: 10.1007/s00436-002-0819-9
30. Rodríguez-Canul R, Argáez-Rodríguez F, Pacheco de la Gala D, Villegas-Perez S, Fraser A, Craig P, et al. Taenia solium metacestode viability in infected pork after preparation with salt pickling or cooking methods common in Yucatan, Mexico. J Food Protect. (2002) 65:666–9. doi: 10.4315/0362-028X-65.4.666
31. Flisser A, Avidan Y, Laiter S, Mintz D, Ongay H. Effect of physical and chemical agents on the viability of the cysticercus of Taenia solium. Salud publica Mexico. (1986) 28:551.
32. Gamble H. Parasites associated with pork and pork products. Rev Sci Tech. (1997) 16:496–506. doi: 10.20506/rst.16.2.1032
33. Bollaerts KE, Messens W, Delhalle L, Aerts M, Van der Stede Y, Dewulf J, et al. Development of a quantitative microbial risk assessment for human salmonellosis through household consumption of fresh minced pork meat in Belgium. Risk Analysis Int J. (2009) 29:820–40. doi: 10.1111/j.1539-6924.2009.01223.x
34. Gonzales-Barron U, Redmond G, Butler F. A consumer-phase exposure assessment of Salmonella Typhimurium from Irish fresh pork sausages: ICooking I, and consumption modules. Food Control. (2010) 21:1693–702. doi: 10.1016/j.foodcont.2010.05.012
35. Carabin H, Millogo A, Ngowi HA, Bauer C, Dermauw V, Koné AC, et al. Effectiveness of a community-based educational programme in reducing the cumulative incidence and prevalence of human Taenia solium cysticercosis in Burkina Faso in 2011–14 (EFECAB): a cluster-randomised controlled trial. Lancet Global Health. (2018) 6:e411–25. doi: 10.1016/S2214-109X(18)30027-5
36. Johansen MV, Trevisan C, GabriËl S, Magnussen P, Braae UC. Are we ready for Taenia solium cysticercosis elimination in sub-Saharan Africa? Parasitology. (2017) 144:59–64. doi: 10.1017/S0031182016000500
37. Braae UC, Gabriël S, Trevisan C, Thomas LF, Magnussen P, Abela-Ridder B, et al. Stepwise approach for the control and eventual elimination of Taenia solium as a public health problem. BMC Infect Dis. (2019) 19:182. doi: 10.1186/s12879-019-3812-y
38. Okello WO, Okello AL, Inthavong P, Tiemann T, Phengsivalouk A, Devleesschauwer B, et al. Improved methods to capture the total societal benefits of zoonotic disease control: demonstrating the cost-effectiveness of an integrated control programme for Taenia solium, soil transmitted helminths and classical swine fever in northern Lao PDR. PLoS Neglect Trop Dis. (2018) 12:e0006782. doi: 10.1371/journal.pntd.0006782
39. Ngowi HA, Mlangwa JED, Carabin H, Mlozi MRS, Kassuku AA, Kimera SI, et al. Financial efficiency of health and pig management education intervention in controlling porcine cysticercosis in Mbulu District, northern Tanzania. Livestock Res Rural Dev. (2007) 19. Available online at: http://www.lrrd.org/lrrd19/5/ngow19062.htm
40. Welburn S, Beange I, Ducrotoy M, Okello A. The neglected zoonoses—the case for integrated control and advocacy. Clin Microb Infect. (2015) 21:433–43. doi: 10.1016/j.cmi.2015.04.011
41. Assana E, Amadou F, Thys E, Lightowlers MW, Zoli AP, Dorny P, et al. Pig-farming systems and porcine cysticercosis in the north of Cameroon. J Helminthol. (2010) 84:441–6. doi: 10.1017/S0022149X10000167
42. Alvarez L, Saumell C, Fusé L, Moreno L, Ceballos L, Domingue G, et al. Efficacy of a single high oxfendazole dose against gastrointestinal nematodes in naturally infected pigs. Vet Parasitol. (2013) 194:70–4. doi: 10.1016/j.vetpar.2013.01.003
43. Poudel I, Sah K, Subedi S, Singh DK, Kushwaha P, Colston A, et al. Implementation of a practical and effective pilot intervention against transmission of Taenia solium. by pigs in the Banke district of Nepal. PLoS Neglect Trop Dis. (2019) 13:e0006838. doi: 10.1371/journal.pntd.0006838
44. Okello AL, Thomas L, Inthavong P, Ash A, Khamlome B, Keokamphet C, et al. Assessing the impact of a joint human-porcine intervention package for Taenia solium. control: results of a pilot study from northern Lao PDR. Acta Tropica. (2016) 159:185–91. doi: 10.1016/j.actatropica.2016.03.012
45. Wabacha JK, Maribei JM, Mulei CM, Kyule MN, Zessin KH, Oluoch-Kosura W. Characterisation of smallholder pig production in Kikuyu Division, central Kenya. Prevent Vet Med. (2004) 63:183–95. doi: 10.1016/j.prevetmed.2004.02.007
46. Dorny P, Phiri IK, Vercruysse J, Gabriel S, Willingham Iii AL, Brandt J, et al. A Bayesian approach for estimating values for prevalence and diagnostic test characteristics of porcine cysticercosis. Int J Parasitol. (2004) 34:569–76. doi: 10.1016/j.ijpara.2003.11.014
47. Levy M, Dewey C, Poljak Z, Weersink A, Mutua F. Comparing the operations and challenges of pig butchers in rural and peri-urban settings of western Kenya. Afr J Agric Res. (2014) 9:125–36. doi: 10.5897/AJAR12.2174
48. Jaffee S, Henson S, Unnevehr L, Grace D, Cassou E. The Safe Food Imperative. Washington, DC: World Bank (2018).
49. Thomas LF, de Glanville WA, Cook EA, Barend MDC, Handel I, Wamae CN, et al. Modelling the risk of Taenia solium exposure from pork produced in western Kenya. PLoS Neglect Trop Dis. (2017) 11:e0005371. doi: 10.1371/journal.pntd.0005371
50. Fèvre EM, Glanville WA, Thomas LF, Cook EA, Kariuki S, Wamae CN. An integrated study of human and animal infectious disease in the Lake Victoria crescent small-holder crop-livestock production system, Kenya. BMC Infect Dis. (2017) 17:457. doi: 10.1186/s12879-017-2559-6
51. Thomas LF, Harrison LJS, Toye P, de Glanville WA, Cook EAJ, Wamae CN, et al. Prevalence of Taenia solium. cysticercosis in pigs entering the food chain in western Kenya. Trop Animal Health Prod. (2015) 48:1–6. doi: 10.1007/s11250-015-0949-6
52. Thomas LF. Epidemiology of Taenia solium Cysticercosis in Western Kenya School of Biological Sciences. University of Edinburgh (2014).
53. Carter NA, Dewey CE, Thomas LF, Lukuyu B, Grace D, de Lange C. Nutrient requirements and low-cost balanced diets, based on seasonally available local feedstuffs, for local pigs on smallholder farms in Western Kenya. Trop Anim Health Prod. (2016) 48:337–47. doi: 10.1007/s11250-015-0957-6
54. Kagira JM, Kanyari PN, Maingi N, Githigia SM, Ng'ang'a JC, Karuga JW. Characteristics of the smallholder free-range pig production system in western Kenya. Trop Anim Health Prod. (2010) 42:865–73. doi: 10.1007/s11250-009-9500-y
55. Tiemann TT, Phengvilaysouk A, Keonouchanh S. Herd dynamics reflect constraints for pig production and farmer attitudes in smallholder systems in Lao PDR. Animal Prod Sci. (2017) 58:2158–166. doi: 10.1071/AN16730
56. Braae UC, Penrith M-L, Ngowi H, Lekule F, Johansen MV. Awareness concerning optimal pig production management and animal welfare among smallholder farmers in Tanzania. Anim Welfare. (2016) 25:439–46. doi: 10.7120/09627286.25.4.439
57. Phengsavanh P, Ogle B, Stür W, Frankow-Lindberg B, Lindberg J. Smallholder pig rearing systems in Northern Lao PDR. Asian Australasian J Anim Sci. (2011) 24:867–74. doi: 10.5713/ajas.2011.10289
58. Tekle T, Tesfay A, Kifleyohannes T. Smallholder pig production and its constraints in Mekelle and southern zone of Tigray region, north Ethiopia. Livestock Res Rural Dev. (2013) 25:1–5. Available online at: http://www.lrrd.cipav.org.co/lrrd25/10/tekl25184.htm
59. Chauhan A, Patel B, Maurya R, Kumar S, Shukla S, Kumar S. Pig production system as a source of livelihood in Indian scenario: an overview. Int J Sci Environ Technol. (2016) 5:2089–96. Available online at: http://www.ijset.net/index_issue.php?iid=45.
60. Kimbi E, Lekule F, Mlangwa J, Mejer H, Thamsborg S. Smallholder pigs production systems in Tanzania. J Agric Sci Technol. (2015) 5:47–60. doi: 10.17265/2161-6256/2015.01A.007
61. Assana E, Kyngdon CT, Gauci CG, Geerts S, Dorny P, De Deken R, et al. Elimination of Taenia solium transmission to pigs in a field trial of the TSOL18 vaccine in Cameroon. Int J Parasitol. (2010) 40:515–9. doi: 10.1016/j.ijpara.2010.01.006
62. Hobbs EC, Mwape KE, Devleesschauwer B, Gabriël S, Chembensofu M, Mambwe M, et al. Taenia solium from a community perspective: preliminary costing data in the Katete and Sinda districts in Eastern Zambia. Veter Parasitol. (2018) 251:63–7. doi: 10.1016/j.vetpar.2018.01.001
63. Levy M, Dewey C, Weersink A, Mutua F. Comparative profitability of pig butcher businesses in western Kenya. In: International Symposia on Veterinary Epidemiology and Economics (ISVEE) proceedings, ISVEE 12: Proceedings of the 12th Symposium of the International Society for Veterinary Epidemiology and Economics, Durban, (2009).
64. Mutua F, Dewey C, Arimi S, Ogara W, Levy M, Schelling E. A description of local pig feeding systems in village smallholder farms of Western Kenya. Trop Anim Health Product. (2012) 44:1157–62. doi: 10.1007/s11250-011-0052-6
65. Detsky AS, Naglie IG. A clinician's guide to cost-effectiveness analysis. Ann Intern Med. (1990) 113:147–54. doi: 10.7326/0003-4819-113-2-147
66. Antle JM. Benefits and costs of food safety regulation. Food Policy. (1999) 24:605–23. doi: 10.1016/S0306-9192(99)00068-8
67. Trevisan C, Devleesschauwer B, Schmidt V, Winkler AS, Harrison W, Johansen MV. The societal cost of Taenia solium cysticercosis in Tanzania. Acta Trop. (2017) 165:141–54. doi: 10.1016/j.actatropica.2015.12.021
68. Andreoni J, Harbaugh W, Vesterlund L. The carrot or the stick: rewards, punishments, and cooperation. Am Econ Rev. (2003) 93:893–902. doi: 10.1257/000282803322157142
69. Nissen S, Poulsen IH, Nejsum P, Olsen A, Roepstorff A, Rubaire-Akiiki C, et al. Prevalence of gastrointestinal nematodes in growing pigs in Kabale District in Uganda. Trop Anim Health Prod. (2011) 43:567–72. doi: 10.1007/s11250-010-9732-x
70. Kagira JM, Paul NK, Samuel MG, Ndicho M, Ng'ang'a JC, Gachohi JM. Risk factors associated with occurrence of nematodes in free range pigs in Busia District, Kenya. Trop Anim Health Prod. (2012) 44:657–64. doi: 10.1007/s11250-011-9951-9
71. Purvis H, Whittier J, Boyles S, Johnson L, Ritchie H, Rust S, et al. Weight gain and reproductive performance of spring-born beef heifer calves intraruminally administered oxfendazole. J Anim Sci. (1994) 72:817–23. doi: 10.2527/1994.724817x
72. Lai J, Wang HH, Ortega DL, Widmar NJO. Factoring Chinese consumers' risk perceptions into their willingness to pay for pork safety, environmental stewardship, and animal welfare. Food Control. (2018) 85:423–31. doi: 10.1016/j.foodcont.2017.09.032
73. Wu Y, Li E, Samuel SN. Food consumption in urban China: an empirical analysis. Appl Econ. (1995) 27:509–15. doi: 10.1080/00036849500000138
74. Robinson T, Pozzi F. Mapping supply and demand for animal-source foods to 2030. Anim Prod Health Working Paper. (2011) 2:1–154.
75. Shibia M, Rahman S, Chidmi B. Consumer demand for meat in Kenya: an examination of the linear approximate almost ideal demand system. Selected Paper Prepared for Presentation at the 2017 Southern Agricultural Economics Association (SAEA) Annual Meetings in Mobile. Mobile (2017).
76. Peters D. Assessment of the Potential of Sweet Potato as Livestock Feed in East Africa: Rwanda, Uganda, and Kenya. The International Potato Center (CIP) in Nairobi (2008).
77. Kikafunda J, Tumwine J. Diet and socio-economic factors and their association with the nutritional status of pre-school children in a low income suburb of Kampala City, Uganda. East Afr Med J. (2006) 83:565–74. doi: 10.4314/eamj.v83i10.9470
78. Ngigi M, Okello J, Lagerkvist C, Karanja N, Mburu J. Urban consumers' willingness to pay for quality of leafy vegetables along the value chain: the case of Nairobi kale consumers, Kenya. Int J Bus Soc Sci. (2011) 2:208–16. Available online at: http://ijbssnet.com/journal/index/313.
79. Garcia HH, Gonzalez AE, Tsang VC, O'Neal SE, Llanos-Zavalaga F, Gonzalvez G, et al. Elimination of Taenia solium transmission in Northern Peru. New Engl J Med. (2016) 374:2335–44. doi: 10.1056/NEJMoa1515520
80. Edwards DS, Johnston AM, Mead GC. Meat inspection: an overview of present practices andfuture trends. Veter J. (1997) 154:135–47. doi: 10.1016/S1090-0233(97)80051-2
Keywords: Taenia solium, cysticercosis, control, interventions, economics, incentives
Citation: Thomas LF, Cook EAJ, Fèvre EM and Rushton J (2019) Control of Taenia solium; A Case for Public and Private Sector Investment. Front. Vet. Sci. 6:176. doi: 10.3389/fvets.2019.00176
Received: 28 December 2018; Accepted: 20 May 2019;
Published: 20 June 2019.
Edited by:
Flavie Vial, Animal and Plant Health Agency, United KingdomReviewed by:
Anna Sophie Fahrion, Safoso, SwitzerlandIsaac Khozozo Phiri, University of Zambia, Zambia
Copyright © 2019 Thomas, Cook, Fèvre and Rushton. This is an open-access article distributed under the terms of the Creative Commons Attribution License (CC BY). The use, distribution or reproduction in other forums is permitted, provided the original author(s) and the copyright owner(s) are credited and that the original publication in this journal is cited, in accordance with accepted academic practice. No use, distribution or reproduction is permitted which does not comply with these terms.
*Correspondence: Lian F. Thomas, bGlhbi50aG9tYXNAbGl2ZXJwb29sLmFjLnVr