- 1Laboratory for Equine and Comparative Orthopedic Research, Department of Veterinary Clinical Sciences, School of Veterinary Medicine, Louisiana State University, Baton Rouge, LA, United States
- 2Department of Veterinary Clinical Sciences, School of Veterinary Medicine, Louisiana State University, Baton Rouge, LA, United States
- 3Gilad & Gilad LLC, Henderson, NV, United States
- 4P.A.W.S. (Partners in Animal Wellness Services) Veterinary Center, Tucson, AZ, United States
Background: Pain from coxofemoral joint (CFJ) osteoarthritis (OA) characteristic of canine hip dysplasia (CHD) afflicts many dogs. Intervertebral disc (IVD) degeneration is a common CFJ OA comorbidity. Non-steroidal anti-inflammatory drug (NSAID) administration is standard for treatment of pain from degenerative joint disease. Potential side effects and tolerance from prolonged administration drive efforts to identify compounds that may be alternatives to or combined with NSAIDs. Agmatine, decarboxylated arginine, reportedly alleviates neuropathic pain, a likely component of OA pain. The objective of this study was to compare treatment response to agmatine and carprofen in dogs with varying degrees of CFJ OA with or without IVD degeneration and to test the hypothesis that agmatine improves hindlimb use comparably to carprofen and more than placebo.
Methods: Nine hound-type dogs received oral carprofen (4.4 mg/kg, sid) for 7 days. Six months later, oral agmatine sulfate (25 mg/kg, bid) or placebo (hydroxypropyl methylcellulose, bid) was administered to the same dogs for 28 days with a 2 week washout period between treatments. Validated pain assessment scores were measured before treatment and every seven days throughout the treatment periods. Serum chemistry levels and ground reaction forces (GRF) were quantified before and after each treatment period. A board-certified radiologist quantified radiographic CFJ OA based on Orthopedic Foundation for Animals criteria and IVD degeneration on magnetic resonance images. GRFs were compared among treatments at each time point and among time points for each treatment.
Results: There were no detectable adverse effects with any treatment. Significant results included improved GRFs in dogs with mild CFJ OA (N = 3) following agmatine administration compared to carprofen or placebo and a trend for improved GRFs in dogs with moderate CFJ OA (N = 2) following carprofen vs. agmatine or placebo. Neither agmatine nor carprofen improved GRFs in dogs with severe CFJ OA (N = 4). The GRFs improved in dogs with IVD degeneration (N = 3) following carprofen treatment compared to agmatine or placebo regardless of CFJ OA score, but no effect was observed in dogs with normal lumbar spines (N = 6).
Conclusions: Results support agmatine over carprofen treatment to improve limb use in dogs with early or mild CFJ OA, while carprofen may be the better choice for dogs with moderate CFJ OA or IVD degeneration regardless of CFJ OA severity.
Introduction
Osteoarthritis (OA) affects up to 12 million dogs in the U.S., the majority of which are older than 8 years (1, 2). Canine hip dysplasia (CHD) is among the most common degenerative orthopedic conditions in canine companions and joint changes are biphasic, starting with coxofemoral joint (CFJ) capsule, periarticular and articular damage, and followed by joint degeneration and OA (1, 3–6). Dogs with CFJ laxity associated with CHD are reported to have a higher risk of OA in other joints including vertebral (7–9). Intervertebral disc (IVD) degeneration is a frequent comorbidity or component of CHD pathology (8). Similar to CFJ changes, IVD degeneration progresses with advancing age with many dogs over 7 years old affected by nucleus pulposus degeneration and/or IVD protrusion (10, 11). Pain from IVD degeneration can be intrinsic to the disc or extrinsic radicular or facet joint pain (12, 13).
Customized pain management with non-steroidal anti-inflammatory drugs (NSAIDs) is a standard part of conservative or post-surgical treatment regimens for dogs with CHD associated joint changes and/or IVD degeneration (14, 15). Although life-threatening adverse effects are rare, a recent assessment of causality showed that NSAIDs have the potential to cause or exacerbate digestive tract, renal, and hepatobiliary disorders (16). Of NSAIDs approved for use in dogs, carprofen is one of the mainstays. In a recent study, signs of pain were lower in 74% of canine OA patients after 120 days of carprofen while 5% had adverse gastrointestinal effects associated with the drug (17). With a 100-fold higher selectivity for cyclooxygenase 2 vs. 1, adverse effects are less prevalent than with other less specific NSAIDs (18).
Contributions to joint pain are known to be both neurogenic and musculoskeletal in origin (19). Current knowledge supports that contributions of each wax and wane throughout the course of the disease, though neuropathic pain may predominate during the early stages based on information from human OA (20). Pain from OA is multifaceted and thought to be exacerbated by central sensitization that facilitates nociceptive transmission and increased gain in the spinal cord (21). In human patients, mechanical joint hyperesthesia may be a consequence of altered pain modulation at the spinal or supraspinal level (19). The phenomenon was recently confirmed in dogs undergoing total hip replacement for painful CFJ disease (21). Additionally, it is proposed that neuropathic pain is the reason some human patients with minor CFJ changes do not respond to conventional analgesic treatment (20). Comprehensive therapy to specifically target distinct sources of pain will improve the mobility of dogs with CFJ OA with or without IVD degeneration.
Agmatine is a ubiquitous compound formed during the natural process of arginine decarboxylation (22, 23). The compound is proposed to have many roles in physiologic homeostasis including neuromodulation, neuroprotection, mitochondrial function, and antioxidant and antineoplastic effects (24–26). Agmatine administration alleviates thermal allodynia and hyperalgesia in rat models of both constrictive nerve injury and streptozocin (STZ) induced diabetes (27, 28), and the effects on neuropathic pain are thought to be dose-dependent (28, 29). Proposed mechanisms of action include stimulation of α2-adrenergic and imidazoline receptors combined with inhibition of N-methyl-D-aspartate receptors and all isoforms of nitric oxide synthase (30–33). Given the potential for alleviation of neuropathic joint pain, agmatine may be a useful adjunct to current mechanisms for improving hind limb use in dogs with CFJ OA with or without IVD degeneration.
Based on the current understanding of the complexity of CFJ pain from OA and the potential for pain emanating from IVD degeneration, we hypothesized that agmatine improves hind limb use comparably to carprofen and more than placebo. To test this hypothesis, a prospective, randomized, crossover study was performed to quantify changes in validated subjective pain scores, serum chemistry values and hind limb ground reaction forces (GRFs) following oral administration of carprofen, agmatine, and placebo to dogs with varying degrees of CFJ OA with or without IVD degeneration.
Materials and Methods
Animals
Nine adult, purpose-bred, hound-type dogs from a teaching and research colony owned by Louisiana State University were included in the study (34, 35). Dogs were housed in temperature-regulated, 1.2 × 2.4 m runs, with daily 1 h free play sessions for the duration of the study. They had free-choice kibble (Laboratory Canine Diet 5006, LabDiet, St. Louis, USA) and ad libitum water. The study was performed in accordance with Institutional and National Institutes of Health regulations governing the treatment of vertebrate animals and initiated after approval by the Institutional Animal Care and Use Committee.
Study Design
The study was a prospective, randomized, placebo-controlled, crossover design. All dogs were treated with carprofen (4.4 mg/kg, PO, sid; quellin®, BAYER, Leverkusen, Germany) for 7 days. Approximately 6 months later, the same dogs were randomly assigned using a randomized block design to receive agmatine sulfate (25 mg/kg, PO, bid; G-Agmatine®, Gilad & Gilad LLC, Henderson, USA) or hydroxypropyl methylcellulose (PO, bid) for 28 days with a 14 day washout period between treatments. All medication containers were coded, and investigators were unaware of treatment identities until all data collection, reduction and analysis was complete. Prior to and at the end of each treatment phase, blood was drawn by venipuncture to measure plasma levels of aspartate aminotransferase (AST), alanine aminotransferase (ALT), alkaline phosphatase (ALP), blood urea nitrogen (BUN), and creatinine (CREAT), and ground reaction forces (GRFs) were quantified. Radiographs were performed prior to study initiation and magnetic resonance imaging (MRI) after the study conclusion. As part of the monitoring regimen for adverse drug reactions, all dogs were assessed prior to and after every 7 days of treatment using the Colorado State University Canine Acute Pain Scale during carprofen administration and with the Canine Brief Pain Inventory and the Glasgow Composite Pain Scale Short Form during agmatine and placebo administration (36–38). The same investigators performed all assessments, and they were not aware of specific treatment administration at any point during the study.
Radiographs
Ventrodorsal extended hip pelvic radiographs were performed with the dogs under general anesthesia. All dogs were fasted for at least 8 h prior to anesthesia. They were premedicated with butorphanol (0.3 mg/kg, IM) and dexmedetomidine (3 μg/kg, IM). Propofol (3.5 mg/kg, IV) was administered for induction and tracheal intubation. Anesthesia was maintained with isoflurane in oxygen in a semi-closed circle system.
Radiographic changes were quantified by a board-certified radiologist based on Orthopedic Foundation for Animals (OFA) criteria. Numeric values were assigned to each CFJ as follows: 0 (none) = no acetabular dysplasia, no OA; 1 (mild) = acetabular dysplasia, no OA; 2 (moderate) = acetabular dysplasia, OA; or 3 (severe) = complete femoral head luxation, marked OA (39). Both joints in each dog were assigned a value and the highest value of the two was used as the OA severity score. This was to ensure accurate representation of OA severity for the duration of the study.
Magnetic Resonance Imaging
Magnetic resonance imaging scans were performed on all dogs at the end of the study. Imaging was performed with the dogs positioned in dorsal recumbency under general anesthesia identically to radiographic imaging. Sagittal and transverse T2-W, sagittal fast inversion recovery (FIR), transverse proton density (PD), and dorsal T1-W sequences (Hitachi Echelon 1.5 T, Twinsburg, OH, USA) were performed. Images were evaluated by the same board-certified radiologist as above for presence or absence of disc dehydration, disc bulging, and spinal cord compression in the lumbar spine. The presence of any sign was considered confirmation of IVD degeneration.
Gait Kinetics
Kinetic gait analysis was performed prior to and after each treatment regimen. The process was similar to that described previously (40). Briefly, a 900 × 900 mm force platform (Model # OR6-WP-1000, Advanced Mechanical Technology, Inc., Watertown, USA) embedded in the center of a runway was used for all trials. A series of five retroreflective photocell sensors (Mek92–PAD, Joslyn Clark Controls, Inc., Lancaster, USA) were used to calculate trial velocity and acceleration. The force platform surface has the same color and texture as the runway. Dogs were conditioned to the force platform and experienced handlers trotted them for all trials. A trial was considered successful if a fore foot contacted the force platform followed by contact of the ipsilateral hind foot at a velocity of 1.70–2.40 m/s and acceleration of 0.9 to −0.9 m/s2, a comfortable trotting pace for the study subjects. Three trials that varied <5% in velocity were selected for each dog at each time point. All trials were recorded at a rate of 1,000 Hz and processed with commercially available software (Acquire v7.3, Sharon Software Inc., Dewitt, USA). Measured forces included y (craniocaudal, braking and propulsion) and z (vertical) peak force and impulse. The mean of the GRF values for both right and left limbs was used as a single value for individual dogs (34). Percent change in each GRF measure was calculated as [(GRF after treatment–GRF at baseline)/(GRF at baseline)] × 100.
Statistical Analysis
Multivariate analysis of variance models was used to compare fixed effects of treatment with random effects of dog and time. Significance level was set at p < 0.05. Data was presented as mean ± SEM.
Results
Animals
Three males and 6 females, 3.7 ± 0.6 years of age (range 2–6 years) with a body weight of 26.6 ± 0.9 kg (range 23–30.4 kg), were included in the study. All dogs had evidence of CFJ pathology (Table 1). Three dogs had abnormal lumbar spine MRI findings. Serum chemistry results were within normal limits and there was no impact on subjective pain evaluations with any treatment. Subjectively, none of the dogs favored any limbs or showed detectable signs of distress or acute pain over the course of the study.
Gait Kinetics
The primary outcome measures in the investigation were from kinetic gait analysis, the established gold standard for quantification of limb pain (41). Baseline kinetic values were not significantly different between treatments for any of the dogs. Significant findings included higher increases in vertical and braking peak force following treatment with agmatine vs. carprofen or placebo and a greater increase in vertical peak force following treatment with carprofen vs. placebo in dogs with an OA score of 1 (Figure 1, Tables 2, 3). Although change in vertical peak force was greater following treatment with carprofen vs. agmatine or placebo in dogs with an OA score of 2, it was not statistically significant. Notably, vertical peak force increased following carprofen treatment in 1 dog, whereas it did not increase in the other (Supplementary Figure 1). There was little change in GRFs in dogs with an OA score of 3 with any of the treatments; changes were <5% with an exception of a higher change in propulsion impulse after placebo vs. carprofen treatment. In comparisons among dogs with IVD degeneration when considering all OA scores together, vertical peak force increased more after carprofen vs. agmatine or placebo treatment (Figure 2). Additionally, peak braking force increase was greater after carprofen vs. placebo treatment. There were no differences in GRF percent changes among treatments in dogs with no detectable IVD degeneration.
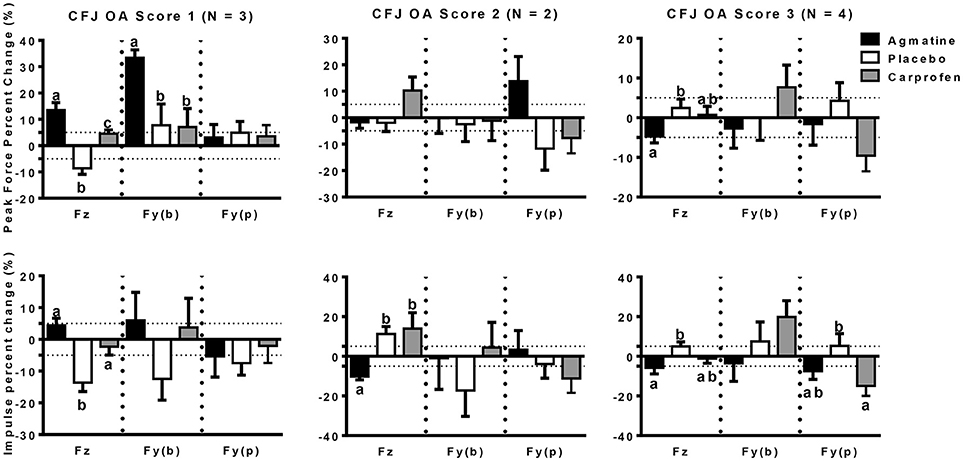
Figure 1. Peak force (PF: upper panels) and impulse (Imp: lower panels) percent change (mean +/- SEM) in dogs with different radiographic coxofemoral joint osteoarthritis scores (CFJ OA score 1: left panels; score 2: central panels; score 3: right panels) following treatment with agmatine (black bars), placebo (white bars), or carprofen (gray bars). In each panel, Fz represents vertical force (left 3 bars), Fy(b) represents braking force (central 3 bars), and Fy(p) represents propulsion force (right 3 bars). Columns with different letters are significantly different among treatment groups for the indicated ground reaction force (p < 0.05). The dashed horizontal lines demarcate 5% positive (upper) or negative (lower) changes in each ground reaction force.
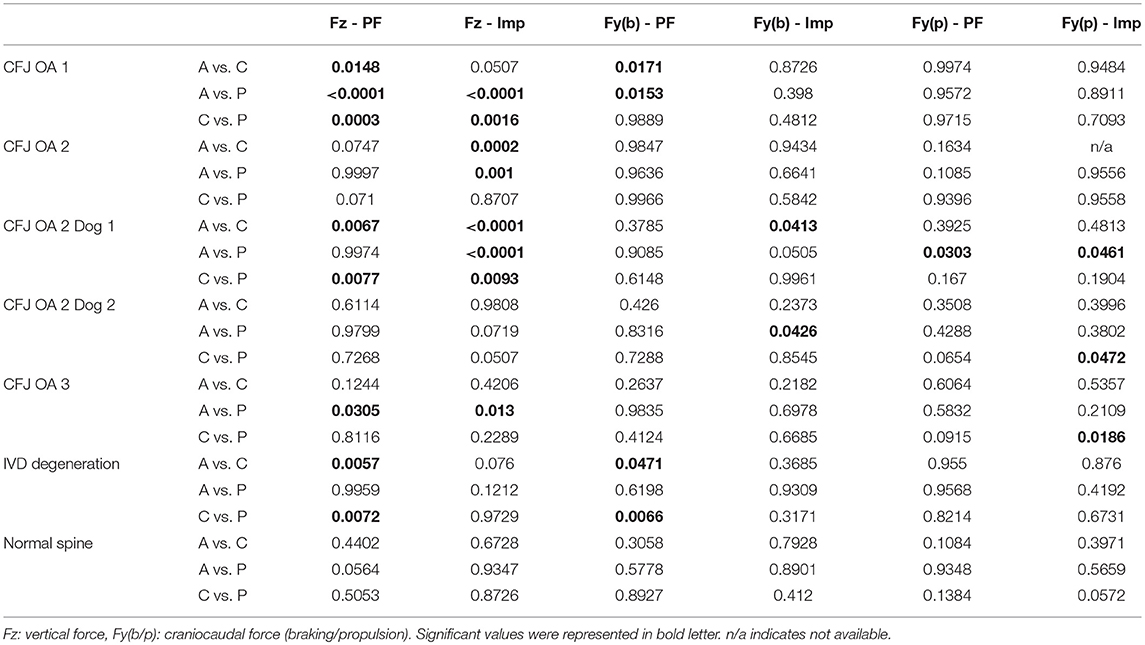
Table 3. P-values of multiple comparison for GRF in peak force (PF) and impulse (Imp) between Agmatine (A), placebo (P), and Carprofen (C) treatment groups based on different grades of radiographic CFJ OA (1–3) and presence or absence of IVD degeneration (IVD Degeneration or Normal Spine).
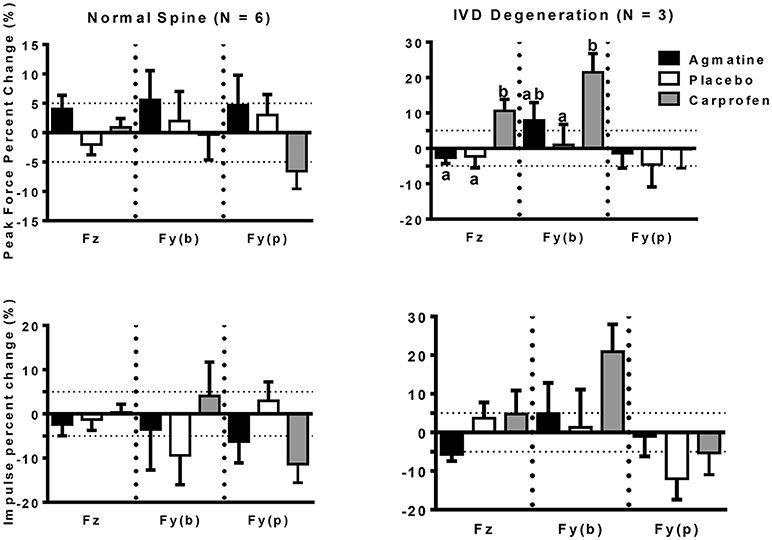
Figure 2. Peak force (PF: upper panels) and impulse (Imp: lower panels) percent change (mean +/– SEM) in dogs with normal spine (left panels) or intervertebral disc degeneration (IVD degeneration: right panels) following treatment with agmatine (black bars), placebo (white bars), or carprofen (gray bars). In each panel, Fz represents vertical force (left 3 bars), Fy(b) represents braking force (central 3 bars), and Fy(p) represents propulsion force (right 3 bars). Columns with different letters are significantly different among treatment groups for the indicated ground reaction force (p < 0.05). The dashed horizontal lines demarcate 5% positive (upper) or negative (lower) changes in each ground reaction force.
Discussion
The results of this study suggest that agmatine may improve hind limb use in dogs with early CFJ OA while carprofen may be more effective in dogs with moderate joint degeneration or IVD degeneration regardless of CFJ OA. Based on these results, the hypothesis is rejected, and it appears that agmatine may be warranted for dogs with CFJ pain from early joint changes, but carprofen is the therapeutic choice for those with more advanced disease with or without concurrent IVD pathology.
The goal of this study was to evaluate gait after a meaningful period of therapeutic drug levels. Based on existing information, carprofen reaches maximum plasma levels in dogs after 1 h (42). As indicated previously, investigators were not aware of the identity of the medications tested in the study prior to completion of data collection, reduction, and analysis. This is in spite of the fact that carprofen was administered for a shorter period of time given concerns about potential for development of gastrointestinal problems in the specific dog population included in the study. Signs of gastrointestinal adverse effects can occur within 2–4 weeks of carprofen administration (43), and the American Animal Hospital Association recommends inclusion of a minimum 7 day washout period between NSAIDs if continual treatment is required (44). The pharmokinetics of agmatine in dogs have yet to be established, so the duration and dose were derived from existing information in other species (23, 45), some of which include direct intrathecal or intraperitoneal administration (27, 28). Neuropathic pain without OA in humans was alleviated following 14 days of agmatine (23). The adminstration period in this study was doubled to help ensure sufficient time with therapeutic agmatine levels for chronic musculoskeletal pain. Based on study findings, agmatine did not have any adverse effects over the duration of the study which appeared to be sufficient to reduce pain from mild CFJ OA.
The fact that the time periods of drug administration were not identical is a limitation of the study. It is possible that gait parameters in dogs with mild CFJ OA might have improved with longer carprofen treatment based on the tendency (p = 0.11) for increased peak vertical force measured in dogs with coxofemoral and elbow joint OA after 28 days of half the dose (2 mg/kg) used in this study (46). Additionally, oral administration of carprofen reportedly improved clinical signs in dogs with OA in various joints after 4 months of administration (17). Clear improvement in one dog with moderate OA and IVD degeneration following carprofen treatment while another with moderate OA and no IVD degeneration did not respond suggests that the duration of carprofen administration was sufficient for pain relief. However, the small sample size and lack of serum agmatine levels precludes any conclusions on this point.
Quantification of GRFs is an established mechanism to assess therapeutic efficacy for limb and back pain in dogs and other species (34, 47, 48). Careful quality control is necessary to ensure meaningful results and this was implicit in study standards that required variation of 5% or less among trials. The crossover nature of the study provided a direct comparison of treatments for each dog. The MRI assessments performed after the last study phase ensured that the IVD degeneration scores reflected the current condition of the lumbar spine. While it is possible that OA and/or IVD progressed slightly over the study period, gait kinetic values, the established gold standard for evaluation of joint pain, were the primary outcome measures in this study (41). The fact that baseline kinetic parameters did not change significantly between treatments makes it unlikely that CFJ OA or IVD degeneration changed appreciably. The small number of dogs with evidence of IVD degeneration or moderate OA allows only a presumption of superior carprofen pain control for the conditions. Additional studies with a much larger population containing various combinations of spine and CFJ pathology are necessary to confirm the benefits of carprofen over agamatine for IVD degeneration and moderate CFJ OA.
Agmatine may provide a mechanism to reduce CFJ pain during initial disease stages and carprofen for later stages, or combined therapy may be more effective than either alone. A multi-modal approach to pain management offers a wider range of options to maintain canine activity levels as needs change (49, 50). Additional work to determine the effects of combined therapies may show synergistic effects based on existing work that supports administration of agmatine with other compounds that inhibit N-methyl-D-aspartate receptors or nitric oxide synthase (45). Combining agmatine with gabapentin, reported to be beneficial for canine neuropathic pain, may be another option (51, 52).
Conclusions
This pilot study establishes the potential benefits of agmatine treatment for early stage canine CFJ OA. Agmatine administration may conceivably improve limb use and associated quality of life. Further investigation is required to confirm the promising findings of this early pilot study in a heterogeneous canine population. Future work may show benefits of treatment regimens that combine agmatine with established pain management, including NSAIDs. This study establishes one potential dosage, treatment route and interval for effective pain relief in dogs with early stage CFJ OA. The information is a valid starting point to test the compound for management of discomfort for distinct conditions in diverse canine companions.
Data Availability Statement
The datasets supporting the conclusions of this article are included within the article and its additional files. All datasets used and/or analyzed during the current study are available from the corresponding author on reasonable request.
Author Contributions
TT, RK, and ML contributed to the study design, data collection, data reduction, analysis and interpretation, and manuscript preparation. CT and NR contributed to data collection and interpretation and manuscript preparation. GG and RA contributed to manuscript editting. All authors read and approved the final version of the manuscript.
Funding
Funding for this study was provided by the American Holistic Veterinary Medical Foundation (Grant #LSU166206159).
Conflict of Interest Statement
GG is co-owner and CEO of Gilad & Gilad, LLC the company that provided the agmatine treatment used in this study (G-Agmatine R, US Patent No. 8916612).
The remaining authors declare that the research was conducted in the absence of any commercial or financial relationships that could be construed as a potential conflict of interest.
Acknowledgments
The authors thank Evan Boatwright, Robert Walton, Pengju Wang, and Qingqiu Yang for assistance with ground reaction force data collection. They also thank Chin-Chi Li for assistance with statistical analysis.
Supplementary Material
The Supplementary Material for this article can be found online at: https://www.frontiersin.org/articles/10.3389/fvets.2018.00311/full#supplementary-material
Supplementary Figure 1. Peak force (PF: upper panels) and impulse (Imp: lower panels) percent change (mean +/- SEM) in 2 dogs (dog 1 and 2) with coxofemoral joint osteoarthritis scores 2 (CFJ OA score 2) following treatment with agmatine (black bars), placebo (white bars), or carprofen (gray bars). In each panel, Fz represents vertical force (left 3 bars), Fy(b) represents braking force (central 3 bars), and Fy(p) represents propulsion force (right 3 bars). Columns with different letters are significantly different among treatment groups for the indicated ground reaction force (p < 0.05). The dashed horizontal lines demarcate 5% positive (upper) or negative (lower) changes in each ground reaction force.
Abbreviations
CFJ, coxofemoral joint; OA, osteoarthritis; CHD, canine hip dysplasia; IVD, intervertebral disc; NSAID, non-steroidal anti-inflammatory drug; GRF, ground reaction force; AST, aspartate aminotransferase; ALT, alanine aminotransferase; ALP, alkaline phosphatase; BUN, blood urea nitrogen; CREAT, creatinine; MRI, magnetic resonance imaging; OFA, Orthopedic Foundation for Animals; FIR, fast inversion recovery; PD, proton density.
References
1. Tobias KM. Veterinary Surgery: Small Animal: 2-Volume Set St. Louis, MO: Elsevier Health Sciences (2013).
2. Black LL, Gaynor J, Adams C, Dhupa S, Sams AE, Taylor R, et al. Effect of intraarticular injection of autologous adipose-derived mesenchymal stem and regenerative cells on clinical signs of chronic osteoarthritis of the elbow joint in dogs. Vet Ther. (2008) 9:192–200.
3. King MD. Etiopathogenesis of canine hip dysplasia, prevalence, and genetics. Vet Clin N Am Small Anim Pract. (2017) 47:753–67. doi: 10.1016/j.cvsm.2017.03.001
4. Fries CL, Remedios AM. The pathogenesis and diagnosis of canine hip dysplasia: a review. Can Vet J. (1995) 36:494–502.
5. Alexander JW. The pathogenesis of canine hip dysplasia. Vet Clin N Am Small Anim Pract. (1992) 22:503–11. doi: 10.1016/S0195-5616(92)50051-1
6. Gold RM, Gregor TP, Huck JL, McKelvie PJ, Smith GK. Effects of osteoarthritis on radiographic measures of laxity and congruence in hip joints of labrador retrievers. J Am Vet Med Assoc. (2009) 234:1549–54. doi: 10.2460/javma.234.12.1549
7. Runge JJ, Kelly SP, Gregor TP, Kotwal S, Smith GK. Distraction index as a risk factor for osteoarthritis associated with hip dysplasia in four large dog breeds. J Small Anim Pract. (2010) 51:264–9. doi: 10.1111/j.1748-5827.2010.00937.x
8. Olsewski JM, Lust G, Rendano VT, Summers BA. Degenerative joint disease: multiple joint involvement in young and mature dogs. Am J Vet Res. (1983) 44:1300–8.
9. Madsen JS. The joint capsule and joint laxity in dogs with hip dysplasia. J Am Vet Med Assoc. (1997) 210:1463–5.
10. Hansen HJ. A pathologic-anatomical interpretation of disc degeneration in dogs. Acta Orthop Scand. (1951) 20:280–93. doi: 10.3109/17453675108991175
11. Hansen HJ. Comparative views of the pathology of disk degeneration in animals. Lab Invest. (1959) 8:1242–65.
12. Forsythe WB, Ghoshal NG. Innervation of the canine thoracolumbar vertebral column. Anat Rec. (1984) 208:57–63. doi: 10.1002/ar.1092080107
13. Rea W, Kapur S, Mutagi H. Intervertebral disc as a source of pain. Continuing Education in Anaesthes Crit Care Pain (2012) 12:279–82. doi: 10.1093/bjaceaccp/mks028
14. Brisson BA. Intervertebral disc disease in dogs. Vet Clin N Am Small Anim Pract. (2010) 40:829–58. doi: 10.1016/j.cvsm.2010.06.001
15. Moreau M, Dupuis J, Bonneau NH, Desnoyers M. Clinical evaluation of a nutraceutical, carprofen and meloxicam for the treatment of dogs with osteoarthritis. Vet Record (2003) 152:323–9. doi: 10.1136/vr.152.11.323
16. Payne-Johnson M, Becskei C, Chaudhry Y, Stegemann MR. Comparative efficacy and safety of mavacoxib and carprofen in the treatment of canine osteoarthritis. Vet Rec. (2015) 176:284. doi: 10.1136/vr.102397
17. Autefage A, Gossellin J. Efficacy and safety of the long-term oral administration of carprofen in the treatment of osteoarthritis in dogs. Revue Méd Vét. (2007) 1:119–27.
18. Ricketts AP, Lundy KM, Seibel SB. Evaluation of selective inhibition of canine cyclooxygenase 1 and 2 by carprofen and other nonsteroidal anti-inflammatory drugs. Am J Vet Res. (1998) 59:1441–6.
19. Gwilym SE, Keltner JR, Warnaby CE, Carr AJ, Chizh B, Chessell I, et al. Psychophysical and functional imaging evidence supporting the presence of central sensitization in a cohort of osteoarthritis patients. Arthritis Rheumat. (2009) 61:1226–34. doi: 10.1002/art.24837
20. Dimitroulas T, Duarte RV, Behura A, Kitas GD, Raphael JH. Neuropathic pain in osteoarthritis: a review of pathophysiological mechanisms and implications for treatment. Semin Arthritis Rheumat. (2014) 44:145–54. doi: 10.1016/j.semarthrit.2014.05.011
21. Tomas A, Marcellin-Little DJ, Roe SC, Motsinger-Reif A, Lascelles BD. Relationship between mechanical thresholds and limb use in dogs with coxofemoral joint oa-associated pain and the modulating effects of pain alleviation from total hip replacement on mechanical thresholds. Vet Surgery (2014) 43:542–8. doi: 10.1111/j.1532-950X.2014.12160.x
22. Wu G, Bazer FW, Davis TA, Kim SW, Li P, Marc Rhoads J, et al. Arginine metabolism and nutrition in growth, health and disease. Amino Acids (2009) 37:153–68. doi: 10.1007/s00726-008-0210-y
23. Keynan O, Mirovsky Y, Dekel S, Gilad VH, Gilad GM. Safety and efficacy of dietary agmatine sulfate in lumbar disc-associated radiculopathy. an open-label, dose-escalating study followed by a randomized, double-blind, placebo-controlled trial. Pain Med. (2010) 11:356–68. doi: 10.1111/j.1526-4637.2010.00808.x
24. Piletz JE, Aricioglu F, Cheng JT, Fairbanks CA, Gilad VH, Haenisch B, et al. Agmatine: clinical applications after 100 years in translation. Drug Discov Today (2013) 18:880–93. doi: 10.1016/j.drudis.2013.05.017
25. Zhu MY, Wang WP, Huang J, Feng YZ, Regunathan S, Bissette G. Repeated immobilization stress alters rat hippocampal and prefrontal cortical morphology in parallel with endogenous agmatine and arginine decarboxylase levels. Neurochem Int. (2008) 53:346–54. doi: 10.1016/j.neuint.2008.09.001
26. Gilad GM, Gilad VH. Brain polyamine stress response: recurrence after repetitive stressor and inhibition by lithium. J Neurochem. (1996) 67:1992–6. doi: 10.1046/j.1471-4159.1996.67051992.x
27. Karadag HC, Ulugol A, Tamer M, Ipci Y, Dokmeci I. Systemic agmatine attenuates tactile allodynia in two experimental neuropathic pain models in rats. Neurosci Lett. (2003) 339:88–90. doi: 10.1016/S0304-3940(02)01456-8
28. Onal A, Delen Y, Ulker S, Soykan N. Agmatine attenuates neuropathic pain in rats: possible mediation of nitric oxide and noradrenergic activity in the brainstem and cerebellum. Life Sci. (2003) 73:413–28. doi: 10.1016/S0024-3205(03)00297-2
29. Aricioglu F, Paul IA, Regunathan S. Agmatine reduces only peripheral-related behavioral signs, not the central signs, of morphine withdrawal in nNOS deficient transgenic mice. Neurosci Lett. (2004) 354:153–7. doi: 10.1016/j.neulet.2003.10.010
30. Galea E, Regunathan S, Eliopoulos V, Feinstein DL, Reis DJ. Inhibition of mammalian nitric oxide synthases by agmatine, an endogenous polyamine formed by decarboxylation of arginine. Biochem J. (1996) 316 (Pt 1):247–9.
31. Pinthong D, Wright IK, Hanmer C, Millns P, Mason R, Kendall DA, et al. Agmatine recognizes alpha 2-adrenoceptor binding sites but neither activates nor inhibits alpha 2-adrenoceptors. Naun-Schmied Arch Pharmacol. (1995) 351:10–6. doi: 10.1007/BF00169058
32. Raasch W, Schafer U, Chun J, Dominiak P. Biological significance of agmatine, an endogenous ligand at imidazoline binding sites. Br J Pharmacol. (2001) 133:755–80. doi: 10.1038/sj.bjp.0704153
33. Roerig SC. Spinal and supraspinal agmatine activate different receptors to enhance spinal morphine antinociception. Ann NY Acad Sci. (2003) 1009:116–26. doi: 10.1196/annals.1304.011
34. Lopez MJ, Quinn MM, Markel MD. Evaluation of gait kinetics in puppies with coxofemoral joint laxity. Am J Vet Res. (2006) 67:236–41. doi: 10.2460/ajvr.67.2.236
35. D'Amico LL, Xie L, Abell LK, Brown KT, Lopez MJ. Relationships of hip joint volume ratios with degrees of joint laxity and degenerative disease from youth to maturity in a canine population predisposed to hip joint osteoarthritis. Am J Vet Res. (2011) 72:376–83. doi: 10.2460/ajvr.72.3.376
36. Brown DC, Boston RC, Coyne JC, Farrar JT. Ability of the canine brief pain inventory to detect response to treatment in dogs with osteoarthritis. J Am Vet Med Assoc. (2008) 233:1278–83. doi: 10.2460/javma.233.8.1278
37. Reid J, Nolan A, Hughes J, Lascelles D, Pawson P, Scott E. Development of the short-form Glasgow Composite Measure Pain Scale (CMPS-SF) and derivation of an analgesic intervention score. Anim Welfare (2007) 16:97.
38. Hellyer P, Uhrig S, Robinson N. Canine Acute Pain Scale and Feline Acute Pain Scale. Fort Collins, CO: Colorado State University Veterinary Medical Center (2006).
39. Flückiger M. Scoring radiographs for canine hip dysplasia-The big three organisations in the world. Eur J Companion Animal Pract. (2007) 17:135–40.
40. Lopez MJ, Borne A, Monroe WT, Bommala P, Kelly L, Zhang N. Novel anterior cruciate ligament graft fixation device reduces slippage. Med Devices (2013) 6:59–68. doi: 10.2147/MDER.S43802
41. Brown DC, Boston RC, Farrar JT. Comparison of force plate gait analysis and owner assessment of pain using the canine brief pain inventory in dogs with osteoarthritis. J Vet Internal Med. (2013) 27:22–30. doi: 10.1111/jvim.12004
42. McKellar QA, Delatour P, Lees P. Stereospecific pharmacodynamics and pharmacokinetics of carprofen in the dog. J Vet Pharmacol Ther. (1994) 17:447–54. doi: 10.1111/j.1365-2885.1994.tb00276.x
43. Hampshire VA, Doddy FM, Post LO, Koogler TL, Burgess TM, Batten PO, et al. Adverse drug event reports at the United States Food And Drug Administration Center for Veterinary Medicine. J Am Vet Med Assoc. (2004) 225:533–6. doi: 10.2460/javma.2004.225.533
44. Epstein ME, Rodan I, Griffenhagen G, Kadrlik J, Petty MC, Robertson SA, et al. 2015 AAHA/AAFP pain management guidelines for dogs and cats. J Feline Med Surgery (2015) 17:251–72. doi: 10.1177/1098612X15572062
45. Courteix C, Privat AM, Pelissier T, Hernandez A, Eschalier A, Fialip J. Agmatine induces antihyperalgesic effects in diabetic rats and a superadditive interaction with R(-)-3-(2-carboxypiperazine-4-yl)-propyl-1-phosphonic acid, a N-methyl-D-aspartate-receptor antagonist. J Pharmacol Exp Ther. (2007) 322:1237–45. doi: 10.1124/jpet.107.123018
46. Lipscomb VJ, AliAbadi FS, Lees P, Pead MJ, Muir P. Clinical efficacy and pharmacokinetics of carprofen in the treatment of dogs with osteoarthritis. Vet Record (2002) 150:684–9. doi: 10.1136/vr.150.22.684
47. Mirza MH, Bommala P, Richbourg HA, Rademacher N, Kearney MT, Lopez MJ. Gait changes vary among horses with naturally occurring osteoarthritis following intra-articular administration of autologous platelet-rich plasma. Front Vet Sci. (2016) 3:29. doi: 10.3389/fvets.2016.00029
48. van Klaveren NJ, Suwankong N, De Boer S, van den Brom WE, Voorhout G, Hazewinkel HA, et al. Force plate analysis before and after dorsal decompression for treatment of degenerative lumbosacral stenosis in dogs. Vet Surgery (2005) 34:450–6. doi: 10.1111/j.1532-950X.2005.00068.x
49. Tsiklauri N, Nozadze I, Gurtskaia G, Tsagareli MG. Antinociceptive tolerance to NSAIDs in the rat formalin test is mediated by the opioid mechanism. Pharmacol Reports (2017) 69:168–75. doi: 10.1016/j.pharep.2016.10.004
50. Tsiklauri N, Gurtskaia G, Tsagareli M. Study of non-opioid analgesics tolerance in young and adult rats. Georgian Medical News (2008) 158:40–4.
51. Plessas IN, Rusbridge C, Driver CJ, Chandler KE, Craig A, McGonnell IM, et al. Long-term outcome of Cavalier King Charles spaniel dogs with clinical signs associated with Chiari-like malformation and syringomyelia. Vet Rec. (2012) 171:501. doi: 10.1136/vr.100449
Keywords: Agmatine, coxofemoral joint, hip dysplasia, osteoarthritis, intervertebral disc, gait, ground reaction force, canine
Citation: Taguchi T, Koh R, Takawira C, Rademacher N, Gilad GM, Aronson RD and Lopez MJ (2018) Agmatine for Pain Management in Dogs With Coxofemoral Joint Osteoarthritis: A Pilot Study. Front. Vet. Sci. 5:311. doi: 10.3389/fvets.2018.00311
Received: 12 July 2018; Accepted: 26 November 2018;
Published: 12 December 2018.
Edited by:
Fausto Cremonesi, University of Milan, ItalyReviewed by:
Sherman Orye Canapp, Veterinary Orthopedic Sports Medicine Group, United StatesMaria Fahie, Western University of Health Sciences, United States
Copyright © 2018 Taguchi, Koh, Takawira, Rademacher, Gilad, Aronson and Lopez. This is an open-access article distributed under the terms of the Creative Commons Attribution License (CC BY). The use, distribution or reproduction in other forums is permitted, provided the original author(s) and the copyright owner(s) are credited and that the original publication in this journal is cited, in accordance with accepted academic practice. No use, distribution or reproduction is permitted which does not comply with these terms.
*Correspondence: Mandi J. Lopez, bWxvcGV6QGxzdS5lZHU=