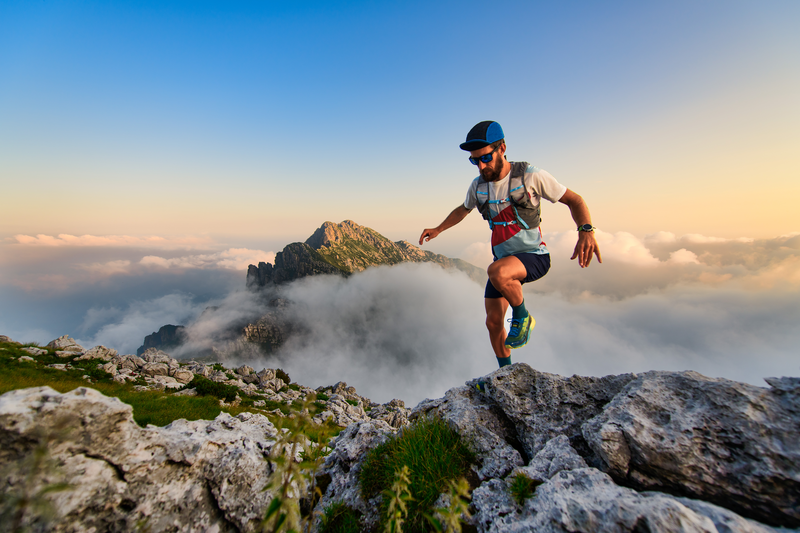
95% of researchers rate our articles as excellent or good
Learn more about the work of our research integrity team to safeguard the quality of each article we publish.
Find out more
ORIGINAL RESEARCH article
Front. Vet. Sci. , 20 November 2018
Sec. Comparative and Clinical Medicine
Volume 5 - 2018 | https://doi.org/10.3389/fvets.2018.00288
This article is part of the Research Topic One Step at a Time: Advances in Osteoarthritis View all 12 articles
Galectins are potent regulators of cell adhesion, growth and apoptosis in diverse cell types, including chondrocytes and synovial fibroblasts. Elevations in synovial fluid galectin-3 have been observed in rheumatoid arthritis, juvenile idiopathic arthritis and experimental inflammatory arthritis in animal models, whereas galectin-1 is thought to be protective. Less is known about galectins-1 and-3 in osteoarthritis (OA). Therefore, the purpose of this study was: (1) to determine whether galectin-1 and-3 synovial fluid concentrations and synovial membrane and cartilage histochemical staining were altered following osteochondral injury in an experimental equine osteoarthritis (OA) model and (2) to measure galectin-1 and-3 mRNA expression and synovial fluid concentrations in naturally occurring equine carpal OA. Synovial fluid galectin-1 and-3 concentrations were quantified using custom ELISAs in two research horse cohorts undergoing experimental OA induction (n = 5 and 4) and in a cohort of horses with naturally occurring carpal OA (n = 57). Galectin mRNA expression in synovial membrane and cartilage tissue obtained from carpal joints of horses with naturally occurring OA was measured using RT-qPCR, and galectin immunostaining was assessed in synovial membrane and osteochondral tissues in the experimental model (n = 5). Synovial fluid galectin-1 and-3 concentrations increased following experimental carpal osteochondral fragmentation. Cartilage galectin-1 mRNA expression increased with OA severity in naturally occurring disease. The superficial zone of healthy articular cartilage stained intensely for galectin-3 in sham-operated joints, whereas galectin-1 staining was nearly absent. Chondrocyte galectin-1 and-3 immunoreactivity increased following cartilage injury, particularly in galectin-1 positive chondrones. Galectins-1 and-3 are present in healthy equine synovial fluid and increase following post-traumatic OA. Healthy superficial zone chondrocytes express galectin-3, whereas galectin-1 chondrocyte staining is limited predominantly to chondrones and injured cartilage. Further work is needed to clarify the functions of galectins-1 and-3 in healthy and OA joints.
Galectins are potent regulators of cell adhesion, growth, and apoptosis in diverse tissues and organs, including synovial joints. Galectin-1 and galectin-3 are expressed in synovial fibroblasts, articular chondrocytes, and hypertrophic growth plate chondrocytes (1–3). Synovial fibroblasts express higher levels of galectin-1 and-3 as compared to articular chondrocytes (4), and intracellular galectin-3 promotes chondrocyte survival in both articular and hypertrophic chondrocytes (5, 6).
Associations between increased galectin-3 in synovial fluid, synovial tissues, and sera of human patients with inflammatory arthritis have been observed in several studies (7–9), and strong expression of galectin-3 at sites of joint destruction has led authors to suggest that galectin-3 plays a role in rheumatoid arthritis (RA) pathogenesis (10). Some authors have even proposed that galectin-3 may be a potential therapeutic target for RA (11, 12). Conversely, decreased galectin-1 concentrations and increased anti-Gal-1 antibodies have been detected in RA patients (13). Galectin-1 expression is downregulated and galectin-3 expression is upregulated in synovial tissue from patients with juvenile idiopathic arthritis (8). Most rodent experimental models of inflammatory arthritis suggest that galectin-1 is protective (14–17), whereas galectin-3 promotes joint inflammation (16, 18). For example, galectin-1 knockout (KO) mice develop earlier onset and more severe collagen-induced arthritis (14), and galectin-3 KO mice have reduced inflammation and bone erosion in response to antigen-induced arthritis as compared to wild-type mice (18). In addition, while both recombinant protein and genetic delivery of galectin-1 are protective in rodent models of collagen-induced arthritis (15–17), administration of galectin-3 shRNA protects rodents from collagen-induced arthritis (16). Classification of galectin-3 as a driver or inhibitor of inflammatory arthritis is likely affected by its intracellular or extracellular localization (6, 19), with rodent knockout models emphasizing intracellular galectin signaling.
The role of galectins in osteoarthritis (OA) and post-traumatic osteoarthritis (PTOA) is not well understood. What little is known about galectin-1 and-3 synovial fluid levels or synovial membrane localization in human OA is derived from studies where OA patients were used as a comparison group to RA patients (7). Galectin-1 and-3 levels have not been evaluated in synovial fluid from healthy human patients, with the exception of a “healthy control” group in two studies in which synovial fluid was obtained pre-operatively from patients with knee trauma or meniscal tears (7, 20). Lectin/galectin staining in human OA cartilage has revealed increased galectin-1 and galectin-3 chondrocyte immunostaining at sites of cartilage damage (21–23), with increasing galectin-1 positivity correlated with cartilage Mankin scores (21, 23). Galectin-1 and-3 mRNA was expressed in human OA chondrocytes at higher levels than galectins-2, 4, 7, 8, and 9; however, levels of galectin expression in healthy chondrocytes were not studied (23). Investigation of in vitro signaling pathways in human OA chondrocytes revealed that both galectins-1 and-3 promote an inflammatory gene signature, at least in part through their role as upstream NF-κB signaling effectors (21, 24). On the other hand, galectin-3 KO mice demonstrate increased cartilage damage in a mono-iodoacetate injection model of OA (6), and galectin-3 KO mice also demonstrate increased bone resorption and accelerated trabecular bone loss as compared to wild-type mice (25), suggesting a protective role for galectin-3 in OA.
To the authors' knowledge, galectins have only been evaluated in animal models of inflammatory arthritis and not in PTOA models. Therefore, critical gaps in knowledge include understanding: (1) how synovial fluid galectin-1 and-3 concentrations change over time following joint injury, (2) how galectin-1 and-3 mRNA expression and synovial fluid levels differ in healthy as compared to OA joints, and (3) whether galectin-1 and-3 immunostaining differs between healthy and OA cartilage in PTOA. Horses are athletic animals that commonly develop PTOA in the course of their performance careers (26). Synovitis, cartilage impact injury, osteochondral fragmentation and subchondral bone injury are common in the high-motion carpal joints (27). Because PTOA in horses can better recapitulate certain aspects of human PTOA pathogenesis than chemically induced models in rodents (28), we chose to evaluate galectins-1 and-3 in the equine model. Synovial fluid and articular tissues were obtained from horses with naturally occurring OA, and the carpal osteochondral fragment high-speed treadmill exercise model of OA was used to evaluate serial changes in synovial fluid galectins and cartilage immunohistochemistry. Biochemical, histologic, and inflammatory changes are well-characterized in the equine carpal osteochondral fragment model of OA (27, 29, 30), and this model is commonly used to test the therapeutic effects of intra-articular or systemic OA therapies (27, 31).
Therefore, the objectives of this study were to: (1) compare galectin-1 and-3 mRNA expression and synovial fluid concentrations in healthy and OA joint tissues from horses with naturally occurring OA, and (2) to determine whether galectin-1 and-3 serial synovial fluid concentrations and galectin immunostaining were altered following osteochondral injury in an experimental equine OA model.
All experimental protocols were approved by the university Institutional Animal Care and Use Committee (protocol numbers: 2011-0027 and 2012-0097). All sample collection was performed following humane euthanasia of horses using sodium pentobarbital or obtained from discarded tissues following arthroscopic surgery of horses with informed consent from owners.
Synovial fluid samples collected from two distinct equine experimental cohorts (n = 5 and n = 4) undergoing carpal fragmentation were used to measure serial galectin-1 and-3 concentrations. Synovial membrane biopsies and osteochondral tissues were collected from the first cohort (n = 5) for immunohistochemistry following euthanasia on day 70 post-fragmentation. Analysis of synovial fluid lubricin concentrations and lubricin immunostaining has previously been reported in the first cohort (32). Horses in both cohorts were subjected to carpal osteochondral fragmentation in one randomly assigned joint, while the opposite joint served as a sham-operated control. Two weeks post-operatively, horses commenced a high-speed treadmill exercise program 5 times weekly, continuing throughout the study duration of either 70 or 75 days. Five Thoroughbred horses (n = 3 females and 2 castrated males), aged 2–6 years old, were enrolled in the first cohort, and four Thoroughbred horses (n = 2 females and 2 castrated males), aged 2–6 years old, were enrolled in the second cohort. Experimental protocols were roughly similar between the two groups; however, the timing of synovial fluid collection and study duration differed slightly. All horses were housed in 3.65 × 3.65 m box stalls and engaged in similar treadmill exercise programs, consisting of walking (5 km/h) for 5 min, followed by trotting (16–18 km/h) for 2 min, galloping (28–32 km/h) for 2 min, and ending with 2 min of trotting (16–19 km/h) exercise performed in the morning. Synovial fluid aspirates were processed similarly, and synovial fluid supernatants were stored in aliquots at −80°C following centrifugation at 3,000x g for 5 min to pellet any cellular debris. Synovial fluid samples were collected and banked from the first cohort approximately 2 years prior to the second cohort, and all samples were frozen at −80°C for long-term storage. Synovial fluid samples from the first cohort were subjected to up to 3 freeze-thaw cycles prior to ELISA measurements, whereas samples from the second cohort were only subjected to 1 freeze-thaw cycle.
Synovial fluid and discarded tissues, including synovial membrane and osteochondral tissues, were harvested where available from the antebrachialcarpal (ACJ) and middle carpal joints (MCJ) of horses undergoing arthroscopic surgery at the Cornell University Equine Hospital, with informed owner consent. Each joint was assessed as healthy (grade 0) or assigned an osteoarthritis (OA) severity score of mild (1), moderate (2) or severe (3) on the basis of radiographic evidence of osteophytes, enthesiophytes, osteoproliferation, joint space narrowing or chronic fracture lines as previously described (33). Synovial fluid and tissues were also collected from horses donated for research purposes, and joint scores were assessed on the basis of radiographic and/or gross dissection findings. Thoroughbred, Standardbred or Quarter Horse females (n = 34), intact males (n = 6), or castrated males (n = 17) ranging in age from 2 to 13 years were included. A total of 54 and 52 synovial fluid samples were quantified using galectin-1 and-3 ELISAs, and 57 synovial membrane and 34 cartilage tissue samples were analyzed via RT-qPCR.
Equine galectin-1 (GenBank ID: KY264050.1) and galectin-3 (GenBank ID: KY264051.1) were cloned, recombinantly expressed in E. coli, and purified using lactosyl sepharose chromatography and FPLC gel filtration as previously reported (34). Galectin ELISA antibody reactivity to recombinant equine galectin standards has previously been described (4). Both the goat anti-mouse Gal-1 antibody (AF1245; R&D Systems, Minneapolis, MN) and the goat anti-human Gal-3 antibody (sc-19280; Santa Cruz Biotechnology, Dallas, TX) were biotinylated using the Mix-n-Stain biotin antibody labeling kit (Biotium Inc., Fremont, CA), and biotinylated goat anti-mouse Gal-3 antibody (BAF1197, R&D Systems, Minneapolis, MN) was obtained from the manufacturer. Synovial fluid samples were resolved on 12% SDS-PAGE gels and probed with the biotinylated primary antibodies to confirm antibody reactivity to equine synovial fluid galectins. All 3 biotinylated primary antibodies were used at a concentration of 0.15 μg/mL in blocking buffer (3% BSA in 0.1% PBS-Tween for anti-mouse Gal-1 and anti-mouse Gal-3 antibodies; 1% Gelatin in 0.1% PBS-Tween for anti-human Gal-3). Streptavidin-HRP was applied at 4 ng/mL in 0.1% PBS-Tween for all blots.
Custom galectin-1 competitive inhibition ELISAs (4) were performed on banked equine synovial fluid samples from two cohorts of horses subjected to carpal osteochondral fragmentation. Briefly, 96-well high-binding plates (Corning Inc., Corning, NY) were coated with 1 μg/mL of goat anti-mouse Gal-1 capture antibody in sodium carbonate buffer, pH 9.6 at 4°C. After 3 rinses in 0.1% PBS-Tween, protein free blocking buffer (Thermo Fisher Scientific, Rockford, IL) was added for 1 h. Unlabeled recombinant equine galectin-1 standards (2 μg/mL to 15.6 ng/mL) were diluted in 200 ng/mL biotinylated recombinant equine galectin-1 in 0.1% PBS-Tween. Synovial fluid samples pre-diluted 1:50 in PBS were further diluted 1:1, for a final dilution of 1:100, in 200 ng/mL of biotinylated recombinant equine galectin-1. Blocking buffer was aspirated, and 100 μL of recombinant equine galectin-1 standards or synovial fluid samples were added to the plate in duplicate and incubated for 1 h at RT. Plates were rinsed in 0.1% PBS-Tween, incubated with 100 μL of streptavidin HRP for 30 min. TMB reagent was added for 10 min prior to halting the reaction with 1N H2SO4. Absorbance was measured at 450 nm with 540 nm background subtraction.
For the custom galectin-3 sandwich ELISA, 96-well plates were coated with 2 μg/mL of goat anti-human Gal-3 capture antibody (sc-19280) using similar methodology as for Gal-1. After rinsing in 0.1% PBS-Tween, protein-free blocking buffer was added for 1 h, followed by serial dilutions of recombinant equine galectin-3 standards (400 ng/mL to 1.6 ng/mL) in duplicate. Synovial fluid samples pre-diluted 1:50 in PBS were further diluted 1:1 in 1% BSA in PBS, for a final dilution of 1:100, and added in duplicate to the plate. Following 1 h incubation at RT, the plate was rinsed in 0.1% PBS-Tween, and biotinylated goat anti-mouse Gal-3 pAb (BAF1197) was added at 200 ng/mL for 1 h. Following rinsing, 100 μL of streptavidin HRP was added for 30 min prior to adding 1N H2SO4. Absorbance was measured at 450 nm with 540 nm background subtraction.
Synovial membrane and cartilage tissues were snap-frozen in liquid nitrogen and stored at −80°C for up to 3 years. The frozen tissues were crushed and ground into fine powder in liquid nitrogen with a mortar and pestle prior to isolation of RNA. Total RNA was extracted using the E.Z.N.A Tissue RNA Kit (Omega BioTek, Inc., Norcross, GA) for synovial membrane or the RNeasy Lipid Tissue Mini Kit (QIAGEN Sciences Inc., Germantown, MD) for cartilage. DNase I was added during RNA extraction to remove genomic DNA. RNA purity and concentration were assessed with a multimode plate reader (Tecan Spark® 10M, Tecan, Austria) with a NanoQuant PlateTM. Expression of galectin-1 and galectin-3 mRNA was quantified using the Taqman RNA-to-CT one-step kit (Applied Biosystems, Foster City, CA) and the ABI PRISM 7900 HT Sequence Detection System (Applied Biosystems, Foster City, CA). As previously reported, Primer Express Software Version 2.0 (Applied Biosystems, Foster City, CA) was used to design forward and reverse primers and probes for equine galectins-1 and-3 (4). Primer and probe sequences are listed in Table 1. Equal amounts (10 ng per reaction) of total RNA were added in a 20 μl reaction volume for all samples. The RT-qPCR reactions were all performed in duplicate. The levels of gene expression were calculated using the standard curves generated with serial dilutions of E. coli-expressed equine Gal-1 and Gal-3 as standards. All data were normalized with the housekeeping gene equine 18S rRNA. A qPCR checklist is provided to document the technical aspects of qPCR Protocols (Supplementary Material).
Synovial membrane and osteochondral tissue sections from the radial carpal bone and opposing third carpal bone were stained for galectin-1 and galectin-3 using previously reported techniques (34). Briefly, osteochondral sections were fixed in 4% paraformaldehyde, de-calcified in 10% EDTA for 3 weeks and embedded in paraffin, while synovial membrane sections were embedded in paraffin immediately after fixation. Following deparaffinization, sections were treated with 1% hyaluronidase (Sigma-Aldrich, St. Louis, MO) in 20 mM sodium acetate for 30 min at 37°C, followed by 3% hydrogen peroxide for an additional 30 min. Blocking in normal rabbit serum was performed, followed by incubation with a goat anti-mouse galectin-1 antibody (AF1245; R&D) or goat anti-human galectin-3 antibody (sc-19280; Santa Cruz) at 1:100 dilution for 1 h at room temperature. After washing, sections were incubated with a biotinylated rabbit anti-goat IgG (Vectastain, Vector Labs) and immunodetected with the Vectastain ABC Kit and ImmPACT DAB reagent (Vector Labs). Negative controls were performed by omission of primary antibody. Sections were rinsed in PBS, counterstained with Harris hematoxylin, coverslipped and imaged with a 20x objective using a ScanScope (ScanScope CS0, Aperio). Images were saved as.tif files in Aperio's Image Scope software, cropped in Adobe Photoshop CC and formatted in Adobe Illustrator CC.
Synovial membrane tissue sections were imaged but not quantified due to the presence of strong galectin-1 and galectin-3 immunostaining in all sections. Osteochondral sections from the radial carpal bone and third carpal bone were scored independently by two observers. Although observers were blinded to individual animal identity, blinding to treatment group (sham-operated or OA) was not possible due to the presence of obviously injured articular cartilage within some osteochondral sections. Where possible, separate scores were assessed for injured cartilage regions vs. healthy cartilage regions within the same section. Chondrocytes within each cartilage zone (superficial, middle and deep) were assigned an immunostaining intensity score for both galectin-1 and galectin-3 where: 0–none, 1–weak, 2–moderate, and 3–strong. In addition, the percentage of galectin-1-positive chondrocytes was calculated for the entire articular cartilage section, including superficial, middle, and deep zones combined.
To assess the effect of treatment (sham vs. OA) and time (day) on galectin concentrations in synovial fluid, galectin ELISA data were first tested for normality using a Shapiro-Wilk W test and were found to be right skewed. Log transformation was performed to achieve normality. In order to account for the hierarchical nature of the data in the experimental models, a mixed linear model was employed because each horse was repeatedly measured on each limb and each limb repeatedly over days. The fixed effects in the model included treatment (sham vs. OA), day and a treatment*day interaction term, and random effects included horse and individual limb nested within horse to account for the non-independence of the observations. Predefined post hoc comparisons of specific contrasts for each time point were performed to assess differences between sham and OA joints, with a Bonferroni correction applied based on the number of multiple comparisons to correct for the false discovery rate. Model diagnostics were performed and showed normality and homoscedasticity of residuals. Spearman correlation analysis was performed to determine associations between synovial fluid galectin-1 and galectin-3 levels and previously published lubricin ELISA data for the first experimental cohort and for the naturally occurring OA cases (32). Raw RT-qPCR and ELISA data from naturally occurring samples were log-transformed to achieve normality of the data, and data were analyzed using a one-way ANOVA with Dunnett's post hoc tests for multiple comparison correction, designating healthy carpal joints (OA severity = 0) as the control group. Significance was set at α = 0.05.
Immunohistochemistry scores (0 to 3 scale) were treated as ordinal categorical outcomes, and weighted kappa statistics were calculated for inter-observer agreement. Immunostaining results were assessed using Wilcoxon matched-pairs signed rank tests due to the small sample size and non-normal distribution of data. For the percentage of galectin-1 positive chondrocytes throughout the entire cartilage section, scores were treated as continuous outcomes and analyzed using Wilcoxon matched-pairs signed rank tests. All modeling and parametric analyses were performed using JMP Pro 13 software (SAS; Cary, NC), and non-parametric test statistics, kappa statistics and graphs were generated using Prism 7 (GraphPad; La Jolla, CA).
Synovial fluid galectin-1 and galectin-3 concentrations increased following carpal osteochondral fragmentation in both experimental cohorts (Figure 1). In the carpal osteochondral fragment model, galectin-1 synovial fluid concentrations increased by up to 4-fold (median: 74.8 μg/mL vs. 16.9 μg/mL on day 7), and galectin-3 synovial fluid concentrations increased by as much as 5-fold in the OA joint as compared to the sham-operated joint (median: 24.3 ng/mL vs. 4.6 ng/mL on day 14). Galectin-1 synovial fluid concentrations were most elevated acutely after injury, whereas elevations in galectin-3 were sustained up to the end of the study duration (day 75) in one cohort of horses (Figure 1D). Galectin-3 was moderately correlated with synovial fluid lubricin (ρ = 0.47, P < 0.0001), whereas galectin-1 was weakly correlated with lubricin (ρ = 0.27, P = 0.007) (32). Galectin-1 and-3 synovial fluid levels were weakly correlated (ρ = 0.31, P = 0.002).
Figure 1. Galectin-1 (A,B) and galectin-3 (C,D) concentrations in equine synovial fluid prior to (day 0) and after arthroscopically-induced osteochondral fragmentation (OA) or sham operation (Sham). Data are displayed as box-and-whisker plots representing the first and third quartiles, median, and spread of concentrations for each serial sampling time point. ELISA data in (A,C) were obtained from the same cohort of horses (n = 5), and ELISA data in (B,D) were obtained from a second cohort of horses (n = 4). Mixed linear model derived P-values for the fixed effect of treatment and day were < 0.001 for models A-D, except for D where day P = 0.32. An interaction between treatment and day was identified for model A (treatment * day P = 0.0007) and C (treatment * day P = 0.03). Note that there is variation in the date of synovial fluid sampling between the two cohorts. Asterisks denote days where OA galectin concentrations were significantly increased as compared to Sham, * = P < 0.05 after Bonferroni correction.
No differences in galectin-1 or galectin-3 concentrations were detected in healthy joints as compared to joints with naturally occurring OA (Figure 2). Galectin-1 and galectin-3 synovial fluid concentrations were weakly correlated (ρ = 0.28, P = 0.04).
Figure 2. Galectin-1 and-3 concentrations in equine synovial fluid obtained from carpal joints of horses with naturally occurring osteoarthritis (OA), classified by severity as mild (1), moderate (2) or severe (3) and from healthy carpal joints (0). Individual data are shown as scatterplots, in addition to means ± S.E.M. There were no significant differences in synovial fluid galectin-1 (A) or galectin-3 (B) concentrations between healthy and OA joints.
There were no differences in galectin-1 or galectin-3 mRNA expression in synovial membrane from healthy and OA joints (Figures 3A,B). In contrast, galectin-1 mRNA expression was significantly upregulated in moderate and severe OA cartilage, with an approximately 12- and 75-fold increase as compared to healthy cartilage (Figure 3C). Galectin-1 mRNA expression was minimal in healthy (grade 0) cartilage. Galectin-3 mRNA levels were greater in severe OA as compared to mild OA (Figures 3C,D).
Figure 3. Galectin-1 and-3 copy # per ng of RNA from synovial membrane (A,B) or cartilage (C,D) tissue obtained from healthy carpal joints (0) or carpal joints with naturally occurring osteoarthritis (1–mild, 2–moderate, 3–severe OA). There were no differences in galectin-1 or-3 mRNA expression in synovial membrane tissue (A,B). Cartilage galectin-1 mRNA expression increased with OA severity score (C, P = 0.0002), whereas galectin-3 mRNA expression was increased in severe OA cartilage as compared to mild OA cartilage (D, P = 0.03). Scatterplots are displayed, with bars representing mean ± S.E.M. Differing letters note statistically significant differences, P < 0.05.
Synovial membrane tissue sections stained intensely for both galectin-1 and galectin-3 (Figure 4), with the most prominent immunoreaction observed in perivascular and intimal regions. Consistent with RT-qPCR data, no differences were noted in galectin synovial membrane immunostaining between sham-operated and OA joints on day 70 post-fragmentation. Weighted kappa statistics revealed moderate inter-observer agreement for both galectin-1 and-3 chondrocyte immunostaining (0.55 and 0.43, respectively). Safranin O staining was decreased in areas of partial-thickness cartilage fibrillation in the osteochondral fragment joint as compared to the sham-operated joint (Figures 5A–D). Superficial zone chondrocytes from healthy articular cartilage stained intensely for galectin-3 (Figures 5I,J), whereas galectin-1 staining was nearly absent (Figures 5E,F), consistent with gene expression data and prior immunostaining results in healthy equine cartilage (34). Galectin-1 immunostaining was increased in superficial and middle zone chondrocytes from injured cartilage (Figures 5G,H, P = 0.02 and 0.03, respectively) and was minimal to absent in deep zone chondrocytes in all cartilage sections. The most intense galectin-1 staining was localized to dividing chondrocytes and chondrocyte clusters (chondrones) within the superficial zone (Figure 5H, arrow). Chondrocyte galectin-1 immunoreactivity was significantly increased in cartilage from the osteochondral fragment joint as compared to the sham-operated joint (Figure 6).
Figure 4. Synovial membrane galectin-1 and galectin-3 immunostaining reveals constitutive galectin expression in both sham-operated (A,D) and osteochondral fragment joints (B,E) from a representative horse 70 days post-injury. Galectin-1 and-3 staining is most prominent in perivascular regions. No differences were observed in galectin-1 or-3 staining between sham-operated and osteochondral fragment joints. Primary antibodies were omitted in (C,F), revealing absence of antigen-independent staining. Scale bar: 200 μm.
Figure 5. Safranin O/Fast Green (A–D), galectin-1 (E–H), and galectin-3 (I–L) immunostaining of third carpal bone cartilage from sham-operated and osteochondral fragment joints from a representative horse 70 days post-injury. Superficial zone chondrocytes and some middle zone chondrocytes stain positively for galectin-3 (I,J) but not galectin-1 (E,F) in sham-operated joints. Cartilage fibrillation, proteoglycan loss and chondrone (arrow) formation is observed in cartilage from the osteochondral fragment joint (C,D). Chondrones stain positively for galectin-1 (G,H-arrow) and galectin-3 (K,L-arrow).
Figure 6. Chondrocyte galectin-1 positive immunoreactivity over the entire thickness of articular cartilage in the equine carpal fragmentation experimental model 70 days post-injury (n = 5). Data are presented as box-and-whiskers plots, where boxes represent the first and third quartiles, the lines within the boxes represent the median, and the lines outside the boxes represent the spread of galectin-1 scores. The P-value indicates the difference between injured (OA) and healthy (Sham) cartilage, produced by Wilcoxon matched-pairs signed rank test.
There were no differences in galectin-3 chondrocyte immunostaining between healthy and injured cartilage. Superficial zone chondrocytes demonstrated intense galectin-3 expression in both healthy and injured cartilage (Figures 5I–L).
Galectins-1 and-3 are present in healthy equine synovial fluid and synovial tissue but increase in response to osteochondral injury. In prior work, we have demonstrated greater galectin-1 and-3 mRNA expression in healthy equine synovial membrane as compared to healthy articular cartilage (4), suggesting that the synovium may be the predominant source of synovial fluid galectins. Synovial fluid galectins-1 and-3 were elevated in experimental OA, with a transient increase in galectin-1 and a sustained increase in galectin-3. Cartilage galectin-1 mRNA expression increased with increasing OA severity, and galectin-1 immunostaining was increased in superficial and middle zone chondrocytes in injured cartilage. Whereas, galectin-3 was constitutively produced by superficial zone chondrocytes, galectin-1 immunostaining was nearly absent in healthy articular cartilage. Thus, although both galectin-1 and-3 synovial fluid concentrations are increased in joint injury, galectin-1 upregulation in injured chondrocytes appears to be a specific response to cartilage injury in horses.
Galectin-1 and-3 synovial fluid concentrations were increased in injured as compared to sham-operated joints. To our knowledge, this is the first study to profile serial galectin synovial fluid measurements prior to and after joint injury, revealing a transient elevation in galectin-1 after injury and a more sustained elevation in galectin-3. This longitudinal data suggests that galectins are increased in response to traumatic joint injury. Although these observations were supported by two distinct equine experimental cohorts, differences in synovial fluid galectin levels were not observed in horses with naturally occurring carpal OA. Because the OA severity grading scale for naturally occurring carpal injury involves assessment of radiographic changes, which lag behind inflammatory changes, and because the OA severity score doesn't account for the duration of injury prior to presentation, we may be missing more acute, transient elevations in galectins in horses at the time that they present to the hospital for surgical treatment.
We observed increased galectin-1 mRNA expression and immunostaining in injured equine cartilage. Interestingly, galectin-1 cartilage mRNA expression increased proportionally with the severity of arthritis. Our findings that galectin-1 mRNA expression is increased in OA cartilage and that galectin-1 immunostaining is increased in regions of focal cartilage damage coincide with prior studies in human OA cartilage (21–23). Differences in galectin-1 immunostaining were not observed in deep zone chondrocytes in the current study; however, cartilage pathology was primarily restricted to the superficial and middle zones in this equine OA model and could explain why galectin-1 immunostaining was not observed in deep zones.
Galectin-1 stimulates a network of NF-kB downstream signaling in human OA chondrocytes as demonstrated by microarray and RT-qPCR (21). Synergistic effects of galectins-1,-3, and−8 have been demonstrated in human OA chondrocytes, providing evidence for cooperativity within this galectin signaling network (24, 35). On the other hand, galectin-1 is suggested to mitigate inflammatory arthritis in rodent models by altering immune cell function, inducing apoptosis of CD4+ T cells and decreasing pro-inflammatory cytokine expression (14–17). Notably, inflammatory arthritis and post-traumatic osteoarthritis are distinct entities despite sharing some similarities, such as synovial inflammation and synovitis (36, 37).
In addition to gene expression data demonstrating that synovial membrane is the predominant source of galectins in synovial joints, galectin immunostaining revealed prominent galectin-1 and-3 immunolocalization in synovial membrane tissue from both sham-operated and OA joints. Whereas, superficial zone chondrocytes consistently stained positively for galectin-3 in healthy cartilage, middle and deep zone chondrocytes were negative. Similar patterns of strong superficial zone chondrocyte galectin-3 staining have been previously observed in healthy equine and human cartilage (2, 34). Chondrocytes in healthy cartilage were immune negative for galectin-1. Galectin-1 positive chondrocytes were only detected in injured cartilage, especially in dividing chondrocytes or chondrones in the superficial and middle zones of injured, fibrillated cartilage.
The identification of increased galectin-3 in RA (7, 38) and juvenile idiopathic arthritis (8) has led authors to hypothesize that galectin-3 precipitates inflammatory arthritis. Elevations in serum galectin-3 concentrations have been detected in patients with early RA and correlated with MRI bone lesions 1 year later (39). However, the link between galectin-3 and OA is less clear and, to our knowledge, no human studies have documented elevations in synovial fluid galectin-3 levels preceding the development of arthritis. Data on the role of galectin-3 in experimental rodent models is conflicting. Gal-3 KO mice are predisposed to OA (6), potentially due to the protective role that galectin-3 plays in chondrocyte survival (5, 6). Galectin-3 KO mice also demonstrate decreased bone formation, increased bone resorption, accelerated trabecular bone loss and reduced bone strength as compared to wild-type mice, suggesting an important role for galectin-3 in bone remodeling and biomechanics (25). On the other hand, exogenous intra-articular galectin-3 administration promoted the development of arthritis in mice (19), and inhibition of galectin-3 through lentiviral-mediated delivery of galectin-3 shRNA ameliorated collagen-induced arthritis in rats (16). The conflicting data with respect to galectin-3 and OA may be due to the distinct functions of intracellular vs. extracellular galectin-3 and differences between inflammatory and PTOA models of arthritis. Intracellular gal-3 promotes chondrocyte survival both in vitro (6) and in vivo (6); whereas administration of exogenous, extracellular galectin-3 exacerbates inflammation (16, 18). Notably, most rodent studies investigating galectins and arthritis have focused on inflammatory models which more closely mimic RA. Horses and other large animal models are more commonly used to study PTOA and better represent the clinical scenario for translation to PTOA in humans (28, 40). Therefore, future work is needed to determine whether increased synovial fluid galectin-3 concentrations in equine PTOA are functioning to protect articular chondrocytes, promote synovial inflammation or both.
Here, we demonstrate constitutive expression of both galectin-1 and-3 in healthy synovial membrane tissue and synovial fluid, while also elucidating the time course of galectin-1 and-3 upregulation following induction of post-traumatic OA. Toegel and Weinmann et al. have suggested that both galectin-1 (21) and galectin-3 (24) promote OA through upstream regulation of NF-kB signaling in chondrocytes. Accordant with these findings, we show that galectin-1 mRNA expression and immunostaining is increased in equine OA cartilage as compared to healthy cartilage. Overall, our data suggests that galectin-1 mRNA expression and protein production is increased in injured equine articular chondrocytes, similar to injured human cartilage (21, 22). Galectin-1 appears to be more specific to articular cartilage injury in horses than galectin-3. In addition, our data suggests that synovial membrane and cartilage galectin expression patterns differ, with constitutive galectin-1 and-3 synovial membrane expression present in all sham-operated, healthy joints.
This study provides evidence for the constitutive expression and production of galectins-1 and-3 in healthy synovial joints. Whereas, galectin-3 is constitutively produced in superficial zone chondrocytes in healthy articular cartilage, both galectin-1 and-3 are expressed in healthy synovial membrane tissue. In addition, we demonstrate that synovial fluid galectins are elevated in response to PTOA and that cartilage galectin-1 expression strongly correlates with OA progression. Galectins may be potential upstream therapeutic targets in OA; however, further work is needed to clarify the mechanistic roles of galectins-1 and-3 in synovial membrane tissue, cartilage and synovial fluid in PTOA. Several small-molecule galectin antagonists and anti-galectin monoclonal antibodies are currently undergoing preclinical testing for fibrosis and cancer therapy and may have applications in other chronic inflammatory diseases, such as OA and RA (41). However, therapeutic targeting of galectins also poses significant challenges due to the context-dependent multifunctionality of galectin signaling (42) and the ability of other galectin family members to compensate for the loss of an individual galectin (35). In addition, because galectins are constitutively expressed in several tissues, off-target effects are of potential concern (43, 44). Constitutive expression of galectins in synovial fluid also suggests that there may be functional roles for galectins in healthy synovial joints, including beneficial roles in cartilage lubrication (34). Additional research is needed to clarify the functions of galectins-1 and-3 in healthy joints and in PTOA in both experimental animal models and human patients. Translational animal models will be critical for pre-clinical testing of galectin-targeted therapies for human OA.
Datasets for equine galectin genomic sequences can be found in the GENBANK repository [https://www.ncbi.nlm.nih.gov/genbank/]. The raw gene expression and ELISA data supporting the conclusions of this manuscript will be made available by the authors, without undue reservation, to any qualified researcher.
HR conceived and designed the project; obtained funding for the project; acquired, analyzed and interpreted data; drafted the article; and approved the final submitted version of the article. AN obtained funding for the project, and both AN and AW contributed to the acquisition, analysis and interpretation of data; critically revised the article for important intellectual content; and approved the final submitted version of the manuscript. JS, SL, RS, and RP contributed to the acquisition, analysis and interpretation of the data; and revised and approved the final submitted version of the manuscript. SM provided statistical expertise for the analysis and interpretation of the data; critically revised the article for important intellectual content; and approved the final submitted version of the manuscript.
Supported by the Cornell University Harry M. Zweig Memorial Fund for Equine Research. The study sponsors were not involved in the study design, collection, analysis and interpretation of the data; in the writing of the manuscript; and in the decision to submit the manuscript for publication.
The authors declare that the research was conducted in the absence of any commercial or financial relationships that could be construed as a potential conflict of interest.
The authors thank John O'Donnell and Holger Sondermann for their technical assistance and use of FPLC equipment for the purification of equine recombinant galectins. Synovial fluid, synovial membrane, osteochondral tissues and associated phenotypic data for a subset of the naturally occurring OA cases were provided by the Cornell Veterinary Biobank, a resource built with the support of NIH grant R24 GM082910 and the Cornell University College of Veterinary Medicine.
The Supplementary Material for this article can be found online at: https://www.frontiersin.org/articles/10.3389/fvets.2018.00288/full#supplementary-material
1. Marsich E, Mozetic P, Ortolani F, Contin M, Marchini M, Vetere A, et al. Galectin-1 in cartilage: expression, influence on chondrocyte growth and interaction with ECM components. Matrix Biol. (2008) 27:513–25. doi: 10.1016/j.matbio.2008.04.003
2. Guévremont M, Martel-Pelletier J, Boileau C, Liu F-T, Richard M, Fernandes J-C, et al. Galectin-3 surface expression on human adult chondrocytes: a potential substrate for collagenase-3. Ann Rheum Dis. (2004) 63:636–43. doi: 10.1136/ard.2003.007229
3. Fowlis D, Colnot C, Ripoche MA, Poirier F. Galectin-3 is expressed in the notochord, developing bones, and skin of the postimplantation mouse embryo. Dev Dyn. (1995) 203:241–51. doi: 10.1002/aja.1002030211
4. Reesink HL, Sutton RM, Shurer CR, Peterson RP, Tan JS, Su J, et al. Galectin-1 and galectin-3 expression in equine mesenchymal stromal cells (MSCs), synovial fibroblasts and chondrocytes, and the effect of inflammation on MSC motility. Stem Cell Res Ther. (2017) 8:243. doi: 10.1186/s13287-017-0691-2
5. Colnot C, Sidhu SS, Balmain N, Poirier F. Uncoupling of chondrocyte death and vascular invasion in mouse galectin 3 null mutant bones. Dev Biol. (2001) 229:203–14. doi: 10.1006/dbio.2000.9933
6. Boileau C, Poirier F, Pelletier J-P, Guévremont M, Duval N, Martel-Pelletier J, et al. Intracellular localisation of galectin-3 has a protective role in chondrocyte survival. Ann Rheum Dis. (2008) 67:175–81. doi: 10.1136/ard.2006.066514
7. Ohshima S, Kuchen S, Seemayer CA, Kyburz D, Hirt A, Klinzing S, et al. Galectin 3 and its binding protein in rheumatoid arthritis. Arthritis Rheum. (2003) 48:2788–95. doi: 10.1002/art.11287
8. Harjacek M, Diaz-Cano S, De Miguel M, Wolfe H, Maldonado CA, Rabinovich GA. Expression of galectins-1 and-3 correlates with defective mononuclear cell apoptosis in patients with juvenile idiopathic arthritis. J Rheumatol. (2001) 28:1914–22.
9. Ezzat MHM, El-Gammasy TMA, Shaheen KYA, Osman AOY. Elevated production of galectin-3 is correlated with juvenile idiopathic arthritis disease activity, severity, and progression. Int J Rheum Dis. (2011) 14:345–52. doi: 10.1111/j.1756-185X.2011.01632.x
10. Filer A, Bik M, Parsonage GN, Fitton J, Trebilcock E, Howlett K, et al. Galectin 3 induces a distinctive pattern of cytokine and chemokine production in rheumatoid synovial fibroblasts via selective signaling pathways. Arthritis Rheum. (2009) 60:1604–14. doi: 10.1002/art.24574
11. Li X, Zhao Q-Y, Yuan F-L. Galectin 3 as a novel therapeutic target in rheumatoid arthritis: comment on the article by Forsman et al. Arthritis Rheum. (2011) 63:3179–80. doi: 10.1002/art.30532
12. Xie Q, Ni M, Wang S-C. Galectin-3, a potential therapeutic target for rheumatoid arthritis? Scand J Immunol. (2018) 87:108. doi: 10.1111/sji.12631
13. Xibillé-Friedmann D, Bustos Rivera-Bahena C, Rojas-Serrano J, Burgos-Vargas R, Montiel-Hernández J-L. A decrease in galectin-1 (Gal-1) levels correlates with an increase in anti-Gal-1 antibodies at the synovial level in patients with rheumatoid arthritis. Scand J Rheumatol. (2013) 42:102–7. doi: 10.3109/03009742.2012.725769
14. Iqbal AJ, Cooper D, Vugler A, Gittens BR, Moore A, Perretti M. Endogenous galectin-1 exerts tonic inhibition on experimental arthritis. J Immunol. (2013) 191:171–7. doi: 10.4049/jimmunol.1203291
15. Rabinovich GA, Daly G, Dreja H, Tailor H, Riera CM, Hirabayashi J, et al. Recombinant galectin-1 and its genetic delivery suppress collagen-induced arthritis via T cell apoptosis. J Exp Med. (1999) 190:385–98.
16. Wang C-R, Shiau A-L, Chen S-Y, Cheng Z-S, Li Y-T, Lee C-H, et al. Intra-articular lentivirus-mediated delivery of galectin-3 shRNA and galectin-1 gene ameliorates collagen-induced arthritis. Gene Ther. (2010) 17:1225–33. doi: 10.1038/gt.2010.78
17. Huang Y-J, Shiau A-L, Chen S-Y, Chen Y-L, Wang C-R, Tsai C-Y, et al. Multivalent structure of galectin-1-nanogold complex serves as potential therapeutics for rheumatoid arthritis by enhancing receptor clustering. Eur Cell Mater (2012) 23:170–81; discussion 181.
18. Forsman H, Islander U, Andréasson E, Andersson A, Onnheim K, Karlström A, et al. Galectin 3 aggravates joint inflammation and destruction in antigen-induced arthritis. Arthritis Rheum. (2011) 63:445–54. doi: 10.1002/art.30118
19. Janelle-Montcalm A, Boileau C, Poirier F, Pelletier J-P, Guévremont M, Duval N, et al. Extracellular localization of galectin-3 has a deleterious role in joint tissues. Arthritis Res Ther. (2007) 9:R20. doi: 10.1186/ar2130
20. Alturfan AA, Eralp L, Emekli N. Investigation of inflammatory and hemostatic parameters in female patients undergoing total knee arthroplasty surgery. Inflammation (2008) 31:414–21. doi: 10.1007/s10753-008-9093-z
21. Toegel S, Weinmann D, André S, Walzer SM, Bilban M, Schmidt S, et al. Galectin-1 couples glycobiology to inflammation in osteoarthritis through the activation of an NF-κB-regulated gene network. J Immunol. (2016) 196:1910–21. doi: 10.4049/jimmunol.1501165
22. Toegel S, Bieder D, André S, Altmann F, Walzer SM, Kaltner H, et al. Glycophenotyping of osteoarthritic cartilage and chondrocytes by RT-qPCR, mass spectrometry, histochemistry with plant/human lectins and lectin localization with a glycoprotein. Arthritis Res Ther. (2013) 15:R147. doi: 10.1186/ar4330
23. Toegel S, Bieder D, André S, Kayser K, Walzer SM, Hobusch G, et al. Human osteoarthritic knee cartilage: fingerprinting of adhesion/growth-regulatory galectins in vitro and in situ indicates differential upregulation in severe degeneration. Histochem Cell Biol. (2014) 142:373–88. doi: 10.1007/s00418-014-1234-x
24. Weinmann D, Schlangen K, André S, Schmidt S, Walzer SM, Kubista B, et al. Galectin-3 induces a pro-degradative/inflammatory gene signature in human chondrocytes, teaming up with galectin-1 in osteoarthritis pathogenesis. Sci Rep. (2016) 6:39112. doi: 10.1038/srep39112
25. Iacobini C, Blasetti Fantauzzi C, Bedini R, Pecci R, Bartolazzi A, et al. Galectin-3 is essential for proper bone cell differentiation and activity, bone remodeling and biomechanical competence in mice. Metabolism (2018) 83:149–58. doi: 10.1016/j.metabol.2018.02.001
26. McIlwraith C. Traumatic arthritis and posttraumatic osteoarthritis in the horse. In: McIlwraith C, Frisbie DD, Kawcak CE, van Weeren PR, editors. Joint Disease in the Horse. St. Louis: Elsevier (2015). p. 33–55.
27. McIlwraith CW, Frisbie DD, Kawcak CE. The horse as a model of naturally occurring osteoarthritis. Bone Joint Res. (2012) 1:297–309. doi: 10.1302/2046-3758.111.2000132
28. Kuyinu EL, Narayanan G, Nair LS, Laurencin CT. Animal models of osteoarthritis: classification, update, and measurement of outcomes. J Orthop Surg Res. (2016) 11:19. doi: 10.1186/s13018-016-0346-5
29. McIlwraith CW, Frisbie DD, Kawcak CE, Fuller CJ, Hurtig M, Cruz A. The OARSI histopathology initiative - recommendations for histological assessments of osteoarthritis in the horse. Osteoarthritis Cartil. (2010) 18(Suppl 3):S93–105. doi: 10.1016/j.joca.2010.05.031
30. Frisbie DDD, Al-Sobayil F, Billinghurst RCC, Kawcak CEE, McIlwraith CWW. Changes in synovial fluid and serum biomarkers with exercise and early osteoarthritis in horses. Osteoarthritis Cartil. (2008) 16:1196–204. doi: 10.1016/j.joca.2008.03.008
31. Frisbie DDD, McIlwraith CWW, Kawcak CEE, Werpy NMM. Evaluation of intra-articular hyaluronan, sodium chondroitin sulfate and N-acetyl-D-glucosamine combination versus saline (0.9% NaCl) for osteoarthritis using an equine model. Vet J. (2013) 197:824–9. doi: 10.1016/j.tvjl.2013.05.033
32. Reesink HL, Watts AE, Mohammed HO, Jay GD, Nixon AJ. Lubricin/proteoglycan 4 increases in both experimental and naturally occurring equine osteoarthritis. Osteoarthr Cartil. (2017) 25:128–137. doi: 10.1016/j.joca.2016.07.021
33. Kamm JL, Nixon AJ, Witte TH. Cytokine and catabolic enzyme expression in synovium, synovial fluid and articular cartilage of naturally osteoarthritic equine carpi. Equine Vet J. (2010) 42:693–9. doi: 10.1111/j.2042-3306.2010.00140.x
34. Reesink HL, Bonnevie ED, Liu S, Shurer CR, Hollander MJ, Bonassar LJ, et al. Galectin-3 binds to lubricin and reinforces the lubricating boundary layer of articular cartilage. Sci Rep. (2016) 6:25463. doi: 10.1038/srep25463
35. Weinmann D, Kenn M, Schmidt S, Schmidt K, Walzer SM, Kubista B, et al. Galectin-8 induces functional disease markers in human osteoarthritis and cooperates with galectins-1 and-3. Cell Mol Life Sci. (2018) 75:4187–205. doi: 10.1007/s00018-018-2856-2
36. Sellam J, Berenbaum F. The role of synovitis in pathophysiology and clinical symptoms of osteoarthritis. Nat Rev Rheumatol (2010) 6: doi: 10.1038/nrrheum.2010.159
37. Wang X, Hunter DJ, Jin X, Ding C. The importance of synovial inflammation in osteoarthritis: current evidence from imaging assessments and clinical trials. Osteoarthr Cartil. (2018) 26:165–74. doi: 10.1016/j.joca.2017.11.015
38. Neidhart M, Rethage J, Kuchen S, Künzler P, Crowl RM, Billingham ME, et al. Retrotransposable L1 elements expressed in rheumatoid arthritis synovial tissue: association with genomic DNA hypomethylation and influence on gene expression. Arthritis Rheum. (2000) 43:2634–47. doi: 10.1002/1529-0131(200012)43:12<2634::AID-ANR3>3.0.CO;2-1
39. Issa SF, Christensen AF, Lindegaard HM, Hetland ML, Hørslev-Petersen K, Stengaard-Pedersen K, et al. Galectin-3 is persistently increased in early rheumatoid arthritis (RA) and associates with anti-CCP seropositivity and MRI bone lesions, while early fibrosis markers correlate with disease activity. Scand J Immunol. (2017) 86:471–8. doi: 10.1111/sji.12619
40. Chu CR, Szczodry M, Bruno S. Animal models for cartilage regeneration and repair. Tissue Eng Part B Rev. (2010) 16:105–15. doi: 10.1089/ten.TEB.2009.0452
41. Li S, Yu Y, Koehn CD, Zhang Z, Su K. Galectins in the pathogenesis of rheumatoid arthritis. J Clin Cell Immunol. (2013) 4:164. doi: 10.4172/2155-9899.1000164
42. Smetana K, André S, Kaltner H, Kopitz J, Gabius H-J. Context-dependent multifunctionality of galectin-1: a challenge for defining the lectin as therapeutic target. Expert Opin Ther Targets (2013) 17:379–92. doi: 10.1517/14728222.2013.750651
43. Yang R-Y, Rabinovich GA, Liu F-T. Galectins: structure, function and therapeutic potential. Expert Rev Mol Med. (2008) 10:e17. doi: 10.1017/S1462399408000719
Keywords: inflammatory arthritis, cartilage, synovium, chondrocyte, synoviocyte, horse, rheumatoid arthritis
Citation: Reesink HL, Nixon AJ, Su J, Liu S, Sutton RM, Mann S, Watts AE and Peterson RP (2018) Galectins-1 and-3 Increase in Equine Post-traumatic Osteoarthritis. Front. Vet. Sci. 5:288. doi: 10.3389/fvets.2018.00288
Received: 14 August 2018; Accepted: 30 October 2018;
Published: 20 November 2018.
Edited by:
Troy N. Trumble, University of Minnesota Twin Cities, United StatesReviewed by:
Emma N. Adam, University of Kentucky, United StatesCopyright © 2018 Reesink, Nixon, Su, Liu, Sutton, Mann, Watts and Peterson. This is an open-access article distributed under the terms of the Creative Commons Attribution License (CC BY). The use, distribution or reproduction in other forums is permitted, provided the original author(s) and the copyright owner(s) are credited and that the original publication in this journal is cited, in accordance with accepted academic practice. No use, distribution or reproduction is permitted which does not comply with these terms.
*Correspondence: Heidi L. Reesink, aGxyNDJAY29ybmVsbC5lZHU=
†Present Address: Sherry Liu, Department of Bioengineering, University of Washington, Seattle, WA, United States
Ryan M. Sutton, Sidney Kimmel Medical College, Thomas Jefferson University, Philadelphia, PA, United States
Ashlee E. Watts, Department of Large Animal Clinical Sciences, Texas A&M University, College Station, TX, United States
Disclaimer: All claims expressed in this article are solely those of the authors and do not necessarily represent those of their affiliated organizations, or those of the publisher, the editors and the reviewers. Any product that may be evaluated in this article or claim that may be made by its manufacturer is not guaranteed or endorsed by the publisher.
Research integrity at Frontiers
Learn more about the work of our research integrity team to safeguard the quality of each article we publish.