- Coordinación de Sistemas Ganaderos, Instituto de Investigaciones Agropecuarias INIA Carillanca, Temuco, Chile
Methane (CH4) formed in the rumen and released to the atmosphere constitutes an energy inefficiency to ruminant production. Redirecting energy in CH4 to fermentation products with a nutritional value to the host animal could increase ruminant productivity and stimulate the adoption of CH4-suppressing strategies. The hypothesis of this research was that inhibiting CH4 formation in the rumen is associated with greater ruminant productivity. The primary objective of this meta-analysis was to evaluate how inhibiting rumen methanogenesis relates with the efficiencies of milk production and growth and fattening. A systematic review of peer-reviewed studies in which rumen methanogenesis was inhibited with chemical compounds was conducted. Experiments were clustered based on research center, year of publication, experimental design, feeding regime, type of animal, production response, inhibitor of CH4 production, and method of CH4 measurement. Response variables were regressed against the random experiment effect nested in its cluster, the random effect of the cluster, the linear and quadratic effects of CH4 production, and the random interaction between CH4 production and the experiment nested in the cluster. When applicable, responses were adjusted by intake of different nutrients included as regressors. Inhibiting rumen methanogenesis tended to associate positively with milk production efficiency, although the relationship was influenced by individual experiments. Likewise, a positive relationship between methanogenesis inhibition and growth and fattening efficiency depended on the inclusion and weighting of individual experiments. Inhibiting rumen methanogenesis negatively associated with dry matter intake. Interpretation of the effects of inhibiting methanogenesis on productivity is limited by the availability of experiments simultaneously reporting energy losses in feces, H2, urine and heat production, as well as net energy partition. It is concluded that inhibiting rumen methanogenesis has not consistently translated into greater animal productivity, and more animal performance experiments are necessary to better characterize the relationships between animal productivity and methanogenesis inhibition in the rumen. A more complete understanding of changes in the flows of nutrients caused by inhibiting rumen methanogenesis and their effect on intake also seems necessary to effectively re-channel energy gained from CH4 suppression toward consistent gains in productivity.
Introduction
Ruminants are important to humans in converting non-usable forages to products such as meat, milk, wool and traction. The mixed rumen microbiota has the ability to digest plant fiber unavailable for humans and produce fermentation products and microbial biomass that the host animal absorbs and converts to products useful for humans. Methane (CH4) is the main sink of metabolic hydrogen ([H]) in rumen fermentation. Metabolism of carbohydrates by the fermentative microbiota of bacteria, protozoa and fungi reduces co-factors, which are re-oxidized mostly by transferring electrons to protons. Dihydrogen (H2) so formed is transferred to methanogenic Archaea, which utilize it to reduce carbon dioxide (CO2) to CH4 (1).
In recent years, there has been considerable research efforts to control the formation of CH4 in the rumen, with the objective of ameliorating CH4 emissions from domestic ruminants. Agriculture accounts for between 10 and 12% of global emissions of greenhouse gases expressed as CO2-equivalents, with the largest contributor being enteric CH4 (2). Because CH4 has a global warming potential 28- to 34-fold greater than CO2, decreases in anthropogenic CH4 emissions are a strategic target for ameliorating climate change (3).
In addition, CH4 emissions represent an energy loss to the animal of between 2 and 12% of gross energy intake (GEI) of ruminants (4). Historically, research on the inhibition of rumen methanogenesis started driven by scientists identifying the formation of CH4 in the rumen as an energy inefficiency: “It is argued that if the methane production could be inhibited specifically, rumen fermentation might change toward greater efficiency. Methane is produced from carbon dioxide and metabolic hydrogen and the manipulation could be effective if the hydrogen saved from methanogenesis could be used in formation of products that might subsequently be used by the host animal” (5). Redirecting [H] from CH4 toward propionate has been proposed as a means to increase the amount of ME available to the animal (6). Energy lost as CH4 is an inefficiency in the conversion of digestible energy (DE) to metabolizable energy (ME), because CH4 is formed from organic matter (OM) digested and fermented in the rumen and hindgut (7).
Unless in the future CH4 mitigation strategies become mandatory or stimulated by government subsidies, it seems unlikely that they will be widely implemented by producers if their adoption is not profitable (8). Thus, if energy lost as CH4 could be capitalized by incorporating it into products that the host animal can absorb and use, producers would more likely adopt strategies to ameliorate CH4 emissions. Gains in productivity are therefore considered critical, and an opportunity, for the design of CH4 mitigation strategies that are at the same time economically beneficial to producers.
Meta-analyses relating the dietary content of fats and oils (9–11), monensin (12), nitrate (13), and 3-nitroxypropanol (14) to CH4 emissions and animal performance, digestion and metabolism, have generated useful applied knowledge about the effectiveness of those ingredients and additives for CH4 mitigation. Apart from dose response analyses, the overall biological response of animal productivity to the methanogenesis inhibition intervention has to the author's knowledge yet to be studied. Patra (15) meta-analyzed the effects of inhibiting CH4 production with phytochemicals on digestion and fermentation. The present analysis evaluates the effects on ruminant productivity and energy partition of decreasing CH4 production in the rumen.
Various strategies are being investigated to control CH4 production by ruminants. Some CH4-abattement strategies, such as dietary changes or selection of more efficient animals, may also impact productivity through means unrelated to methanogenesis inhibition; experiments using these CH4-abattement strategies would thus not be suitable to examine the effects of inhibiting rumen methanogenesis on animal productivity in isolation. In order to understand the effects of decreasing CH4 production in the rumen on ruminant productivity unmasked by other factors, the present analysis focuses only on experiments in which methanogenesis was specifically targeted using chemical inhibitors. It was hypothesized herein that inhibiting CH4 production with chemical compounds enhances animal productivity through improving energy use efficiency. The objectives of this meta-analysis were: (1) To examine using meta-analysis of published results if inhibiting rumen methanogenesis with specific chemical compounds has associated with improvements in the efficiencies of milk production and growth and fattening; (2) To interpret the relationships between rumen methanogenesis inhibition and the efficiencies of milk production and growth and fattening by examining how inhibiting rumen methanogenesis has associated with energy losses in digestion and metabolism.
Methods
Search Criteria
Peer-reviewed publications published in English reporting original research on the inhibition of rumen methanogenesis in vivo using chemical compounds were searched in PubMed (https://www.ncbi.nlm.nih.gov/pubmed/), Web of Science (https://apps.webofknowledge.com/WOS_GeneralSearch_input.do?product=WOS&search_mode=GeneralSearch&SID=5C93Gu6M6z6HUIaDDxS&preferencesSaved=) and Agricola (https://agricola.nal.usda.gov/). Articles were searched in the databases based on the following keywords present in their title or abstract or keywords using the following Boolean operation: (rumen OR ruminant OR ruminants OR dairy OR beef OR sheep OR goats OR buffaloes) AND methane AND inhibition. Also, articles on the inhibition of rumen methanogenesis were obtained from the MitiGate database (2) and the author's personal files. A total of 89, 280, 121, and 333 records were retrieved from PubMed, Web of Science, Agricola and MitiGate, respectively, many of which were present in more than one databases.
Study Eligibility Criteria
Most of the records retrieved referred to in vitro experiments and were not used in the analysis. Of the in vivo studies, only those ones reporting experiments in which rumen methanogenesis was inhibited through the use of specific chemical inhibitors were used to study how inhibiting rumen methanogenesis affected ruminant productivity. Use of chemical additives with known composition and included in relatively small amounts in the diet is thought to be the most likely CH4 amelioration intervention which could affect animal productivity solely through inhibiting methanogenesis, although it is acknowledged that some antimethanogenic chemicals can be toxic to microorganisms other than methanogens and might therefore cause other effects. Other approaches to ameliorate CH4 emissions are less specific and might affect animal productivity through means unrelated to methanogenesis inhibition: dietary manipulation (augmented supply of nutrients or improved nutrient balance), ionophores [improved N utilization efficiency (16)], essential oils [decreased protein and starch degradation (17)], lipid supplementation [enhanced energy supply vs. lesser intake, fiber digestibility and inhibition of de novo milk fatty acids synthesis (10, 11)], defaunation [increased microbial protein production; (18)], tannins and saponins [improved supply of protein digested in the small intestine; (19)], alternative [H] sinks [provision of extra fermentable energy; (20)], and enzyme supplementation [improved fiber digestibility; (11)].
Selecting animals with better feed conversion efficiency has resulted in animals with lower CH4 production (21). The results from the study by Fitzsimons et al. (21) were not included in the present meta-analysis however, because the approach was reversal in the sense that less CH4 production was a consequence of greater productivity, rather than enhanced productivity a consequence of methanogenesis inhibition.
Immunization against methanogens is being studied as a potential anti-methanogenic strategy (22), but results on the effects of immunization against methanogens on milk production or bodymass change, i.e., animal productivity were not found in the present literature search.
Experiments in which nitrate replaced urea as a source of non-protein N were included in the analysis, with the understanding that neither urea or nitrate would contribute dietary gross energy (GE) available to the ruminant, or nutrients other than N. In experiments in which nitrate was used as an inhibitor of CH4 production, the diet composition was carefully checked to ensure that either analyzed organic matter (OM) or GE did not differ by more than 2%, or that, if analyzed dietary OM or GE content were not provided, that nitrate did not replace dietary true protein.
Database
After discarding studies examining strategies to ameliorate CH4 production by ruminants other than chemical inhibitors, a total of 75 studies including 96 experiments in which rumen methanogenesis in vivo was inhibited with chemical additives was obtained. Of these, 44 studies (23–66) with 54 experiments including a total of 163 treatments were used as the final database for the meta-regressions (Table S1), and 42 experiments belonging to 31 studies were discarded because of different reasons detailed in Table S2.
Response variables analyzed were:
1) Milk production efficiency (10 experiments, 26 treatment means), defined as energy-corrected milk (ECM) production adjusted by dry matter intake (DMI). When not provided, ECM production was calculated from milk production (kg) and milk content of fat, protein and lactose (67);
2) Growth and fattening efficiency (13 experiments, 38 treatment means) defined as bodymass gain (BMG) adjusted by DMI;
3) Digestion and metabolism variables: DMI (ad libitum intake experiments only). Feces output of DM (DMf), OM (OMf), N (Nf), and NDF (NDFf) adjusted by intake of DM, OM, N, and NDF, respectively. Energy losses in feces (EF), gases (EG; CH4 + H2), urine (UE), and heat (HP), all adjusted by GEI. Rumen pH, total rumen volatile fatty acids (VFA) concentration, individual VFA molar percentages, rumen ammonium concentration, and total bacteria, protozoa and methanogens 16S rRNA, 18S rRNA, and 16S rRNA or mcrA gene copies, respectively (log10/mL rumen contents)
Clusters
In meta-analysis, estimates of effect sizes belonging to different experiments are not independent due to the fact that experiments differ to different degrees in various aspects, such as research methods employed, research group, animals, location etc. (68). The approach taken herein to account for lack of independence was to model dependence by introducing clusters of experiments in the meta-regressions (69).
Experiments were grouped for each response variable according to clustering variables related to experimental variables detailed in Table S3: research center, year of publication, experimental design, feeding regime, type of animal, production response, inhibitor of CH4 production and method of CH4 measurement. Hierarchical clusters were built for each response variable using the Ward method with standardized data with JMP 13.2.1.
Regressions
Response variables were meta-regressed against the random effect of the experiment (70) nested in the cluster, the linear and quadratic effects of CH4 production, and the random linear interaction between the experiment and CH4 production nested in the cluster. The general model was:
Where Yijk is the treatment mean k of a response variable of interest of the experiment i nested in cluster j, μ is the overall intercept, exp(random, cluster)ij is the random effect of experiment i nested in cluster j, cluster(random)j is the random effect of the cluster j, β1 and β2 are the overall linear and quadratic regression coefficients of CH4 production on Y, respectively, CH4ijk and ijk are the treatment mean and treatment mean squared of CH4 production of treatment k of experiment i nested in cluster j, respectively, E is the random effect of the experiment i nested in cluster j on β1, and resijk is the residual of treatment mean k of experiment i nested in cluster j, assumed to be normally distributed with mean equal to 0 and variance σ.
When production of gases (CH4 or H2) was reported as grams per day, it was converted to liters per day using the molar mass of each gas and the General Law of Gases assuming an absolute temperature of 298 K.
Milk and growth and fattening production efficiencies can be evaluated as the quotient of ECM production or BMG, respectively, to DMI. However, the use of ratios as response variables can be problematic because of correlations between the regressors and the numerator or denominator variable in the ratio (71), with, in the present analysis, CH4 production being largely driven by DMI (72). Therefore, the approach taken for modeling milk and growth and fattening production efficiencies was to regress daily ECM production and BMG against CH4 production adjusted for DMI, as follows:
Where Yikj is the treatment mean k of ECM or BMG of the experiment i nested in cluster j, β0 is the overall linear regression coefficient of DMI, DMIijk is the DMI of treatment k in experiment i of cluster j, with the rest of the variables and parameters in model (2) defined as in model (1).
Similarly, the effects of inhibiting rumen methanogenesis on digestibility of DM, OM, N, and NDF were studied by regressing the fecal daily outputs of dry matter (DMf), organic matter (OMf), nitrogen (Nf), or NDF (NDFf) adjusted by their daily intakes against CH4 production as follows:
Where Yikj is the treatment mean k of DMf, OMf, Nf, or NDFf in experiment i nested in cluster j, β0 is the overall regression coefficient of DMI, organic matter intake (OMI), nitrogen intake (NI), or NDF intake (NDFI), respectively, Xijk is the intake of DM, OM, N, or NDF of treatment k in experiment i of cluster j, respectively, with the rest of the variables and parameters in model (3) defined as in models (1) and (2).
Likewise, responses of energy losses in feces (EF), total gases (CH4 + H2, EG), urine (UE), and heat (HP) were adjusted by GEI included as a regressor:
Where Yijk corresponds to EF, EG, UE or HP of treatment k in experiment i nested in cluster j, β0 is the overall regression coefficient of GEI, GEIijk is the mean of GEI of treatment k in experiment i nested in cluster j, with the rest of the variables and parameters in model (4) defined as in models (1), (2), and (3). Energy in CH4, feces, total gases, urine and heat were expressed in MJ/d. Heats of combustion were obtained from Domalski (73) and McAllister et al. (74).
Fixed effects with P < 0.05 were considered significant, and those with 0.05 ≤ P ≤ 0.10 were considered tendencies. Random interactions experiment by CH4 nested in the cluster with Wald P < 0.05 were considered significant, and those with 0.05 ≤ P ≤ 0.10 were considered tendencies. Quadratic and interaction effects with P > 0.10 were eliminated and the reduced model re-fitted.
In meta-regressions, it is recommended to weight treatment means by the reciprocal of their standard errors (SEM) normalized to unity (75). However, difficulties encountered for conducting this procedure were: (1) Treatment means of ECM were calculated as compound variables from reported milk production and composition, and their SEM is not calculable; (2) SEM are not provided in some of the older studies (24, 27, 29). An alternative weighting scheme, such as the number of animals in the experiment, could not be used because the present analysis included experiments with both randomized plots and blocks, and Latin Squares and cross over designs, the latter obviously including fewer animals. Therefore, regressions were conducted in first instance with unweighted treatment means. As a precaution against the possibility of experiments with few animals or high experimental error having excessive influence in the results, special attention was paid to experiments not reporting SEM when examining the results for the presence of outliers and influential observations (see Analysis of outliers and influential treatment means).
As a second precaution against some experiments having excessive influence on the regressions, SEM of ECM were estimated as linear combinations of the SEM of milk production and composition, acknowledging that this proxy is inaccurate. Subsequently, regressions of ECM and BMG against CH4 production were re-run as above described but with treatment means weighted by the reciprocal of their estimated SEM normalized to unity, and compared to regressions with unweighted treatment means conducted on the same sub-set of experiments.
Analysis of Experimental Co-variables
The potential influence of various experimental co-variables on the response of ECM, BMG and DMI to methanogenesis inhibition was examined by replacing in the regressions the experiment and cluster effects by different co-variables related to the experiment fitted separately, as follows:
Where Yk is the kth treatment mean of the response variable across the entire database, μ is the overall intercept, β0 is the regression coefficient of the intake of the fraction corresponding to the response variable (i.e., intake of DM, OM, N, NDF, or energy), Vk is the DM, OM, N, NDF or energy intake of treatment k across the entire database, β1 and β2 are the linear and quadratic regression coefficients of CH4 production on Y, respectively, CH4k and k are the treatment mean and treatment mean squared of the overall k level of CH4 production, β3 is the regression coefficient of experimental co-variable Z, Zk is the treatment mean of the overall k level of Z, β4 is the regression coefficient of the interaction between CH4k and Zk, and resk is the residual of the overall k level of CH4 production and Z, assumed to be normally distributed with mean equal to 0 and variance σ.
The interaction effect between methanogenesis inhibition and classification type of co-variables on each response variable was studied only for co-variables in which each level of the co-variable was represented in at least two experiments.
Co-variables analyzed were: type of animal (dairy cows, goats, growing heifers, steers, or sheep), feeding regime (restricted or ad libitum; except for DMI), stage of lactation at the beginning of the experiment (for ECM only), type of experimental design (fixed assignment of animals to treatments or treatment switch), type of response (maintenance, milk production or growth and fattening), type of methanogenesis inhibitor, and content of dietary concentrate, N and NDF (DM basis).
Analysis of Outliers and Influential Treatment Means
Homoscedasticity was examined through residual against predicted plots. The assumption of residuals normality was examined through residual normality plots. Outliers were identified as those treatment means whose absolute value studentized residuals were greater than tN−p−1, 0.95, with p being the number of parameters and N the number of treatment means. Influential treatment means were identified as those with a leverage value larger than 2p/N (76). Experiments containing outliers and/or influential treatment means were deleted one at a time and regressions re-fitted in their absence. If the conclusions of the analysis changed after the deletion of experiments containing outliers and/or influential treatment means (significant effects became non-significant or vice versa, or the direction of the response changed), the results are presented and discussed both with and without the experiments containing the outliers and/or influential observations.
JMP® 13.2.1 (SAS Institute, Cary, NC, USA) was used for all statistical analyses.
Results
Descriptive Statistics, Clusters of Experiments, and Methanogenesis Inhibition
A summary of statistics of regressors and response variables is presented in Table 1. The clustering details for the main response variables are presented in Table S4.
Conceptually, the degree of methanogenesis inhibition is the reverse of CH4 production i.e., the greater methanogenesis inhibition the lesser CH4 production. Therefore and because of the main objective of this analysis was to understand the how the intervention of inhibiting rumen methanogenesis associates with animal productivity, results are presented and discussed in terms of the relationships between methanogenesis inhibition and the different response variables.
Milk Production Efficiency
There was no relationship between inhibiting methanogenesis and DMI-adjusted ECM production (P = 0.57; Figure 1 i). If the regression was weighted by the estimated reciprocal of the treatment means SEM normalized to unity, there was a tendency (P = 0.084) toward a positive association between DMI-adjusted ECM production and methanogenesis inhibition (Figure 1 ii). A sensitivity analysis found that this tendency became a significant positive association (P = 0.007; not shown) if eliminating the experiment by van Zijderveld et al. (40), and was not significant (P = 0.67; not shown) if excluding the first experiment by Veneman et al. (59).
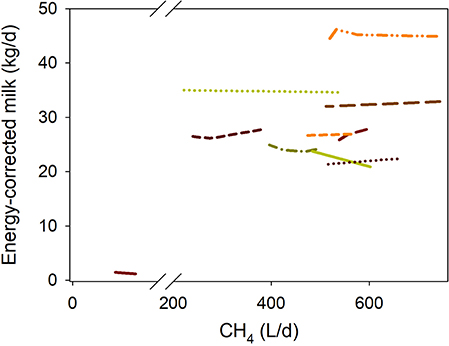
Figure 1. Response of energy-corrected milk (ECM, kg/d) production adjusted by dry mater intake (DMI, kg/d)] to methanogenesis inhibition, including the random effect of the experiment nested in the cluster, and the random effect of the cluster. Each separate line corresponds to a different experiment: (i) Unweighted treatment means: ECM = −0.46 (±3.22; P = 0.89) + 1.50 (±0.18; P < 0.001) DMI – 0.0014 (±0.0023; F = 0.34, P = 0.57) CH4; N = 26, 10 experiments; (ii) Weighted treatment means: ECM = −1.17 (±2.61; P = 0.67) + 1.67 (±0.16; P < 0.001) DMI – 0.0067 (±0.0037; F = 3.33, P = 0.084) CH4; N = 26, 10 experiments.
There were no interactions between methanogenesis inhibition and type of animal (P = 0.97), experimental design (P = 0.49), feeding regime (P = 0.67), dietary N (P = 0.86) or NDF (P = 0.28), or type of methanogenesis inhibitor (P = 0.77) on DMI-adjusted ECM. There was a tendency (P = 0.091) toward a positive association between DMI-adjusted ECM and methanogenesis inhibition with greater dietary concentrate (not shown).
Growth and Fattening Efficiency
Inhibiting CH4 production associated positively with DMI-adjusted BMG (P = 0.003; Figure 2 i). If the regression was weighted by the reciprocal of the treatment means SEM normalized to unity, there was no relationship between CH4 production and DMI-adjusted BMG (P = 0.27; Figure 2 ii). If the regression was conducted in the same sub-set of experiments for which SEM were available [i.e., excluding the experiments by Davies et al. (29) and Tomkins et al. (36), but without weighting the treatment means, inhibiting CH4 production associated positively with DMI-adjusted BMG (P = 0.013; Figure 2 iii); in the latter case, a sensitivity analysis found no relationship (P = 0.25) between CH4 production and DMI-adjusted BMG if the experiment by McCrabb et al. (31) was excluded from the analysis.
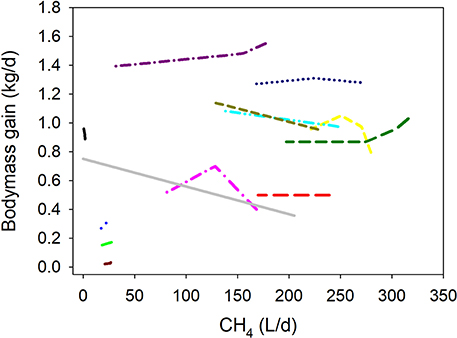
Figure 2. Response of bodymass gain (BMG, kg/d) adjusted by dry matter intake (DMI, kg/d) to methanogenesis inhibition, including the random effect of the experiment nested in the cluster, and the random effect of the cluster. Each separate line corresponds to a different experiment: (i) Unweighted treatment means (all experiments): BMG = 0.026 (±0.14; P = 0.86) + 0.13 (±0.021; P < 0.001) DMI – 0.0010 (±0.00032; F = 10.8, P = 0.003) CH4; N = 38, 13 experiments; (ii) Weighted treatment means: BMG = 0.036 (±0.15; P = 0.81) + 0.11 (±0.023; P < 0.001) DMI – 0.00038 (±0.00034; F = 1.29, P = 0.27) CH4; N = 32, 11 experiments; iii) Unweighted treatment means: BMG = 0.0071 (±0.16; P = 0.96) + 0.13 (±0.023; P < 0.001) DMI – 0.00096 (±0.00036; F = 7.18, P = 0.013) CH4; N = 32, 11 experiments.
There were no interactions between methanogenesis inhibition and type of animal (P = 0.93), experimental design (P = 0.24), feeding regime (P = 0.31), dietary concentrate (P = 0.84), dietary N (P = 0.69), or NDF (P = 0.97), or type of methanogenesis inhibitor (P > 0.75) on DMI-adjusted BMG (not shown).
Dry Matter Intake and Digestibility
Inhibiting methanogenesis associated with decreased DMI (P < 0.001; Figure 3). The predicted DMI at a theoretical 100% methanogenesis inhibition was 10% lower than the mean of control treatments (not shown). There were no interactions between methanogenesis inhibition and type of animal (P = 0.99), production response (P = 0.25), and dietary content of concentrate (P = 0.26), or N (P = 0.50). There was a tendency (P = 0.051) toward a more negative relationship between DMI and methanogenesis inhibition in experiments with a randomized design than in those in which animals were switched between diets, and with lesser content of dietary NDF (P < 0.001) (not shown).
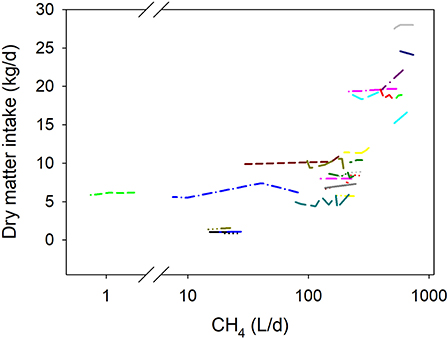
Figure 3. Response of dry matter intake (DMI, kg/d) to methanogenesis inhibition, including the random effect of the experiment nested in the cluster and the random effect of the cluster. Each separate line corresponds to a different experiment: DMI = 9.99 (±2.08; P = 0.001) + 0.0036 (±0.00099; F = 13.4, P < 0.001) CH4; N = 83, 25 experiments.
There were no relationship between methanogenesis inhibition and DMI-adjusted DMf (P = 0.72; Table 2). A sensitivity analysis found a tendency (P = 0.086) toward greater DMI-adjusted DMf with methanogenesis inhibition if the experiment by Reynolds et al. (52) was removed (not shown). There were no relationships between inhibiting CH4 production and OMI-adjusted OMf (P = 0.84), NI-adjusted Nf (P = 0.58), or NDFI-adjusted NDFf (P = 0.83; Table 2).
Energy Losses in Feces, Gases, Urine, and Heat
There was a quadratic (P < 0.001) negative association between methanogenesis inhibition and energy output in feces adjusted by GEI (Figure 4). Inhibition of rumen methanogenesis negatively associated with total energy losses in gases (CH4 plus H2) (P < 0.001; Figure 5). For each MJ saved in CH4, there was a numerical increase of 0.027 MJ in energy losses as H2 (P = 0.13; not shown). There were no relationships between inhibition of rumen methanogenesis and energy losses in urine (P = 0.55; Figure 6) or heat (P = 0.33; Figure 7).
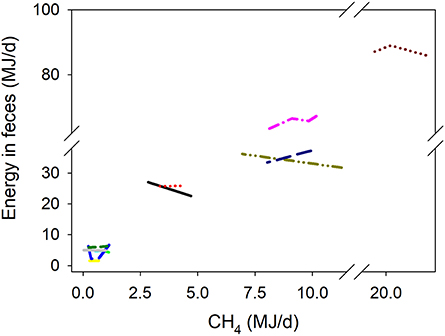
Figure 4. Response of energy losses in feces (EF, MJ/d) adjusted by gross energy intake (GEI, MJ/d) to methanogenesis inhibition, including the random effect of the experiment nested in the cluster, and the random effect of the cluster. Each separate line corresponds to a different experiment: EF = 0.24 (±1.81; P = 0.90) + 0.34 (±0.019; P < 0.001) GEI – 0.34 (±0.36; F = 0.48, P = 0.35) CH4 – 0.088 (±0.018; F = 15.8, P < 0.001) (CH4 – 5.12)2; N = 31, 12 experiments.
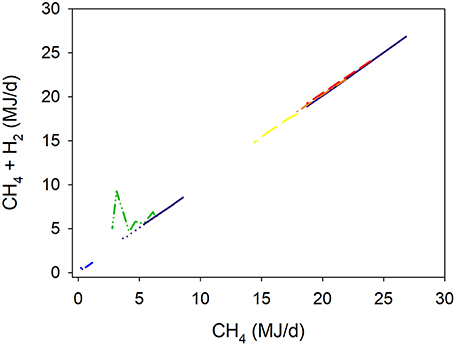
Figure 5. Response of energy losses in gases (CH4 + H2, EG, MJ/d) adjusted by gross energy intake (GEI, MJ/d) to methanogenesis inhibition, including the random effect of the experiment nested in the cluster, and the random effect of the cluster. Each separate line corresponds to a different experiment: EG = 0.85 (±0.64; P = 0.28) + 0.0013 (±0.0030; P = 0.67) GEI + 0.92 (±0.064; F = 208, P < 0.001) CH4; N = 38, 9 experiments.
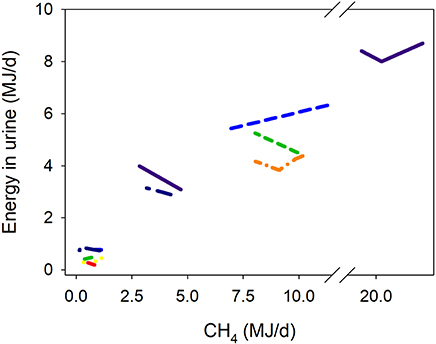
Figure 6. Response of energy losses in urine (UE, MJ/d) adjusted by gross energy intake (GEI, MJ/d) to methanogenesis inhibition, including the random effect of the experiment nested in the cluster, and the random effect of the cluster. Each separate line corresponds to a different experiment: UE = 1.02 (±0.77; P = 0.29) + 0.018 (±0.0049; P < 0.001) GEI – 0.042 (±0.070; F = 0.37, P = 0.55) CH4; N = 29, 11 experiments.
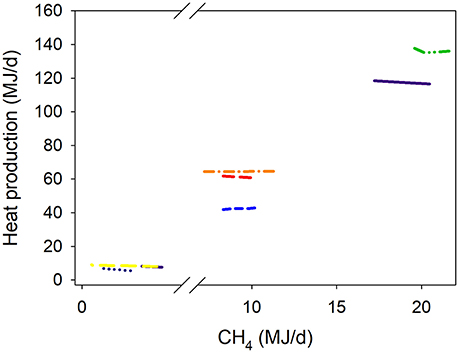
Figure 7. Response of heat production (HP, MJ/d) adjusted by gross energy intake (GEI, MJ/d) to methanogenesis inhibition, including the random effect of the experiment nested in the cluster, and the random effect of the cluster. Each separate line corresponds to a different experiment: HP = 2.27 (±11.6; P = 0.86) + 0.36 (±0.042; P < 0.001) GEI – 0.011 (±0.011; F = 1.04, P = 0.33) CH4; N = 21, 8 experiments.
Rumen Variables
Inhibiting rumen methanogenesis was positively associated with rumen pH (P = 0.023), and had a negative, quadratic (P = 0.056) relationship with total VFA concentration (P = 0.002; Table 3). Acetate concentration associated negatively with methanogenesis inhibition (P < 0.001; Table 3).
There were no relationships between the inhibition of rumen methanogenesis and the concentration of propionate (P = 0.53), butyrate (P = 0.34), and isobutyrate (P = 0.43; Table 3). There was a positive relationship between the inhibition of rumen methanogenesis and valerate concentration (P = 0.048; Table 3). A sensitivity analysis found no relationship between methanogenesis inhibition and valerate concentration if eliminating the experiments by El-Zaiat et al. (47) (P = 0.34) or Haisan et al. (48) (P = 0.14; not shown). Inhibiting methanogenesis associated with increased concentration of isovalerate (P = 0.013; Table 3). There was no relationship between methanogenesis inhibition and rumen ammonium concentration (P = 0.11; Table 3).
There was no relationship between inhibition of rumen methanogenesis and total bacterial 16S rRNA gene copies (P = 0.77) or total protozoal 18S rRNA gene copies (P = 0.42; Table 3). There was a negative relationship between methanogenic Archaea 16S rRNA and mcrA gene copies and methanogenesis inhibition (P = 0.009; Table 3).
Discussion
Animal Performance
Inhibiting rumen methanogenesis saves energy otherwise lost as CH4 and would theoretically result in greater efficiency of energy utilization. In the present analysis, inhibiting rumen methanogenesis tended to positively associate with improved milk production efficiency when treatment means were weighted by the reciprocal of their SEM. The fact that this tendency found could change toward significance or non-significance by omitting or weighting particular experiments indicates the need for more experiments to obtain more consistent conclusions.
The expected ECM production adjusted by DMI at a theoretical 100% inhibition of methanogenesis would be 3.56 ± 1.97 kg/d greater than the mean of the methanogenesis-uninhibited control treatments, representing 11.2 ± 6.19 MJ. The average CH4 production of the control treatments of the ECM analysis was of 532 L/d, equivalent to 19.4 MJ, which would be saved at a theoretical 100% methanogenesis. At the same time, the predicted energy losses as H2 at 100% methanogenesis inhibition would have augmented by 1.27 MJ/d, resulting in about 18.1 MJ/d of energy saved in gases. Therefore, the energy saved in gases not produced would be transferred to milk with an efficiency of approximately 62% (calculations not shown). Energy saved in gases becomes metabolizable energy (ME). Conversion of ME to net energy (NE) has been reported to be of 64% (77), which compares well with the 62% figure obtained herein.
Similarly to milk production efficiency, benefits of inhibiting rumen methanogenesis on growth and fattening efficiency also depended on whether treatment means were weighted by the reciprocal of their SEM, with the added complexity that SEM were available for 11 out of the 13 DMI-adjusted BMG experiments. Thus, part of the discrepancy between the analyses with weighted or non-weighted treatment means is due to the omission of the experiments by Davies et al. (29) and Tomkins et al. (36) with weighted treatment means. Again, the influence of the presence and weight of individual experiments on the results also indicates the need for more research on the response of growth and fattening to the inhibition of rumen methanogenesis.
Inhibition of CH4 production in the rumen cannot be considered as an isolated intervention and can potentially have consequences both on products of rumen fermentation other than CH4 and on post-absorptive metabolism. The discussion that follows examines whether inhibiting methanogenesis could have altered energy losses in the rumen or post-ruminally, and net energy partition.
Energy Losses
The positive association between energy output in feces and the inhibition of rumen methanogenesis does not agree with the lack of relationship observed in the fecal output of DM, OM, N, and NDF adjusted by the corresponding intakes of those fractions. Part of the discrepancy between the relationships in the fecal output of energy on the one hand and DM, OM, N, and NDF on the other hand, with methanogenesis inhibition, is due to differences in the subset of experiments used for each analysis, as different experiments reported digestibility of different fractions. This highlights the importance of conducting more methanogenesis inhibition experiments simultaneously determining the responses in the digestibilities of DM, OM, N, NDF, and energy. In particular, there is much scarcity of determinations of energy digestibility in milk production experiments, as in the only milk production experiment in which energy digestibility was reported methanogenesis inhibition was <10% of the control treatment (52). The lack of relationships between the output of DM and OM in feces and methanogenesis inhibition found herein largely agrees with in situ experiments (37, 54), although one cannot rule out that if decreases in rumen digestibility occurred in some experiments they were somewhat compensated by post-ruminal digestion.
In the present analysis, rumen VFA concentration had a negative relationship with methanogenesis inhibition. In vivo VFA concentration is not an accurate proxy of fermentative activity, as it does not consider changes in rumen volume (78) and in VFA rates of absorption (79, 80). In in vitro batch and continuous cultures, in which actual VFA production can be measured, inhibiting methanogenesis resulted in a decrease in estimated fermented hexoses calculated from the VFA production stoichiometry, and generally a decrease in enthalpy in total VFA (81).
The increase in energy losses as H2 in relation to energy saved in CH4 not produced varied widely across experiments and was on average much lower than energy saved in CH4. Importantly, in some experiments in which the energy gain obtained from inhibiting CH4 production surpassed the energy losses as H2 there was still no increase in productivity associated (25, 26, 56, 60, 62). Thus, the increase in energy losses as H2 does not seem to account for, at least as the only explanation, the lack of consistent improvements in ruminant productivity associated to rumen methanogenesis inhibition. That said, the addition of phloroglucinol to the methanogenesis-inhibited rumens of steers, which partially relieve H2 accumulation by approximately 50%, translated into greater bodymass gain of steers (66).
Energy losses in urine and heat were unrelated to rumen methanogenesis inhibition and therefore seem unlikely to explain the lack of consistent positive relationships between ruminant productivity and the decrease in energy losses as CH4, although, the same as with other response variables, more experiments are needed to estimate relationships between methanogenesis inhibition and energy losses in urine and heat more accurately.
Net Energy Partition
Less DMI with methanogenesis inhibition could imply decreased NE intake and increased proportion of NE utilized for maintenance, leaving less NE available for production purposes, i.e., the opposite of the dilution of maintenance effect (82). It would therefore be important to better understand the relationship between the inhibition of rumen methanogenesis and DMI. Decrease in DMI can take place through retarding rumen particle outflow i.e., physical filling (83). Inhibiting methanogenesis with nitrate resulted in no changes in rumen fluid outflow rate or volume, but DMI was not affected in that experiment (37). The effect of inhibiting rumen methanogenesis on the outflow rate of rumen particles has not been reported to the author's knowledge.
Intake could also be controlled metabolically through the flow of absorbed propionate, which stimulates the oxidation of acetyl-CoA in the liver, and may act as a satiety signal (83). Inhibiting methanogenesis did not relate to propionate concentration in the present analysis. However, if treatments using nitrate, whose reduction is estimated to be thermodynamically very favorable in the rumen (84) and thus competes with propionate formation for reducing equivalents, were not considered, inhibiting methanogenesis associated positively with propionate concentration (P = 0.037; not shown). Nevertheless, it is unknown if the actual flows of propionate production and absorption are affected by inhibiting CH4 formation in the rumen; the effects of inhibiting rumen methanogenesis on the flows of VFA production and absorption are identified as a gap in knowledge necessary to understand, and perhaps if possible intervene or manage, if inhibiting rumen CH4 production is to translate into benefits in animal productivity.
Potentially poor palatability caused by chemical inhibitors of methanogenesis is not thought to have a general effect on intake due to the variation among products in their chemical nature.
Net energy for production can be utilized in various biosynthetic processes. Lactating animals can direct an important proportion of NE for milk production. However, in mid and late lactation, part of NE for production is used to replenish adipose tissue mobilized in early lactation. In the experiments by Haisan et al. (48), Hristov et al. (56), and Haisan et al. (65), although no productivity gain was obtained in terms of energy output in milk, animals in methanogenesis-inhibited treatments either tended to gain, or numerically gained more bodymass than control animals. In fact, if the extra bodymass gained corresponded entirely to adipose tissue, and assuming a heat of combustion of 32.2 MJ per kilogram of adipose tissue (85), the energy gained in bodymass accretion would surpass the energy saved in CH4 emissions in the experiment by Haisan et al. (48), and would be roughly comparable to the energy saved in gases emissions in the experiment by Hristov et al. (56). Haisan et al. (65) arrived at similar conclusions estimating energy expenditure in numerical extra bodymass gain with methanogenesis inhibition. Conversely, in the experiment by Abecia et al. (42), in which inhibiting rumen methanogenesis resulted in improved energy output in milk per kilogram DMI, there were no associated differences in bodymass changes.
At present, the number of experiments reporting changes in bodymass and body condition score in lactating animals is insufficient to arrive at solid conclusions about the potential importance of changes in NE partition and replenishment of body reserves that might be induced by rumen methanogenesis inhibition. Furthermore, changes in bodymass and body condition score may not reflect energy balance with accuracy. The replenishment of body reserves of lactating ruminants has important implications to reproductive function efficiency and metabolic diseases such as ketosis (86–88). To the knowledge of this author, the long-term effects of inhibiting rumen methanogenesis on reproductive efficiency and metabolic diseases have not been evaluated.
Likewise, in growing and fattening animals, body mass accretion can occur as different proportions of lean and adipose tissue, which has profound implications to the energy content of gained bodymass. Tomkins et al. (36) found a numerical increase in P8 fat depth in the carcass of steers fed a methanogenesis inhibitor. The same as with milk production, information is very scarce about the possible effects of inhibiting methanogenesis on NE partition, including the partition of NE for gain among accretion of different tissues.
Other Aspects
It is also possible that methanogenesis inhibition simply needs to be more extreme for its energy saving effects to be more consistent. The maximal inhibition of methanogenesis in milk production and in growth and fattening experiments in the present database was on average of 28 and 48%, respectively (not shown). There was only one milk production experiment in which CH4 production was inhibited by 60%, although there were no effects on ECM or ECM per kg DMI (48). It is tempting to think that the sheer greater extent of methanogenesis inhibition in some growth and fattening experiments might have resulted in the productivity gains observed (31, 36).
The potential consequences of changes in the acetate to propionate production ratio that might occur on NE allocation toward milk production or body reserves have been discussed (89) but in vivo experimental work on the effects of methanogenesis inhibition on acetate and propionate flows is missing. Furthermore, diets used in methanogenesis inhibition experiments are formulated to match nutrient requirements. If inhibiting CH4 production augments the flow of absorbed propionate, some decrease in glucogenic precursors supplied by the basal diet may be needed for the extra supply of glucogenic precursors resulting from inhibiting methanogenesis to consistently benefit animal production.
Final Remarks
Gains in productivity appear as important for adoption of CH4-mitigation techniques by producers. However, a consistent association between the inhibition of rumen methanogenesis and improvements in ruminant productivity could not be confirmed by the present research. The reader is cautioned about the limitations of the present analysis in terms of number of experiments and uniformity of the response variables reported in them. Also, some of the older experiments used few animals and estimates of variation were not reported.
Capitalizing energy savings in CH4 not formed as animal productivity may require refinements of the methanogenesis inhibition intervention, such as modifications of basal diets and/or combinations with other interventions. Development of rational strategies to translate methanogenesis inhibition into gains in productivity will likely require a more complete understanding of some existing gaps in knowledge such as the effects of inhibiting CH4 production in the rumen on digestion and on the flows of VFA production and absorption, the mechanisms through which inhibiting methanogenesis affects DMI, and effects on NE partition. At the same time, more performance experiments both on milk production and growth and fattening are needed, including treatments targeting both moderate and severe methanogenesis inhibition.
Ethics Statement
This study is a meta-analysis of published literature. It did not involve any experimental work. It involves summarization of published work and mathematical modeling.
Author Contributions
EU conceived the research, compiled the database, conducted the meta-analysis, interpreted the results and wrote and edited the manuscript.
Funding
Support from Comisión Nacional de Investigación Científica y Tecnológica (CONICYT) through project Fondecyt 1160764 is gratefully acknowledged.
Conflict of Interest Statement
The author declares that the research was conducted in the absence of any commercial or financial relationships that could be construed as a potential conflict of interest.
Acknowledgments
The author appreciates insightful discussion with Franz Roos and Jeff Firkins.
Supplementary Material
The Supplementary Material for this article can be found online at: https://www.frontiersin.org/articles/10.3389/fvets.2018.00113/full#supplementary-material
Abbreviations
BMG, bodymass gain; CH4, methane; CO2, carbon dioxide; DE, digestible energy; DM, dry matter; DMD. apparent digestibility of dry matter; DMI, dry matter intake; ECM, energy-corrected milk; GE, gross energy; GEI, gross energy intake; [H], metabolic hydrogen; H2, dihydrogen; HP, heat production; ME, metabolizable energy; ND, apparent digestibility of nitrogen; NDFD, neutral detergent fiber digestibility; NE, net energy; NEm, net energy for maintenance; OM, organic matter; OMD, apparent digestibility of organic matter; SEM, standard error of the mean; UE, urine energy; VFA, volatile fatty acids.
References
1. Russell JB, Wallace RJ. Energy-yielding and energy-consuming reactions. In. The Rumen Microbial Ecosystem, Hobson PN, Stewart CS, editors. 2nd ed. London: Blackie Academic & Professional (1997). p. 246–82.
2. Veneman JB, Saetnan ER, Clare AJ, Newbold CJ. MitiGate; an online meta-analysis database for quantification of mitigation strategies for enteric methane emissions. Sci Total Environ. (2016) 572:1166–74. doi: 10.1016/j.scitotenv.2016.08.029
3. Duin EC, Wagner T, Shima S, Prakash D, Cronin B, Yanez-Ruiz DR, et al. Mode of action uncovered for the specific reduction of methane emissions from ruminants by the small molecule 3-nitrooxypropanol. Proc Natl Acad Sci USA. (2016) 113:6172–7. doi: 10.1073/pnas.1600298113
5. Czerkawski JW, Breckenridge G. New inhibitors of methane production by rumen micro- organisms. Experiments with animals and other practical possibilities. Brit J Nutr. (1975) 34:447–57.
6. Martin SA, Macy JM. Effects of monensin, pyromellitic diimide and 2-bromoethanesulfonic acid on rumen fermentation in vitro. J Anim Sci. (1985) 60:544–50.
7. Hill J, McSweeney C, Wright AD, Bishop-Hurley G, Kalantar-Zadeh K. Measuring Methane Production from Ruminants. Trends Biotechnol. (2016) 34:26–35. doi: 10.1016/j.tibtech.2015.10.004
8. Hristov AN, Oh J, Lee C, Meinen R, Montes F, Ott T, et al. Mitigation of Greenhouse Gas Emissions in Livestock Production – a Review of Technical Options for Non-CO2 Emissions. Rome: FAO (2013).
9. Eugène M, Masse' D, Chiquette J, Benchaar C. Meta-analysis on the effects of lipid supplementation on methane production in lactating dairy cows. Can J Anim Sci. (2008) 88:331–7. doi: 10.4141/CJAS07112
10. Martin C, Morgavi DP, Doreau M. Methane mitigation in ruminants: from microbe to the farm scale. Animal (2010) 4:351–65. doi: 10.1017/S1751731109990620
11. Beauchemin KA, Kreuzer M, O'Mara F, McAllister TA. Nutritional management for enteric methane abatement: a review. Aust J Exp Agr. (2008) 48:21–7. doi: 10.1071/EA07199
12. Appuhamy JA, Strathe AB, Jayasundara S, Wagner-Riddle C, Dijkstra J, France J, et al. Anti-methanogenic effects of monensin in dairy and beef cattle: a meta-analysis. J Dairy Sci. (2013) 96:5161–73. doi: 10.3168/jds.2012-5923
13. Lee C, Beauchemin KA. A review of feeding supplementary nitrate to ruminant animals: nitrate toxicity, methane emissions, and production performance. Can J Anim Sci. (2014) 94:557–70. doi: 10.4141/cjas-2014-069
14. Jayanegara A, Sarwono KA, Kondo M, Matsui H, Ridla M, Laconi EB, et al. Use of 3-nitrooxypropanol as feed additive for mitigating enteric methane emissions from ruminants: a meta-analysis. Ital J Anim Sci. (2017) 17:1–7. doi: 10.1080/1828051X.2017.1404945
15. Patra AK. Meta-analyses of effects of phytochemicals on digestibility and rumen fermentation characteristics associated with methanogenesis. J Sci Food Agric. (2010) 90:2700–8. doi: 10.1002/jsfa.4143
16. Nagaraja TG, Newbold CJ, van Nevel CJ, Demeyer DI. Manipulation of rumen fermentation. In.The Rumen Microbial Ecosystem, Hobson PN, Stewart CS, editors. 2nd ed. London: Blackie Academic & Professional (1997). p. 524–632.
17. Patra AK, Saxena J. A new perspective on the use of plant secondary metabolites to inhibit methanogenesis in the rumen. Phytochemistry (2010) 71:1198–222. doi: 10.1016/j.phytochem.2010.05.010
18. Newbold CJ, de la Fuente G, Belanche A, Ramos-Morales E, McEwan NR. The role of ciliate protozoa in the rumen. Front Microbiol. (2015) 6:1313. doi: 10.3389/fmicb.2015.01313
19. Patra A, Saxena A. Exploitation of dietary tannins to improve rumenmetabolism and ruminant nutrition. J Sci Food Agric. (2011) 91:24–37. doi: 10.1002/jsfa.4152
20. Carro MD, Ungerfeld EM. Utilization of organic acids to manipulate ruminal fermentation and improve ruminant productivity. In. Rumen Microbiology: From Evolution to Revolution, Puniya AK, Singh R, Kamra DN, editors. New Dehli: Springer (2015). p. 177–97.
21. Fitzsimons C, Kenny DA, Deighton MH, Fahey AG, McGee M. Methane emissions, body composition, and rumen fermentation traits of beef heifers differing in residual feed intake. J Anim Sci. (2013) 91:5789–800. doi: 10.2527/jas.2013-6956
22. Wedlock DN, Janssen PH, Leahy SC, Shu D, Buddle BM. Progress in the development of vaccines against rumen methanogens. Animal (2013) 7(Suppl. 2):244–52. doi: 10.1017/s1751731113000682
23. Czerkawski JW, Blaxter KL, Wainman FW. The effect of functional groups other than carboxyl on the metabolism of C18 and C12 alkyl compounds by sheep. Br J Nutr. (1966) 20:495–508.
24. Clapperton JL, Czerkawski JW. The effect of tertiary branched-chain carboxylic acids on the energy metabolism of sheep. Brit J Nutr. (1971) 26:459–68.
25. Johnson DE. Effects of a hemiacetal of chloral and starch on methane production and energy balance of sheep fed a pelleted diet. J Anim Sci. (1972) 35:1064–8.
26. Johnson DE. Adaptational responses in nitrogen and energy balance of lambs fed a methane inhibitor. J Anim Sci. (1974) 38:154–7.
27. Sawyer MS, Hoover WH, Sniffen CJ. Effect of a ruminal methane inhibitor on growth and energy metabolism in the ovine. J Anim Sci. (1974) 38:908–14.
28. Cole NA, McCroskey JE. Effects of hemiacetal of chloral and starch on the performance of beef steers. J Anim Sci. (1975) 41:1735–41.
29. Davies A, Nwaonu HN, Stanier G, Boyle FT. Properties of a novel series of inhibitors of rumen methanogenesis; in vitro and in vivo experiments including growth trials on 2,4-bis (trichloromethyl)-benzo [1, 3]dioxin-6-carboxylic acid. Brit J Nutr. (1982) 47:565–76.
30. Mathers JC, Miller EL. Some effects of chloral hydrate on rumen fermentation and digestion in sheep. J Agric Sci. (Camb) (1982) 99:215–24.
31. McCrabb GJ, Berger KT, T. M, May C, Hunter RA. Inhibiting methane production in Brahman cattle by dietary supplementation with a novel compound and the effects on growth. Aust J Agr Res. (1997) 48:323–9. doi: 10.1071/AR96119
32. Lila ZA, Mohammed N, Tatsuoka N, Kanda S, Kurokawa Y, Itabashi H. Effect of cyclodextrin diallyl maleate on methane production, ruminal fermentation and microbes in vitro and in vivo. Anim Sci J. (2004) 75:15–22. doi: 10.1111/j.1740-0929.2004.00149.x
33. Mohammed N, Lila ZA, Tatsuoka N, Hara K, Mikuni K, Hara K, et al. Effects of cyclodextrin-iodopropane complex on methane production, ruminal fermentation and microbes, digestibility and blood metabolites in steers. Anim Sci J. (2004) 75:131–7. doi: 10.1111/j.1740-0929.2004.00167.x
34. Tomkins NW, Hunter RA. Methane reduction in beef cattle using a novel antimethanogen. Proceedings of the Australian Society of Animal Production 25th Biennial Conference : the New Realities; 2004 4-8th July, 2004 University of Melbourne. Parkville, VIC; Collingwood, VIC: CSIRO Publishing (2004).
35. Denman SE, Tomkins NW, McSweeney CS. Quantitation and diversity analysis of ruminal methanogenic populations in response to the antimethanogenic compound bromochloromethane. FEMS Microbiol Ecol. (2007) 62:313–22. doi: 10.1111/j.1574-6941.2007.00394.x
36. Tomkins NW, Colegate SM, Hunter RA. A bromochloromethane formulation reduces enteric methanogenesis in cattle fed grain-based diets. Anim Prod Sci. (2009) 49:1053–8. doi: 10.1071/EA08223
37. Nolan JV, Hegarty RS, Hegarty J, Godwin IR, Woodgate R. Effects of dietary nitrate on fermentation, methane production and digesta kinetics in sheep. Anim Prod Sci. (2010) 50:801–6. doi: 10.1071/AN09211
38. van Zijderveld SM, Gerrits WJJ, Apajalahti JA, Newbold JR, Dijkstra J, Leng RA, et al. Nitrate and sulfate: effective alternative hydrogen sinks for mitigation of ruminal methane production in sheep. J Dairy Sci. (2010) 93:5856–66. doi: 10.3168/jds.2010-3281
39. Brown EG, Anderson RC, Carstens GE, Gutiérrez-Bañuelos H, McReynolds JL, Slay LJ, et al. Effects of oral nitroethane administration on enteric methane emissions and ruminal fermentation in cattle. Anim Feed Sci Technol. (2011) 166–167:275–81. doi: 10.1016/j.anifeedsci.2011.04.017
40. van Zijderveld SM, Gerrits WJJ, Dijkstra J, Newbold JR, Hulshof RBA, Perdok HB. Persistency of methane mitigation by dietary nitrate supplementation in dairy cows. J Dairy Sci. (2011) 94:4028–38. doi: 10.3168/jds.2011-4236
41. Mitsumori M, Shinkai T, Takenaka A, Enishi O, Higuchi K, Kobayashi Y, et al. Responses in digestion, rumen fermentation and microbial populations to inhibition of methane formation by a halogenated methane analogue. Brit J Nutr. (2012) 108:482–91. doi: 10.1017/S0007114511005794
42. Abecia L, Toral PG, Martín-García AI, Martínez G, Tomkins NW, Molina-Alcaide E, et al. Effect of bromochloromethane on methane emission, rumen fermentation pattern, milk yield, and fatty acid profile in lactating dairy goats. J Dairy Sci. (2012) 95:2027–36. doi: 10.3168/jds.2011-4831
43. Hulshof RB, Berndt A, Gerrits WJ, Dijkstra J, van Zijderveld SM, Newbold JR, et al. Dietary nitrate supplementation reduces methane emission in beef cattle fed sugarcane-based diets. J Anim Sci. (2012) 90:2317–23. doi: 10.2527/jas.2011-4209
44. Li L, Davis J, Nolan J, Hegarty R. An initial investigation on rumen fermentation pattern and methane emission of sheep offered diets containing urea or nitrate as the nitrogen source. Anim Prod Sci. (2012) 52:653–8. doi: 10.1071/AN11254
45. Shinkai T, Enishi O, Mitsumori M, Higuchi K, Kobayashi Y, Takenaka A, et al. Mitigation of methane production from cattle by feeding cashew nut shell liquid. J Dairy Sci. (2012) 95:5308–16. doi: 10.3168/jds.2012-5554
46. Martinez-Fernandez G, Abecia L, Martin-Garcia AI, Ramos-Morales E, Hervas G, Molina-Alcaide E, et al. In vitro-in vivo study on the effects of plant compounds on rumen fermentation, microbial abundances and methane emissions in goats. Animal (2013) 7:1925–34. doi: 10.1017/s1751731113001699
47. El-Zaiat HM, Araujo RC, Soltan YA, Morsy AS, Louvandini H, Pires AV, et al. Encapsulated nitrate and cashew nut shell liquid on blood and rumen constituents, methane emission, and growth performance of lambs. J Anim Sci. (2014) 92:2214–24. doi: 10.2527/jas.2013-7084
48. Haisan J, Sun Y, Guan LL, Beauchemin KA, Iwaasa A, Duval S, et al. The effects of feeding 3-nitrooxypropanol on methane emissions and productivity of Holstein cows in mid lactation. J Dairy Sci. (2014) 97:3110–9. doi: 10.3168/jds.2013-7834
49. Lund P, Dahl R, Yang H, Hellwing A, Cao B, Weisbjerg M. The acute effect of addition of nitrate on in vitro and in vivo methane emission in dairy cows. Anim Prod Sci. (2014) 54:1432–5. doi: 10.1071/AN14339
50. Newbold JR, van Zijderveld SM, Hulshof RB, Fokkink WB, Leng RA, Terencio P, et al. The effect of incremental levels of dietary nitrate on methane emissions in Holstein steers and performance in Nelore bulls. J Anim Sci. (2014) 92:5032–40. doi: 10.2527/jas.2014-7677
51. de Raphélis-Soissan V, Li L, Godwin IR, Barnett MC, Perdok HB, Hegarty RS. Use of nitrate and Propionibacterium acidipropionici to reduce methane emissions and increase wool growth of Merino sheep. Anim Prod Sci. (2014) 54:1860–6. doi: 10.1071/AN14329
52. Reynolds CK, Humphries DJ, Kirton P, Kindermann M, Duval S, Steinberg W. Effects of 3-nitrooxypropanol on methane emission, digestion, and energy and nitrogen balance of lactating dairy cows. J Dairy Sci. (2014) 97:3777–89. doi: 10.3168/jds.2013-7397
53. Romero-Perez A, Okine EK, McGinn SM, Guan LL, Oba M, Duval SM, et al. The potential of 3-nitrooxypropanol to lower enteric methane emissions from beef cattle. J Anim Sci. (2014) 92:4682–93. doi: 10.2527/jas2014-7573
54. Martínez-Fernández G, Abecia L, Arco A, Cantalapiedra-Hijar G, Martín-García AI, Molina-Alcaide E, et al. Effects of ethyl-3-nitrooxy propionate and 3-nitrooxypropanol on ruminal fermentation, microbial abundance, and methane emissions in sheep. J Dairy Sci. (2014) 97:3790–9. doi: 10.3168/jds.2013-7398
55. Mitsumori M, Matsui H, Tajima K, Shinkai T, Takenaka A, Denman SE, et al. Effect of bromochloromethane and fumarate on phylogenetic diversity of the formyltetrahydrofolate synthetase gene in bovine rumen. Anim Sci J. (2014) 85:25–31. doi: 10.1111/asj.12072
56. Hristov AN, Oh J, Giallongo F, Frederick TW, Harper MT, Weeks HL, et al. An inhibitor persistently decreased enteric methane emission from dairy cows with no negative effect on milk production. Proc Natl Acad Sci USA. (2015) 112:10663–8. doi: 10.1073/pnas.1504124112
57. Lee C, Araujo RC, Koenig KM, Beauchemin KA. Effects of encapsulated nitrate on enteric methane production and nitrogen and energy utilization in beef heifers. J Anim Sci. (2015) 93:2391–404. doi: 10.2527/jas.2014-8845
58. Romero-Perez A, Okine EK, McGinn SM, Guan LL, Oba M, Duval SM, et al. Sustained reduction in methane production from long-term addition of 3-nitrooxypropanol to a beef cattle diet. J Anim Sci. (2015) 93:1780–91. doi: 10.2527/jas.2014-8726
59. Veneman JB, Muetzel S, Hart KJ, Faulkner CL, Moorby JM, Perdok HB, et al. Does dietary mitigation of enteric methane production affect rumen function and animal productivity in dairy cows? PLoS ONE (2015) 10:e0140282. doi: 10.1371/journal.pone.0140282
60. Lopes JC, de Matos LF, Harper MT, Giallongo F, Oh J, Gruen D, et al. Effect of 3-nitrooxypropanol on methane and hydrogen emissions, methane isotopic signature, and ruminal fermentation in dairy cows. J Dairy Sci. (2016) 99:5335–44. doi: 10.3168/jds.2015-10832
61. Martinez-Fernandez G, Denman SE, Yang C, Cheung J, Mitsumori M, McSweeney CS. Methane inhibition alters the microbial community, hydrogen flow, and fermentation response in the rumen of cattle. Front Microbiol. (2016) 7:1122. doi: 10.3389/fmicb.2016.01122
62. Olijhoek DW, Hellwing AL, Brask M, Weisbjerg MR, Hojberg O, Larsen MK, et al. Effect of dietary nitrate level on enteric methane production, hydrogen emission, rumen fermentation, and nutrient digestibility in dairy cows. J Dairy Sci. (2016) 99:6191–205. doi: 10.3168/jds.2015-10691
63. Vyas D, McGinn SM, Duval SM, Kindermann M, Beauchemin KA. Effects of sustained reduction of enteric methane emissions with dietary supplementation of 3-nitrooxypropanol on growth performance of growing and finishing beef cattle. J Anim Sci. (2016) 94:2024–34. doi: 10.2527/jas.2015-0268
64. Vyas D, McGinn SM, Duval SM, Kindermann MK, Beauchemin KA. Optimal dose of 3-nitrooxypropanol for decreasing enteric methane emissions from beef cattle fed high-forage and high-grain diets. Anim Prod Sci. (2016) 58:1049–55. doi: 10.1071/AN15705
65. Haisan J, Sun Y, Guan LL, Beauchemin KA, Iwaasa A, Duval S, et al. The effects of feeding 3-nitrooxypropanol at two doses on milk production, rumen fermentation, plasma metabolites, nutrient digestibility, and methane emissions in lactating Holstein cows. Anim Prod Sci. (2017) 57:282–9. doi: 10.1071/AN15219
66. Martinez-Fernandez G, Denman SE, Cheung J, McSweeney C. Phloroglucinol degradation in the rumen promotes the capture of excess hydrogen generated from methanogenesis inhibition. Front Microbiol. (2017) 8:1871. doi: 10.3389/fmicb.2017.01871
67. Tyrrell HF, Reid JT. Prediciting the energy value of cow's milk. J Dairy Sci. (1965) 48:1215–23.
68. Henderson C. The influence of extracellular hydrogen on the metabolism of Bacteroides rurninicola, Anaerovibrio lipolytica and Selenomonas ruminantiurn. J Gen Microbiol. (1980) 119:485–91.
69. Van den Noortgate W, López-López JA, Marín-Martínez F, Sánchez-Meca J. Three-level meta-analysis of dependent effect sizes. Behav Res Methods (2013) 45:576–94. doi: 10.3758/s13428-012-0261-6
70. St-Pierre NR. Invited review: Integrating quantitative findings from multiple studies using mixed model methodology. J Dairy Sci. (2001) 84:741–55. doi: 10.3168/jds.S0022-0302(01)74530-4
71. Kronmal RA. Spurious correlation and the fallacy of the ratio standard revisited. J R Statist Soc A (1993) 156:379–92. doi: 10.2307/2983064
72. Shibata M, Terada F. Factors affecting methane production and mitigation in ruminants. Anim Sci J. (2010) 81:2–10. doi: 10.1111/j.1740-0929.2009.00687.x
73. Domalski ES. Selected values of heats of combustion and heats of formation of organic compounds containing the elements C, H, N, O, P, and S. J Phys Chem Ref Data (1972) 1:221–77.
74. McAllister S, Chen JY, Fernandez-Pello A. Fundamentals of Combustion Processes. New York, NY: Springer (2011).
75. Sauvant D, Schmidely P, Daudin JJ, St-Pierre NR. Meta-analyses of experimental data in animal nutrition. Animal (2008) 2:1203–14. doi: 10.1017/S1751731108002280
76. Belsey DA, Kuh E, Welsch RE. Regression Diagnostics: Identifying Influential Data and Sources of Collinearity. New York: John Wiley & Sons (1980).
77. NRC. Nutrient Requirements of Dairy Cattle. 7th ed. Press NA, editor. Washington, DC: National Research Council (2001). p. 406.
78. Hall MB, Nennich TD, Doane PH, Brink GE. Total volatile fatty acid concentrations are unreliable estimators of treatment effects on ruminal fermentation in vivo. J Dairy Sci. (2015) 98:3988–99. doi: 10.3168/jds.2014-8854
79. Dijkstra J, Boer H, Van Bruchem J, Bruining M, Tamminga S. Absorption of volatile fatty acids from the rumen of lactating dairy cows as influenced by volatile fatty acid concentration, pH and rumen liquid volume. Br J Nutr. (1993) 69:385–96.
80. Storm AC, Kristensen NB, Hanigan MD. A model of ruminal volatile fatty acid absorption kinetics and rumen epithelial blood flow in lactating Holstein cows. J Dairy Sci. (2012) 95:2919–34. doi: 10.3168/jds.2011-4239
81. Ungerfeld EM. Shifts in metabolic hydrogen sinks in the methanogenesis-inhibited ruminal fermentation: a meta-analysis. Front Microbiol. (2015) 6:1–17. doi: 10.3389/fmicb.2015.00037
82. Capper JL, Cady RA, Bauman DE. The environmental impact of dairy production: 1944 compared with 2007. J Anim Sci. (2009) 87:2160–7. doi: 10.2527/jas.2009-1781
83. Allen MS. Drives and limits to feed intake in ruminants. Anim Prod Sci. (2014) 54:1513–24. doi: 10.1071/AN14478
84. Ungerfeld EM, Kohn RA. The role of thermodynamics in the control of ruminal fermentation. In. Ruminant Physiology, Sejrsen K, Hvelplund T, Nielsen MO, editors. Wageningen: Wageningen Academic Publishers (2006). p. 55–85.
85. Hall KD. What is the required energy deficit per unit weight loss? Int J Obes (Lond.) (2008) 32:573–6. doi: 10.1038/sj.ijo.0803720
86. Butler WR, Smith RD. Interrelationships between energy balance and postpartum reproductive function in dairy cattle. J Dairy Sci. (1989) 72:767–83. doi: 10.3168/jds.S0022-0302(89)79169-4
87. Montiel F, Ahuja C. Body condition and suckling as factors influencing the duration of postpartum anestrus in cattle: a review. Anim Reprod Sci. (2005) 85:1–26. doi: 10.1016/j.anireprosci.2003.11.001
88. Roche JR, Kay JK, Friggens NC, Loor JJ, Berry DP. Assessing and managing body condition score for the prevention of metabolic disease in dairy cows. Vet Clin North Am Food Anim Pract. (2013) 29:323–36. doi: 10.1016/j.cvfa.2013.03.003
Keywords: rumen, methane, methanogenesis, energy, inhibition, ruminants, productivity, meta-analysis
Citation: Ungerfeld EM (2018) Inhibition of Rumen Methanogenesis and Ruminant Productivity: A Meta-Analysis. Front. Vet. Sci. 5:113. doi: 10.3389/fvets.2018.00113
Received: 17 November 2017; Accepted: 14 May 2018;
Published: 19 June 2018.
Edited by:
Amlan Kumar Patra, West Bengal University of Animal and Fishery Sciences, IndiaReviewed by:
Manuel Gonzalez Ronquillo, Universidad Autónoma del Estado de México, MexicoYosra Ahmed Soltan, Alexandria University, Egypt
Ian John Lean, Scibus, Australia
Copyright © 2018 Ungerfeld. This is an open-access article distributed under the terms of the Creative Commons Attribution License (CC BY). The use, distribution or reproduction in other forums is permitted, provided the original author(s) and the copyright owner are credited and that the original publication in this journal is cited, in accordance with accepted academic practice. No use, distribution or reproduction is permitted which does not comply with these terms.
*Correspondence: Emilio M. Ungerfeld, emilio.ungerfeld@inia.cl