- 1Department of Poultry Science, University of Arkansas, Fayetteville, AR, USA
- 2Facultad de Medicina Veterinaria y Zootecnia, Universidad Nacional Autónoma de México, Mexico City, Mexico
- 3Department of Animal Science, The Ohio State University, Columbus, OH, USA
Fluorescein isothiocyanate dextran (FITC-d) is a 3–5 kDa marker used to measure tight junction permeability. We have previously shown that intestinal barrier function can be adversely affected by stress, poorly digested diets, or feed restriction (FR), resulting in increased intestinal inflammation-associated permeability. However, further optimization adjustments of the current FITC-d methodology are possible to enhance precision and efficacy of results in future. The objective of the present study was to optimize our current model to obtain a larger difference between control and treated groups, by optimizing the FITC-d measurement as a biomarker in a 24-h FR model to induce gut permeability in broiler chickens. One in vitro and four in vivo independent experiments were conducted. The results of the present study suggest that by increasing the dose of FITC-d (8.32 versus 4.16 mg/kg); shortening the collection time of blood samples (1 versus 2.5 h); using a pool of non-FITC-d serum as a blank, compared to previously used PBS; adding a standard curve to set a limit of detection and modifying the software’s optimal sensitivity value, it was possible to obtain more consistent and reliable results.
Introduction
Intestinal epithelial cells are not only responsible for digestion, secretion, and absorption but also act as a physical barrier separating external environmental agents from the internal host environment. In addition to preventing the entry of harmful intraluminal microorganisms, antigens, and toxins, this barrier increases the bodies’ tolerance to nutrients, water, and electrolytes (1–3). Microbes that live inside and/or on animals outnumber the animals’ actual somatic and germ cells by an estimated 10-fold (4). Hence, any alterations in gut permeability are associated with bacterial translocation to the portal and/or systemic circulation leading to systemic bacterial infections (5, 6). Consequently, our laboratory has recently developed several models to induce intestinal inflammation in poultry. Those models include high non-starch polysaccharides diets (7, 8); dexamethasone (9); dextran sodium sulfate (DSS) (10, 11); and 24-h feed restriction (FR) (12, 13). In the above models, inflammation causes disruption of the epithelial tight junctions (TJs) increasing bacterial translocation and leakage of serum fluorescein isothiocyanate dextran (FITC-d). FITC-d is a large molecule (3–5 kDa) which under normal conditions is not able to cross the epithelial barrier (14). However, during intestinal inflammation, the TJs are disrupted allowing the FITC-d molecule to enter circulation. Previous results from our laboratory have demonstrated that in poultry, chemically induced disruption of TJs with DSS (10) increases transmucosal permeability as seen by elevated serum levels of FITC-d (11). On the other hand, recently, we have shown that dietary inclusion of a Bacillus-based direct-fed microbial ameliorated the adverse gut permeability inflammatory effects related to utilization of rye-based diets in turkeys and in broiler chickens (15, 16). We have previously shown that FITC-d can be used as a biomarker for intestinal barrier function (7–12). However, further optimization adjustments of the current FITC-d methodology are possible to enhance precision and efficacy of results in future studies as can be observed in Figure 1 and Table 5. The objective of the present study was to optimize our current FITC-d model to obtain a larger difference between control and treated groups, using our 24-h FR model to induce gut permeability in broiler chickens.
Materials and Methods
Fluorescein Isothiocyanate Dextran
Fluorescein isothiocyanate dextran (MW 3–5 KDa; Sigma Aldrich Co., St. Louis, MO, USA) was used as a marker of paracellular transport and mucosal barrier dysfunction.
In Vitro Evaluation of Different Fluorescence Gain Using Blank Chicken Sera from Chickens without FITC-d
Unlike absorbance assays where the gain on the plate reader is fixed and not user changeable, fluorescence assays have varying concentration ranges and require the gain on the photomultiplier to be adjusted. In this in vitro experiment, the following formula was used to predict the relative fluorescence units when changing the gain:
To determine if fluorescence changes with varying gain, blank chicken sera and 0.9% saline were compared. Non-FITC-d chicken sera was diluted 1:5 in 0.9% saline onto black 96-well fluorescent plates and measured from gain 40 to gain 80 with continuous increments of 10. Non-FITC-d sera were also used to develop a standard curve adapted for every plate using six two-fold serial dilutions from the highest value 6,400 ng/mL until it reach 0 ng/mL (Table 1).
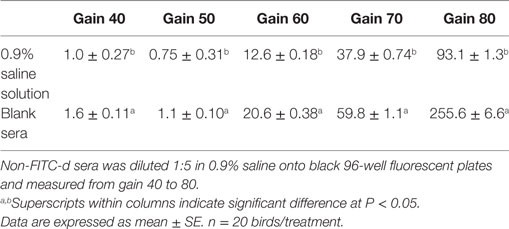
Table 1. Evaluation of different fluorescence gain using blank chicken sera, from chickens without fluorescein isothiocyanate dextran (FITC-d), versus 0.9% saline solution.
Experimental Animals
Four in vivo experiments were conducted to determine the optimal procedure for using FITC-d as a biomarker for intestinal permeability. In all trials, broiler chickens were obtained from a primary breeder company and all experiments were conducted in battery cages in a controlled age-appropriate environment.
FR Model
In all experiments, intestinal permeability was induced using FR as previously published (12, 13). Chickens were randomly assigned to each experimental group and had unrestricted access to feed and water from 1 to 10 days of age. Beginning at 10 days, chickens in control FITC-d groups were allowed to continue with ad libitum access to feed, while chickens in FR FITC-d groups were subjected to 24 h of FR. Concentration of FITC-d was given based on group body weight; therefore, groups were weighed the day before FR began. At 11 days of age, chickens in all groups were given an appropriate dose of FITC-d by oral gavage for each experiment. After 1 h, or 2.5 h respectively, chickens were euthanized with CO2 asphyxiation. Blood samples were collected from the femoral vein to quantify levels of FITC-d.
Serum Determination of FITC-d
In all experiments, blood was centrifuged (1,000 × g for 15 min) to separate the serum from the red blood cells. FITC-d levels of diluted sera were measured at excitation wavelength of 485 nm and emission wavelength of 528 nm (Synergy HT, Multi-mode microplate reader, BioTek Instruments, Inc., VT, USA). Fluorescence measurements were then compared to a standard curve with known FITC-d concentrations (old method) or non-FITC-d sera obtain from each independent experiment, respectively, to develop a standard curve as described in the in vitro methods.
Experimental Designs
Experiment 1: Comparing Two Dilution Methods on Serum FITC-d Read at Gain 70 in a 24-h FR Model
Eighty chickens were randomly assigned to one of four groups (n = 20/group): (1) control no FITC-d; (2) FR no FITC-d; (3) control FITC-d 4.16 mg/kg; and (4) FR FITC-d 4.16 mg/kg. Control groups had ad libitum access to feed, meanwhile FR groups were feed restricted for 24 h before sampling. Serum was diluted at 1:5 or 1:10 to determine if a higher dilution factor would eliminate some of the background fluorescence. Readings were performed with a gain 70.
Experiment 2: Comparing Two Sampling Collection Times and Different Gain Readings of Serum FITC-d in a 24-h FR Model
In this experiment, all chickens received FITC-d (4.16 mg/kg) and samples were collected at 1 or 2.5 h post FITC-d administration. Eighty chickens were randomly assigned to one of four groups (n = 20/group): (1) control FITC-d collected 1 h post gavage; (2) FR FITC-d collected 1 h post-gavage; (3) control FITC-d collected 2.5 h post-gavage; and (4) FR FITC-d collected 2.5 h post-gavage. Control groups had ad libitum access to feed, meanwhile FR groups were feed restricted for 24 h before sampling. Serum was diluted at 1:5 and readings were done with gains 30, 35, 40, and 45.
Experiment 3: Comparing Collection Time of Serum FITC-d Diluted 1:5 and Read at Gain 40 in a 24-h FR Model
In this experiment, all chickens received FITC-d (8.32 mg/kg) and samples were collected at 1 or 2.5 h post FITC-d administration. Eighty chickens were randomly assigned to one of four groups (n = 20/group): (1) control FITC-d collected 1 h post-gavage; (2) FR FITC-d collected 1 h post-gavage; (3) control FITC-d collected 2.5 h post-gavage; and (4) FR FITC-d collected 2.5 h post-gavage. Control groups had ad libitum access to feed, meanwhile FR groups were feed restricted for 24 h before sampling. Serum was diluted at 1:5 and readings were done using gain 40.
Experiment 4: Comparing the Old Method versus Optimized Method of Serum FITC-d in a 24-h FR Model
The objective of this experiment was to compare our previous FITC-d method to the new optimized FITC-d method. Eighty chickens were randomly assigned to one of four groups (n = 20/group): (1) control FITC-d (4.16 mg/kg) collected 2.5 h post-gavage; (2) FR FITC-d (4.16 mg/kg) collected 2.5 h post-gavage; (3) control FITC-d (8.32 mg/kg) collected 1 h post-gavage; and (4) FR FITC-d (8.32 mg/kg) collected 1 h post-gavage. In the old method, serum was diluted 1:5, fluorescence measurements were quantified using an equation from a previously determined standard curve with known FITC-d concentrations using 0.9% saline solution as a blank and measuring samples at gain 70. In the optimized method, serum from non-FITC-d chickens was obtained, to be used as a blank. Additionally, for each plate, a standard curve was adapted diluting known concentrations of FITC-d in the 1:5 diluted blank sera as described above in the in vitro method. All serum samples were also diluted 1:5 for fluorescence reading at gain 40 (Table 5).
Statistical Analysis
All data were subjected to Analysis of Variance as a completely randomized design using the General Linear Models procedure of SAS (17). In all trials, data are expressed as mean ± standard error. Significant differences among the means were determined by using Tukey’s multiple-range test at P < 0.05.
Results
The results of the in vitro evaluation of different fluorescence gains using blank chicken sera, from chickens without FITC-d, versus 0.9% saline solution are summarized in Table 1. There was a significant difference between blank sera and 0.9% saline solution at each of the gains measured (40, 50, 60, 70, 80) (Table 1). This indicates that blank sera has a higher amount of fluorescence activity than 0.9% saline and is affected by the gain. Table 2 illustrates the results from Experiment 1 comparing two serum dilution methods on serum FITC-d (4.16 mg/kg) read at gain 70 in a 24-h FR model. In this study, using the same sera, samples were diluted 1:5 and 1:10 to determine if a higher dilution factor would eliminate some of the background fluorescence. A significant reduction in the background fluorescence was observed in all samples diluted at 1:10 (P < 0.05). Interestingly, serum samples from FR chickens treated with FITC-d diluted at 1:5 or 1:10 showed significantly higher amounts of serum FITC-d concentration when compared with control chickens.
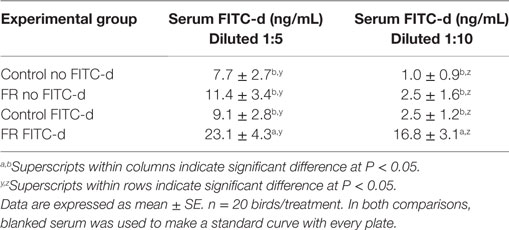
Table 2. Comparing two serum dilution methods on serum fluorescein isothiocyanate dextran (FITC-d) (4.16 mg/kg) read at gain 70 in a 24-h feed restriction (FR) model to induce gut permeability in broiler chickens (Experiment 1).
Results from Experiment 2 comparing two sampling collection times and different gain readings of serum FITC-d (4.16 mg/kg) in a 24-h FR model are summarized in Table 3. Collecting the blood samples 1 h post FITC-d gavage not only showed significant increases in serum FITC-d concentration in chickens that received FR when compared with control chickens at all four gain readings but also the window of differences between feed restricted and control broilers were more evident when compared with serum collected at 2.5 h (Table 3).
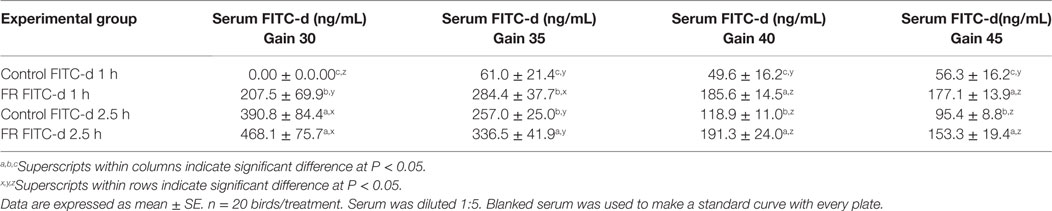
Table 3. Comparing two sampling collection times and different gain readings of serum fluorescein isothiocyanate dextran (FITC-d) (4.16 mg/kg) in a 24-h feed restriction (FR) model to induce gut permeability in broiler chickens (Experiment 2).
Table 4 displays the results from Experiment 3, comparing collection time of serum FITC-d doubling the dose of FITC-d (8.32 mg/kg). Serum was diluted at 1:5 and read at gain 40 in a 24-h FR model. These results confirmed and extended the results of Experiment 2. Sample collection time gives a stronger reading of serum FITC-d in FR chickens when is performed 1 h after FITC-d oral administration when compared with 2.5 h (Table 4).
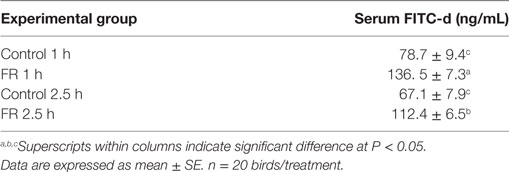
Table 4. Comparing collection times of serum fluorescein isothiocyanate dextran (FITC-d) (8.32 mg/kg) diluted 1:5 and read at gain 40 in a 24-h feed restriction (FR) model to induce gut permeability in broiler chickens (Experiment 3).
The results from Experiment 4, comparing old method versus optimized method of serum FITC-d in a 24-h FR model are summarized in Figure 1 and Table 5. In the old method, chickens received 4.16 mg/kg FITC-d, serum samples were collected 2.5 h post gavage, samples were diluted 1:5 and fluorescence was measured using a previously determined standard curve, 0.9% saline solution was used as a blank and measured at gain 70. No significant differences were observed between control and FR chickens. In contrast, in the optimized method, chickens receiving 8.32 mg/kg FITC-d, serum samples were collected 1 h post gavage, were diluted 1:5, non-FITC-d serum was used as a blank, a standard curve was developed for each plate and a reading of gain 40, showed significant differences between control and FR chickens (Figure 1; Table 5).
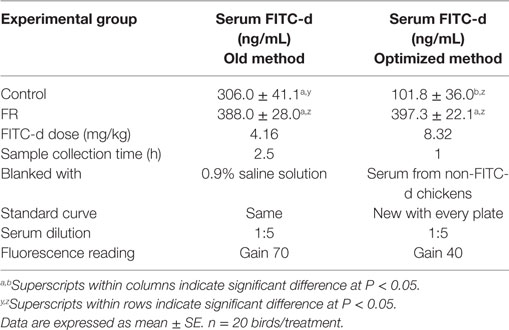
Table 5. Comparing old method versus optimized method of serum fluorescein isothiocyanate dextran (FITC-d) in a 24-h feed restriction (FR) model to induce gut permeability in broiler chickens (Experiment 4).
Discussion
Stress is known to affect gastrointestinal tract (GIT) homeostasis by altering gut motility, permeability, as well as alterations in ion, fluid, and mucus secretion and absorption (18–21). Several investigators have reported that acute or chronic stress modifies gut permeability associated with a temporary redistribution of TJ proteins (22–25). Some of these alterations are linked to mast cells in the brain–gut axis which secrete several neurotransmitters and pro inflammatory cytokines, with profound effects on GIT physiology (26–28). Another hormone that increases during acute or chronic stress is corticotrophin-releasing factor, which increases intestinal paracellular permeability via mast cell-dependent release of TNF-α and proteases (29–31). Moreover, excessive cortisol may lead to GIT disturbances, opportunistic infections, and impaired wound healing (32–34). Due to intensive selection, modern chickens are the most efficient meat-producing animals because of their fast growth, supported by a virtually unlimited voluntary feed intake. However, these features also cause many problems in breeder hens because of the negative correlation between muscle growth and reproduction effectiveness. Hence, commercial restricted feeding programs in broiler breeders have been implemented, with negative effects on welfare and health, as birds are continuously hungry (35). Previous research in poultry has shown that FR increases plasma levels of corticosterone causing disruption of gut barrier integrity, systemic, and local inflammation (36–39). Similarly, we have previously shown in poultry that intestinal barrier function can be adversely affected by stress, poorly digested diets (7, 8), or FR (12, 13), resulting in increased intestinal inflammation-associated permeability. In those studies, we have described a correlation of liver bacterial translocation and serum concentrations of FITC-d as markers used to measure TJ permeability. FITC-d is a 3–5 kDa marker used to measure TJ permeability in chickens using enteric inflammation models. However, inconsistent results obtained from unpublished data suggested that current FITC-d methodology required further optimization. FITC-d has also been reported to be a viable method to measure enteric leakage in the murine model (40). However, they used a different methodology. Therefore, FITC-d methodology may vary with the animal model and this should be taken into consideration when using it to measure gut permeability. The results of the present study suggest that by increasing the dose of FITC-d (8.32 versus 4.16 mg/kg); shortening the collection time of the blood (1 versus 2.5 h); using a pool of non-FITC-d serum as a blank, compared to previously used 0.9% saline; generating a standard curve with every plate to set a limit of detection and modifying the software’s optimal sensitivity value, it is possible to obtain more consistent and reliable results when measuring gut leakage in poultry.
Ethics Statement
All animal handling procedures were in compliance with Institutional Animal Care and Use Committee at the University of Arkansas.
Author Contributions
MB, RM-G, JL, and GT contributed to the overall study design and supervised all research. BM, YY, KT, LG, and AW carried out the experiments and acquisition of data. MB and GT drafted and revised the first version of the manuscript. JL and GT analyzed the data. LB, XH-V, BH, and GT drafted the article and revised it critically for important intellectual content. XH-V and GT were responsible for the final editing of the manuscript. All the authors reviewed and finally approved the manuscript.
Conflict of Interest Statement
The authors declare that the research was conducted in the absence of any commercial or financial relationships that could be construed as a potential conflict of interest.
Acknowledgments
This research was supported by the Arkansas Bioscience Institute under the project: development of an avian model for evaluation early enteric microbial colonization on the gastrointestinal tract and immune function.
References
1. Salzman NH. Microbiota-immune system interaction: an uneasy alliance. Curr Opin Microbiol (2011) 14:99–105. doi: 10.1016/j.mib.2010.09.018
2. Elson CO, Cong Y. Host-microbiota interactions in inflammatory bowel disease. Gut Microbes (2012) 3:332–44. doi:10.4161/gmic.20228
3. Salminen S, Isolauri E. Intestinal colonization, microbiota, and probiotics. J Pediatrics (2006) 149:S115–20. doi:10.1016/j.jpeds.2006.06.062
4. Neish AS. Microbes in gastrointestinal health and disease. Gastroenterology (2009) 136:65–80. doi:10.1053/j.gastro.2008.10.080
5. Seki E, Schnabl B. Role of innate immunity and the microbiota in liver fibrosis: crosstalk between the liver and gut. J Physiol (2012) 590:447–58. doi:10.1113/jphysiol.2011.219691
6. Ilan Y. Leaky gut and the liver: a role for bacterial translocation in nonalcoholic steatohepatitis. World J Gastroenterol (2012) 18:2609–18. doi:10.3748/wjg.v18.i21.2609
7. Tellez G, Latorre JD, Kuttappan VA, Hargis BM, Hernandez-Velasco X. Rye affects bacterial translocation, intestinal viscosity, microbiota composition and bone mineralization in turkey poults. PLoS One (2015) 10(4):e0122390. doi:10.1371/journal.pone.0122390
8. Tellez G, Latorre JD, Kuttappan VA, Kogut MH, Wolfenden A, Hernandez-Velasco X, et al. Utilization of rye as energy source affects bacterial translocation, intestinal viscosity, microbiota composition, and bone mineralization in broiler chickens. Front Genet (2014) 5:339. doi:10.3389/fgene
9. Vicuña E, Kuttappan V, Galarza-Seeber R, Latorre J, Faulkner O, Hargis B, et al. Effect of dexamethasone in feed on intestinal permeability, differential white blood cell counts, and immune organs in broiler chicks. Poult Sci (2015) 94(9):2075–80. doi:10.3382/ps/pev211
10. Kuttappan V, Berghman L, Vicuña E, Latorre J, Menconi A, Wolchok J, et al. Poultry enteric inflammation model with dextran sodium sulfate mediated chemical induction and feed restriction in broilers. Poult Sci (2015) 94(6):1220–6. doi:10.3382/ps/pev114
11. Menconi A, Hernandez-Velasco X, Vicuña E, Kuttappan V, Faulkner O, Tellez G, et al. Histopathological and morphometric changes induced by a dextran sodium sulfate (DSS) model in broilers. Poult Sci (2015) 94(5):906–11. doi:10.3382/ps/pev054
12. Vicuña E, Kuttappan V, Tellez G, Hernandez-Velasco X, Seeber-Galarza R, Latorre J, et al. Dose titration of FITC-d for optimal measurement of enteric inflammation in broiler chicks. Poult Sci (2015) 94(6):1353–9. doi:10.3382/ps/pev111
13. Kuttappan VA, Vicuña EA, Latorre JD, Wolfenden AD, Téllez GI, Hargis BM, et al. Evaluation of gastrointestinal leakage in multiple enteric inflammation models in chickens. Front Vet Sci (2015) 2:66. doi:10.3389/fvets.2015.00066
14. Yan Y, Kolachala V, Dalmasso G, Nguyen H, Laroui H, Sitaraman SV, et al. Temporal and spatial analysis of clinical and molecular parameters in dextran sodium sulfate induced colitis. PLoS One (2009) 4(6):e6073. doi:10.1371/journal.pone.0006073
15. Latorre J, Hernandez-Velasco X, Bielke L, Vicente J, Wolfenden R, Menconi A, et al. Evaluation of a Bacillus direct-fed microbial candidate on digesta viscosity, bacterial translocation, microbiota composition and bone mineralisation in broiler chickens fed on a rye-based diet. Br Poult Sci (2015) 56(6):723–32. doi:10.1080/00071668.2015.1101053
16. Latorre JD, Hernandez-Velasco X, Kogut MH, Vicente JL, Wolfenden R, Wolfenden A, et al. Role of a Bacillus subtilis direct-fed microbial on digesta viscosity, bacterial translocation, and bone mineralization in turkey poults fed with a rye-based diet. Front Vet Sci (2014) 2014(1):26. doi:10.3389/fvets.2014.00026
18. Alverdy J, Aoys E. The effect of glucocorticoid administration on bacterial translocation. Evidence for an acquired mucosal immunodeficient state. Ann Surg (1991) 214:719–23. doi:10.1097/00000658-199112000-00012
19. Collins SM, Bercik P. The relationship between intestinal microbiota and the central nervous system in normal gastrointestinal function and disease. Gastroenterology (2009) 136:2003–14. doi:10.1053/j.gastro.2009.01.075
20. Verbrugghe E, Boyen F, Van Parys A, Van Deun K, Croubels S, Thompson A, et al. Stress induced Salmonella typhimurium recrudescence in pigs coincides with cortisol induced increased intracellular proliferation in macrophages. Vet Res (2011) 42:118. doi:10.1186/1297-9716-42-118
21. Karavolos MH, Winzer K, Williams P, Khan C. Pathogen espionage: multiple bacterial adrenergic sensors eavesdrop on host communication systems. Mol Microbiol (2013) 87:455–65. doi:10.1111/mmi.12110
22. Maejima K, Deitch E, Berg RD. Bacterial translocation from the gastrointestinal tracts of rats receiving thermal injury. Infect Immun (1984) 43:6–10.
23. Assimakopoulos SF, Gogos C, Labropoulou-Karatza C. Could antioxidants be the “magic pill” for cirrhosis-related complications? A pathophysiological appraisal. Med Hypotheses (2011) 77:419–23. doi:10.1016/j.mehy.2011.05.034
24. Koh TS, Peng RK, Klasing KC. Dietary copper level affects copper metabolism during lipopolysaccharide-induced immunological stress in chicks. Poult Sci (1996) 75:867–72. doi:10.3382/ps.0750867
25. Matter K, Balda MS. Epithelial tight junctions, gene expression and nucleo-junctional interplay. J Cell Sci (2007) 120:1505–11. doi:10.1242/jcs.005975
26. Lamprecht M, Frauwallner A. Exercise, intestinal barrier dysfunction and probiotic supplementation. Med Sport Sci (2012) 59:47–56. doi:10.1159/000342169
27. Bailey MT, Dowd SE, Galley JD, Hufnagle AR, Allen RG, Lyte M. Exposure to a social stressor alters the structure of the intestinal microbiota: implications for stressor-induced immunomodulation. Brain Behav Immun (2011) 25(3):397–407. doi:10.1016/j.bbi.2010.10.023
28. Groschwitz KR, Hogan SP. Intestinal barrier function: molecular regulation and disease pathogenesis. J Allergy Clin Immunol (2009) 124(1):3–20. doi:10.1016/j.jaci.2009.05.038
29. Taché Y, Perdue MH. Role of peripheral CRF signalling pathways in stress-related alterations of gut motility and mucosal function. Neurogastroenterol Motil (2004) 16:137–42. doi:10.1111/j.1743-3150.2004.00490.x
30. Teitelbaum AA, Gareau MG, Jury J, Yang PC, Perdue MH. Chronic peripheral administration of corticotropin-releasing factor causes colonic barrier dysfunction similar to psychological stress. Am J Physiol Gastrointest Liver Physiol (2008) 295:G452–9. doi:10.1152/ajpgi.90210.2008
31. Overman EL, Rivier JE, Moeser AJ. CRF induces intestinal epithelial barrier injury via the release of mast cell proteases and TNF-α. PLoS One (2012) 7(6):e39935. doi:10.1371/journal.pone.0039935
32. Moeser AJ, Ryan KA, Nighot PK, Blikslager AT. Gastrointestinal dysfunction induced by early weaning is attenuated by delayed weaning and mast cell blockade in pigs. Am J Physiol Gastrointest Liver Physiol (2007) 293:G413–21. doi:10.1152/ajpgi.00304.2006
33. Smith F, Clark JE, Overman BL, Tozel CC, Huang JH, Rivier JE, et al. Early weaning stress impairs development of mucosal barrier function in the porcine intestine. Am J Physiol Gastrointest Liver Physiol (2010) 298:G352–63. doi:10.1152/ajpgi.00081.2009
34. Galley JD, Bailey MT. Impact of stressor exposure on the interplay between commensal microbiota and host inflammation. Gut Microbes (2014) 5(3):390–6. doi:10.4161/gmic.28683
35. Decuypere E, Bruggeman V, Everaert N, Li Y, Boonen R, De Tavernier J, et al. The broiler breeder paradox: ethical, genetic and physiological perspectives, and suggestions for solutions. Br Poult Sci (2010) 51:569–79. doi:10.1080/00071668.2010.519121
36. Khajavi M, Rahimi S, Hassan Z, Kamali M, Mousavi T. Effect of feed restriction early in life on humoral and cellular immunity of two commercial broiler strains under heat stress conditions. Br Poult Sci (2003) 44:490–7. doi:10.1080/000071660310001598328
37. De Jong IC, van Voorst AS, Blokhuis HJ. Parameters for quantification of hunger in broiler breeders. Physiol Behav (2003) 78:773–83. doi:10.1016/S0031-9384(03)00058-1
38. Abu-Dieyeh Z. Effect of chronic heat stress and long-term feed restriction on broiler performance. Int J Poult Sci (2006) 5:185–90. doi:10.3923/ijps.2006.185.190
39. Hangalapura B, Nieuwland M, Reilingh GDV, Buyse J, Van Den Brand H, Kemp B, et al. Severe feed restriction enhances innate immunity but suppresses cellular immunity in chicken lines divergently selected for antibody responses. Poult Sci (2005) 84:1520–9. doi:10.1093/ps/84.10.1520
Keywords: broiler chickens, enteric inflammation, fluorescein isothiocyanate dextran, feed restriction, gut permeability
Citation: Baxter MFA, Merino-Guzman R, Latorre JD, Mahaffey BD, Yang Y, Teague KD, Graham LE, Wolfenden AD, Hernandez-Velasco X, Bielke LR, Hargis BM and Tellez G (2017) Optimizing Fluorescein Isothiocyanate Dextran Measurement As a Biomarker in a 24-h Feed Restriction Model to Induce Gut Permeability in Broiler Chickens. Front. Vet. Sci. 4:56. doi: 10.3389/fvets.2017.00056
Received: 13 February 2017; Accepted: 03 April 2017;
Published: 19 April 2017
Edited by:
Carl James Yeoman, Montana State University, USAReviewed by:
Geetha Samak, DVS College of Arts and Science, IndiaAndrew Patterson, Pennsylvania State University, USA
Copyright: © 2017 Baxter, Merino-Guzman, Latorre, Mahaffey, Yang, Teague, Graham, Wolfenden, Hernandez-Velasco, Bielke, Hargis and Tellez. This is an open-access article distributed under the terms of the Creative Commons Attribution License (CC BY). The use, distribution or reproduction in other forums is permitted, provided the original author(s) or licensor are credited and that the original publication in this journal is cited, in accordance with accepted academic practice. No use, distribution or reproduction is permitted which does not comply with these terms.
*Correspondence: Guillermo Tellez, gtellez@uark.edu