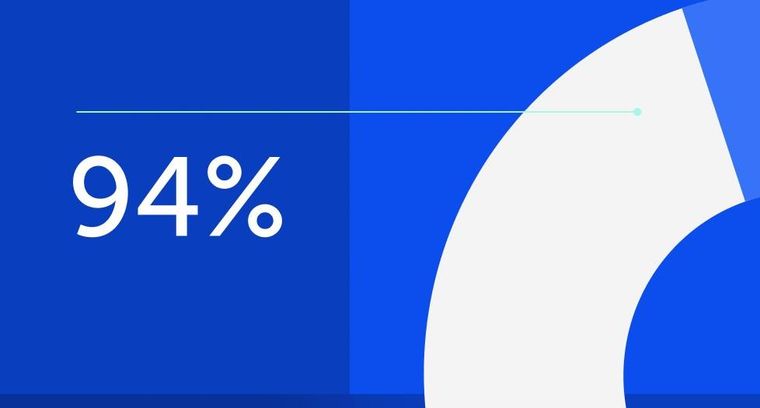
94% of researchers rate our articles as excellent or good
Learn more about the work of our research integrity team to safeguard the quality of each article we publish.
Find out more
ORIGINAL RESEARCH article
Front. Toxicol., 13 December 2023
Sec. Developmental and Reproductive Toxicology
Volume 5 - 2023 | https://doi.org/10.3389/ftox.2023.1264238
This article is part of the Research TopicEarly-life Exposure to Environmental Pollutants: Effects on Fetal Growth, Child Development, and Health in Later LifeView all 3 articles
Background: Prenatal exposures to endocrine disrupting chemicals (EDCs) are correlated with adverse behavioral outcomes, but the effects of combinations of these chemicals are unclear. The aim of this study was to determine the dose-dependent effects of prenatal exposure to EDCs on male and female behavior.
Methods: Pregnant Sprague-Dawley rats were orally dosed with vehicle, bisphenol A (BPA) (5 μg/kg body weight (BW)/day), low-dose (LD) diethylhexyl phthalate (DEHP) (5 μg/kg BW/day), high-dose (HD) DEHP (7.5 mg/kg BW/day), a combination of BPA and LD-DEHP (B + D (LD)), or a combination of BPA and HD-DEHP (B + D (HD)) on gestational days 6–21. Adult offspring were subjected to the Open Field Test (OFT), Elevated Plus Maze (EPM), and Shock Probe Defensive Burying test (SPDB) in adulthood. Body, adrenal gland, and pituitary gland weights were collected at sacrifice. Corticosterone (CORT) was measured in the serum.
Results: Female EDC-exposed offspring showed anxiolytic effects in the OFT, while male offspring were unaffected. DEHP (HD) male offspring demonstrated a feminization of behavior in the EPM. Most EDC-exposed male offspring buried less in the SPDB, while their female counterparts showed reduced shock reactivity, indicating sex-specific maladaptive alterations in defensive behaviors. Additionally, DEHP (LD) males and females and B + D (LD) females displayed increased immobility in this test. DEHP (LD) alone and in combination with BPA led to lower adrenal gland weights, but only in male offspring. Finally, females treated with a mixture of B + D (HD) had elevated CORT levels.
Conclusion: Prenatal exposure to BPA, DEHP, or a mixture of the two, affects behavior, CORT levels, and adrenal gland weights in a sex- and dose-dependent manner.
The global rise in the prevalence of neuropsychiatric disorders can be partially attributed to the widespread use of endocrine disrupting chemicals (EDCs) (Kajta and Wójtowicz, 2013; Mustieles et al., 2015). These environmental contaminants have been repeatedly associated with reproductive (Sifakis et al., 2017), metabolic (Janardhanan, 2018), and developmental (Mouritsen et al., 2010) abnormalities. Some of the most prevalent EDCs in the environment are the plasticizers bisphenol A (BPA) and diethylhexyl phthalate (DEHP), which are typically found in water bottles and food can linings (Chapin et al., 2008), medical devices (Erythropel et al., 2014), and personal care products (Rowdhwal and Chen, 2018). Humans are primarily exposed to these particular EDCs via ingestion of contaminated food and beverages containing BPA and/or DEHP that has leached from the plastics (Kang et al., 2006; Erythropel et al., 2014).
In pregnant females, these chemicals can readily cross the placental barrier and affect fetal brain development in utero (Singh et al., 1975; Balakrishnan et al., 2010). Additionally, there appears to be a sex bias in the prevalence of multiple neuropsychiatric disorders (Ramtekkar et al., 2010; Altemus et al., 2014; Baio et al., 2018). It is highly likely that sex differences in the risk for developing these disorders may stem from exposures to EDCs in utero, a sensitive critical period of fetal development (Mallozzi et al., 2016).
Exposure to BPA during the in utero and early postnatal stages exerts a variety of sex-specific effects on stress-related behaviors and hormones, including corticosterone (CORT). Low-dose prenatal BPA exposure (2–200 μg/kg/day) induces anxiogenic effects and reduces locomotor activity in the Open Field Test (OFT) in female mouse offspring; however, the reverse is observed in males (Kundakovic et al., 2013). These effects are compounded with increasing doses, suggesting a dose-dependent response. Furthermore, perinatal treatment with 5 μg/kg of BPA eliminates sex differences in rats in the Elevated Plus Maze (EPM), without affecting learning and memory in the Morris Water Maze (Jones and Watson, 2012). In addition, studies report increased basal and post-stress levels of CORT accompanied by increased anxiety-like behavior in female rat offspring with perinatal BPA exposure (40 μg/kg/day) (Poimenova et al., 2010). BPA-exposed male offspring, on the other hand, display an even higher post-stress CORT response than females. However, perinatal treatment with a lower BPA dose (2 μg/kg/day) can induce anxiolytic effects in female rat offspring without affecting CORT levels before or after stress exposure (Chen et al., 2014). Yet their BPA-exposed male counterparts have persistently heightened pre- and post-stress CORT concentrations. The variability observed in BPA effects across studies may be attributed to methodological differences, including window and route of BPA exposure, the species studied, and age of offspring at evaluation.
Perinatal DEHP appears to have a non-monotonic dose response on exploratory and locomotor activity. Low DEHP doses (5–400 μg/kg/day) are associated with increased home cage exploration in mouse offspring of both sexes (Quinnies et al., 2017), whereas higher doses (10–200 mg/kg/day) lead to decreased locomotor activity in the OFT in female mice (Xu et al., 2015). Anxiogenic effects in the OFT and EPM are additionally observed in both male and female mice following low-dose (5 and 40 μg/kg) (Quinnies et al., 2017), as well as high-dose (50–750 mg/kg) (Dai et al., 2015; Xu et al., 2015; Barakat et al., 2018) perinatal DEHP exposure. Moreover, male offspring appear to show increased dose-dependent deficits in learning and memory following prenatal DEHP exposure (Lin et al., 2015; Barakat et al., 2018). Although no changes were observed in CORT in male offspring with high-dose DEHP exposure (Martinez-Arguelles et al., 2011), prenatal treatment with 150 mg/kg of DEHP has been shown to transgenerationally reduce CORT concentrations in female mouse offspring instead (Quinnies et al., 2015). Thus, it appears that alterations in CORT levels in EDC-exposed offspring depend on the type of EDC and dose used.
An especially problematic aspect of these contaminants is that, in reality, they exist in combination with one another in the environment–as mixtures. Determining the mechanisms of action underlying EDC mixtures is a relatively novel area of research, but studies agree that EDC mixture effects are more elusive and vary from those of individual EDCs (Biemann et al., 2014; Suteau et al., 2020). EDC mixtures, including BPA and DEHP combinations, are associated with a variety of consequences on the metabolic (Naville et al., 2013; Labaronne et al., 2017), reproductive (Borman et al., 2017; Balci et al., 2020; Christiansen et al., 2020), and cardiovascular (Shang et al., 2019) systems in male and female rodents. Yet, there is an alarming lack of data on sex-specific outcomes on behavior following exposures to BPA and DEHP mixtures.
The objective of the present study was to assess sex differences in stress-related behaviors, cognition, and the hypothalamic-pituitary-adrenal (HPA) axis following prenatal BPA or DEHP exposure individually or in combination at low and high doses. Our hypothesis was that prenatal EDC exposure, particularly in combination, would induce sex-specific and dose-dependent alterations in behavioral responses to stress that are mediated by the HPA axis.
Adult female Sprague-Dawley (SD) rats were purchased from Envigo (Indianapolis, IN) and housed in rooms that were light- (12:12 light-dark cycle) and temperature-controlled (23.2°C ± 2°C, 50% ± 20% relative humidity) at the University of Georgia. Food and water were provided ad libitum. The rats were fed Pico Lab Rodent Diet 20 (LabDiet). Animals were housed in polycarbonate cages with corn cob bedding. Bisphenol exposures from the environment (cages, water bottles, etc.) were not controlled for since all animals were maintained in the same environment. After a week of acclimation, each of the female breeders underwent vaginal cytology for 10 consecutive days to track their individual estrous cycles. Once in proestrus, a female was randomly assigned a male by generating random numbers using the standard = RAND () function in Microsoft Excel, and the two were co-housed for 1 day. The presence of a vaginal plug was used to confirm the occurrence of mating. Gestational day (GD) 0 represented the day of copulation.
BPA (Lot MKBH 2096V; Catalog No. 239658; Purity: ≥99.0%) and DEHP (Lot BCBR8079V; Catalog No. 36735; Purity: ≥98.0%) were purchased from Sigma Aldrich (St. Louis, MO). Stock solutions were made in dimethylsulfoxide (DMSO; 1 μg/μL for BPA and low dose DEHP and 1 mg/μL for high dose DEHP). Doses were calculated daily based on BW and mixed with 20 µL Phosphate Buffered Saline (PBS) for oral dosing. The final concentration of DMSO in the daily dose was less than 10%. Daily oral dosing occurred from GD 6–21 as per our previously published study (Dagher et al., 2021). The vehicle (20 µL of PBS) or EDC treatments were discharged into the oral cavity using a micropipette to avoid any local irritation to the gastrointestinal tract and potential stress to the pregnant dam.
Since BPA has been studied extensively, we used it as a positive control and therefore only tested the effects of a single low dose. The BPA dose was selected because it is significantly lower than the Environmental Protection Agency (EPA) recommended no-observed-adverse-effect-level (NOAEL) dose of 5 mg/kg/day (EPA, 2010) and it is also 10-fold below the current daily reference dose of 50 μg/kg/day (Almeida et al., 2018). Additionally, this dose is within the estimated range of BPA exposure in humans (0.4–5 μg/kg/day) (Lakind and Naiman, 2008). The high dose of DEHP was selected since it is higher than the established NOAEL dose of 4.8 mg/kg/day (Blystone et al., 2010), whereas the low DEHP dose is significantly lower than this. The low dose of DEHP used in this study lies within the range of the typical daily intake of this chemical in adult humans (1–30 μg/kg/day) (Shelby, 2006), but is well below the EPA reference dose of 20 μg/kg/day (EPA, 1987).
The experimental design is demonstrated in Figure 1. The dam was considered the experimental unit. Each dam was randomly assigned to one of 6 different treatment groups: control (20 µL PBS; n = 6), BPA (5 μg/kg BW/day; n = 7), low-dose (LD) DEHP (5 μg/kg BW/day; n = 6), high-dose (HD) DEHP (7.5 mg/kg BW/day; n = 6), a combination of BPA and LD DEHP (5 μg/kg/day of BPA +5 μg/kg/day of DEHP; n = 6), and a combination of BPA and HD DEHP (5 μg/kg/day of BPA +7.5 mg/kg/day of DEHP; n = 7). Generation of random assignment numbers was completed using the standard = RAND () function in Microsoft Excel. Litters were equalized in number prior to weaning. No differences in developmental trajectory were observed in the mothers and offspring (Dagher et al., 2021). One male pup and one female pup from each dam then underwent behavioral testing when they were 3–4 months of age. This was immediately followed by euthanasia and collection of trunk blood and certain organs for further processing.
FIGURE 1. Summary of the experimental design of the study. Pregnant Sprague-Dawley dams were orally dosed daily from gestational days (GD) 6–21 with vehicle (Control) (20 µL PBS; n = 6), BPA (5 μg/kg/day; n = 7), low-dose (LD) DEHP (5 μg/kg/day; n = 6), high-dose (HD) DEHP (7.5 mg/kg/day; n = 6), a mixture of BPA + LD DEHP (5 μg/kg/day of BPA +5 μg/kg/day of DEHP; n = 6), or a mixture of BPA + HD DEHP (5 μg/kg/day of BPA +7.5 mg/kg/day of DEHP; n = 7). Adult male and female offspring aged 3 months or older were administered a battery of behavioral tests once, following which they were immediately euthanized. Pituitary and adrenal glands were dissected and weighed upon euthanasia. Trunk blood was collected for the measurement of serum CORT using radioimmunoassay. Experimental design schematic was created using Biorender. com. Note: EDC, endocrine disrupting chemical; PBS, Phosphate Buffered Saline; BPA, Bisphenol A; DEHP, diethylhexyl phthalate; LD, low-dose; HD, high-dose; BW, body weight; CORT, corticosterone.
The adult male and female offspring of the treated dams were transferred to another facility on campus 1 week prior to behavioral testing, where they remained undisturbed during this habituation period for a week. Animals were group housed (two to four rats per cage) with rats of the same sex and dose group in polycarbonate cages with corn cob bedding. Rooms were maintained at 23.3°C ± 3°C on a 12:12 reverse light-dark cycle. All behavioral testing occurred during the dark cycle. All animals had access to food and water ad libitum in their home cages, including before and after each testing session.
The animals were administered a battery of behavioral tests including the Open Field Test (OFT), Elevated Plus Maze (EPM), and Shock Probe Defensive Burying (SPDB). The Novel Object Recognition test (NOR) was also administered, but only to the animals in the LD group since these offspring demonstrated more intriguing behavioral effects and we wanted to examine how their cognition was affected as a result. The order for the tests was OFT, EPM, SPDB, followed by NOR. The tests were administered in succession and each rat was exposed to each test only once. Rats were then euthanized after completion of the behavioral testing. Both testing and euthanasia for each rat occurred in the same day.
The animals’ behaviors in each test were video recorded by a direct overhead webcam (Microsoft), and all videos were manually scored by experimenters unaware of the treatment groups. Rats were habituated to the testing areas for 5 min prior to testing. They remained in their cages during this time. All boxes and equipment were disinfected between every trial. Vaginal smears were obtained from all female rats for 2–10 days prior to behavioral testing to ensure that females were tested when they were in estrus.
Each animal was placed in a transparent plexiglass test chamber (43.3 cm long x 43.3 cm wide x 30.5 cm high) consisting of a center zone and a perimeter zone (Hooversmith et al., 2019). The area considered the center zone measured 25.3 cm2. At the beginning of the testing session, all rats were placed in the lower left corner of the box facing the opposite wall. The animal was allowed to freely explore the box for 10 min (Hooversmith et al., 2019). OFT behaviors were automatically recorded using Activity Monitor software (Med Associates, Fairfax, VT, United States) on a desktop computer to automate behavioral testing and provide unbiased analyses of data. The following measures were recorded during the testing session: number of entries and time spent in the center and perimeter zones, frequency and time spent rearing, as well as distance traveled, and time spent ambulating within the box. Animals were tested in the OFT under red lights.
The testing apparatus consisted of a wooden maze painted black matte with two pairs of arms set perpendicular to each other and placed 50 cm above the floor. The arms extended from a central platform (9 cm2) and formed a pair of open arms (45 × 9 cm) and a pair of closed arms (45 × 9 × 38 cm) (Simone et al., 2015). The open arms were not bound by walls and the closed arms were enclosed by high walls and no ceilings. Animals were tested under red lights. To begin the testing session, the animal was placed on the central platform facing an open arm opposite the experimenter. The number of entries and time spent in each arm and crosses through the central platform were recorded during the 5-min testing session (Sciolino et al., 2012; Balasubramanian et al., 2014). Entry into an arm was considered as the presence of all four feet of the rat in the arm.
Animals were placed in a covered clear polycarbonate cage (20 × 40 × 20 cm) containing bedding at the beginning of the testing session. An electrified probe extended 6 cm into the cage and 2 cm above the bedding (Simone et al., 2015). The experimenter administered a mild shock of 3 mA DC (E13-08, Coulbourn Instruments, Allentown, PA) to the animal after initial contact with the probe. The intensity of the animal’s response to the shock, or shock reactivity, on a scale of one to four was manually recorded: 1—Flinch involving only head or forepaw, without immediate ambulation away from the probe; 2—Whole-body flinch and ambulation to far end of chamber; 3—Hopping away and running; 4—Jumping away and running. Following this, the frequency and time spent engaging in the following behaviors were measured during the 10-min testing session: burying, immobility, rearing, exploring the shock-probe, and grooming. Testing occurred under red lights. After returning the rat to its home cage at the end of the session, the height of the highest point of the bedding (representing the ending height) was measured and manually recorded.
The test chambers had opaque walls with no ceiling (52 × 35 × 32.5 cm, Sterlite). A variety of objects that varied in size (maximum size: 10.5 cm high x 18.5 cm wide), shape, and material (plastic, glass, metal, and ceramic) were used. These particular objects were selected because they were previously tested for object preference bias by Simone et al. (Simone et al., 2015), and no significant differences were found in bias or exploration of these objects. All objects were glued to a small jar that was fixed in place by screwing the jar to a lid within the box. The test consisted of the following procedure: 1) The rat was allowed to freely explore two randomized identical objects for 5 min during the familiarization phase (T1). Randomization was completed using a computer based random number generator. 2) The rat was returned to its home cage with food and water for 45 min during the retention phase. 3) The rat was returned to the test chamber for 3 min for the test phase (T2), in which one of the identical objects from T1 was replaced with a novel object. The other identical T1 object was replaced with a duplicate object. The novel object and locations of the objects in T2 were counterbalanced across all rats. Animals were tested in the NOR under red lights.
For the T1 phase, the average exploration time was measured, and the discrimination index (DI) was calculated. Exploration was defined as sniffing the object within 2 cm from the edge or touching the object. Climbing on/over or rearing on the objects were not counted as part of the exploration time. DI in this phase was defined as the difference in exploration time between the right object and left object divided by the total exploration time of the right and left objects (Aubele et al., 2008). For the T2 phase, we calculated both the DI and the recognition index (RI). DI in this phase was defined as the difference in exploration time between the novel object and familiar object divided by the total exploration time of the novel and familiar objects (Ennaceur and Delacour, 1988). Finally, the RI was considered the main index of novel object recognition in our study because it is a more sensitive measure of recognition memory compared to the DI, which may be influenced by differences in exploration levels across animals (Akkerman et al., 2012; Antunes and Biala, 2012). The RI was defined as the percent of time spent exploring the novel object relative to the total amount of time spent exploring both the novel and familiar objects (Mumby et al., 2002; Botton et al., 2010).
Immediately following behavioral testing, female offspring in estrus (as confirmed by vaginal cytology) and male offspring were euthanized by rapid decapitation. Pituitary glands and adrenal glands were dissected, weighed, and stored at −80°C for further processing.
Following the sacrifice of the rats, trunk blood was collected, centrifuged, and serum was separated and stored at −80°C. Serum corticosterone levels were measured in duplicate using a double antibody radioimmunoassay (MP Biomedicals, Santa Ana, CA; SKU:07120121), according to the manufacturer’s protocol. Values were expressed as ng/mL.
Prism 9.0.0 (GraphPad, Inc.) software was used to perform statistical analyses. Behavioral data, organ weights, and corticosterone levels were analyzed by two-way ANOVA (EDC exposure × sex). Interaction effects between EDC exposure and sex were also assessed. One-way ANOVA analyses were further used to identify main effects of EDC exposure separately by sex. Statistical differences between control and EDC groups in behavioral parameters were measured using Fisher’s LSD post hoc test. Differences between control and EDC groups in body weights, organ weights, and corticosterone levels were analyzed using Tukey’s multiple comparisons post hoc test. Values that were statistical outliers were excluded from all of the analyses. p-value <0.05 was considered to indicate a statistically significant difference. Data was expressed as mean ± standard error of mean (SEM).
No significant differences were observed in distance traveled (Figures 2E,F) or time spent (Table 1) ambulating within the chamber in males or females from any of the groups. A significant main effect of sex (F (1, 58) = 8.4, p = 0.0054) was observed in rearing frequency (mean ± SEM) (Table 1). DEHP (HD) females (114.0 ± 29.7) displayed a marked increase in rearing compared to their male counterparts (53.2 ± 2.7; p = 0.002), suggesting a possible anxiety-like response in DEHP (HD) females. No additional differences were found in this measure, or in rearing time (Table 1).
FIGURE 2. Behavioral effects of prenatal exposure to vehicle or EDCs in female and male rat offspring in the open field test (OFT). (A) Center zone time in female offspring, (B) center zone time in male offspring, (C) perimeter zone entries in female offspring, (D) perimeter zone entries in male offspring, (E) locomotor activity in female offspring, and (F) locomotor activity in male offspring. Behavioral data were collected from adult male and female offspring prenatally exposed to vehicle (Control) (males: n = 6; females: n = 5–6), BPA (males: n = 6; females: n = 7), DEHP (LD) (males: n = 5–6; females: n = 6), DEHP (HD) (males: n = 5–6; females: n = 6), a mixture of BPA + DEHP (LD) (males: n = 5; females: n = 6), or a mixture of BPA + DEHP (HD) (males: n = 7; females: n = 6). Data were analyzed by one-way ANOVA, followed by Fisher’s LSD post hoc test. *p < 0.05, **p < 0.01, comparison between control and EDC-exposed female offspring. Error bars represent the standard error of the mean (SEM).
TABLE 1. Behavioral data of male and female offspring following low-dose (5 µg) and high-dose (7.5 mg) prenatal EDC exposure.
Figures 2A–D and Table 1 depict the results from the OFT. There was a significant effect of EDC exposure (F (5, 30) = 3.116, p = 0.0221) in the time spent in the center zone (%, mean ± SEM); however, females in the BPA (p = 0.0633) and B + D (LD) (p = 0.05) groups only spent a little more time in the center zone compared to control (Figure 2A), possibly suggesting that they had less anxiety than controls. On the other hand, control (p = 0.0327) and B + D (HD) (p = 0.0518) females spent less time in the center zone compared to their male counterparts, possibly indicating that these females are more anxiety-prone than the corresponding males (Table 1). Additionally, DEHP (LD) (p = 0.0227), DEHP (HD) (p = 0.0042), and B + D (HD) (p = 0.0255) females had fewer entries into the perimeter zone than control females (F (5, 31) = 2.997, p = 0.0262) (Figure 2C), suggesting a possible reduction in anxiety levels in these females. A main effect of sex was additionally determined in perimeter zone entries (F (1, 61) = 5.4, p = 0.0237), with significant differences found only within the BPA group (p = 0.0281) (Table 1). Finally, there was no effect of EDC exposure in the amount of time males (F (5, 29) = 0.2649, p = 0.9286) or females (F (5, 31) = 0.8330, p = 0.5363) spent in the perimeter zone. However, a main effect of sex (F (1, 60) = 16, p = 0.0002) was observed in this measure (Table 1). BPA (p = 0.0267) and B + D (HD) (p = 0.0063) females spent more time in the periphery (118.3% and 128.6% more, respectively) compared to their male counterparts, indicating higher levels of anxiety.
We did not observe any significant effects of EDC exposure on exploration in the EPM in either sex (Figures 3E,F). However, a main effect of sex (F (1, 59) = 17, p = 0.0001) was discovered in the amount of time spent in the central platform of the EPM (Table 1). Furthermore, an interaction effect between EDC exposure and sex (F (5, 59) = 3.0, p = 0.0190) was also determined in the central platform time. Females in a majority of the groups spent significantly more time in the center. Control (p = 0.0010), BPA (p = 0.0069), DEHP (HD) (p = 0.0217), and B + D (HD) (p = 0.0079) females exhibited robust increases (142.4%, 96.5%, 134.3%, 120.0%, respectively) in center time than their male counterparts, which may be interpreted as increased risk assessment of the maze.
FIGURE 3. Behavioral effects of prenatal exposure to vehicle or EDCs in adult male and female rat offspring in the elevated plus maze (EPM). (A) Time spent in open arms relative to total arms in male offspring, (B) time spent in open arms relative to total arms in female offspring, (C) number of entries into open arms relative to total arms in male offspring, (D) number of entries into open arms relative to total arms in female offspring, (E) total exploration of the maze in male offspring, and (F) total exploration of the maze in female offspring. Data were collected from adult male and female offspring prenatally exposed to vehicle (Control) (males: n = 6; females: n = 6), BPA (males: n = 5–6; females: n = 7), DEHP (LD) (males: n = 6; females: n = 5–6), DEHP (HD) (males: n = 6; females: n = 6), a mixture of BPA + DEHP (LD) (males: n = 4–5; females: n = 6), or a mixture of BPA + DEHP (HD) (males: n = 7; females: n = 6). Data were analyzed by one-way ANOVA, followed by Fisher’s LSD post hoc test. *p < 0.05, comparison between control and EDC-exposed male offspring. Error bars represent the standard error of the mean (SEM).
Interaction effects were observed in both open-to-total (OTT) arm time ratio (F (5, 60) = 2.9, p = 0.0221) and OTT arm entries ratio (F (5, 59) = 3.0, p = 0.0188). DEHP (HD) male offspring had increased ratios (mean ± SEM) of OTT arm time (0.6 ± 0.1) (Figure 3A) and OTT arm entries (0.5 ± 0.1) (Figure 3C) compared to control males (OTT time: 0.3 ± 0.1; OTT entries: 0.4 ± 0.1), suggesting that DEHP (HD) males had reduced anxiety. A similar effect was observed in control (p = 0.0477) and BPA (p = 0.0002) females, with them having considerably higher ratios for OTT arm time (64.3% and 195.2%, respectively) compared to their male counterparts. BPA females also demonstrated a higher ratio of OTT arm entries than BPA males (females: 0.6 ± 0.1; males: 0.3 ± 0.0; p = 0.0007). More interestingly, the enhanced OTT arm time and entries in DEHP (HD) males resembled that in control females (OTT time: 0.6 ± 0.1; OTT entries: 0.5 ± 0.1), suggesting a possible feminization of behavior in these males.
An interaction effect was further observed in closed arm time (F (5, 60) = 2.4, p = 0.0444). The corollary of our OTT arm time findings was true in the time spent in the closed arms, with females in the control (p = 0.0197) and BPA (p = 0.0001) groups spending less time compared to the males (Table 1), confirming reduced anxiety in these two groups. Additionally, B + D (HD) females also spent less time in the closed arms (40.7 ± 7.3; p = 0.0506) than their male counterparts (60.5 ± 2.7) (Table 1).
Figure 4 A-D display the results from the SPDB test. A majority of the EDC-treated male offspring demonstrated robust reductions in the amount of time spent burying (%, mean ± SEM) (F (5, 28) = 2.793, p = 0.0361) (Figure 4A). Compared to control males (36.9 ± 12.4), males prenatally exposed to BPA (14.8 ± 8.5; p = 0.0518), DEHP (LD) (0.3 ± 0.3; p = 0.0034), DEHP (HD) (1.8 ± 1.0; p = 0.0046), and B + D (HD) (13.7 ± 5.1; p = 0.0353) spent significantly less time burying the probe, which indicates a decrease in active coping. DEHP (LD) males (37.4 ± 13.2; p = 0.0027) also spent substantially more time immobile than control males (4.2 ± 1.6) (Figure 4C), which is suggestive of passive coping (Fucich et al., 2018). Similar to DEHP (LD) males, DEHP (LD) (p = 0.01) and B + D (LD) (p = 0.0134) females had over 600% increases in immobility time compared to control females (Figure 4D), indicating that they were prone to passive coping as well. No other differences were observed in burying or immobility frequency, rearing frequency or time, probe exploration frequency or time, or grooming frequency or time in male or female offspring (Table 1).
FIGURE 4. Behavioral effects of prenatal exposure to vehicle or EDCs in adult male and female rat offspring in the shock probe defensive burying (SPDB) test. (A) Amount of time spent burying in male offspring, (B) amount of time spent burying in female offspring, (C) amount of time spent immobile in male offspring, and (D) amount of time spent immobile in female offspring. Data were collected from adult male and female offspring prenatally exposed to vehicle (Control) (males: n = 5–6; females: n = 5–6), BPA (males: n = 5–6; females: n = 7), DEHP (LD) (males: n = 5–6; females: n = 6), DEHP (HD) (males: n = 5–6; females: n = 5–6), a mixture of BPA + DEHP (LD) (males: n = 4–5; females: n = 6), or a mixture of BPA + DEHP (HD) (males: n = 6–7; females: n = 5–6). Data were analyzed by one-way ANOVA, followed by Fisher’s LSD post hoc test. *p < 0.05, **p < 0.01, comparison between control and EDC-exposed offspring. Error bars represent the standard error of the mean (SEM).
In addition to these behavioral parameters, a significant EDC effect was observed in female shock reactivity (mean ± SEM) (F (5, 31) = 3.786, p = 0.0086) (Table 1). Females prenatally treated with BPA (1.1 ± 0.1; p = 0.0089), DEHP (LD) (1.0 ± 0.0; p = 0.0018), B + D (LD) (1.0 ± 0.0; p = 0.0018), and B + D (HD) (1.0 ± 0.0; p = 0.0018) displayed moderately decreased shock reactivity in comparison with control females (1.7 ± 0.2), suggesting possible reduction in fear responses. No such differences were observed in males, nor were there differences in bedding height after EDC exposure in both male and female offspring (Table 1).
The results from the NOR are displayed in Figure 5 and Table 1. Two rats were excluded in the EDC-treated groups due to the rats climbing out of the testing box in one or both trials. No significant differences were observed in the EDC-exposed offspring in any of the NOR measures.
FIGURE 5. Behavioral effects of prenatal exposure to vehicle or EDCs in adult male and female rat offspring in the novel object recognition (NOR) test. (A) Object recognition in male offspring and (B) object recognition in female offspring. Data were collected from adult male and female offspring prenatally exposed to vehicle (Control) (males: n = 5; females: n = 6), BPA (males: n = 6; females: n = 5), DEHP (LD) (males: n = 6; females: n = 6), or a mixture of BPA + DEHP (LD) (males: n = 5; females: n = 5). Data were analyzed by one-way ANOVA, followed by Fisher’s LSD post hoc test. Error bars represent the standard error of the mean (SEM).
An interaction effect was observed in CORT levels (F (5, 35) = 8.427, p < 0.0001). BPA in combination with DEHP (HD) exposure significantly increased CORT levels in females compared to controls (p = 0.0006) (Figure 6B). However, male offspring did not demonstrate any alterations in CORT as a result of EDC exposure. Sex differences were further observed in DEHP (LD) (p = 0.0189), B + D (LD) (p = 0.0046), and B + D (HD) (p = 0.0008) offspring. In the LD groups, male offspring had significantly higher CORT concentrations than their female counterparts, but this sex difference was reversed in the B + D (HD) group. No further changes were observed in CORT.
FIGURE 6. Hypothalamic-pituitary-adrenal (HPA) axis activity in adult male and female offspring prenatally exposed to EDCs or mixtures. (A) Serum corticosterone (CORT) levels (ng/mL) measured in male offspring, (B) serum CORT levels (ng/mL) measured in female offspring, (C) adrenal gland relative weights (g/kg BW) in male offspring, and (D) adrenal gland relative weights (g/kg BW) in female offspring. Data were collected from adult male and female offspring prenatally exposed to vehicle (Control) (males: n = 6; females: n = 6), BPA (males: n = 6; females: n = 7), DEHP (LD) (males: n = 6; females: n = 6), DEHP (HD) (males: n = 6; females: n = 6), a mixture of BPA + DEHP (LD) (males: n = 5; females: n = 6), or a mixture of BPA + DEHP (HD) (males: n = 7; females: n = 6). Data were analyzed by one-way ANOVA, followed by Tukey’s multiple comparisons test. *p < 0.05, ***p < 0.001, comparison between control and EDC-exposed male offspring. Error bars represent the standard error of the mean (SEM).
There was a significant EDC exposure effect (F (5, 30) = 3.082, p = 0.0232) in adrenal gland relative weights, with DEHP (LD) (p = 0.0362) and B + D (LD) (p = 0.0267) male offspring having lower weights than corresponding controls (Figure 6C). In contrast, no significant EDC effects were found in female adrenal gland weights (Figure 6D). Furthermore, there were no differences in male or female body weights (BWs) or pituitary gland relative weights due to EDC treatment (Table 2). However, males had higher body weights and lower pituitary gland relative weights, compared to the female offspring. Moreover, females exposed to both low and high doses of DEHP (p = 0.0269 and p = 0.0234, respectively) and B + D (p = 0.0114 and p < 0.0001, respectively) had significantly higher adrenal weights than their male counterparts.
TABLE 2. Sex differences in body and organ weights of male and female offspring following low-dose (5 µg) and high-dose (7.5 mg) prenatal EDC exposure.
In this study, we aimed to determine sex differences in the adverse behavioral and cognitive effects resulting from prenatal EDC exposure. The results of our study revealed a myriad of effects on stress-related behaviors, CORT, and adrenal gland weights. Firstly, BPA-treated female offspring displayed a near-significant increase in time spent in the center zone of the OFT, indicative of decreased anxiety-like behavior. Each of the behavioral paradigms incorporated in our study evaluates distinct aspects of stress-related behaviors, with the OFT assessing exploration of a novel environment (Choleris et al., 2001; Ramos, 2008). Hence, our BPA female offspring showed anxiolytic effects in response to novelty. BPA males, on the other hand, spent less time burying in the SPDB. Burying is an adaptive coping style since it embodies an active coping behavioral response to stress (Hori et al., 2004; Bondi et al., 2007). A decrease in burying represents a maladaptive reduction in active coping mechanisms (Fucich and Morilak, 2018). The BPA females in our study also exhibited altered defensive behaviors, but in the form of reduced shock reactivity in the SPDB. These effects taken together suggest that prenatal exposure to BPA at a low dose of 5 µg may lead to aberrant effects on sex-specific defensive behaviors and fear responses.
Prenatal exposure to DEHP at low and high doses yielded varying effects in each of the behavioral tests. In the OFT, both LD and HD DEHP-treated female offspring had fewer entries into the perimeter zone, but showed no changes in perimeter zone time. While a reduction of entries into the perimeter zone of the OFT may represent an anxiolytic-like effect due to reduced activity in the periphery, changes in exploration of the center zone in this test are typically used to measure anxiety-like behavior (Seibenhener and Wooten, 2015; Scholl et al., 2019). DEHP females were unaffected in the center zone parameters, rendering unclear anxiety-related effects.
DEHP (HD) males exhibited decreased anxiety-like behavior in the EPM, which represents a riskier environment and evaluates unconditioned anxiety (Lapiz-Bluhm et al., 2008; Ramos, 2008). This contrasts with studies that have observed anxiogenic effects in the EPM in males with perinatal low-dose (5–40 µg) and high-dose (10–50 mg) DEHP exposure (Xu et al., 2015; Quinnies et al., 2017). These inconsistencies could be attributed to differences in DEHP exposure period, species tested, and age at evaluation. In the aforementioned studies, DEHP was administered to mice throughout the gestational and early postnatal stages, and increased EPM anxiety levels were observed in pubertal male offspring. The anxiolytic effect in our DEHP (HD) males additionally resembled the behavior of control females. The sex difference between HD males and females in the proportion of time spent in the open arms was also reversed compared to controls, and this particular effect was not noted in any other EDC group. This suggests a potential feminization of HD males in anxiety-like behavior. This is consistent with the anti-androgenic effects of DEHP commonly discerned in exposed males (Moore et al., 2001; Vo et al., 2009a; Vo et al., 2009b; Christiansen et al., 2010; Carbone et al., 2013; Wang et al., 2016). We can further confirm based on our results that this particular effect is not present at a lower DEHP dose of 5 µg.
In the SPDB, we observed robust decreases in burying in LD and HD males in the DEHP-exposed groups. LD males and females also engaged in increased immobility, which was not reflected in HD offspring. In addition, we observed significantly reduced shock reactivity in LD, but not HD, females. These findings imply aberrant changes in defensive behaviors–reduced active coping in DEHP males, regardless of dose, and a definite shift to passive coping in DEHP (LD) male and female offspring. An increase in immobility reflects a preference for passive reactive coping mechanisms (Koolhaas et al., 1999; Fucich and Morilak, 2018), a maladaptive stress response that is often observed in individuals with affective disorders, including depression (Koolhaas et al., 1999; Olff et al., 2005; Southwick et al., 2005; Mahmoud et al., 2012). Immobility can also be interpreted as a measure of anxiety-like behavior (Nijssen and Schelvis, 1987).
Heightened immobility responses in the Forced Swim Test, an indicator of depressive-like behavior, have previously been reported in both male and female offspring perinatally treated with DEHP at high doses (10–200 mg/kg) (Xu et al., 2015). Our study is the first to demonstrate an acute stress-induced passive behavioral phenotype in the SPDB in male and female offspring exposed to low-dose DEHP in utero. Furthermore, our DEHP (LD) males also showed a reduction in adrenal gland relative weights. This is intriguing because adrenal hormones have been implicated in freezing behavior, with adrenalectomy impairing the duration of fear-induced freezing (Bohus, 1987). Hence, the lower adrenal weights in our DEHP (LD) males may have contributed to their behavioral outcomes. Additional experiments should examine mineralocorticoid and glucocorticoid receptor alterations for further insight.
Treatment with BPA and DEHP in combination altered the behavior of female offspring more than male offspring. B + D (LD) females exhibited anxiolytic effects in the OFT, but passive coping responses in the SPDB. B + D (HD) females showed unclear and slightly conflicting anxiety-like behavioral responses as well. In the OFT, they displayed significantly fewer entries into the perimeter zone, but they also spent the least amount of time in the center zone compared to other female offspring. However, this was not significantly different from control females. Moreover, B + D (HD) females had elevated CORT concentrations relative to their control counterparts. CORT levels were not significantly altered in any of the other treatment groups, regardless of sex. Studies have identified higher CORT levels in female offspring with perinatal BPA exposure at 40 μg/kg (Poimenova et al., 2010; Panagiotidou et al., 2014), but blunted CORT levels in female offspring three generations following prenatal high-dose DEHP exposure (150 mg/kg) (Quinnies et al., 2015). To our knowledge, ours is the first study to report a sex-specific increase in CORT following treatment with a mixture of BPA + DEHP. Interestingly, this outcome was not coupled with any robust behavioral changes.
Similarly, B + D (LD) males were not affected in any of the behavioral measures, but instead had lower adrenal gland relative weights, comparable to DEHP (LD) males. This result is particularly striking because it suggests that B + D (LD) male offspring may be more impacted at the organ systems level. Ours is the first study to our knowledge to determine associations between B + D exposure and reductions in these organ weights in male offspring. B + D (HD) males, on the other hand, did show behavioral alterations. They demonstrated significant reductions in active coping in the SPDB, with no accompanying alterations in CORT or adrenal weights. Therefore, the dose of B + D at which male offspring are exposed to determines the nature of the outcomes they will exhibit.
A major limitation of our study was the fact that the pregnant dams were group housed during the EDC treatment period. As a result, any potential contamination of the animals with bisphenol metabolites released through urine or feces in the cages was not controlled for. However, there were no variations in the types of cages used and materials provided within the cages, and all animals were provided water in glass bottles. Therefore, any additional bisphenol exposure from the environment would have been similar across treatments. Measuring the bisphenol levels in the animals and identifying any differences between EDC-exposed and control animals would help determine if there was bisphenol contamination. Moreover, larger sample sizes in all of our groups may have potentially exposed further significant effects, especially in those parameters that were near-significant.
In addition, all of the female offspring in our study were tested while in the estrus stage of the estrous cycle. This stage is characterized by reduced levels of estradiol (E2) and progesterone P), and is generally correlated with lower stress hormone levels relative to the proestrus stage, during which E2 and P levels are higher (Viau and Meaney, 1991). Anxiety-like behavioral responses are therefore influenced by ovarian steroids such as E2 in females (MohanKumar et al., 2018), but the effects vary based on a multitude of factors, including age. A prior study from our lab reported that chronic exposure to low-dose E2 (20 ng/day for 90 days) increases anxiety-like behavior in female rats (Balasubramanian et al., 2014). Studies have also demonstrated that certain anxiety-related behaviors are independent of estrous cycle stage in female rodents (Scholl et al., 2019). Hence, the fact that only estrus females were used in our study is not a major limitation.
To summarize, offspring with prenatal EDC exposures were dose- and sex-specifically affected in each of the behavioral measures assessed. BPA offspring were impacted in anxiety-like and defensive behaviors. DEHP (LD) offspring were more severely affected in terms of defensive behaviors, whereas DEHP (HD) offspring displayed aberrant anxiety-like behavior. Low dose B + D exposure modified anxiety-like and defensive behaviors, but high-dose exposure affected defensive behaviors and CORT. DEHP and B + D males showed a reduction in adrenal gland weights following low-dose exposure. These findings raise significant health concerns, especially considering the detectable levels of these chemicals in pregnant women’s urine (Arbuckle et al., 2014; Jensen et al., 2016; Muerkoster et al., 2020). Exposure pathways include dermal contact via clothing (Kaylor et al., 2023), food packaging (Pacyga et al., 2019) and the use of personal care products (Fisher et al., 2019). The potential long-term repercussions of these low-dose exposures on offspring behavior warrant attention, emphasizing the need for future rodent studies to delve into molecular mechanisms that influence these behavioral changes. Exploring neurotransmitter systems and hormone receptor dysregulation in the brain could offer valuable insights. Additionally, conducting extensive long-term studies in humans can further elucidate potential behavioral risks.
The original contributions presented in the study are included in the article, further inquiries can be directed to the corresponding author.
The animal study was approved by IACUC, University of Georgia, Athens, Georgia, United States. The study was conducted in accordance with the local legislation and institutional requirements.
AK: Formal Analysis, Investigation, Project administration, Supervision, Visualization, Writing–original draft. JS: Formal Analysis, Supervision, Validation, Writing–review and editing. AC: Data curation, Investigation, Validation, Writing–review and editing. JG: Data curation, Investigation, Validation, Writing–review and editing. MA: Investigation, Visualization, Writing–review and editing. NM: Data curation, Investigation, Writing–review and editing. HB: Data curation, Investigation, Writing–review and editing. PH: Conceptualization, Funding acquisition, Methodology, Resources, Writing–review and editing. PM: Conceptualization, Funding acquisition, Methodology, Project administration, Supervision, Writing–review and editing. SM: Conceptualization, Funding acquisition, Methodology, Supervision, Writing–review and editing, Project administration, Writing–original draft.
The author(s) declare financial support was received for the research, authorship, and/or publication of this article. This work was supported by the University of Georgia research foundation and start-up funds provided to PM and SM.
We would like to thank Elyssa Campbell for her technical assistance.
The authors declare that the research was conducted in the absence of any commercial or financial relationships that could be construed as a potential conflict of interest.
The author(s) declared that they were an editorial board member of Frontiers, at the time of submission. This had no impact on the peer review process and the final decision.
All claims expressed in this article are solely those of the authors and do not necessarily represent those of their affiliated organizations, or those of the publisher, the editors and the reviewers. Any product that may be evaluated in this article, or claim that may be made by its manufacturer, is not guaranteed or endorsed by the publisher.
Akkerman, S., Blokland, A., Reneerkens, O., Van Goethem, N. P., Bollen, E., Gijselaers, H. J., et al. (2012). Object recognition testing: methodological considerations on exploration and discrimination measures. Behav Brain Res, 232, 335–347. doi:10.1016/j.bbr.2012.03.022
Almeida, S., Raposo, A., Almeida-González, M., and Carrascosa, C. (2018). Bisphenol A: food exposure and impact on human health. Compr. Rev. Food Sci. Food Saf. 17, 1503–1517. doi:10.1111/1541-4337.12388
Altemus, M., Sarvaiya, N., and Neill Epperson, C. (2014). Sex differences in anxiety and depression clinical perspectives. Front. Neuroendocrinol. 35, 320–330. doi:10.1016/j.yfrne.2014.05.004
Antunes, M., and Biala, G. (2012). The novel object recognition memory: neurobiology, test procedure, and its modifications. Cogn. Process 13, 93–110. doi:10.1007/s10339-011-0430-z
Arbuckle, T. E., Davis, K., Marro, L., Fisher, M., Legrand, M., Leblanc, A., et al. (2014). Phthalate and bisphenol A exposure among pregnant women in Canada--results from the MIREC study. Environ. Int. 68, 55–65. doi:10.1016/j.envint.2014.02.010
Aubele, T., Kaufman, R., Montalmant, F., and Kritzer, M. F. (2008). Effects of gonadectomy and hormone replacement on a spontaneous novel object recognition task in adult male rats. Horm. Behav. 54, 244–252. doi:10.1016/j.yhbeh.2008.04.001
Baio, J., Wiggins, L., Christensen, D. L., Maenner, M. J., Daniels, J., Warren, Z., et al. (2018). Prevalence of autism spectrum disorder among children aged 8 Years - autism and developmental disabilities monitoring network, 11 sites, United States, 2014. MMWR Surveill. Summ. 67, 1–23. doi:10.15585/mmwr.ss6706a1
Balakrishnan, B., Henare, K., Thorstensen, E. B., Ponnampalam, A. P., and Mitchell, M. D. (2010). Transfer of bisphenol A across the human placenta. Am. J. Obstet. Gynecol. 202, 393.e1–e7. doi:10.1016/j.ajog.2010.01.025
Balasubramanian, P., Subramanian, M., Nunez, J. L., Mohankumar, S. M., and Mohankumar, P. S. (2014). Chronic estradiol treatment decreases brain derived neurotrophic factor (BDNF) expression and monoamine levels in the amygdala--implications for behavioral disorders. Behav Brain Res. 261, 127–133. doi:10.1016/j.bbr.2013.12.018
Balci, A., Ozkemahli, G., Erkekoglu, P., Zeybek, N. D., Yersal, N., and Kocer-Gumusel, B. (2020). Histopathologic, apoptotic and autophagic, effects of prenatal bisphenol A and/or di(2-ethylhexyl) phthalate exposure on prepubertal rat testis. Environ. Sci. Pollut. Res. Int. 27, 20104–20116. doi:10.1007/s11356-020-08274-6
Barakat, R., Lin, P. C., Park, C. J., Best-Popescu, C., Bakry, H. H., Abosalem, M. E., et al. (2018). Prenatal exposure to DEHP induces neuronal degeneration and neurobehavioral abnormalities in adult male mice. Toxicol. Sci. 164, 439–452. doi:10.1093/toxsci/kfy103
Biemann, R., Fischer, B., and Navarrete Santos, A. (2014). Adipogenic effects of a combination of the endocrine-disrupting compounds bisphenol A, diethylhexylphthalate, and tributyltin. Obes. Facts 7, 48–56. doi:10.1159/000358913
Blystone, C. R., Kissling, G. E., Bishop, J. B., Chapin, R. E., Wolfe, G. W., and Foster, P. M. (2010). Determination of the di-(2-ethylhexyl) phthalate NOAEL for reproductive development in the rat: importance of the retention of extra animals to adulthood. Toxicol. Sci. 116, 640–646. doi:10.1093/toxsci/kfq147
Bohus, B. (1987). “Limbic-midbrain mechanisms and behavioral physiology interactions with CRF, ACTH and adrenal hormones,” in Neurobiological approaches to human disease (Toronto: Hans Huber Publishers), 267–285.
Bondi, C. O., Barrera, G., Lapiz, M. D., Bedard, T., Mahan, A., and Morilak, D. A. (2007). Noradrenergic facilitation of shock-probe defensive burying in lateral septum of rats, and modulation by chronic treatment with desipramine. Prog. Neuropsychopharmacol. Biol. Psychiatry 31, 482–495. doi:10.1016/j.pnpbp.2006.11.015
Borman, E. D., Foster, W. G., and Decatanzaro, D. (2017). Concurrent administration of diethylhexyl phthalate reduces the threshold dose at which bisphenol A disrupts blastocyst implantation and cadherins in mice. Environ. Toxicol. Pharmacol. 49, 105–111. doi:10.1016/j.etap.2016.12.003
Botton, P. H., Costa, M. S., Ardais, A. P., Mioranzza, S., Souza, D. O., Da Rocha, J. B., et al. (2010). Caffeine prevents disruption of memory consolidation in the inhibitory avoidance and novel object recognition tasks by scopolamine in adult mice. Behav Brain Res. 214, 254–259. doi:10.1016/j.bbr.2010.05.034
Carbone, S., Ponzo, O. J., Gobetto, N., Samaniego, Y. A., Reynoso, R., Scacchi, P., et al. (2013). Antiandrogenic effect of perinatal exposure to the endocrine disruptor di-(2-ethylhexyl) phthalate increases anxiety-like behavior in male rats during sexual maturation. Horm. Behav. 63, 692–699. doi:10.1016/j.yhbeh.2013.01.006
Chapin, R. E., Adams, J., Boekelheide, K., Gray, L. E., Hayward, S. W., Lees, P. S., et al. (2008). NTP-CERHR expert panel report on the reproductive and developmental toxicity of bisphenol A. Birth Defects Res. B Dev. Reprod. Toxicol. 83, 157–395. doi:10.1002/bdrb.20147
Chen, F., Zhou, L., Bai, Y., Zhou, R., and Chen, L. (2014). Sex differences in the adult HPA axis and affective behaviors are altered by perinatal exposure to a low dose of bisphenol A. Brain Res. 1571, 12–24. doi:10.1016/j.brainres.2014.05.010
Choleris, E., Thomas, A. W., Kavaliers, M., and Prato, F. S. (2001). A detailed ethological analysis of the mouse open field test: effects of diazepam, chlordiazepoxide and an extremely low frequency pulsed magnetic field. Neurosci. Biobehav Rev. 25, 235–260. doi:10.1016/s0149-7634(01)00011-2
Christiansen, S., Axelstad, M., Scholze, M., Johansson, H. K. L., Hass, U., Mandrup, K., et al. (2020). Grouping of endocrine disrupting chemicals for mixture risk assessment - evidence from a rat study. Environ. Int. 142, 105870. doi:10.1016/j.envint.2020.105870
Christiansen, S., Boberg, J., Axelstad, M., Dalgaard, M., Vinggaard, A. M., Metzdorff, S. B., et al. (2010). Low-dose perinatal exposure to di(2-ethylhexyl) phthalate induces anti-androgenic effects in male rats. Reprod. Toxicol. 30, 313–321. doi:10.1016/j.reprotox.2010.04.005
Dagher, J. B., Hahn-Townsend, C. K., Kaimal, A., Mansi, M. A., Henriquez, J. E., Tran, D. G., et al. (2021). Independent and combined effects of Bisphenol A and Diethylhexyl Phthalate on gestational outcomes and offspring development in Sprague-Dawley rats. Chemosphere 263, 128307. doi:10.1016/j.chemosphere.2020.128307
Dai, Y., Yang, Y., Xu, X., and Hu, Y. (2015). Effects of uterine and lactational exposure to di-(2-ethylhexyl) phthalate on spatial memory and NMDA receptor of hippocampus in mice. Horm. Behav. 71, 41–48. doi:10.1016/j.yhbeh.2015.03.008
Ennaceur, A., and Delacour, J. (1988). A new one-trial test for neurobiological studies of memory in rats. 1: behavioral data. Behav Brain Res. 31, 47–59. doi:10.1016/0166-4328(88)90157-x
EPA (1987). Integrated risk information system (IRIS) chemical assessment summary: di(2-ethylhexyl)phthalate (DEHP); CASRN 117-81-7. Available online: https://cfpub.epa.gov/ncea/iris/iris_documents/documents/subst/0014_summary.pdf.
EPA (2010). Bisphenol A action plan (casrn 80-05-7). Available online: https://19january2017snapshot.epa.gov/sites/production/files/2015-09/documents/bpa_action_plan.pdf.
Erythropel, H. C., Maric, M., Nicell, J. A., Leask, R. L., and Yargeau, V. (2014). Leaching of the plasticizer di(2-ethylhexyl)phthalate (DEHP) from plastic containers and the question of human exposure. Appl. Microbiol. Biotechnol. 98, 9967–9981. doi:10.1007/s00253-014-6183-8
Fisher, M., Arbuckle, T. E., Macpherson, S., Braun, J. M., Feeley, M., and Gaudreau, E. (2019). Phthalate and BPA exposure in women and newborns through personal care product use and food packaging. Environ. Sci. Technol. 53, 10813–10826. doi:10.1021/acs.est.9b02372
Fucich, E. A., and Morilak, D. A. (2018). Shock-probe defensive burying test to measure active versus passive coping style in response to an aversive stimulus in rats. Bio Protoc. 8, e2998. doi:10.21769/BioProtoc.2998
Fucich, E. A., Paredes, D., Saunders, M. O., and Morilak, D. A. (2018). Activity in the ventral medial prefrontal cortex is necessary for the therapeutic effects of extinction in rats. J. Neurosci. 38, 1408–1417. doi:10.1523/JNEUROSCI.0635-17.2017
Hooversmith, J. M., Bhatti, D. L., and Holmes, P. V. (2019). Galanin administration into the prelimbic cortex impairs consolidation and expression of contextual fear conditioning. Behav Brain Res. 375, 112160. doi:10.1016/j.bbr.2019.112160
Hori, N., Yuyama, N., and Tamura, K. (2004). Biting suppresses stress-induced expression of corticotropin-releasing factor (CRF) in the rat hypothalamus. J. Dent. Res. 83, 124–128. doi:10.1177/154405910408300208
Janardhanan, R. (2018). Endocrine disrupting chemical induced "pollution of metabolic pathways": a case of shifting paradigms with implications for vascular diseases. Curr. Drug Targets 19, 1024–1037. doi:10.2174/1389450119666180514122244
Jensen, T. K., Frederiksen, H., Kyhl, H. B., Lassen, T. H., Swan, S. H., Bornehag, C. G., et al. (2016). Prenatal exposure to phthalates and anogenital distance in male infants from a low-exposed Danish cohort (2010-2012). Environ. Health Perspect. 124, 1107–1113. doi:10.1289/ehp.1509870
Jones, B. A., and Watson, N. V. (2012). Perinatal BPA exposure demasculinizes males in measures of affect but has no effect on water maze learning in adulthood. Horm. Behav. 61, 605–610. doi:10.1016/j.yhbeh.2012.02.011
Kajta, M., and Wójtowicz, A. K. (2013). Impact of endocrine-disrupting chemicals on neural development and the onset of neurological disorders. Pharmacol. Rep. 65, 1632–1639. doi:10.1016/s1734-1140(13)71524-x
Kang, J. H., Kondo, F., and Katayama, Y. (2006). Human exposure to bisphenol A. Toxicology 226, 79–89. doi:10.1016/j.tox.2006.06.009
Kaylor, S. D., Snell Taylor, S. J., and Herrick, J. D. (2023). Estimates of biomass reductions of ozone sensitive herbaceous plants in California. Sci. Total Environ. 878, 163134. doi:10.1016/j.scitotenv.2023.163134
Koolhaas, J. M., Korte, S. M., De Boer, S. F., Van Der Vegt, B. J., Van Reenen, C. G., Hopster, H., et al. (1999). Coping styles in animals: current status in behavior and stress-physiology. Neurosci. Biobehav Rev. 23, 925–935. doi:10.1016/s0149-7634(99)00026-3
Kundakovic, M., Gudsnuk, K., Franks, B., Madrid, J., Miller, R. L., Perera, F. P., et al. (2013). Sex-specific epigenetic disruption and behavioral changes following low-dose in utero bisphenol A exposure. Proc. Natl. Acad. Sci. U. S. A. 110, 9956–9961. doi:10.1073/pnas.1214056110
Labaronne, E., Pinteur, C., Vega, N., Pesenti, S., Julien, B., Meugnier-Fouilloux, E., et al. (2017). Low-dose pollutant mixture triggers metabolic disturbances in female mice leading to common and specific features as compared to a high-fat diet. J. Nutr. Biochem. 45, 83–93. doi:10.1016/j.jnutbio.2017.04.001
Lakind, J. S., and Naiman, D. Q. (2008). Bisphenol A (BPA) daily intakes in the United States: estimates from the 2003-2004 NHANES urinary BPA data. J. Expo. Sci. Environ. Epidemiol. 18, 608–615. doi:10.1038/jes.2008.20
Lapiz-Bluhm, M. D., Bondi, C. O., Doyen, J., Rodriguez, G. A., Bédard-Arana, T., and Morilak, D. A. (2008). Behavioural assays to model cognitive and affective dimensions of depression and anxiety in rats. J. Neuroendocrinol. 20, 1115–1137. doi:10.1111/j.1365-2826.2008.01772.x
Lin, H., Yuan, K., Li, L., Liu, S., Li, S., Hu, G., et al. (2015). In utero exposure to diethylhexyl phthalate affects rat brain development: a behavioral and genomic approach. Int. J. Environ. Res. Public Health 12, 13696–13710. doi:10.3390/ijerph121113696
Mahmoud, J. S., Staten, R., Hall, L. A., and Lennie, T. A. (2012). The relationship among young adult college students' depression, anxiety, stress, demographics, life satisfaction, and coping styles. Issues Ment. Health Nurs. 33, 149–156. doi:10.3109/01612840.2011.632708
Mallozzi, M., Bordi, G., Garo, C., and Caserta, D. (2016). The effect of maternal exposure to endocrine disrupting chemicals on fetal and neonatal development: a review on the major concerns. Birth Defects Res. C Embryo Today 108, 224–242. doi:10.1002/bdrc.21137
Martinez-Arguelles, D. B., Guichard, T., Culty, M., Zirkin, B. R., and Papadopoulos, V. (2011). In utero exposure to the antiandrogen di-(2-ethylhexyl) phthalate decreases adrenal aldosterone production in the adult rat. Biol. Reprod. 85, 51–61. doi:10.1095/biolreprod.110.089920
Mohankumar, S. M. J., Balasubramanian, P., Subramanian, M., and Mohankumar, P. S. (2018). Chronic estradiol exposure - harmful effects on behavior, cardiovascular and reproductive functions. Reproduction 156, R169–R186. doi:10.1530/REP-18-0116
Moore, R. W., Rudy, T. A., Lin, T. M., Ko, K., and Peterson, R. E. (2001). Abnormalities of sexual development in male rats with in utero and lactational exposure to the antiandrogenic plasticizer Di(2-ethylhexyl) phthalate. Environ. Health Perspect. 109, 229–237. doi:10.1289/ehp.01109229
Mouritsen, A., Aksglaede, L., Sorensen, K., Mogensen, S. S., Leffers, H., Main, K. M., et al. (2010). Hypothesis: exposure to endocrine-disrupting chemicals may interfere with timing of puberty. Int. J. Androl. 33, 346–359. doi:10.1111/j.1365-2605.2010.01051.x
Muerkoster, A. P., Frederiksen, H., Juul, A., Andersson, A. M., Jensen, R. C., Glintborg, D., et al. (2020). Maternal phthalate exposure associated with decreased testosterone/LH ratio in male offspring during mini-puberty. Odense Child Cohort. Environ. Int. 144, 106025. doi:10.1016/j.envint.2020.106025
Mumby, D. G., Glenn, M. J., Nesbitt, C., and Kyriazis, D. A. (2002). Dissociation in retrograde memory for object discriminations and object recognition in rats with perirhinal cortex damage. Behav Brain Res. 132, 215–226. doi:10.1016/s0166-4328(01)00444-2
Mustieles, V., Pérez-Lobato, R., Olea, N., and Fernández, M. F. (2015). Bisphenol A: human exposure and neurobehavior. Neurotoxicology 49, 174–184. doi:10.1016/j.neuro.2015.06.002
Naville, D., Pinteur, C., Vega, N., Menade, Y., Vigier, M., Le Bourdais, A., et al. (2013). Low-dose food contaminants trigger sex-specific, hepatic metabolic changes in the progeny of obese mice. Faseb J. 27, 3860–3870. doi:10.1096/fj.13-231670
Nijssen, A., and Schelvis, P. R. (1987). Effect of an anti-anxiety drug in a learned helplessness experiment. Neuropsychobiology 18, 195–198. doi:10.1159/000118417
Olff, M., Langeland, W., and Gersons, B. P. (2005). Effects of appraisal and coping on the neuroendocrine response to extreme stress. Neurosci. Biobehav Rev. 29, 457–467. doi:10.1016/j.neubiorev.2004.12.006
Pacyga, D. C., Sathyanarayana, S., and Strakovsky, R. S. (2019). Dietary predictors of phthalate and bisphenol exposures in pregnant women. Adv. Nutr. 10, 803–815. doi:10.1093/advances/nmz029
Panagiotidou, E., Zerva, S., Mitsiou, D. J., Alexis, M. N., and Kitraki, E. (2014). Perinatal exposure to low-dose bisphenol A affects the neuroendocrine stress response in rats. J. Endocrinol. 220, 207–218. doi:10.1530/JOE-13-0416
Poimenova, A., Markaki, E., Rahiotis, C., and Kitraki, E. (2010). Corticosterone-regulated actions in the rat brain are affected by perinatal exposure to low dose of bisphenol A. Neuroscience 167, 741–749. doi:10.1016/j.neuroscience.2010.02.051
Quinnies, K. M., Doyle, T. J., Kim, K. H., and Rissman, E. F. (2015). Transgenerational effects of di-(2-ethylhexyl) phthalate (DEHP) on stress hormones and behavior. Endocrinology 156, 3077–3083. doi:10.1210/EN.2015-1326
Quinnies, K. M., Harris, E. P., Snyder, R. W., Sumner, S. S., and Rissman, E. F. (2017). Direct and transgenerational effects of low doses of perinatal di-(2-ethylhexyl) phthalate (DEHP) on social behaviors in mice. PLoS One 12, e0171977. doi:10.1371/journal.pone.0171977
Ramos, A. (2008). Animal models of anxiety: do I need multiple tests? Trends Pharmacol. Sci. 29, 493–498. doi:10.1016/j.tips.2008.07.005
Ramtekkar, U. P., Reiersen, A. M., Todorov, A. A., and Todd, R. D. (2010). Sex and age differences in attention-deficit/hyperactivity disorder symptoms and diagnoses: implications for DSM-V and ICD-11. J. Am. Acad. Child. Adolesc. Psychiatry 49, 217–228.e3. e1-3. doi:10.1016/j.jaac.2009.11.011
Rowdhwal, S. S. S., and Chen, J. (2018). Toxic effects of di-2-ethylhexyl phthalate: an overview. Biomed. Res. Int. 2018, 1750368. doi:10.1155/2018/1750368
Scholl, J. L., Afzal, A., Fox, L. C., Watt, M. J., and Forster, G. L. (2019). Sex differences in anxiety-like behaviors in rats. Physiol. Behav. 211, 112670. doi:10.1016/j.physbeh.2019.112670
Sciolino, N. R., Dishman, R. K., and Holmes, P. V. (2012). Voluntary exercise offers anxiolytic potential and amplifies galanin gene expression in the locus coeruleus of the rat. Behav Brain Res. 233, 191–200. doi:10.1016/j.bbr.2012.05.001
Seibenhener, M. L., and Wooten, M. C. (2015). Use of the Open Field Maze to measure locomotor and anxiety-like behavior in mice. J. Vis. Exp., e52434. doi:10.3791/52434
Shang, J., Corriveau, J., Champoux-Jenane, A., Gagnon, J., Moss, E., Dumas, P., et al. (2019). Recovery from a myocardial infarction is impaired in male C57bl/6 N mice acutely exposed to the bisphenols and phthalates that escape from medical devices used in cardiac surgery. Toxicol. Sci. 168, 78–94. doi:10.1093/toxsci/kfy276
Shelby, M. D. (2006). Ntp cerhr Mon, v, vii-7, II-iii-xiii passim.NTP-CERHR monograph on the potential human reproductive and developmental effects of di (2-ethylhexyl) phthalate (DEHP)
Sifakis, S., Androutsopoulos, V. P., Tsatsakis, A. M., and Spandidos, D. A. (2017). Human exposure to endocrine disrupting chemicals: effects on the male and female reproductive systems. Environ. Toxicol. Pharmacol. 51, 56–70. doi:10.1016/j.etap.2017.02.024
Simone, J., Bogue, E. A., Bhatti, D. L., Day, L. E., Farr, N. A., Grossman, A. M., et al. (2015). Ethinyl estradiol and levonorgestrel alter cognition and anxiety in rats concurrent with a decrease in tyrosine hydroxylase expression in the locus coeruleus and brain-derived neurotrophic factor expression in the hippocampus. Psychoneuroendocrinology 62, 265–278. doi:10.1016/j.psyneuen.2015.08.015
Singh, A. R., Lawrence, W. H., and Autian, J. (1975). Maternal-fetal transfer of 14C-di-2-ethylhexyl phthalate and 14C-diethyl phthalate in rats. J. Pharm. Sci. 64, 1347–1350. doi:10.1002/jps.2600640819
Southwick, S. M., Vythilingam, M., and Charney, D. S. (2005). The psychobiology of depression and resilience to stress: implications for prevention and treatment. Annu. Rev. Clin. Psychol. 1, 255–291. doi:10.1146/annurev.clinpsy.1.102803.143948
Suteau, V., Briet, C., Lebeault, M., Gourdin, L., Henrion, D., Rodien, P., et al. (2020). Human amniotic fluid-based exposure levels of phthalates and bisphenol A mixture reduce INSL3/RXFP2 signaling. Environ. Int. 138, 105585. doi:10.1016/j.envint.2020.105585
Viau, V., and Meaney, M. J. (1991). Variations in the hypothalamic-pituitary-adrenal response to stress during the estrous cycle in the rat. Endocrinology 129, 2503–2511. doi:10.1210/endo-129-5-2503
Vo, T. T., Jung, E. M., Dang, V. H., Jung, K., Baek, J., Choi, K. C., et al. (2009a). Differential effects of flutamide and di-(2-ethylhexyl) phthalate on male reproductive organs in a rat model. J. Reprod. Dev. 55, 400–411. doi:10.1262/jrd.20220
Vo, T. T., Jung, E. M., Dang, V. H., Yoo, Y. M., Choi, K. C., Yu, F. H., et al. (2009b). Di-(2 ethylhexyl) phthalate and flutamide alter gene expression in the testis of immature male rats. Reprod. Biol. Endocrinol. 7, 104. doi:10.1186/1477-7827-7-104
Wang, R., Xu, X., Weng, H., Yan, S., and Sun, Y. (2016). Effects of early pubertal exposure to di-(2-ethylhexyl) phthalate on social behavior of mice. Horm. Behav. 80, 117–124. doi:10.1016/j.yhbeh.2016.01.012
Keywords: BPA, DEHP, mixture, prenatal exposure, behavior, sex differences
Citation: Kaimal A, Hooversmith JM, Cherry AD, Garrity JT, Al Mansi MH, Martin NM, Buechter H, Holmes PV, MohanKumar PS and MohanKumar SMJ (2023) Prenatal exposure to bisphenol A and/or diethylhexyl phthalate alters stress responses in rat offspring in a sex- and dose-dependent manner. Front. Toxicol. 5:1264238. doi: 10.3389/ftox.2023.1264238
Received: 24 July 2023; Accepted: 29 November 2023;
Published: 13 December 2023.
Edited by:
Xinyuan Zhao, Nantong University, ChinaReviewed by:
Christy Lite Samuel, The University of Texas at Austin, United StatesCopyright © 2023 Kaimal, Hooversmith, Cherry, Garrity, Al Mansi, Martin, Buechter, Holmes, MohanKumar and MohanKumar. This is an open-access article distributed under the terms of the Creative Commons Attribution License (CC BY). The use, distribution or reproduction in other forums is permitted, provided the original author(s) and the copyright owner(s) are credited and that the original publication in this journal is cited, in accordance with accepted academic practice. No use, distribution or reproduction is permitted which does not comply with these terms.
*Correspondence: Sheba M. J. MohanKumar, c2hlYmFta0B1Z2EuZWR1
Disclaimer: All claims expressed in this article are solely those of the authors and do not necessarily represent those of their affiliated organizations, or those of the publisher, the editors and the reviewers. Any product that may be evaluated in this article or claim that may be made by its manufacturer is not guaranteed or endorsed by the publisher.
Research integrity at Frontiers
Learn more about the work of our research integrity team to safeguard the quality of each article we publish.