- Institute for In Vitro Sciences, Inc. (IIVS), Gaithersburg, MD, United States
Aging is one of the most dynamic biological processes in the human body and is known to carry significant impacts on individuals’ self-esteem. Skin pigmentation is a highly heritable trait made possible by complex, strictly controlled cellular and molecular mechanisms. Genetic, environmental and endocrine factors contribute to the modulation of melanin’s amount, type and distribution in the skin layers. One of the hallmarks of extrinsic skin aging induced by environmental stress factors is the alteration of the constitutive pigmentation pattern clinically defined as senile lentigines and/or melasma or other pigmentary dyschromias. The complexity of pollutants and tobacco smoke as environmental stress factors warrants a thorough understanding of the mechanisms by which they impact skin pigmentation through repeated and long-term exposure. Pre-clinical and clinical studies demonstrated that pollutants are known to induce reactive oxygen species (ROS) or inflammatory events that lead directly or indirectly to skin hyperpigmentation. Another mechanistic direction is provided by Aryl hydrocarbon Receptors (AhR) which were shown to mediate processes leading to skin hyperpigmentation in response to pollutants by regulation of melanogenic enzymes and transcription factors involved in melanin biosynthesis pathway. In this context, we will discuss a diverse range of New Approach Methodologies (NAMs) capable to provide mechanistic insights of the cellular and molecular pathways involved in the action of environmental stress factors on skin pigmentation and to support the design of raw ingredients and formulations intended to counter their impact and of any subsequently needed clinical studies.
1 Introduction
Aging is a complex of progressive, irreversible and unavoidable structural and functional changes that the human body naturally progresses through, all highly dependable on and influenced by intrinsic and extrinsic factors within or outside our control. Deciphering the complicated pathways responsible for aging and discovering possibilities to delay the process has been a challenging task for many researchers. Skin aging in particular is of interest and concern to the medical field since it has multiple immediate medical, psychological and social impacts on one’s self-esteem as skin’s unique texture and color may change significantly in response to stress factors (Gupta and Gilchrest, 2005).
In this review, we will focus on the activity and impact of extrinsic factors that contribute to skin aging, specifically to skin pigmentation. Of these factors, growing evidence demonstrate that environmental aggressors contribute to skin aging through induction of hyperpigmentation among several other pathways. Based on the available studies, we will primarily address the effects induced to skin pigmentation by prolonged or repetitive exposure to tobacco, Particulate Matter (PM) [including Diesel Exhaust Particles (DEPs)], Polycyclic Aromatic Hydrocarbons (PAHs), and to a lesser extent Polychlorinated Biphenyls (PCBs).
Through our review of available sources, we identified 16 manuscripts reporting on the action of air pollution on skin pigmentation, and 11 on tobacco’s impact. The search and identification of these manuscripts was conducted using primarily the PubMed database and the individual websites of the journals the papers were published in. The search terms used to narrow the resources as pertaining to the topic of the manuscript were: “in vitro”, “NAMs”, “in vivo”, “clinical”, “ex vivo”, “melanocytes”, “keratinocytes”, “3D model”, “melanogenesis”, “melanin synthesis”, “skin pigmentation”, skin aging”, “pollution”, “smoking”, “tobacco”, “tobacco smoke”, “nicotine”, “smoking”. As for the inclusion criteria, the manuscripts considered for our analysis had to associate the pollutants (of choice, see exclusion criteria) and tobacco (and associated terms) to their mechanism of action being investigate and/or to products being researched for their anti-pollution and anti-tobacco-induced aging skin potential. Also as an inclusion criteria, only the manuscripts investigating specifically skin pigmentation as sign of skin aging were considered. The manuscripts that met the following exclusion criteria were not considered for our analysis: non peer-reviewed; investigating pollutants other than PM, PAHs, PCBs, DEPs; introducing a hypothetical mechanism not supported at least by preliminary data or by cross-referenced studies (entirely speculative); investigated the impact of external factors on skin pigmentation other than pollutants and tobacco (as narrowed down by these criterion) given the scope of the manuscript. Our analysis identified a tendency to use clinical studies, especially to research tobacco’s impact on skin pigmentation. Clinical studies are designed to investigate the effects of various toxic agents on skin at a visual, measurable level, thus providing valuable epidemiological evidence. They are however limited in their capacity to shed light on the cellular and molecular mechanisms which are key to the design of products to counteract the deleterious effects of these toxicants. Besides being relatively complex and expensive to conduct, clinical studies are more difficult to standardize in terms of design to fit the goals and endpoints of interest. Furthermore, clinical studies researching skin pigmentation are even more challenging to set up as they need to eliminate or limit the contribution of confounding factors (e.g., UV) to melanin production.
Our analysis also identified an encouraging trend in the reduction of animal-based studies focused on the impact of air pollution on skin pigmentation. In general, rodents (i.e., rats or mice) or pigs represent the main in vivo test systems used to study skin responses to toxicants (Abd et al., 2016). Rodent skin differs significantly from human skin in terms of thickness and higher density of hair follicles which subsequently increase dermal penetration (Todo, 2017). In comparison, the porcine skin resembles structurally the properties of human skin (Debeer et al., 2013), however the animals are more challenging to handle and have higher fat storage (Summerfield et al., 2015), which could be a confounding factor as many pollutants are stored in the hypodermis (La Merrill et al., 2013). Finally, research on skin aging is relatively difficult to replicate in an animal test system which makes the observations very challenging, if not impossible. Therefore, aging is often induced chemically or biologically in an animal-based research setting. As a consequence, this approach is less reliable and more difficult to execute compared to the convenience of smaller scale but quite complex platforms based on alternative test systems.
Advances in the toxicological methodologies and increasing ethical concerns regarding animal experimentation led to the development of various alternative methods based on the Replacement, Reduction and Refinement (3Rs) of animal studies (Naik et al., 2023). In our review we identified that more than 50% of the reported experiments were conducted using non-animal test systems to investigate the impact of environmental stress factors on skin pigmentation. This is a very encouraging trend which should be further supported and promoted to help expand the research on tobacco’s impact on skin color. The design of these experiments facilitates an in-depth understanding of the mechanisms involved in the impact of air pollution and tobacco smoke on skin pigmentation and aging process. It is our opinion that this level of experimental complexity can be accomplished by using modern New Approach Methodologies (NAMs) which are based on in chemico, in vitro, and ex vivo test system models. These technologies offer endless possibilities to gain a detailed understanding of the mechanisms of action and also to design potent and safe actives capable to counteract the damaging action of aggressors and to inform future directions of clinical studies, as detailed in this review. We will also provide insights into study design, selection of appropriate test system, type of toxicant, route and length of exposure, and human exposure relevance. Last but not least, we will discuss possible future research directions into untapped mechanisms which may hold the key to efficient treatments addressing skin hyperpigmentation induced by pollutants.
2 Extrinsic skin aging induced by environmental stress factors: impact on skin pigmentation
The skin is a complex organ responsible for multiple functions that are critical for the overall body physiology such as protection (against biological, mechanical or chemical factors), sensation (touch, temperature, pain, pressure), regulation of temperature, absorption and excretion, synthesis of vitamin D and aesthetic and social functions. Extrinsic and intrinsic factors act in concert upon the physiology and functionality of the largest organ in the human body and are responsible for skin aging. Extrinsic skin aging is induced by stressors originating in the environment and is typified by coarse wrinkles, elastosis and irregular pigmentation.
Besides the obvious changes aging brings in one’s life quality, this global phenomenon is highly dependent on the sense of perception and self-awareness that seems to have increased in recent decades, alongside individuals’ desire to improve their appearance by maintaining a healthy skin and delaying the aging process. Signs of skin aging, such as senile lentigines (age spots), melasma or other pigmentary dyschromias motivate aging population to seek treatment for these conditions. While for a long time exposure to sun was considered the main contributory factor to aging, with direct impact on skin pigmentation, recent studies point towards other detrimental external factors such as air pollution. The World Health Organization (WHO) reports that air pollution is the most significant and urgent public problem of the 21st century (WHO, 2021). Although tobacco smoke is technically considered part of the air pollution, for the purpose of this review we will treat them separately as they relate to distinct human behaviors. While exposure to tobacco smoke can be considered a voluntary behavior and therefore controllable (with the exception of secondhand smoke exposure), exposure to air pollution is often involuntary and less or not at all controlled.
2.1 Air pollution
High pollution levels were initially identified in urban areas, however they became a global concern and the world’s largest single environmental health risk factor associated with millions of deaths annually (WHO, 2021). The anti-pollution movement in cosmetic and personal care industry began in the Asia Pacific region and it has now reached the western markets, launching hundreds of commercially successful products addressing this global concern (Pitman, 2017). One of the targets for these products is skin hyperpigmentation as the production of melanin is a very sensitive marker to changes induced by stress factors, including pollution. Integration of the complex biological pathway of melanin biosynthesis in the world wide context of skin tone uniqueness combined with geographically specific pollution concerns makes the research of the molecular mechanisms involved even more important.
The source and composition of pollutants are important aspects to take into account when conducting research focused on their effects on skin pigmentation. Air pollutants are emitted directly from the source (volcanic eruptions, forest fires, biological decay, power plants, industries, car emissions, fossil fuel burning, etc.) or following exposure to heat or UV (Krutmann et al., 2014). PM is a key component of air pollution that has been thoroughly investigated. The particle size, concentration and chemical properties of PM vary widely geographically and in time (Kampa and Castanas, 2008). Because of their textured structure, the particles have an ideal surface for the attachment of other toxicants such as PAHs, which originate from wood burning, combustion of organic material including coal burning, automobile exhaust fumes, and cigarette smoke. PAHs are highly lipophilic and therefore can easily penetrate through the skin barrier (Jux et al., 2011) and reach the melanocytes, the pigment producing cells resident in the skin. Multiple studies have shown that exposure to other air pollutants such as DEPs is associated with clinical signs of hyperpigmentation (Krutmann et al., 2020; Grether-Beck et al., 2021). Last but not least, PCBs are highly persistent environmental pollutants known to have accidentally contaminated rice oil alongside polychlorinated dibenzofurans (PCDFs) and polychlorinated quaterphenyls (PCQs) (Yusho accident, Japan, 1968 and Yu-Cheng accident, Taiwan, 1979) (Masuda, 1985). One of the symptoms for the poisoning that occurred during these incidents, though not the most impactful in terms of toxic effects, was increased skin pigmentation.
Impact of air pollution on animal species has been the subject of observations in populated areas and provided more evidence into the long-term effects on skin. For example, increased levels of pigmentation were observed to be common in several animal species (e.g., pigeons and moths) found in urban industrial environments (Chatelain et al., 2014; Van’t Hof et al., 2016). Observations on Emydocephalus annulatus showed that overproduction of melanin occurred more frequently in skin of sea snakes living in polluted water compared to unpolluted environments (Goiran et al., 2017). These observations support the contribution of pollution to skin hyperpigmentation.
The ability of particles to penetrate the skin layers is still debatable especially since studies report conflicting results likely due to having used a variety of particle types and different experimental models and designs (Toll et al., 2004; Baroli et al., 2007). Ambient PM was shown to penetrate the skin either following the route of hair follicles and sweat ducts (Lademann et al., 2004; Koohgoli et al., 2017) or transepidermally (Jin et al., 2018). Thus, they may be able to reach the melanocytes and release the highly lipophilic and toxic surface-bound PAHs. Air pollutants are also capable to affect directly the function of skin cells by hijacking classic signaling pathways otherwise known to be UV’s routes of action on skin pigmentation. It was recently demonstrated that particles can also penetrate the stratum corneum (Burke, 2018). Further mechanistic studies are necessary to elucidate the contribution of the particles and other attached environmental stress factors to extrinsic skin aging in order to find reliable means to counteract their actions.
2.2 Tobacco smoke
Of an equally complex composition to air pollutants is the tobacco smoke, an aerosolized by-product of tobacco combustion during the smoking of cigarettes and related products and consisting of a particulate and a gas phase. While nicotine is known to be absorbed through skin (Benowitz and Hukkanen, 2009), details regarding the penetration of other fractions of the tobacco smoke through dermal layers is lacking. Even though nicotine is easy to use on its own in research experiments, the reality indicates that the most harmful toxicants are found primarily in the gas phase. It is therefore of the utmost importance to perform hazard and risk assessment using a combination of both phases to better represent the in-use exposure and to take into consideration any synergistic effects based on the interactions between the two phases. The particulate phase may include PAHs and tobacco specific byproducts, while the gas phase may contain dioxins among other harmful radicals. Of the dioxins, 2,3,7,8-tetrachlorodibenzo para-dioxin (TCDD) was demonstrated to be the most toxic molecule and one of the first environmental-related molecule shown to stimulate skin pigmentation (Luecke et al., 2010), thus opening up the investigations into premature skin aging induced by tobacco (Kennedy et al., 2003; Morita et al., 2009).
2.3 Melanin biosynthesis pathways
Melanocytes are highly specialized cells responsible for melanin biosynthesis through a complex pathway hosted by membrane-bound organelles known as melanosomes (Hearing, 1997). Following four stages of maturation, the melanosomes are transferred through the dendrites of the melanocytes to the surrounding keratinocytes where they play a critical role in photo-protection. The established “epidermal melanin unit” is comprised of a ratio of one melanocyte to 40 keratinocytes in the basal and suprabasal layers (Ostrowski and Fisher, 2019). The epidermal melanin unit responds rapidly to a multitude of environmental stimuli, of which UV was identified early as one of the main contributing factors to stimulate the melanin production. Melanocytes respond directly to UV exposure and through their close communication with the keratinocytes.
Melanin biosynthesis is tightly regulated by three melanogenic enzymes, Tyrosinase (TYR), Tyrosinase-related protein 1 (TYRP-1), and Dopachrome Tautomerase (DCT, also referred to as Tyrosinase-related protein 2 - TRP-2), located in melanosomes (Basrur et al., 2003). Tyrosinase is a key enzyme of the melanogenic pathway and primarily responsible for melanin biosynthesis. It catalyzes the oxidation of L-Tyrosine to L-DOPA which subsequently is oxidized to L-DOPAquinone that ultimately produces DOPAchrome, which is further converted into 5,6-dihidroxyindole-2carboxylic acid (DHICA) by DCT. TYRP-1 can oxidize DHICA to indole-5-6quinone carboxylic acid, and further to DHI-melanin (Figure 1—Melanin Synthesis); the DHI and DHICA moieties are part of eumelanin. Pheomelanin synthesis is based on the production of Cysteinyl-DOPA conjugates from DOPAquinone and consists of benzothiazine and benzothiazole groups. TYRP-1 plays a key role in the correct trafficking of TYR to melanosomes (Toyofuku et al., 2001), while DCT is involved in crucial detoxification processes within melanosomes (Milac and Negroiu, 2018). Activation of TYR, TYRP-1, and DCT is controlled by Microphthalmia Transcription Factor (MITF), a key transcriptional regulator of the pathway (D’Mello et al., 2016), which has been subjected to detailed studies looking into mechanisms of hyperpigmentation (Figure 1).
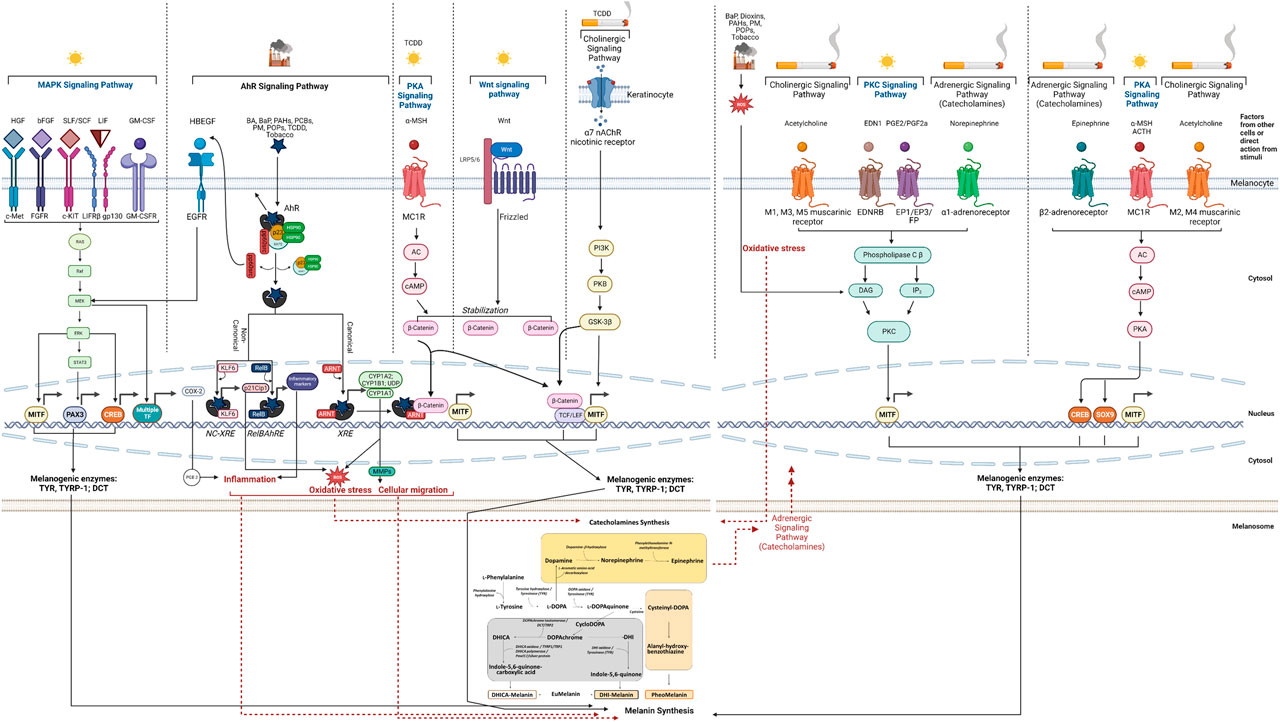
FIGURE 1. Overview of demonstrated or possible mechanisms and pathways involved in melanin synthesis induced by air pollution and tobacco. The mechanisms are depicted to take place within the melanocytes and in their specific cellular compartments (cytosol, nucleus and melanosome), except where the participation of other cells (i.e., keratinocytes) is indicated. Abbreviations: AC, Adenylate Cyclase; ACTH, Adenochorticoprotic Hormone; AhR, Aryl hydrocarbon Receptor; ARNT, Aryl Hydrocarbon Receptor Nuclear Translocator; BA, Benzanthrone; BaP, benzo[a]pyrene; bFGF, basic Fibroblast Growth Factor; cAMP; cyclic Adenosine Monophosphate; CREB, cAMP Response Element-Binding Protein; COX, Cyclooxygenase; CYP, cytochrome; DAG, Diacylglycerol; DCT, DOPAchrome Tautomerase; DHI, 5,6-dihydroxyindole; DHICA, 5,6-dihydroxyindole-2-carboxylic acid; DOPA, L-3,4-dihydroxyphenylalanine; EDN1 (EDN-1, ET-1), Endothelin-1; EGF, Epidermal Growth Factor; EDNRB, Endothelin Receptor Type B; EGFR, Epidermal Growth Factor Receptor; EP, Prostaglandin E Receptor; ERK, Extracellular-signal Regulated Kinase; FGFR, Fibroblast Growth Factor Receptor; FP, Prostaglandin F Receptor; GM-CSF, Granulocyte-Macrophage Colony-Stimulating Factor; GM-CSFR, Granulocyte-Macrophage Colony-Stimulating Factor Receptor; GSK-3β, Glycogen Synthase Kinase 3 beta; HBEGF, Heparin Binding EGF Like Growth Factor; HGF, Hepatocyte Growth Factor; HMG, High Mobility Group; HSP, Heat Shock Protein; IL, Interleukin; IP3, Inositol triphosphate; KLF, Krüppel-like Factor; LDL, Low-Density Lipoprotein; LEF, Lymphoid Enhancer Factor; LIF, Leukemia Inhibitory Factor; LIFR, Leukemia Inhibitory Factor Receptor; LRP, LDL Receptor-related Protein; MAPK, Mitogen Activated Protein Kinase; MC1R, Melanocortin 1 Receptor; MEK, MAPK kinase; MITF, Microphthalmia-Associated Transcription Factor; MMP, Matrix Metalloproteinase; MSH, Melanocyte Stimulating Hormone; nAChR, nicotinic Acetylcholine Receptor; NC-XRE, Nonconsensus Xenobiotic Response Element; p21Cip1 (Cyclin Dependent Kinase Inhibitor); PAH, Polycyclic Aromatic hydrocarbon; PAX, Paired Box; PCB, Polychlorinated Biphenyls; PI3K, Phosphoinositide 3-Kinase; PK, Protein Kinase; PM, Particulate Matter; POP, Persistent Organic Pollutant; PGE, Prostaglandin E; PGF, Prostaglandin F; Raf, Rapidly Accelerated Fibrosarcoma; Ras, Rat Sarcoma; RelB, RELB Proto-Oncogene, NF-kB Subunit); RebBARE, RelB Aryl hydrocarbon Receptor Response Element; ROS, Reactive Oxygen Species; SCF, Stem Cell Factor; SLF, Steel Factor; SOX, SRY-related HMG box; STAT, Signal Transducer and Activator of Transcription; TCDD, 2,3,7,8-tetrachlorodibenzo-p-dioxin; TCF, T-Cell Factor; TF, Transcription Factor; TYR, Tyrosinase; TYRP-1, Tyrosinase Related Protein 1; Wnt, Wingless-related integration site; XAP2, hepatitis B virus X-associated Protein (also known as AIP, Aryl hydrocarbon Receptor Interacting Protein); XRE, Xenobiotic Response Element. Created with BioRender.com.
There are two types of skin pigmentation: constitutive and facultative. The constitutive skin color is determined genetically, is not directly affected by sun exposure (or other factors) and has photo-protective properties (Ostrowski and Fisher, 2019). Facultative skin color (tan) is induced by UV exposure, hormonal changes, disease or other stress factors (Ostrowski and Fisher, 2019). Dark skin was shown to have large and numerous melanosomes that are individually bound within a membrane compared to lighter skin, which traditionally produces less melanin and contains smaller melanosomes (Alaluf et al., 2002) aggregated and bound in groups as complexes (Thong et al., 2003; Coelho et al., 2009). These specific characteristics generate the tremendous variation of skin tones in relation to ethnicity and response to toxicants (pollutants) and to products designed to counteract their deleterious effects on skin pigmentation. The complexity of pollutants in terms of composition needs to be superimposed on the melanogenic pathway and its governing factors in order to understand the impacts and to address them at the cellular and molecular level.
3 Intracellular signaling pathways crosstalk: impacts of air pollution and tobacco smoke
The regulation of the complex melanogenesis pathway involves a multitude of genes, signaling systems and transcription factors which control the process at various levels, from the melanoblasts development to melanocyte survival, differentiation, and melanin production. In the following sections we will focus on several signaling pathways that govern the melanin synthesis, some established and others that are speculative to a degree. Many pathways have been historically demonstrated primarily based on the impact of UV on skin pigmentation. Even though UV is not the subject of this manuscript, we will use the pathways it impacts to evaluate the crosstalk with others that are affected by air pollution and tobacco.
3.1 Mitogen-Activated Protein Kinase (MAPK) signaling pathway and AhR pathway
MAPK signaling plays a key role in cellular proliferation, cell metabolism, and inflammation. It is also known to regulate the transcription of cyclic adenosine monophosphate (cAMP) responsive-element binding protein (CREB) (Estrada et al., 2022) and MITF, which is the master regulator of the melanogenic enzymes in charge of pigments synthesis (D’Mello et al., 2016). Of the multiple promoters of MITF gene, M promoter is selectively used in melanocytes and targeted by several transcriptional factors that impact the melanogenic pathway: paired box 3 (PAX3) protein, SRY-related High Mobility Group (HMG) box 9 and 10 (SOX9, SOX10), lymphoid enhancer factor 1 (LEF-1), one cut domain 2 (ONECUT-2), MITF itself (Steingrímsson et al., 2004; Passeron et al., 2007), and CREB. Through a ligand-receptor system, melanocytes respond to increased levels of hepatocyte growth factor (HGF), basic fibroblast growth factor (bFGF), steel factor (SLF/SCF), leukemia inhibitory factor (LIF) and granulocyte-macrophage colony-stimulating factor (GM-CSF) produced by keratinocytes following UV exposure. They ultimately activate the MAPK pathway (Figure 1), thereby phosphorylating MITF and upregulating the expression of TYR, TYRP-1 and DCT (Yarden et al., 1987; Bonaventure et al., 2013).
Of the signaling systems that act upon the MAPK cascade, the one governed by SLF/SCF/c-KIT plays a key role in melanocyte homeostasis. c-KIT has functional xenobiotic-responsive elements (XREs) in its promoter, which are recognized by the Aryl hydrocarbon Receptor (AhR) (Jux et al., 2011), the key player of one of the signaling pathways pollutants act upon, subsequently impacting skin pigmentation. The MAPK-AhR connection is one of the many cross talking points discussed in this part of the manuscript. For simplicity, the direct connection between c-KIT and AhR pathway is not marked on Figure 1.
AhR is a highly conserved cytosolic transcription factor activated by a broad variety of exogenous and endogenous ligands (Nebert, 2017; Rothhammer and Quintana, 2019). In the absence of a ligand, AhR exists in an inactive/latent state as part of a cytosolic multi-protein complex, consisting of two heat-shock protein 90 molecules (HSP90), immunophilin-like hepatitis B virus X-associated Protein (XAP)2, the co-chaperone protein p23, and pp60src (Nebert, 2017). Activation of the receptor following the interaction with a ligand follows either a canonical or a non-canonical pathway, as further explained.
In the canonical signaling pathway, binding of a ligand to the AhR induces conformational changes that facilitate the nuclear translocation of the AhR-ligand complex, releasing hsp90, XAP2 and p23 (Salminen, 2022). AhR subsequently dimerizes with its partner, a structurally related nuclear protein, aryl hydrocarbon receptor nuclear translocator (ARNT) (Salminen, 2022). The complex binding to XREs induces the transcription of genes involved in the response to toxicants such as those encoding for both phase I and phase II xenobiotic metabolism enzymes [cytochromes P450 (CYP) 1A1, CYP1A2, CYP1B1 and UDP glucuronosyltransferase 1 family polypeptide A6 (UGT1A6)] (Ikuta et al., 2009). The activity of these enzymes can be deleterious because they generate reactive oxygen species (ROS). The contribution of ROS to melanin production is discussed in Section 3.5 as one key cross talking point between several intracellular pathways affecting melanin production. As the canonical pathway unfolds, the dissociated pp60src activates the epidermal growth factor receptor (EGFR) and induces its internalization and nuclear translocation (Agostinis et al., 2007). As a result, the downstream MAPK signal transduction is activated (Noakes, 2015), with direct impact on skin pigmentation (Figure 1). This also results in the transcriptional induction of cyclooxygenase-2 (COX-2) which is responsible for the production of Prostaglandin E2 (PGE2) (Matsumura, 2009), a key player in two complex pathways that crosstalk with the melanogenesis pathway: PGE2 induces downstream inflammatory events that lead to hyperpigmentation (as discussed in Section 3.6) and acts directly on the Protein Kinase C (PKC) pathway (Section 3.3).
As part of the non-canonical AhR signaling pathway, AhR binds to non-consensus XRE (NC-XRE) sites (Huang and Elferink, 2012) that share marked homology with the DNA binding sequence of the Krüppel-like factors (KLFs) family (Wilson et al., 2013). Of this family, KLF6 regulates expression of the p21Cip1 cyclin-dependent kinase inhibitor (Bieker, 2001), which is known to lead to ROS accumulation (crosstalk). RelB gene was shown to mediate another non-canonical pathway by interaction with AhR and binding to an unrecognized genomic RelB/AhR responsive element (RelBAhRE) (Vogel et al., 2007). As a result, production of inflammatory markers was shown to stimulate melanin production (Khmaladze et al., 2020; Fernández-Martos et al., 2021; Nkengne et al., 2021; Moon et al., 2022 - detailed in Table 1), another cross talking point in the complex pathways network governing melanogenesis (see Section 3.6).

Table 1. Summary of in vitro, ex vivo, in vivo and clinical studies reporting on the impact of pollution and tobacco on skin pigmentation, including effects of ingredients or products with anti-pollution or anti-tobacco-induced hyperpigmentation activity.
Originally, AhR was identified as a TCDD-binding receptor protein and was later demonstrated to also recognize benzo[a]pyrene (BaP) (Tsuji et al., 2011; Nakamura et al., 2016), other dioxins, PAHs (Schecter et al., 2006), benzanthrone (BA) (Abbas et al., 2017), and related persistent organic pollutants (POPs) (Nebert, 2017) as ligands leading to hyperpigmentation (Luecke et al., 2010; Jux et al., 2011; Esser et al., 2013). TCDD, BaP, and benzanthrone are also components of tobacco and research by Tsuji et al., 2011; van den Bogaard et al., 2013; Nakamura et al., 2016; Abbas et al., 2017 supported their involvement in stimulation of melanogenesis. Furthermore, AhR also mediates melanoblast to melanocyte maturation and plays wider roles in skin melanogenesis (Esser et al., 2013; Nakamura et al., 2013). The contribution of AhR to melanin production in response to environmental stimuli is further supported by evidence provided by UV exposure as another proof of pollutants using established intracellular pathways leading to hyperpigmentation. For example, genes targeted by AhR were shown to be induced by UVB irradiation (Noakes, 2015). In sum, skin’s exposure to environmental stimuli can activate hyperpigmentation via AhR activation and subsequent increase of TYR activity, melanin synthesis, and upregulation of TYRP-1, DCT, and MITF expression (Krutmann et al., 2008; Luecke et al., 2010; Nakamura et al., 2013; Nakamura et al., 2016; Abbas et al., 2017; Leijs et al., 2018; Chan et al., 2021; Shi et al., 2021; Ahn et al., 2022) (Table 1).
3.2 Protein Kinase A (PKA) signaling pathway
The activation of adenylate cyclase (AC) upon exposure to UV as primary stimulus or to other factors catalyzes the conversion of ATP to the second messenger cAMP, which attaches to PKA leading to its activation. PKA subsequently phosphorylates CREB that can enhance MITF’s overexpression (Shin et al., 2015), with direct contribution to melanin production. Proteolytic cleavage of the proopiomelanocortin (POMC) protein results in the formation of adrenocorticotropic hormone (ACTH) and alpha-melanocyte-stimulating hormone (α-MSH). Both these factors are produced by keratinocytes in response to UV exposure and act as agonists to melanocortin 1 receptor (MC1R) on melanocytes, thereby increasing cAMP levels (Yamaguchi and Hearing, 2009) (Figure 1). At this point, the PKA and MAPK cascades crosstalk and contribute to melanin production through the common factor CREB (Figure 1). Evidence of tobacco or air pollutants acting directly on this signaling pathway are lacking. Given the common players among the MAPK, PKA and AhR pathways, it is conceivable to believe that the PKA could be chosen by environmental stimuli as direct or indirect port of entry to melanocytes. The only link between the AhR and PKA signaling routes was identified in the study by Bahraman et al., 2021 showing that the increase of α-MSH-induced expression of AhR may be due to the influence of cAMP directly or indirectly via the Wingless-related integration site (Wnt)/β-catenin pathway or both pathways. The participation of β-catenin to skin pigmentation in response to stimuli is discussed later (Section 3.4). Last but not least, there is another intersection point that could be of interest in the context discussed herein: between the PKA and adrenergic signaling pathways (Section 3.9).
3.3 Protein Kinase C (PKC) signaling pathway
The PKC pathway regulates melanogenesis (Gordon and Gilchrest, 1989) following the binding of Endothelin (EDN) 1 to its receptor (EDNRB) and of PGE2/PGF2 to their respective receptors, EP1/EP3/FP, in response to UV exposure (Park et al., 2006) (Figure 1). After the complex formation, phospholipase C β (PLCβ) is activated and catalyzes the production of inositol triphosphate (IP3) and diacylglycerol (DAG). IP3 induces the production of cytosolic Ca2+ response in melanocyte dendrites (Stanisz et al., 2012; Belote and Simon, 2020), whereas DAG activates PKC, which enhances the expression of MITF directly or indirectly via the MAPK cascade (Serre et al., 2018) (crosstalk) (Figure 1). In addition, the EP3 receptor controls the effects of PGE2 on cAMP in melanocytes that stimulates TYR activity and proliferation (Scott et al., 2004; Scott et al., 2005) (cross talk with PKA signaling pathway - see Section 3.2). The signaling of the fourth receptor of PGE2, EP4, stimulates cAMP production in melanocytes and subsequently TYR and the formation of dendrites (Starner et al., 2010), with direct implications in melanin biosynthesis (for simplicity, not displayed in Figure 1).
PKC usually resides in an inactive state in the cytoplasm but is activated by DAG generated from UV-irradiated cell membranes (Bae-Harboe and Park, 2012). DAG induces PKC translocation from the cytoplasm to membranes where PKC catalyzes the phosphorylation of serine residues on the cytoplasmic domain of TYR, thus activating it (Park et al., 1999). This is also another cross talking point with ROS-generation pathways that increase oxidative stress in response to toxicants, leading to high production of DAG and subsequent stimulation of melanin production (Costin and Hearing, 2007) (Figure 1). Finally, the cholinergic (muscarinic) and adrenergic (α1) signaling pathways crosstalk with the PKC cascade in response to stimuli (Figure 1) and have been speculated or demonstrated to stimulate the melanin production in skin (discussed later, in Sections 3.8 and 3.9, respectively). Direct impact of tobacco and environmental toxicants on PKC signaling is also lacking, however this is a pathway of much interest to investigate further as it already offers multiple communication points with other routes directly taken by pollutants to act on skin pigmentation.
3.4 Wnt/β-catenin signaling pathway; synergy with PKA, Protein Kinase B (PKB) and AhR pathways
The Wnt signaling pathway mediates melanogenesis by enhancing MITF’s expression. As part of this cascade, Frizzled (FZD) receptors bind to the transmembrane molecule Low-Density Lipoprotein Receptor-related Protein (LRP5/6) to form the LRP-FZD dimer complex that modulates cell differentiation and proliferation (Nusse and Clevers, 2017). Wnt’s expression is upregulated by exposure to UV (Yamada et al., 2019) and in response it connects with the LPR-FZD complex. This binding stabilizes the cytoplasmic β-catenin which then translocates to the nucleus where it binds with the T Cell/Lymphoid Enhancing (TCF/LEF) transcription factor to synergistically upregulate MITF gene expression (Takeda et al., 2000; Steingrímsson et al., 2004; Pillaiyar et al., 2017) (Figure 1), with direct downstream upregulating effects on melanogenic enzymes. This pathway cross talks with multiple others to impact melanin production: AhR signaling pathway (Bahraman et al., 2021) (Section 3.1), PKA cascade through α-MSH (Yamaguchi and Hearing, 2009) (Section 3.2), and PKB pathway through the Glycogen Synthase Kinase (GSK)-3β route (detailed herein).
The intricacies of AhR and Wnt/β-catenin pathways crosstalk are still not fully understood. Several studies using animal test systems or liver cells supported the impact of TCDD from tobacco smoke on AhR activation regulated by the Wnt/β-catenin signaling (Mathew et al., 2008; Procházková et al., 2011). Previous publications reported on a direct connection between the components of this intracellular route whereby β-catenin’s expression was strongly induced in melanocytes after exposure to AhR ligands (Nakamura et al., 2013). Another entry of AhR to the Wnt/β-catenin pathway is governed by the α-MSH-induced PKA cascade (Bahraman et al., 2021). These studies providing direct or indirect links between multiple signaling routes exemplify the complexity of the intracellular pathways contributing to melanin production as a response of melanocytes to stress factors.
Another crosstalk is based upon Wnt’s activity on the PKB pathway: when Wnt acts on its cell surface receptor LRP/FZD (He et al., 2004), Dishevelled (Dvl) cytoplasmic phosphoprotein acting directly downstream to FZD induces the accumulation of β-catenin in the cytoplasm by inhibiting the GSK-3β-dependent phosphorylation of β-catenin (Kishida et al., 2001). Accumulated β-catenin is then translocated into the nucleus where it follows the path described above to stimulate MITF and subsequently the melanogenic pathway. Also acting at this crossroads is TCDD, the high-affinity ligand of AhR, which was shown to be able to increase the phosphorylation level of PKB and GSK-3β (Xu et al., 2014) and to activate β-catenin (Al-Dhfyan, 2017). This crosstalk between AhR and PKB/GSK-3β/β-catenin pathway remains not thoroughly understood and likely not fully explored. To complicate the mechanistic profile even further, it has been reported that AhR could activate PKB in a ROS-dependent manner (Liao et al., 2014) or through binding Src kinase in the cell membrane (Ye et al., 2018). All these interactions between factors and intracellular routes have been supported by experiments in various cells lines, with no direct relation to keratinocytes or melanocytes. Therefore, work to verify them in skin lineages should be conducted to determine any direct implications on skin pigmentation in response to pollutants and tobacco. Other mechanisms are also possible and further investigations are surely needed to clarify the complexity of communication between these pathways.
3.5 Oxidative stress pathways
Air pollutants become bioavailable to the skin layers via nanoparticles and play a key role in the synthesis of quinones which are responsible for the production of ROS. Increased amounts of ROS and free radicals within the cells overcome skin’s innate enzymatic (glutathione peroxidase, glutathione reductase, superoxide dismutase, and catalase) and non-enzymatic antioxidant defense systems (vitamin E, vitamin C, and glutathione) (Thiele et al., 1997). The interaction of ROS and free radicals with the lipid-rich plasma membranes initiates a lipid peroxidation reaction that ultimately triggers an increase of metalloproteinases (MMPs) (Farage et al., 2008), which represent a key cross talking point between multiple pathways. ROS also stimulate the release of pro-inflammatory mediators, which results in a vicious cycle of inflammation and metabolic impairments, with downstream damaging activities on skin pigmentation (cross talking, see Section 3.6). Therefore, ROS are at the crossroads of multiple physiological pathways directly or indirectly impacted by environmental stimuli.
Epidermal melanocytes are especially susceptible to high ROS concentrations due to their specialized function to synthesize melanin through a process that itself induces oxidative stress. ROS are generated in the skin from sources that can be extrinsic (UV, pollution, tobacco) and intrinsic (metabolically generated pro-oxidants), and were shown to accelerate skin pigmentation in experiments using antioxidants. Keratinocytes adjacent to melanocytes have a significant contribution to UV-induced skin pigmentation. Furthermore, H2O2 (generated by UVB irradiation) (Schallreuter et al., 2004) activates epidermal phenylalanine hydroxylase, which produces L-Tyrosine from L-Phenylalanine, and thus contributes to melanogenesis by increasing the concentration of L-Tyrosine, the initial substrate of TYR (Figure 1—Melanin Synthesis Pathway). Additional studies have also demonstrated another mechanism involving H2O2 in the regulation of TYR via p53 through transcription of the hepatocyte nuclear factor (HNF)-1α, which in turn can also affect the POMC response (Schallreuter et al., 2008), with downstream action on the PKA pathway (see Section 3.2). Many studies reported that UV can induce the formation of ROS and Reactive Nitrogen Species (RNS) that act as signaling messengers to stimulate melanin production (Mastore et al., 2005; Dong et al., 2010; Kim and Lee, 2013) by increasing the amount of TYR and TYRP-1 (Sasaki et al., 2000). While the molecular mechanisms are still subject to investigation, studies showed that nitric oxide (NO) may play a vital role in the activation of TYR through guanylate cyclase and guanosine 3′,5′-cyclic monophosphate (cGMP)-dependent protein kinase (PKG) or by increasing its expression in melanocytes (Sasaki et al., 2000).
Through crosstalk, ROS activate the MAPK family subsequently inducing the activator protein 1 (AP-1), a transcription factor which plays an essential role in the transcriptional regulation of MMP-1, MMP-3, MMP-9, and MMP-12, found to be elevated in aged human skin (Shin et al., 2015; Mujahid et al., 2017). Nuclear factor-κB (NF-κB) is another transcription factor activated by ROS (Cui et al., 2007) and known to mediate the responses to UV irradiation and photo-aging, primarily by upregulation of MMP-1 and MMP-3 in dermal fibroblasts (Pittayapruek et al., 2016). In relation to pigmentation, upon melanocytes activation, their migration is initiated by the decoupling from the basement membrane (Haass and Herlyn, 2005) and further from keratinocytes (Birlea et al., 2017). MMPs induce the decoupling of melanocytes from keratinocytes and coordinate the attachment of melanocytes to the next epidermal unit. Therefore, it is conceivable that pollutants could impact this pathway and ultimately play a role in the associated stimulation of melanin synthesis, however more research in this regard is needed. For simplicity, these possible pathways are not included in Figure 1.
Of the pollutants known to impact the skin, BaP and hazardous dioxins activate AhR with a robust ROS generation, leading to acne and chloracne (Tsuji et al., 2011; Furue et al., 2014). The BaP/AhR signal also induces CYP1A1 (Li et al., 2003; Tsuji et al., 2011; Mescher et al., 2019), which further converts PAHs to quinones capable to upregulate ROS synthesis (Li et al., 2003). Furthermore, PM2.5 contains environmental metals and PAHs (Nel et al., 2006) that may increase the PAH-induced ROS generation (Chen and Lippmann, 2009; Magnani et al., 2016). Further supporting evidence was reported by Murphy et al., 2004 showing that the AhR pathway is activated by both TCDD and all-trans retinoic acid which increase MMP-1 expression in normal human keratinocytes. TCDD-induced expression of MMP-1 through AP-1 route (Murphy et al., 2004). Human melanocytes and some melanoma cell lines are activated by TCDD, and were shown to express both AhR and ARNT, and to increase MMP expression and activity following the exposure to toxicants (Villano et al., 2006). All these interactions are cross talking points between complex pathways that pollutants follow to impact melanin production in the skin.
In terms of tobacco’s participation to skin pigmentation, significantly increased levels of MMP-1 mRNA were observed in the dermal connective tissue of smokers compared to nonsmokers in a clinical study (Lahmann et al., 2001). Tobacco smoke extract induced the production of MMP-1 and MMP-3 and resulted in abnormal regulation of extracellular matrix deposition in human cultured skin fibroblasts (Yin et al., 2003). Moreover, tobacco smoke is a major source of PAHs exposure in humans. In this regard, it has been shown that tobacco smoke extract induced MMP-1 expression via activation of AhR signaling pathway in human fibroblasts and keratinocytes (Ono et al., 2013). Supportive evidence regarding the contribution of pollutants and tobacco to skin hyperpigmentation via ROS is also provided by multiple other studies (Vierkötter et al., 2010; Cho et al., 2012; Ishiwata et al., 2013; Delijewski et al., 2014; Peng et al., 2017; Leijs et al., 2018; Milani et al., 2019; Khmaladze et al., 2020; Krutmann et al., 2020; Suo et al., 2020; Yang et al., 2020; Chan et al., 2021; Grether-Beck et al., 2021; Moon et al., 2022) discussed in detail in Table 1.
3.6 Inflammation pathways
Skin exposure to air pollution toxicants induces mechanisms of cell detoxification which are active over extended periods of time and lead to elevated ROS levels and lipid peroxidation, resulting in skin alterations, including hyperpigmentation (Vierkötter et al., 2010; Patatian et al., 2021). Air pollution also induces inflammation, activates the AhR pathway, and leads to multiple alterations in skin’s physiology (Cho et al., 2012; Mancebo and Wang, 2015; Damevska et al., 2021; Nkengne et al., 2021). It seems that AhR is at the crossroads of these multiple pathways as it was shown to determine the severity of symptoms in chronic skin inflammation (Di Meglio et al., 2014). Its activation is essential for melanocyte survival and melanogenesis, which can be linked to the appearance of senile lentigines (Nakamura et al., 2015).
Recent research has shown that IL-33 stimulates phosphorylation of p38 and CREB, and consequently increases the expression of TYR, TYRP-1 and DCT through MITF, resulting in augmentation of melanogenesis (Zhou et al., 2014). Abundant IL-33 mRNA was found to be induced by UVB in human keratinocytes (Meephansan et al., 2012) and fibroblasts (Arend et al., 2008; Byrne et al., 2011), suggesting that skin’s response to stress factors leads to IL-33 release and ultimately to skin pigmentation. By involving mast cells (Ali et al., 2007; Moulin et al., 2007), macrophages (Schmitz et al., 2005), CD 4+T cells, basophils, dendritic cells and neutrophils, IL-33 is likely promoting Th2-skewed skin inflammation, which is another indirect effect on pigmentation (Hsu et al., 2020). Through activation of NF-kB, IL-33 may be able to regulate outcome of diseases such as atopic dermatitis, which can ultimately trigger pigmentation (Cevikbas and Steinhoff, 2012).
IL-18 is known to modulate both innate and adaptive immunity, and its dysregulation can cause autoimmune or inflammatory disease. Recent studies suggest its participation in regulation of pigmentation based on its activity to promote melanogenesis through upregulation of TYRP-1 and DCT expression (Zhou et al., 2013; Zhou et al., 2016), and by activating pathways that ultimately increase MITF’s expression. Furthermore, Chen et al., 2010 showed that the combination of keratinocyte growth factor (KGF) and IL-1α increased melanin deposition and is responsible for initial stage of human solar lentigines.
PGE2 is released in significant amounts by keratinocytes exposed to UV and in inflammatory conditions such as wound healing, and stimulates the formation of dendrites in melanocytes (Pentland et al., 1990; Pentland and Mahoney, 1990). PGE2 is released by keratinocytes and melanocytes through a process controlled by phospholipase A2 (PLA2), COX and prostaglandin E synthase (Gledhill et al., 2010). PGF2α is produced by fibroblasts and keratinocytes and was shown to stimulate melanocyte dendrite formation and TYR activation (Scott et al., 2005). Therefore, prostaglandins are potent inflammatory mediators with demonstrated activity on the melanogenic pathway and offer an established route for any pollutants to act upon. Further targeted experiments in this regard should be conducted to confirm this hypothesis.
Besides lentigines, post-inflammatory hyperpigmentation (PIH) is another skin condition typified by dark and flattened spots on the body (Markiewicz et al., 2022). Uneven skin tone is caused by exaggerated melanin production, often further aggravated by UV exposure. Inflammatory markers can also trigger PIH associated with skin conditions such as acne, atopic dermatitis, etc. (Kaufman et al., 2018). The PIH profile is characterized by production of cytokines, chemokines, and ROS during the inflammation that ultimately stimulates melanocyte growth, melanin production and its transfer to keratinocytes. Factors promoting these inflammatory events are EGF, IL-1, IL-6, tumor necrosis factor (TNF), leukotrienes (LT)C4 and LTD4, prostaglandins E2 and D2, and thromboxane-2 (Taylor et al., 2009). All these factors intersect on multiple other pathways that directly or indirectly augment the production of melanin in the skin and open wide the gates for environmental stimuli to enhance the pigmentation and accelerate skin aging. The direct connections however still await confirmation through thorough research that can be accomplished by NAMs that cast a wide mechanistic net and provide needed clarifications.
3.7 Nicotine: affinity to melanin
A significant body of evidence is reported in the scientific literature highlighting important aspects of the relationship between nicotine and melanin, primarily based on animal studies (Tsujimoto et al., 1975; Leeds and Turner, 1977). Nicotine itself and other tobacco constituents, specifically the carcinogens N′-nitrosonornicotine (NNN), 4-(methylnitrosamino)-1-(3-pyridyl)-1-butanone (NNK), and BaP, have been shown to accumulate in tissues containing melanin (Domellöf et al., 1987). Although many studies demonstrated that tobacco smoke causes skin aging mediated by its effects on fibroblasts and keratinocytes (Morita, 2007; Morita et al., 2009; Ono et al., 2013), narrowing of blood vessels (Argacha et al., 2008) and loss of vitamin C (reviewed by Carr and Rowe, 2020), only recently several studies reported on the mechanisms underlying smoker’s pigmentation.
Nicotine was shown to have affinity for melanin partly due to its precursor role in the pigment synthesis (Mizuno et al., 1997) and to its irreversible binding to the complex melanin molecules (King et al., 2009; Delijewski et al., 2014; Tamai et al., 2014). Nicotine and tobacco-specific toxic byproducts may also be trapped in tissues containing melanin (see also Section 3.10 regarding the possible contribution of adipose tissue), which results in extended exposure and associated health issues noticed in smokers (Szüts et al., 1978; Szparaga et al., 2021). The activity of melanogenesis enzymes may be a factor contributing to the release or degradation of toxic tobacco compounds (Brittebo and Tjälve, 1981). The role of melanin in tissue uptake of nicotine and tobacco-specific toxicants may be of particular concern to individuals with highly pigmented skin (Yerger and Malone, 2006). It was also shown that nicotine has the ability to form adducts with DOPAquinone, a precursor of melanin production (Dehn et al., 2001). Furthermore, nicotine can be incorporated in melanin molecules during hair formation (Stout and Ruth, 1999).
3.8 Cholinergic pathway
The cholinergic signaling system (nicotinic and muscarinic receptors) plays an important role in keratinocytes and melanocytes physiology (Kurzen et al., 2007; Wu et al., 2020a; Wu et al., 2020). Nicotine and related byproducts are known to impact the skin primarily by activating nicotinic acetylcholine receptors (nAChRs) (Grando et al., 2006) and downstream by mediating their complex actions in tobacco users (Benowitz and Hukkanen, 2009). Nicotine was shown to induce pigmentation in oral mucosal lesions following application of sublingual tablets (2 mg) for 3–6 months in a smoking cessation study (Wallström et al., 1999). Nicotine was also found to induce melanosome dispersion in dermal melanocytes (melanophores) of a teleostean fish (Visconti et al., 1984).
Within the cholinergic system, α7 nAChR plays a key regulatory role in modulating melanosome uptake in keratinocytes being induced by UV exposure (Figure 1). The exposure to UV is a stimulus to release acetylcholine from keratinocytes (Wu et al., 2020a; Wu et al., 2020), which further triggers the activation of α7 nAChR, localized on the surface of keratinocytes, leading to the intracellular influx of calcium. The mobilization of calcium ultimately initiates phagocytosis of melanosomes by keratinocytes (Guo et al., 2023).
While melanocytes appear to lack α7 nAChR, primary human sebocytes in vitro and sebaceous glands were reported to express the receptor (Li et al., 2013), which leaves room for speculation in terms of the contribution of adipose tissue to pigmentation when exposed to tobacco or other pollutants (see Section 3.10). Therefore, the evidence available thus far indicates that the impact of nicotine on pigmentation is mediated primarily by keratinocytes and their response to stimuli. Interesting reports showed some possibilities of signaling mediated by α7 nAChR however provided by cell lineages other than melanocytes. For example, the receptor was shown to transduce signals through the PI3K cascade in rat neuronal cells (Kihara et al., 2001), a path that in melanocytes acts directly onto MITF (see Section 3.4). Liu et al., 2017a showed using dopaminergic neurons that α7 nAChR mechanisms play a key role in a Parkinson’s disease mouse model via regulation of the Wnt/β-catenin signaling pathway, known to also impact pigmentation through the PKA and AhR synergistic effects. Since melanocytes derive from neural crests, this association can be explored from the perspective of the nicotinic receptors to determine if tobacco and pollutants can directly join the pathway and impact the melanin production in skin as a result.
3.9 Adrenergic pathway
The autocrine adrenergic intra- and intercellular signal transduction network in the human epidermis contributes significantly to the regulation of vital functions within the epidermal melanin unit. The epidermal adrenergic signals control multiple cellular processes such as cell growth and differentiation, motility, calcium homeostasis, and pigmentation via the α1-and β2-adrenoceptors.
It was established that human epidermis has the capacity to synthesize catecholamines (norepinephrine and epinephrine) from the essential amino acid L-phenylalanine. The first step leads to the production of L-Tyrosine catalyzed by phenylalanine hydroxylase in the presence of iron, molecular oxygen, and the cofactor/electron donor (6R)-L-erythro-5,6,7,8-tetrahydrobiopterin, followed by the rate-limiting step via tyrosine hydroxylase controlled by the same cofactor (Nagatsu et al., 1964) (Figures 1 – Catecholamines Synthesis). The binding of catecholamines to the β2-adrenergic receptor triggers the activation of cAMP production and the downstream PKA signaling pathway (cross talk, see Section 3.2) (Figure 1). Gillbro et al., 2004 demonstrated that a specific functional β2-adrenergic signal exists in human melanocytes leading to pigmentation through the cAMP/PKA pathway, which is now considered the main axis for the catecholamine control of melanogenesis. In a cross talking manner, the epidermal β2-adrenergic signaling also increases cAMP in response to release of epinephrine by keratinocytes, which results in calcium increase via activated PKC (Schallreuter et al., 1992; Koizumi et al., 1997). Therefore, cAMP can be considered the liaison between the PKC and PKA pathways.
It was also established that human melanocytes express α1-adrenoceptors, which are induced by norepinephrine yielding the IP3 and DAG signals (Figure 1). While the direct link between nicotine and adrenergic receptors in the skin is not supported currently by publications, indirect evidence comes to make the possibility worth investigating it further. For example, Choi et al., 2020 demonstrated the anti-melanogenic potential of carvedilol, a nonselective beta blocker with weak α1-blocking activities. Carvedilol reduced melanin content and cellular TYR activity without impact on cellular viability of human and mouse melanocytes. The compound also downregulated MITF, TYRP-1, DCT, and CREB.
Furthermore, the catecholamine synthesis pathway (Figure 1) uses Dopamine which is also involved directly in the melanin synthesis; therefore, increase in Dopamine in response to stimuli could increase the melanin production directly or indirectly.
3.10 Melanogenesis and the contribution of adipose tissue
PCBs and similar derivatives such as dioxins, polybrominated diphenyl ethers (PBDEs), hexabromocyclododecane (HBCD) etc. are often grouped under the umbrella of POPs. Included in this group even though it is not itself a POP is the endocrine disruptor bisphenol A (BPA) which acts as a POP. By sequestering POPs, the adipose tissue can protect other organs and tissues from toxic overload, however this storage may prove in long term detrimental to the body. A relatively recent study (Liu et al., 2017b) showed that PM2.5 inhibited sebocyte proliferation, reduced lipid synthesis and induced inflammatory cytokines IL-1α, IL-6, and IL-8. Additionally, the expression of AhR, ARNT, cytochrome P450 1A1 was significantly increased following PM2.5 exposure.
Even though the impact of pollutants on various cell lines was investigated, experiments targeting the adipose tissue are rather rare. Going further, from a pigmentation perspective, the contribution of adipose tissue is often overlooked and seldom researched with few exceptions. For example, Baranova et al., 2005 reported a statistically significant overexpression of TYR, DCT, melanosome transport protein RAB27a, and melan-A (MLANA) in visceral adipose tissue of morbidly obese patients. A later follow-up study by Randhawa et al., 2009 confirmed the expression of TYR, DCT, TYRP-1, and MITF and subsequent melanin biosynthesis in adipose tissue. Furthermore, TYR activity in the adipose tissue samples was much higher compared to samples collected from non-obese subjects. One of the limitations of investigating the impact of pollutants on pigmentation in adipose tissues is given by the incapacity of cultured adipocytes to sustain the enzymatic activity of TYR or the production of melanin, nor adequate post-translational modifications or the correct folding of TYR. We consider the participation of the adipose tissue to the melanin synthesis and its significant role in storing POPs with demonstrated impact on melanogenesis an avenue yet to be fully explored (see also Section 3.8 for further speculations on sebocytes possible contribution to pigmentation via the cholinergic pathway as they express the α7 nAChR).
3.11 IRE1 pathway involvement in correct folding of melanogenic enzymes
Proteins’ accumulation in the endoplasmic reticulum (ER) is a trigger of the unfolded protein response (UPR). Inositol-requiring protein 1 (Ire1), Protein Kinase R (PKR)-like endoplasmic reticulum kinase (Perk), and activating transcription factor (Atf)-6 are components of the UPR (Bertolotti et al., 2000). One very recent study showed that PM can increase melanin production in melanocyte, mouse skin, and human skin models. The expressions of unfolded protein response molecules was also increased in response to PM exposure. The IRE1α signaling pathway was consistently upregulated and it was shown to be further related to PM-triggered melanogenesis (Ahn et al., 2022).
PM treatment of melanocytes induced cAMP and the expressions of phosphorylated Calcium-Calmodulin-dependent Protein Kinase II (CAMKII) and CREB. In parallel, PKA was shown to induce IRE1α phosphorylation (Mao et al., 2011; Asada et al., 2015) which in turn phosphorylates CAMKII and CREB (Kim et al., 2010; Vo et al., 2019). Based on these discoveries, it was hypothesized that PM induced melanogenesis through PKA signaling in a cross talking manner with the IRE1α signaling pathway (Ahn et al., 2022). These preliminary results make the investigation of the folding pathways appealing in the attempt to determine if a direct impact of pollutants and tobacco exists, with downstream effects on skin hyperpigmentation. For simplicity this speculative pathway is not presented in Figure 1.
Investigation of Tyr folding in mouse melanoma cells was conducted using N-butyldeoxynojirimycin (NB-DNJ), an N-alkylated imino-sugar analog of glucose which inhibits α-glucosidase I and II activity. As a result, most glycans on Tyr molecule are arrested as glycosylated structures and prevented from undergoing any further processing (Branza-Nichita et al., 1999). This inhibition of early N-glycan processing in the ER may impact the maturation of the amino acid chain by preventing the correct association with ER chaperones calnexin/calreticulin responsible for folding. This study showed that NB-DNJ dramatically impacts the folding and maturation of Tyr. As a consequence, its enzymatic activity was almost entirely abolished, resulting in a complete loss of pigmentation in the treated cells. However, the enzyme was correctly transported to the melanosomes. It should be pointed out that NB-DNJ is the active ingredient in Zavesca (miglustat), which is an oral drug used to inhibit the biosynthesis of macromolecular substrates that accumulate pathologically in glycosphyinolipidoses such as type I Gaucher disease. While repurposing of drugs is a common practice in pharma, allowing an existing licensed drug to be reused for a different indication, this step has never been taken for cosmetic purposes. Since imino-sugar derivatives may hold potential as depigmenting agents and they could be considered as cosmetic candidates for the treatment of various pigmentary conditions such as senile lentigines, melasma, etc. (Costin et al., 2002), this possibility of repurposing is intriguing and appealing.
4 Testing platforms used to study pigmentation in the context of skin aging induced by pollution and tobacco
Manufacturers of products designed to address the damaging effects of pollutants and tobacco on skin face increasing demands for safe and efficacious products in response to population’s awareness of poor environmental conditions and their impact on life quality. Designing products that target modulation of skin tone is particularly challenging given the wide range of ethnic skin types. This is an aspect that needs to be taken into account in addition to variation in seasonal weather patterns, differences in life styles and personal care and cosmetics usage and associated benefit expectations (Mistry, 2017). Society nowadays exercises a sophisticated inquisitiveness exemplified by demands for more ethical, natural formulations in cosmetic research, supported by mechanistic rationales to motivate the consumer consider the next product on the market (Juliano and Magrini, 2017). While challenging to explain complex molecular pathways in marketing terms accessible to masses, this demand is to the advantage of the scientists who reported multiple lines of evidence not only regarding the mechanisms of pollutants’ action onto skin and pigmentation, but also explored those mechanisms to design potent ingredients and finished products. Leveraging the existing population habits and an in-depth understanding of cellular and molecular mechanisms that contribute to the efficacy of products helped science deliver anti-pollution benefits.
Currently, there are no established standardized anti-pollution tests or international guidelines for product development. The many different types of methodologies we discussed thus far (in vitro, ex vivo, in vivo and clinical) and summarized in Table 1 are usually designed as fit for purpose and may be used to support efficacy claims addressing tobacco use or pollution exposure as causes of hyperpigmentation which is a hallmark of skin aging. Many brands rely on supplier data (Spencer, 2016; Whitehouse, 2016) while others use post-marketing surveillance data for claims support. However, in vitro and clinical test data should be used as they provide direct support to establish safety and efficacy of products with biological activity. As such, we will attempt in this section of the manuscript to narrow down the contender methods and endpoints that could be used as a portfolio of tests to research the impact of pollution and tobacco on skin and to support the efficacy of actives to counteract their damaging effect on pigmentation.
4.1 Position of NAMs in the cosmetic science research framework
Our analysis of the studies reporting data regarding the impact of pollution and tobacco on skin pigmentation revealed two key aspects: 1) there is more research conducted and/or published on pollution compared to tobacco, with a visible focus on PM; and 2) overall, the NAMs are used more frequently compared to animal or clinical studies, which is an approach we encourage to continue and be further explored.
For example, we identified 16 manuscripts reporting on pollution’s contribution to skin hyperpigmentation of which 11 addressed the PM (and 2 of those were specifically on DEPs), 4 were on PAHs and 1 for PCBs (Table 1) in terms of the type of toxicant. Regarding the study design, the manuscripts reported a total number of 32 experiments conducted as follows (determined using the length of treatment column): 7 clinical studies, 15 cell-based methods, 2 based on reconstructed tissue models, 4 on ex vivo explants and 4 using animal test systems. As already mentioned, PM was thoroughly investigated using a comprehensive portfolio of in vitro, in vivo, ex vivo and clinical approaches, thus providing a fuller picture on how PM impacts skin pigmentation, at a macroscopic and molecular, mechanistic level. This approach based primarily on NAMs should be expanded and applied for the research of other pollutants in order to obtain fast, human-relevant, reliable mechanistic insights. The cell-based assays used to investigate pollution’s contribution to hyperpigmentation were based on primary or immortalized human keratinocytes or melanocytes of various degrees of pigment producing capacity; in rare cases mouse melanocytes were used (Krutmann et al., 2008; Luecke et al., 2010; Abbas et al., 2017). In general, the endpoints of interest were conducted for safety assessment (viability, apoptosis) or to gain mechanistic knowledge on pollution’s impact on the key players of the melanogenic pathway. Of these assays, many were biochemical (enzymatic activity, melanin content) (Suo et al., 2020; Shi et al., 2021), histological, based on gene and protein expression (Suo et al., 2020; Yang et al., 2020; Shi et al., 2021; Yang et al., 2022), including key markers of various intracellular pathways. Of similar versatility in terms of endpoints and data output were the ex vivo platforms and those based on reconstructed tissue models (Krutmann et al., 2020; Grether-Beck et al., 2021). The animal studies usually employed histological endpoints or assessed melanin content, melanocyte density (Krutmann et al., 2008; Abbas et al., 2017), while clinical studies were focused primarily on the impact of pollutants on skin color at a macroscopic level (Vierkötter et al., 2010; Peng et al., 2017; Nkengne et al., 2021) (refer to Section 4.3; Subsection 4.3.3. Endpoints for more details on endpoints).
For tobacco research analyzed in our manuscript (Table 1), the majority of studies were clinical (8), with a small number of pre-clinical studies (3) based on cell culture models using human melanocytes to address the effect of cigarette smoke (Nakamura et al., 2013) and nicotine (Delijewski et al., 2014) on enzymatic activity relating to melanin production. While the majority of the clinical studies address skin pigmentation on a surface level, few others have collected more than “skin deep” measurements by analyzing bodily fluids for enzymes (King et al., 2009; Dalrymple et al., 2022) and metabolites (Liakoni et al., 2019).
Our analysis draws encouraging conclusions regarding the importance and position of NAMs in cosmetic research focused on environmental toxicants and provides an up-to-date view to support the advances made by this field relying less and less on animal testing. Using NAMs provides several advantages over animal or clinical studies to evaluate the effects of air pollutants and tobacco on skin pigmentation and aging. Multiple alternative methods based on the 3Rs were developed in response to increasing ethical concerns regarding animal experimentation (Russell and Burch, 1959). The 3Rs principles were first used in 1998 when the United Kingdom implemented the first total ban on animal-tested cosmetic products. Besides overcoming ethical concerns related to animal test systems, in vitro methodologies offer other scientific advantages over animal studies such as reproducibility and high throughput, which support complex mechanistic studies to investigate the activity of toxicants on skin and the design of actives to counteract them. Animal or clinical studies are reliant on availability of animal species and human volunteers, which can increase the time to recruit and conduct these lengthy studies that provide limited molecular insights about cellular pathways. Animal testing can be performed on a variety of species, however the results are not entirely reflective of a human skin exposure mainly because of the differences in architectures and immune responses between animal and human skin. With careful selection, proper training and skillful handling, NAMs are now indispensable for modern dermatology and skin aging research. In vitro anti-pollution tests facilitate a deeper understanding of changes occurring in the skin cells. One of the biggest advantages of these assays is the control of specified conditions and the endless possibilities to customize the testing parameters to fit the purpose of the experiments. In an animal test system or in clinical studies, this flexibility is not always possible, thus limiting the mechanistic data output.
4.2 Use of NAMs to design potent actives counteracting effects of pollution and tobacco on skin pigmentation
We integrated in Table 1 the existing studies that took advantage of the knowledge reported on mechanisms of action used by pollution and tobacco on skin pigmentation to design efficacious actives to counteract their deleterious effects. We identified a total of 5 reports and all of those only addressed the efficacy of actives on pollution exposure and impact on skin pigmentation. Of the total of 14 experiments these studies reported on, 1 was a clinical study, 9 were cell-based and 4 used ex vivo human explants. Even though based on a relatively small number of studies, our analysis draw several important conclusions as basis for future directions discussed in more details later: 1) efficacy of raw ingredients and finished products to counteract tobacco’s impact on skin pigmentation is either not researched or published on; 2) it is encouraging to see that no animal studies and a limited number (1) of clinical studies were conducted while the vast majority of the results were generated using cell- and explant-based experiments. The limited number of publications is somewhat understood or justified given the competitive market and the need to maintain a reasonable margin without disclosing confidential intellectual property information. However, to the benefit of the consumer, of the science supporting safe and efficacious products, encouraging the scientists to publish will avoid duplicating efforts and delaying launching of important products on the market.
There exist several active compounds, natural extracts and vitamins that have been investigated and showed capacity to inhibit or reverse the effects of pollution on skin pigmentation. Cultural preference plays a major role in the design and priority testing of anti-pollution products. For example, while tanned skin is often considered attractive in the United States, many Asian countries consider fair skin to be preferable (Khmaladze et al., 2020) and hyperpigmentation due to pollution is a concern. Highly populated cities in China, India and Pakistan have a correspondingly high level of pollution which is a growing consumer concern when considering cosmetic products (Mistry, 2017). This growing global awareness of pollution affecting the skin generated an increased cultural demand for anti-pollution products.
As outlined in Table 1, in vitro, ex vivo and clinical studies have all been used to assess the efficacy of these products. Clinical studies can address changes in skin color and epidermal morphological changes. In vitro methods (cell-based) can address pigmentation changes the human eye cannot identify such as gene expression, cell viability or death, cytokine activity and melanin content. Ex vivo models can also address many of these “below the surface” activities but can be more difficult to obtain as a reliable test system, in addition to the population variability that should be considered. In this context, science advanced tremendously and provides nowadays commercially available pigmented reconstructed tissue models that can not only eliminate the sourcing issue and population variability, but also offer the possibility to compare models of various phenotypes and explore their responses to toxicants in terms of skin pigmentation (see Section 4.3; Subsection 4.3.1. Test system).
Natural extracts from plants such as Deschampsia Antarctica (Milani et al., 2019; Fernández-Martos et al., 2021) and Korean Red Ginseng (Moon et al., 2022) were evaluated for anti-pollution claims. In a clinical study, Deschampsi Antarctica reduced dark spots (Milani et al., 2019); the study by Fernández-Martos et al., 2021 supported mechanistically the claims on this natural extract and identified the AhR and inflammatory pathways as those on which the extract is effective. Furthermore, the same study used an ex vivo human skin model that was pre-treated with Deschampsia Antarctica and then exposed to different pollutants. The pre-treatment prevented downregulation of E-cadherin receptors and overexpression of AhR which were shown to be in response to pollutants (Fernández-Martos et al., 2021). Pre-treatment of human keratinocytes, co-culture of human keratinocytes and melanocytes and murine macrophages with Korean Red Ginseng mitigated the increase of melanin in response to PM10 treatment (Moon et al., 2022). These datasets indicate another key aspect of NAMs: they allow the design of the experiments accommodate a preventive (pre-treatment) or therapeutic (post-exposure treatment) approach which may be difficult to execute in a clinical or animal setting. In this way, the potential of these ingredients to prevent or to treat the effects of pollutants can be addressed and is supported with mechanistic justification.
Besides botanical extracts which are very appealing given their natural origin (for a comprehensive review see Mistry, 2017), various chemically derived actives were of interest and tested using cell-based assays or as formulations using explants. For example, actives A, B, C and D (see Table 1 for definitions) were tested on human keratinocytes, human dermal fibroblasts, human melanocytes and a co-culture of melanocytes and keratinocytes (Khmaladze et al., 2020). In general, a pre-treatment or treatment with the actives upregulated genes involved in the regulation of melanogenic pathway, and inhibited melanin and cytokine expression. A formulation of the active ingredients applied to an ex vivo human skin model also inhibited genes related to expression of pigmentation. Vitamins C and E formulated with active ingredients (see Table 1) applied onto an ex vivo human skin model reduced hyperpigmentation and gene expression of MMP-1 (Krutmann et al., 2020).
Overall, the products currently offered on the market address several mechanisms of action in terms of routes by which pollution impacts skin pigmentation: oxidative stress, inflammation, direct action on the melanin pathway (through MITF, Moon et al., 2022), and the AhR governed pathway (Table 1). The fact that the knowledge gained din academic research is now applied to design efficacious products is a huge step, which should be further encouraged, supported and published.
4.3 Where do we go from here? Study design: points to consider
Based on our analysis of the pollutants reported to impact skin pigmentation, on the current status of test systems, endpoints and mechanisms of action, we summarize below several key findings and points in support of future directions research could consider in this field.
4.3.1 Test system
When considering the appropriate and relevant experimental design to investigate the impact pollutants and tobacco have on skin pigmentation or to test the efficacy of products with anti-pollution claims, several options exist depending on the endpoint desired. Human immortalized melanocytes and keratinocytes are relatively easy to obtain through established commercial vendors such as American Type Culture Collection (ATCC) (Manassas, VA, United States) and can be maintained for years at a low cost. Specific lines can also be obtained from academic sources, however they need to be carefully and thoroughly characterized and tested for reliability over time. Using a 2D (cellular) model to test anti-pollution properties can provide information on melanin content, cell viability and changes in gene expression. This is a great model when testing individual ingredients for mechanistic purposes as often cellular assays are dilution based to ensure bioavailability of the ingredient to the cells.
Formulations often contain a multitude of ingredients and therefore solubility can be challenging, so a more robust test system such as ex vivo or reconstructed (3D) tissue models would be more appropriate. The formulation can be applied directly to the surface of the skin model for a longer duration which would be more reflective of the application of the final product. Using a 3D model can give insight on full penetration of cell layers, anti-inflammatory response, gene expression and cell viability, however they are often more expensive than a 2D model. Ex vivo models can provide similar endpoints as a reconstructed tissue model but are limited on donor availability. Clinical studies can also use a final formulation product for multiple applications or longer exposures, however they have several limitations such as expense, variability between subjects, and high variety of protocols used.
There exist several reconstructed human pigmented tissue model systems that could accommodate the testing of cosmetic ingredients and formulations. Suppliers such as BioCell Biotechnology (Guangdong, China - MelaKutis model), Creative Bioarray (Frankfurt am Main, Germany - 3D human Pigmented Skin Model), EpiSkin (Lyon, France - Reconstructed Human Pigmented Epidermis, RHPE, models), MatTek Corporation (Ashland, MA, United States-Melanoderm™), Phenion (Henkel) (Düsseldorf, Germany-epics-M), StratiCELL (Les Isnes, Belgium-various models mimicking pigmentary skin conditions), and TegoScience (Seoul, Korea-NeoDerm-ME) offer these tissue models commercially (Gendreau et al., 2013). Using an established model from a reputable source (i.e., not “home grown”) not only ensures reproducibility in testing but also standardizes the model across multiple laboratories.
At the time of this review, there were no research reports assessing the effect of tobacco on pigmentation using a reconstructed human tissue model. We identify this to be a major area to focus testing of tobacco products on. In addition to the advantages of using a NAM to assess pigmentation changes resulting from tobacco, technology exists that can deliver a full dose of tobacco smoke directly to the reconstructed human tissue model. Smoke chambers such as the Vitrocell Cloud™ chamber (Vitrocell System GmbH, Germany) can be utilized in combination with a 3D model for a robust testing system. Commercially available cigarettes can be “puffed” directly onto the surface of the tissue, replicating a real life exposure to cigarette smoke (Lecas et al., 2016; Prieux et al., 2020; Seurat et al., 2021). After exposure, a variety of endpoints can be used to assess pigmentation changes such as viability, melanin content and distribution, histological analysis and gene expression. This approach has the obvious advantage of replicating in vitro the end-user exposure to whole smoke (particulate and gas phases) that other NAMs cannot accommodate.
The NAMs based on 2D and 3D test systems could be used in a tiered approach. Once the individual toxicants/ingredients are assessed in a screening approach using cell-based assays, the final formulation can be applied directly to ex vivo or 3D tissues for safety and efficacy investigations using a plethora of endpoints that exist (biochemical, histological, gene expression, etc.). Multiple forms and concentrations of the toxicants/ingredients can be applied topically to the more robust test system, to mimic the end-user exposure, or in the media, to mimic a systemic exposure. These approaches cannot be accommodated in cell-based assays and they represent a great advantage of explants and reconstructed tissue models. A repetitive dose of a cosmetic product can also be applied in a 3D model, aligning more with a real life application and avoiding any challenges of losing the cell population during manipulations, especially with non-adherent cells. After treatment, the media can be collected for subsequent analysis of multiple makers specific to molecular pathways of interest.
Depending on the results of the in vitro testing, clinical studies may be applicable. However as described above for the length of time required and reliance on volunteers, clinical testing can be inappropriate if a quick, screening result is desired. Compared to the suite of NAMs we covered in this review, the clinical studies offer the advantage of being able to accommodate a well-rounded testing approach by assessing all parts of atmospheric pollution such as the PM, PAH’s, DEP’s, heavy metals, etc. A clinical study comparing individuals who live in a highly polluted area to those who live in a more rural, less polluted area could provide a fuller picture on the effects of anti-pollution products on the skin (Vierkötter et al., 2010; Peng et al., 2017; Flament et al., 2018; Nkengne et al., 2021). Similarly, tobacco smoke contains more chemical classes than just nicotine and clinical studies can investigate the effects of both the particulate and gas phases. During our analysis, we identified results obtained in seemingly similarly designed clinical studies that were discordant, all of them on tobacco research. For example, the results obtained by Cho et al., 2012 correlated with those obtained by Serri et al., 2010 showing that melanin index values decreased after smoking cessation, however they were not confirmed by Ishiwata et al., 2013 or by Yazdanparast et al., 2019, though the latter investigated the melanin content in the skin of smokers vs. non-smokers (Table 1). Furthermore, studies by King et al., 2009; Tamai et al., 2014 reported correlative results in increased melanin in smokers vs. non-smokers, while the study by Liakoni et al., 2019 did not, likely because in this study the nicotine was administered intravenously.
The clinical studies and NAMs should be used in a tiered, step-wise approach and to fit the purpose of the research. Furthermore, if the possibility of paired in vitro-clinical data exists, it should be taken into consideration especially for screening active candidates designed to counteract the impacts of pollutants and tobacco on skin pigmentation. If any NAMs can be identified as good predictors of clinical studies outcome, the strategy can be used to inform clinical studies and reduce their size, scope, price and length.
4.3.2 Treatment options: toxicants, ingredients, and finished products
Both air pollutants and tobacco are characterized by a huge complexity of components which are in a combined particulate and gaseous form. Studies indicated that the gas phase contains the most harmful toxicants produced by combustion. Therefore, to mimic a “real-life” exposure, both phases should be considered as part of hazard and risk assessment. While many studies investigated the mechanisms responsible for occurrence of melasma, lentigines, etc., their conclusions are tightly linked to the form of toxicant used, which in most of the cases overlooked the gas phase. Therefore, this is an area of critical consideration for future research.
In terms of PM in particular, the most common particles used in vitro and in vivo studies investigating their effects on skin are Standard Reference Materials® from the National Institute of Standards and Technology, 2023 (NIST) (NIST, NP). They represent diesel PM, PM2.5, and a variety of other standards that are geographically specific by collection site. The Joint Research Centre, 2023 (JRC) of the European Commission produces European Reference Materials (ERM) that are used in studies simulating airborne PM (JRC, NP). JRC also provides urban dust particles resembling PM10-like urban dust (with or without PAHs). The National Institute for Environmental Studies (NIES) in Japan offers a PM10-like urban dust certified reference material that was collected from a central ventilating system in a building in Beijing, China (Mori et al., 2008). Several studies reported collection of PM2.5 from seasonal dust storms in Asia and West Africa using an in-house filtering system. Other authors used concentrated ambient air particles (PM2.5) for skin model exposure (Dijkhoff et al., 2020).
In terms of raw ingredients and finished products, industry exercises due diligence in selecting safe and efficacious candidates that are tested for confirmation. Ingredients acting on multiple pathways can be combined and tested for synergistic effects within a finished formula. The ingredients should be tested for toxicity in a model which addresses the activation of these possible pathways to generate a dose response curve, ensuring the ingredient itself is not causing any adverse cytotoxic effects. Once non-toxic concentrations are established, the cells can be pre-treated with the ingredient. The ingredient can be rinsed off and environmental toxins added and compared to a group without pre-treatment for various endpoints such as melanin content and expression of genes encoding for factors relevant to multiple intracellular pathways.
4.3.3 Endpoints
As listed and discussed in detail in Table 1, the endpoints relevant to the pathways governing the melanin production in human skin are of a wide variety. As we indicated in regards to the test system and toxicants thus far, the key is to work with established systems, from commercial sources, with characterized, stable and reliable materials. Given the wide diversity of the endpoints that exist, standardization is lacking in terms of which endpoints to use when action of toxicants or actives on pigmentation is investigated. Industry could take the lead and work together to identify the most relevant and reliable safety and efficacy endpoints. Even if a set of standardized assays would be generated, specific/unique endpoints may still be needed when researching novel pathway that may be of interest. The assays should be qualified for these specific endpoints and cut-off values and ranges established if companies decide using historical databases to deem prototypes safe and efficacious. It is also very important for industry to qualify benchmark materials that can be used to compare the prototypes to in terms of safety and efficacy. As with any assays, proper positive and negative controls should be included in order to assess the performance of each test system and endpoint selected.
Outside the scope of pigmentation itself which was our main interest in this manuscript, other skin aging endpoints can provide insight into how pollution and tobacco affect the skin as a whole. For example, clinical studies often look at multiple skin health factors using the Score of Intrinsic and Extrinsic Skin Aging (SCINEXA) scoring system. This includes measurements of pigment spots but also measures coarse wrinkles, elastosis, telangiectasia, skin laxity and seborrheic keratosis (Vierkötter et al., 2010). Other measurements such as Trans Epidermal Water Loss (TEWL), pH, erythema content, sebum, and skin elasticity and friction can also be performed (Yazdanparast et al., 2019). These are useful for both pollution and tobacco effects because constitutive and facultative skin pigmentation can be measured on one individual but also address population diversity if that is a goal of the research.
The NAMs offer multiple options in terms of endpoints related to skin aging besides those targeting pigmentation. For example, PM exposed to normal human epidermal keratinocytes (NHEKs) decreased the number and size of primary cilia, an organelle responsible for cell to cell signaling for growth factors, nutrients and hormones in the skin (Bae et al., 2019). Ex vivo models have investigated how tobacco decreases skin hydrophobicity and induces changes to the lipid bilayer in the stratum corneum (Percoco et al., 2021). Hammer et al. (2011) used an ex vivo test system (fresh adult full-thickness skin from breast plastic surgery) to investigate the toxicity of cigarette smoke attached to textiles; the study showed that the contaminated textiles represent a potential source of nicotine uptake by skin and induce further adverse effects. Tobacco smoke exposed to a reconstructed human tissue model affected the skin layers, increased production of ROS, and induced an anti-inflammatory response (Lecas et al., 2016). A full thickness human skin model exposed to cigarette smoke (Rasmussen et al., 2010) has demonstrated an increase in ROS generation. Glycation as a reflection of chronological aging was investigated in a reconstructed skin model integrating monocytes in order to address multiple pathways (Pageon et al., 2017). Active compounds (Dead Sea minerals and PolluStop®) were evaluated on a reconstructed human tissue model for irritation and inflammation of the skin and found to reduce both when used together (Portugal-Cohen et al., 2017). Feverfew (a natural antioxidant extract) applied to a human skin model (StrataTest®) reduced ROS levels elevated by PM exposure (Rasmussen et al., 2010).
Pigmented reconstructed models have been used for endpoints related to skin aging though not directly linked to hyperpigmentation induced by toxicants. Some pigmented 3D models were used to investigate the continuous information exchange within the epidermal melanin unit (Bessou-Touya et al., 1998; Hall et al., 2022). Others became more stable in culture (Gibbs et al., 2000 – for 6 weeks; Zoio et al., 2021-50 days) or more complex through addition of fibroblasts as another conversation partner for keratinocytes and melanocytes and important regulator of pigmentation (Hedley et al., 2002; Zöller et al., 2019). Studies reported on the use of 3D models containing these 3 cell lineages to investigate in-depth the cell-cell, cell-matrix and mesenchymal-epithelial interactions that control skin pigmentation (Duval et al., 2012). Diekmann et al. (2016) took this concept a step further by inducing aging to a model generated with melanocytes, keratinocytes and fibroblasts by using Mitomycin-C which was shown previously to induce accelerated senescence in human dermal fibroblast cultures. Gledhill et al., 2015 investigated the melanin transfer in the first human 3D model generated from induced pluripotent stem cells (iPSCs)-derived fibroblasts, keratinocytes and melanocytes. The advantages of this model are enormous in that the contribution of all these cell lineages is possible and it replicates the native skin environment. The complexity of these models allows investigations into endpoints specific for skin aging. The presence of melanocytes in these test systems should qualify them for experiments investigating the impact of pollutants on pigmentation or to screen ingredients with potential to reverse the activity of toxicants on skin pigmentation.
5 Concluding remarks
In this review we provided an overview of the air pollution and tobacco smoke as stress factors that increase pigmentation in the context of extrinsic-induced skin aging and the mechanisms by which they do so. Understanding these mechanisms is of great interest to industries whose main focus is on anti-pollution and anti-tobacco-induced aging skin care and cosmetic products that became an ever increasing necessity due to worsening air quality around the world. In certain cultures, skin color is often related to hierarchies of race, gender, caste and ethnicity and is unavoidably the first phenotypic feature one observes during human interactions given its external positioning within our body. Any minute manipulation of the skin color becomes immediately visible macroscopically since the melanin biosynthesis pathway acts as a barometer in response to voluntary treatments or uncontrollable stress factors. Research into skin pigmentation targets the melanocytes and the tight relationship with the keratinocytes within the epidermal melanin unit. The melanocytes are located deeply in the skin, yet their activity is so highly visible as portrayed by the uniqueness and limitless variety of ethnic skin tones. Equally “praised” for generating beautiful skin color tones and “blamed” for racial discrimination, the inconspicuous melanocytes are probably the most impactful cell type in the human body at a global level. In this manuscript we first traveled deep into the skin to rediscover the melanocytes and identify their response to environmental toxicants. We brought back to surface their message that helps us decipher new mechanisms of actions and molecular pathways and the secrets of how to create solutions in the form of efficacious products. The melanocytes generously equip the scientists with the keys to open the doors to the next-generation of skin lightening actives, the miracle anti-aging cream or natural/non-UV tanning procedure. The possibilities to research a wide variety of pollutants open up an entire new field that awaits for its mysteries be discovered by making use of an equally diverse portfolio of NAMs at our disposal.
Author contributions
KVB: Conceptualization, Investigation, Writing–original draft, Writing–review and editing. G-EC: Conceptualization, Investigation, Writing–original draft, Writing–review and editing, Funding acquisition, Supervision.
Funding
The author(s) declare that no financial support was received for the research, authorship, and/or publication of this article.
Conflict of interest
Authors KVB and G-EC work for the Institute for In Vitro Sciences, Inc. (IIVS).
Publisher’s note
All claims expressed in this article are solely those of the authors and do not necessarily represent those of their affiliated organizations, or those of the publisher, the editors and the reviewers. Any product that may be evaluated in this article, or claim that may be made by its manufacturer, is not guaranteed or endorsed by the publisher.
References
Abbas, S., Alam, S., Singh, K. P., Kumar, M., Gupta, S. K., and Ansari, K. M. (2017). Aryl hydrocarbon receptor activation contributes to benzanthrone-induced hyperpigmentation via modulation of melanogenic signaling pathways. Chem. Res. Toxicol. 30, 625–634. doi:10.1021/acs.chemrestox.6b00364
Abd, E., Yousef, S. A., Pastore, M. N., Telaprolu, K., Mohammed, Y. H., Namjoshi, S., et al. (2016). Skin models for the testing of transdermal drugs. Clin. Pharmacol. 8, 163–176. doi:10.2147/CPAA.S64788
Agostinis, P., Garmyn, M., and Van Laethem, A. (2007). The aryl hydrocarbon receptor: an illuminating effector of the UVB response. Sci. STKE. 403, pe49. doi:10.1126/stke.4032007pe49
Ahn, Y., Lee, E. J., Luo, E., Choi, J., Kim, J. Y., Kim, S., et al. (2022). Particulate matter promotes melanin production through endoplasmic reticulum stress‒mediated IRE1α signaling. J. Invest. Dermatol. 142, 1425–1434.e6. doi:10.1016/j.jid.2021.08.444
Al-Dhfyan, A., Alhoshani, A., and Korashy, H. M. (2017). Aryl hydrocarbon receptor/cytochrome P450 1A1 pathway mediates breast cancer stem cells expansion through PTEN inhibition and β-Catenin and Akt activation. Mol. Cancer. 16, 14. Erratum in: Mol Cancer. 2017 Mar 24;16(1):69. doi:10.1186/s12943-016-0570-y
Alaluf, S., Atkins, D., Barrett, K., Blount, M., Carter, N., and Heath, A. (2002). Ethnic variation in melanin content and composition in photoexposed and photoprotected human skin. Pigment. Cell Res. 15, 112–118. doi:10.1034/j.1600-0749.2002.1o071.x
Ali, S., Huber, M., Kollewe, C., Bischoff, S. C., Falk, W., and Martin, M. U. (2007). IL-1 receptor accessory protein is essential for IL-33-induced activation of T lymphocytes and mast cells. Proc. Natl. Acad. Sci. U. S. A. 104, 18660–18665. doi:10.1073/pnas.0705939104
Arend, W. P., Palmer, G., and Gabay, C. (2008). IL-1, IL-18, and IL-33 families of cytokines. Immunol. Rev. 223, 20–38. doi:10.1111/j.1600-065X.2008.00624.x
Argacha, J. F., Adamopoulos, D., Gujic, M., Fontaine, D., Amyai, N., Berkenboom, G., et al. (2008). Acute effects of passive smoking on peripheral vascular function. Hypertension 51, 1506–1511. doi:10.1161/HYPERTENSIONAHA.107.104059
Asada, R., Kanemoto, S., Matsuhisa, K., Hino, K., Cui, M., Cui, X., et al. (2015). IRE1α-XBP1 is a novel branch in the transcriptional regulation of Ucp1 in brown adipocytes. Sci. Rep. 5, 16580. doi:10.1038/srep16580
Bae, J. E., Kang, G. M., Min, S. H., Jo, D. S., Jung, Y. K., Kim, K., et al. (2019). Primary cilia mediate mitochondrial stress responses to promote dopamine neuron survival in a Parkinson's disease model. Cell Death Dis. 10, 952. doi:10.1038/s41419-019-2184-y
Bae-Harboe, Y. S., and Park, H. Y. (2012). Tyrosinase: A central regulatory protein for cutaneous pigmentation. J. Invest. Dermatol. 132, 2678–2680. doi:10.1038/jid.2012.324
Bahraman, A. G., Jamshidzadeh, A., Keshavarzi, M., Arabnezhad, M. R., Mohammadi, H., and Mohammadi-Bardbori, A. (2021). α-Melanocyte-Stimulating Hormone triggers melanogenesis via activation of the aryl hydrocarbon receptor pathway in B16F10 mouse melanoma cells. Int. J. Toxicol. 40, 153–160. doi:10.1177/1091581820987548
Baranova, A., Collantes, R., Gowder, S. J., Elariny, H., Schlauch, K., Younoszai, A., et al. (2005). Obesity-related differential gene expression in the visceral adipose tissue. Obes. Surg. 15, 758–765. doi:10.1381/0960892054222876
Baroli, B., Ennas, M. G., Loffredo, F., Isola, M., Pinna, R., and López-Quintela, M. A. (2007). Penetration of metallic nanoparticles in human full-thickness skin. J. Invest. Dermatol. 127, 1701–1712. doi:10.1038/sj.jid.5700733
Basrur, V., Yang, F., Kushimoto, T., Higashimoto, Y., Yasumoto, K., Valencia, J., et al. (2003). Proteomic analysis of early melanosomes: identification of novel melanosomal proteins. J. Proteome. Res. 2, 69–79. doi:10.1021/pr025562r
Belote, R. L., and Simon, S. M. (2020). Ca2+ transients in melanocyte dendrites and dendritic spine-like structures evoked by cell-to-cell signaling. J. Cell Biol. 219, e201902014. doi:10.1083/jcb.201902014
Benowitz, N. L., Hukkanen, J., and Jacob, P. (2009). Nicotine chemistry, metabolism, kinetics and biomarkers. Handb. Exp. Pharmacol. 192, 29–60. doi:10.1007/978-3-540-69248-5_2
Bertolotti, A., Zhang, Y., Hendershot, L. M., Harding, H. P., and Ron, D. (2000). Dynamic interaction of BiP and ER stress transducers in the unfolded-protein response. Nat. Cell Biol. 2, 326–332. doi:10.1038/35014014
Bessou-Touya, S., Picardo, M., Maresca, V., Surlève-Bazeille, J. E., Pain, C., and Taïeb, A. (1998). Chimeric human epidermal reconstructs to study the role of melanocytes and keratinocytes in pigmentation and photoprotection. J. Invest. Dermatol. 111, 1103–1108. doi:10.1046/j.1523-1747.1998.00405.x
Bieker, J. J. (2001). Krüppel-like factors: three fingers in many pies. J. Biol. Chem. 276, 34355–34358. doi:10.1074/jbc.R100043200
Birlea, S. A., Costin, G. E., Roop, D. R., and Norris, D. A. (2017). Trends in regenerative medicine: repigmentation in vitiligo through melanocyte stem cell mobilization. Med. Res. Rev. 37, 907–935. doi:10.1002/med.21426
Bonaventure, J., Domingues, M. J., and Larue, L. (2013). Cellular and molecular mechanisms controlling the migration of melanocytes and melanoma cells. Pigment. Cell Melanoma Res. 26, 316–325. doi:10.1111/pcmr.12080
Branza-Nichita, N., Petrescu, A. J., Dwek, R. A., Wormald, M. R., Platt, F. M., and Petrescu, S. M. (1999). Tyrosinase folding and copper loading in vivo: A crucial role for calnexin and alpha-glucosidase II. Biochem. Biophys. Res. Commun. 261, 720–725. doi:10.1006/bbrc.1999.1030
Brittebo, E. B., and Tjälve, H. (1981). Formation of tissue-bound N'-nitrosonornicotine metabolites by the target tissues of Sprague-Dawley and Fisher rats. Carcinogenesis 2, 959–963. doi:10.1093/carcin/2.10.959
Burke, K. E. (2018). Mechanisms of aging and development-A new understanding of environmental damage to the skin and prevention with topical antioxidants. Mech. Ageing Dev. 172, 123–130. doi:10.1016/j.mad.2017.12.003
Byrne, S. N., Beaugie, C., O'Sullivan, C., Leighton, S., and Halliday, G. M. (2011). The immune-modulating cytokine and endogenous Alarmin interleukin-33 is upregulated in skin exposed to inflammatory UVB radiation. Am. J. Pathol. 179, 211–222. doi:10.1016/j.ajpath.2011.03.010
Carr, A. C., and Rowe, S. (2020). Factors affecting vitamin C status and prevalence of deficiency: A global health perspective. Nutrients 12, 1963. doi:10.3390/nu12071963
Cevikbas, F., and Steinhoff, M. (2012). IL-33: A novel danger signal system in atopic dermatitis. J. Invest. Dermatol. 132, 1326–1329. doi:10.1038/jid.2012.66
Chan, T. K., Bramono, D., Bourokba, N., Krishna, V., Wang, S. T., Neo, B. H., et al. (2021). Polycyclic aromatic hydrocarbons regulate the pigmentation pathway and induce DNA damage responses in keratinocytes, a process driven by systemic immunity. J. Dermatol. Sci. 104, 83–94. doi:10.1016/j.jdermsci.2021.09.003
Chatelain, M., Gasparini, J., Jacquin, L., and Frantz, A. (2014). The adaptive function of melanin-based plumage coloration to trace metals. Biol. Lett. 10, 20140164. doi:10.1098/rsbl.2014.0164
Chen, L. C., and Lippmann, M. (2009). Effects of metals within ambient air particulate matter (PM) on human health. Inhal. Toxicol. 21, 1–31. doi:10.1080/08958370802105405
Chen, N., Hu, Y., Li, W. H., Eisinger, M., Seiberg, M., and Lin, C. B. (2010). The role of keratinocyte growth factor in melanogenesis: A possible mechanism for the initiation of solar lentigines. Exp. Dermatol. 19, 865–872. doi:10.1111/j.1600-0625.2009.00957.x
Cho, Y. H., Jeong, D. W., Seo, S. H., Lee, S. Y., Choi, E. J., Kim, Y. J., et al. (2012). Changes in skin color after smoking cessation. Korean J. Fam. Med. 33, 105–109. doi:10.4082/kjfm.2012.33.2.105
Choi, M. E., Yoo, H., Lee, H. R., Moon, I. J., Lee, W. J., Song, Y., et al. (2020). Carvedilol, an adrenergic blocker, suppresses melanin synthesis by inhibiting the cAMP/CREB signaling pathway in human melanocytes and ex vivo human skin culture. Int. J. Mol. Sci. 21, 8796. doi:10.3390/ijms21228796
Coelho, S. G., Choi, W., Brenner, M., Miyamura, Y., Yamaguchi, Y., Wolber, R., et al. (2009). Short- and long-term effects of UV radiation on the pigmentation of human skin. J. Investig. Dermatol. Symp. Proc. 14, 32–35. doi:10.1038/jidsymp.2009.10
Costin, G. E., and Hearing, V. J. (2007). Human skin pigmentation: melanocytes modulate skin color in response to stress. FASEB J. 21, 976–994. doi:10.1096/fj.06-6649rev
Costin, G. E., Trif, M., Nichita, N., Dwek, R. A., and Petrescu, S. M. (2002). pH-sensitive liposomes are efficient carriers for endoplasmic reticulum-targeted drugs in mouse melanoma cells. Biochem. Biophys. Res. Commun. 293, 918–923. doi:10.1016/S0006-291X(02)00317-0
Cui, R., Widlund, H. R., Feige, E., Lin, J. Y., Wilensky, D. L., Igras, V. E., et al. (2007). Central role of p53 in the suntan response and pathologic hyperpigmentation. Cell 128, 853–864. doi:10.1016/j.cell.2006.12.045
D'Mello, S. A., Finlay, G. J., Baguley, B. C., and Askarian-Amiri, M. E. (2016). Signaling pathways in melanogenesis. Int. J. Mol. Sci. 17, 1144. doi:10.3390/ijms17071144
Dalrymple, A., McEwan, M., Brandt, M., Bielfeldt, S., Bean, E. J., Moga, A., et al. (2022). A novel clinical method to measure skin staining reveals activation of skin damage pathways by cigarette smoke. Skin. Res. Technol. 28, 162–170. doi:10.1111/srt.13108
Damevska, K., Simeonovski, V., Darlenski, R., and Damevska, S. (2021). How to prevent skin damage from air pollution part 2: current treatment options. Dermatol. Ther. 34, e15132. doi:10.1111/dth.15132
Debeer, S., Le Luduec, J. B., Kaiserlian, D., Laurent, P., Nicolas, J. F., Dubois, B., et al. (2013). Comparative histology and immunohistochemistry of porcine versus human skin. Eur. J. Dermatol. 23, 456–466. doi:10.1684/ejd.2013.2060
Dehn, D. L., Claffey, D. J., Duncan, M. W., and Ruth, J. A. (2001). Nicotine and cotinine adducts of a melanin intermediate demonstrated by matrix-assisted laser desorption/ionization time-of-flight mass spectrometry. Chem. Res. Toxicol. 14, 275–279. doi:10.1021/tx000205l
Delijewski, M., Wrześniok, D., Otręba, M., Beberok, A., Rok, J., and Buszman, E. (2014). Nicotine impact on melanogenesis and antioxidant defense system in HEMn-DP melanocytes. Mol. Cell. Biochem. 395, 109–116. doi:10.1007/s11010-014-2116-1
Di Meglio, P., Duarte, J. H., Ahlfors, H., Owens, N. D., Li, Y., Villanova, F., et al. (2014). Activation of the aryl hydrocarbon receptor dampens the severity of inflammatory skin conditions. Immunity 40, 989–1001. doi:10.1016/j.immuni.2014.04.019
Diekmann, J., Alili, L., Scholz, O., Giesen, M., Holtkötter, O., and Brenneisen, P. (2016). A three-dimensional skin equivalent reflecting some aspects of in vivo aged skin. Exp. Dermatol. 25, 56–61. doi:10.1111/exd.12866
Dijkhoff, I. M., Drasler, B., Karakocak, B. B., Petri-Fink, A., Valacchi, G., Eeman, M., et al. (2020). Impact of airborne particulate matter on skin: A systematic review from epidemiology to in vitro studies. Fibre Toxicol. 17, 35. doi:10.1186/s12989-020-00366-y
Domellöf, L., Andersson, M., Tjälve, H., Veals, S., Trushin, N., and Hecht, S. S. (1987). Distribution and metabolism of N'-nitrosonornicotine in the miniature pig. Carcinogenesis 8, 1741–1747. doi:10.1093/carcin/8.11.1741
Dong, Y., Cao, J., Wang, H., Zhang, J., Zhu, Z., Bai, R., et al. (2010). Nitric oxide enhances the sensitivity of alpaca melanocytes to respond to alpha-melanocyte-stimulating hormone by up-regulating melanocortin-1 receptor. Biochem. Biophys. Res. Commun. 396, 849–853. doi:10.1016/j.bbrc.2010.05.001
Duval, C., Chagnoleau, C., Pouradier, F., Sextius, P., Condom, E., and Bernerd, F. (2012). Human skin model containing melanocytes: essential role of keratinocyte growth factor for constitutive pigmentation-functional response to α-melanocyte stimulating hormone and forskolin. Tissue Eng. Part. C. Methods. 18, 947–957. doi:10.1089/ten.TEC.2011.0676
Esser, C., Bargen, I., Weighardt, H., Haarmann-Stemmann, T., and Krutmann, J. (2013). Functions of the aryl hydrocarbon receptor in the skin. Semin. Immunopathol. 35, 677–691. doi:10.1007/s00281-013-0394-4
Estrada, C., Mirabal-Ortega, L., Méry, L., Dingli, F., Besse, L., Messaoudi, C., et al. (2022). MITF activity is regulated by a direct interaction with RAF proteins in melanoma cells. Commun. Biol. 5, 101. doi:10.1038/s42003-022-03049-w
Farage, M. A., Miller, K. W., Elsner, P., and Maibach, H. I. (2008). Intrinsic and extrinsic factors in skin ageing: A review. Int. J. Cosmet. Sci. 30, 87–95. doi:10.1111/j.1468-2494.2007.00415.x
Fernández-Martos, S., Calvo-Sánchez, M. I., Lobo-Aldezabal, A., Sánchez-Adrada, A. I., Moreno, C., Vitale, M., et al. (2021). The deleterious effects induced by an acute exposure of human skin to common air pollutants are prevented by extracts of Deschampsia Antarctica. Sci. Rep. 11, 23751. doi:10.1038/s41598-021-03190-2
Flament, F., Bourokba, N., Nouveau, S., Li, J., and Charbonneau, A. (2018). A severe chronic outdoor urban pollution alters some facial aging signs in Chinese women. A tale of two cities. Int. J. Cosmet. Sci. 40, 467–481. doi:10.1111/ics.12487
Furue, M., Takahara, M., Nakahara, T., and Uchi, H. (2014). Role of AhR/ARNT system in skin homeostasis. Arch. Dermatol. Res. 306, 769–779. doi:10.1007/s00403-014-1481-7
Gendreau, I., Angers, L., Jean, J., and Poulio, R. (2013). Pigmented skin models: understand the mechanisms of melanocytes. Intech Chapter 30, 759–785. doi:10.5772/55565
Gibbs, S., Murli, S., De Boer, G., Mulder, A., Mommaas, A. M., and Ponec, M. (2000). Melanosome capping of keratinocytes in pigmented reconstructed epidermis--effect of ultraviolet radiation and 3-isobutyl-1-methyl-xanthine on melanogenesis. Pigment. Cell Res. 13, 458–466. doi:10.1034/j.1600-0749.2000.130608.x
Gillbro, J. M., Marles, L. K., Hibberts, N. A., and Schallreuter, K. U. (2004). Autocrine catecholamine biosynthesis and the beta-adrenoceptor signal promote pigmentation in human epidermal melanocytes. J. Invest. Dermatol. 123, 346–353. doi:10.1111/j.0022-202X.2004.23210.x
Gledhill, K., Guo, Z., Umegaki-Arao, N., Higgins, C. A., Itoh, M., and Christiano, A. M. (2015). Melanin transfer in human 3D skin equivalents generated exclusively from induced pluripotent stem cells. PLoS One 10, e0136713. doi:10.1371/journal.pone.0136713
Gledhill, K., Rhodes, L. E., Brownrigg, M., Haylett, A. K., Masoodi, M., Thody, A. J., et al. (2010). Prostaglandin-E2 is produced by adult human epidermal melanocytes in response to UVB in a melanogenesis-independent manner. Pigment. Cell Melanoma Res. 23, 394–403. doi:10.1111/j.1755-148X.2010.00696.x
Goiran, C., Bustamante, P., and Shine, R. (2017). Industrial melanism in the seasnake Emydocephalus annulatus. Curr. Biol. 27, 2510–2513. doi:10.1016/j.cub.2017.06.073
Gordon, P. R., and Gilchrest, B. A. (1989). Human melanogenesis is stimulated by diacylglycerol. J. Invest. Dermatol. 93, 700–702. doi:10.1111/1523-1747.ep12319900
Grando, S. A., Pittelkow, M. R., and Schallreuter, K. U. (2006). Adrenergic and cholinergic control in the biology of epidermis: physiological and clinical significance. J. Invest. Dermatol. 126, 1948–1965. doi:10.1038/sj.jid.5700151
Grether-Beck, S., Felsner, I., Brenden, H., Marini, A., Jaenicke, T., Aue, N., et al. (2021). Air pollution-induced tanning of human skin. Br. J. Dermatol. 185, 1026–1034. doi:10.1111/bjd.20483
Guo, M. S., Wu, Q., Dong, T. T., and Tsim, K. W. K. (2023). The UV-induced uptake of melanosome by skin keratinocyte is triggered by α7 nicotinic acetylcholine receptor-mediated phagocytosis. FEBS J. 290, 724–744. doi:10.1111/febs.16613
Gupta, M. A., and Gilchrest, B. A. (2005). Psychosocial aspects of aging skin. Dermatol Clin. 23, 643–648. doi:10.1016/j.det.2005.05.012
Haass, N. K., and Herlyn, M. (2005). Normal human melanocyte homeostasis as a paradigm for understanding melanoma. J. Investig. Dermatol. Symp. Proc. 10, 153–163. doi:10.1111/j.1087-0024.2005.200407.x
Hall, M. J., Lopes-Ventura, S., Neto, M. V., Charneca, J., Zoio, P., Seabra, M. C., et al. (2022). Reconstructed human pigmented skin/epidermis models achieve epidermal pigmentation through melanocore transfer. Pigment. Cell Melanoma Res. 35, 425–435. doi:10.1111/pcmr.13039
Hammer, T. R., Fischer, K., Mueller, M., and Hoefer, D. (2011). Effects of cigarette smoke residues from textiles on fibroblasts, neurocytes and zebrafish embryos and nicotine permeation through human skin. Int. J. Hyg. Environ. Health. 214, 384–391. doi:10.1016/j.ijheh.2011.04.007
He, X., Semenov, M., Tamai, K., and Zeng, X. (2004). LDL receptor-related proteins 5 and 6 in wnt/beta-catenin signaling: arrows point the way. Development 131, 1663–1677. doi:10.1242/dev.01117
Hearing, V. J. (1997) The regulation of melanin production. In Drug discovery approaches for developing cosmeceuticals, advanced skin care and cosmetic products (W. Hori, ed) pp. 3.1.1–3.1.21, IBC Library Series, Southborough, Massachusetts
Hedley, S. J., Layton, C., Heaton, M., Chakrabarty, K. H., Dawson, R. A., Gawkrodger, D. J., et al. (2002). Fibroblasts play a regulatory role in the control of pigmentation in reconstructed human skin from skin types I and II. Pigment. Cell Res. 15, 49–56. doi:10.1034/j.1600-0749.2002.00067.x
Hsu, C. L., Chhiba, K. D., Krier-Burris, R., Hosakoppal, S., Berdnikovs, S., Miller, M. L., et al. (2020). Allergic inflammation is initiated by IL-33-dependent crosstalk between mast cells and basophils. PLoS One 15, e0226701. doi:10.1371/journal.pone.0226701
Huang, G., and Elferink, C. J. (2012). A novel nonconsensus xenobiotic response element capable of mediating aryl hydrocarbon receptor-dependent gene expression. Mol. Pharmacol. 81, 338–347. doi:10.1124/mol.111.075952
Ikuta, T., Namiki, T., Fujii-Kuriyama, Y., and Kawajiri, K. (2009). AhR protein trafficking and function in the skin. Biochem. Pharmacol. 77, 588–596. doi:10.1016/j.bcp.2008.10.003
Ishiwata, T., Seyama, K., Hirao, T., Shimada, K., Morio, Y., Miura, K., et al. (2013). Improvement in skin color achieved by smoking cessation. Int. J. Cosmet. Sci. 35, 191–195. doi:10.1111/ics.12025
Jin, S. P., Li, Z., Choi, E. K., Lee, S., Kim, Y. K., Seo, E. Y., et al. (2018). Urban particulate matter in air pollution penetrates into the barrier-disrupted skin and produces ROS-dependent cutaneous inflammatory response in vivo. J. Dermato.l Sci. S0923-1811 (18), 175–183. doi:10.1016/j.jdermsci.2018.04.015
Joint Research Centre (JRC) (2023). Certified reference materials catalogue of the JRC. Available at: https://crm.jrc.ec.europa.eu/(Accessed on June 26, 2023).
Juliano, C., and Magrini, G. A. (2017). Cosmetic functional ingredients from botanical sources for anti-pollution skincare products. Cosmetics 5, 19. doi:10.3390/cosmetics5010019
Jux, B., Kadow, S., Luecke, S., Rannug, A., Krutmann, J., and Esser, C. (2011). The aryl hydrocarbon receptor mediates UVB radiation-induced skin tanning. J. Invest. Dermatol. 131, 203–210. doi:10.1038/jid.2010.269
Kampa, M., and Castanas, E. (2008). Human health effects of air pollution. Environ. Pollut. 151, 362–367. doi:10.1016/j.envpol.2007.06.012
Kaufman, B. P., Aman, T., and Alexis, A. F. (2018). Postinflammatory hyperpigmentation: epidemiology, clinical presentation, pathogenesis and treatment. Am. J. Clin. Dermatol. 19, 489–503. doi:10.1007/s40257-017-0333-6
Kennedy, C., Bastiaens, M. T., Bajdik, C. D., Willemze, R., Westendorp, R. G., Bouwes Bavinck, J. N., et al. (2003). Effect of smoking and sun on the aging skin. J. Invest. Dermatol. 120, 548–554. doi:10.1046/j.1523-1747.2003.12092.x
Khmaladze, I., Österlund, C., Smiljanic, S., Hrapovic, N., Lafon-Kolb, V., Amini, N., et al. (2020). A novel multifunctional skin care formulation with a unique blend of antipollution, brightening and antiaging active complexes. J. Cosmet. Dermatol. 19, 1415–1425. doi:10.1111/jocd.13176
Kihara, T., Shimohama, S., Sawada, H., Honda, K., Nakamizo, T., Shibasaki, H., et al. (2001). Alpha 7 nicotinic receptor transduces signals to phosphatidylinositol 3-kinase to block A beta-amyloid-induced neurotoxicity. J. Biol. Chem. 276, 13541–13546. doi:10.1074/jbc.M008035200
Kim, H. E., and Lee, S. G. (2013). Induction of ATP synthase β by H2O2 induces melanogenesis by activating PAH and cAMP/CREB/MITF signaling in melanoma cells. Int. J. Biochem. Cell Biol. 45, 1217–1222. doi:10.1016/j.biocel.2013.03.006
Kim, Y. H., Joo, H. S., and Kim, D. S. (2010). Nitric oxide induction of IRE1-alpha-dependent CREB phosphorylation in human glioma cells. Nitric Oxide 23, 112–120. doi:10.1016/j.niox.2010.04.009
King, G., Yerger, V. B., Whembolua, G. L., Bendel, R. B., Kittles, R., and Moolchan, E. T. (2009). Link between facultative melanin and tobacco use among African Americans. Pharmacol. Biochem. Behav. 92, 589–596. doi:10.1016/j.pbb.2009.02.011
Kishida, M., Hino, Si., Michiue, T., Yamamoto, H., Kishida, S., Fukui, A., et al. (2001). Synergistic activation of the Wnt signaling pathway by Dvl and casein kinase Iepsilon. J. Biol. Chem. 276, 33147–33155. doi:10.1074/jbc.M103555200
Koizumi, H., Tanaka, H., and Ohkawara, A. (1997). beta-Adrenergic stimulation induces activation of protein kinase C and inositol 1,4,5-trisphosphate increase in epidermis. Exp. Dermatol. 6 (3), 128–132. doi:10.1111/j.1600-0625.1997.tb00159.x
Koohgoli, R., Hudson, L., Naidoo, K., Wilkinson, S., Chavan, B., and Birch-Machin, M. A. (2017). Bad air gets under your skin. Exp. Dermatol. 26, 384–387. doi:10.1111/exd.13257
Krutmann, J., Grether-Beck, S., Valois, A., Brenden, H., Uthe, I., Felsner, I., et al. (2020). 17789 an antioxidant cocktail–containing cosmetic product (Liftactive Cure) prevents air pollution–induced skin hyperpigmentation: results from the ex vivo Düsseldorf Pollution Skin Test. J. Am. Acad. Dermatol. 83, AB199. doi:10.1016/j.jaad.2020.06.883
Krutmann, J., Jux, B., Luecke, S., Fritsche, E., Abel, J., Esser, C., et al. (2008). Involvement of arylhydrocarbon receptor (AhR)-signaling in skin melanogenesis. J. Invest. Dermatol. 128, S220.
Krutmann, J., Liu, W., Li, L., Pan, X., Crawford, M., Sore, G., et al. (2014). Pollution and skin: from epidemiological and mechanistic studies to clinical implications. J. Dermatol. Sci. 76, 163–168. doi:10.1016/j.jdermsci.2014.08.008
Kurzen, H., Wessler, I., Kirkpatrick, C. J., Kawashima, K., and Grando, S. A. (2007). The non-neuronal cholinergic system of human skin. Horm. Metab. Res. 39, 125–135. doi:10.1055/s-2007-961816
La Merrill, M., Emond, C., Kim, M. J., Antignac, J. P., Le Bizec, B., Clément, K., et al. (2013). Toxicological function of adipose tissue: focus on persistent organic pollutants. Environ. Health Perspect. 121, 162–169. doi:10.1289/ehp.1205485
Lademann, J., Schaefer, H., Otberg, N., Teichmann, A., Blume-Peytavi, U., and Sterry, W. (2004). Penetration of microparticles into human skin. Hautarzt 55, 1117–1119. German. doi:10.1007/s00105-004-0841-1
Lahmann, C., Bergemann, J., Harrison, G., and Young, A. R. (2001). Matrix metalloproteinase-1 and skin ageing in smokers. Lancet 357, 935–936. doi:10.1016/S0140-6736(00)04220-3
Lecas, S., Boursier, E., Fitoussi, R., Vié, K., Momas, I., Seta, N., et al. (2016). In vitro model adapted to the study of skin ageing induced by air pollution. Toxicol. Lett. 259, 60–68. doi:10.1016/j.toxlet.2016.07.026
Leeds, J., and Turner, D. M. (1977). Factors affecting the tissue binding of nicotine in various species. Biochem. Pharmacol. 26, 1631–1635. doi:10.1016/0006-2952(77)90080-6
Leijs, M. M., Esser, A., Amann, P. M., Schettgen, T., Gube, M., Merk, H. F., et al. (2018). Hyperpigmentation and higher incidence of cutaneous malignancies in moderate-high PCB- and dioxin exposed individuals. Environ. Res. 164, 221–228. doi:10.1016/j.envres.2018.02.002
Li, N., Hao, M., Phalen, R. F., Hinds, W. C., and Nel, A. E. (2003). Particulate air pollutants and asthma. A paradigm for the role of oxidative stress in PM-induced adverse health effects. Clin. Immunol. 109, 250–265. doi:10.1016/j.clim.2003.08.006
Li, Z. J., Park, S. B., Sohn, K. C., Lee, Y., Seo, Y. J., Kim, C. D., et al. (2013). Regulation of lipid production by acetylcholine signalling in human sebaceous glands. J. Dermatol. Sci. 72, 116–122. doi:10.1016/j.jdermsci.2013.06.009
Liakoni, E., St Helen, G., Dempsey, D. A., Jacob, P., Tyndale, R. F., and Benowitz, N. L. (2019). Relationship between skin melanin index and nicotine pharmacokinetics in African American smokers. Drug Alcohol Depend. 204, 107474. doi:10.1016/j.drugalcdep.2019.04.039
Liao, T. L., Chen, S. C., Tzeng, C. R., and Kao, S. H. (2014). TCDD induces the hypoxia-inducible factor (HIF)-1α regulatory pathway in human trophoblastic JAR cells. Int. J. Mol. Sci. 15, 17733–17750. doi:10.3390/ijms151017733
Liu, Y., Hao, S., Yang, B., Fan, Y., Qin, X., Chen, Y., et al. (2017a). Wnt/β-catenin signaling plays an essential role in α7 nicotinic receptor-mediated neuroprotection of dopaminergic neurons in a mouse Parkinson's disease model. Biochem. Pharmacol. 140, 115–123. doi:10.1016/j.bcp.2017.05.017
Liu, Q., Wu, J., Song, J., Liang, P., Zheng, K., Xiao, G., et al. (2017b). Particulate matter 2.5 regulates lipid synthesis and inflammatory cytokine production in human SZ95 sebocytes. Int. J. Mol. Med. 40, 1029–1036. doi:10.3892/ijmm.2017.3109
Luecke, S., Backlund, M., Jux, B., Esser, C., Krutmann, J., and Rannug, A. (2010). The aryl hydrocarbon receptor (AHR), a novel regulator of human melanogenesis. Pigment. Cell Melanoma Res. 23, 828–833. doi:10.1111/j.1755-148X.2010.00762.x
Magnani, N. D., Muresan, X. M., Belmonte, G., Cervellati, F., Sticozzi, C., Pecorelli, A., et al. (2016). Skin damage mechanisms related to airborne particulate matter exposure. Toxicol. Sci. 149, 227–236. doi:10.1093/toxsci/kfv230
Mancebo, S. E., and Wang, S. Q. (2015). Recognizing the impact of ambient air pollution on skin health. J. Eur. Acad. Dermatol. Venereol. 29, 2326–2332. doi:10.1111/jdv.13250
Mao, T., Shao, M., Qiu, Y., Huang, J., Zhang, Y., Song, B., et al. (2011). PKA phosphorylation couples hepatic inositol-requiring enzyme 1alpha to glucagon signaling in glucose metabolism. Proc. Natl. Acad. Sci. U. S. A. 108, 15852–15857. doi:10.1073/pnas.1107394108
Markiewicz, E., Karaman-Jurukovska, N., Mammone, T., and Idowu, O. C. (2022). Post-inflammatory hyperpigmentation in dark skin: molecular mechanism and skincare implications. Clin. Cosmet. Investig. Dermatol. 15, 2555–2565. doi:10.2147/CCID.S385162
Mastore, M., Kohler, L., and Nappi, A. J. (2005). Production and utilization of hydrogen peroxide associated with melanogenesis and tyrosinase-mediated oxidations of DOPA and dopamine. FEBS J. 272, 2407–2415. doi:10.1111/j.1742-4658.2005.04661.x
Masuda, Y. (1985). Health status of Japanese and Taiwanese after exposure to contaminated rice oil. Environ. Health. Perspect. 60, 321–325. doi:10.1289/ehp.8560321
Mathew, L. K., Sengupta, S. S., Ladu, J., Andreasen, E. A., and Tanguay, R. L. (2008). Crosstalk between AHR and Wnt signaling through R-Spondin1 impairs tissue regeneration in zebrafish. FASEB J. 22, 3087–3096. doi:10.1096/fj.08-109009
Matsumura, F. (2009). The significance of the nongenomic pathway in mediating inflammatory signaling of the dioxin-activated Ah receptor to cause toxic effects. Biochem. Pharmacol. 77, 608–626. doi:10.1016/j.bcp.2008.10.013
Meephansan, J., Tsuda, H., Komine, M., Tominaga, S., and Ohtsuki, M. (2012). Regulation of IL-33 expression by IFN-γ and tumor necrosis factor-α in normal human epidermal keratinocytes. J. Invest. Dermatol. 132, 2593–2600. doi:10.1038/jid.2012.185
Mescher, M., Tigges, J., Rolfes, K. M., Shen, A. L., Yee, J. S., Vogeley, C., et al. (2019). The Toll-like receptor agonist imiquimod is metabolized by aryl hydrocarbon receptor-regulated cytochrome P450 enzymes in human keratinocytes and mouse liver. Arch. Toxicol. 93, 1917–1926. doi:10.1007/s00204-019-02488-5
Milac, A. L., and Negroiu, G. (2018). The multiple roles of tyrosinase-related protein-2/L- Dopachrome tautomerase in melanoma: biomarker, therapeutic target, and molecular driver in tumor progression. InTech. doi:10.5772/intechopen.70513
Milani, M., Hashtroody, B., Piacentini, M., and Celleno, L. (2019). Skin protective effects of an antipollution, antioxidant serum containing Deschampsia antartica extract, ferulic acid and vitamin C: A controlled single-blind, prospective trial in women living in urbanized, high air pollution area. Clin. Cosmet. Investig. Dermatol. 12, 393–399. doi:10.2147/CCID.S204905
Mistry, N. (2017). Guidelines for formulating anti-pollution products. Cosmetics 4, 57. doi:10.3390/cosmetics4040057
Mizuno, A., Uematsu, T., Ishikawa, T., Yoshimine, N., and Nakashima, M. (1997). Clinical outcome of smoking-cessation trial of nicotine chewing gum evaluated by analysis of nicotine in hair. Ther. Drug Monit. 19, 407–412. doi:10.1097/00007691-199708000-00008
Moon, I. J., Kim, W., Kim, S. Y., Lee, J., Yoo, H., Bang, S., et al. (2022). Saponins of Korean Red Ginseng may protect human skin from adipokine-associated inflammation and pigmentation resulting from particulate matter exposure. Nutrients 14, 845. doi:10.3390/nu14040845
Mori, I., Sun, Z., Ukachi, M., Nagano, K., McLeod, C. W., Cox, A. G., et al. (2008). Development and certification of the new NIES CRM 28: urban aerosols for the determination of multielements. Anal. Bioanal. Chem. 391, 1997–2003. doi:10.1007/s00216-008-2076-y
Morita, A. (2007). Tobacco smoke causes premature skin aging. J. Dermatol. Sci. 48, 169–175. doi:10.1016/j.jdermsci.2007.06.015
Morita, A., Torii, K., Maeda, A., and Yamaguchi, Y. (2009). Molecular basis of tobacco smoke-induced premature skin aging. J. Investig. Dermatol. Symp. Proc. 14, 53–55. doi:10.1038/jidsymp.2009.13
Moulin, D., Donzé, O., Talabot-Ayer, D., Mézin, F., Palmer, G., and Gabay, C. (2007). Interleukin (IL)-33 induces the release of pro-inflammatory mediators by mast cells. Cytokine 40, 216–225. doi:10.1016/j.cyto.2007.09.013
Mujahid, N., Liang, Y., Murakami, R., Choi, H. G., Dobry, A. S., Wang, J., et al. (2017). A UV-independent topical small-molecule approach for melanin production in human skin. Cell Rep. 19, 2177–2184. doi:10.1016/j.celrep.2017.05.042
Murphy, K. A., Villano, C. M., Dorn, R., and White, L. A. (2004). Interaction between the aryl hydrocarbon receptor and retinoic acid pathways increases matrix metalloproteinase-1 expression in keratinocytes. J. Biol. Chem. 279, 25284–25293. doi:10.1074/jbc.M402168200
Nagatsu, T., Levitt, M., and Udenfriend, S. (1964). A rapid and simple radioassay for tyrosine hydroxylase activity. Anal. Biochem. 9, 122–126. doi:10.1016/0003-2697(64)90092-2
Naik, N. N., Vadloori, B., Poosala, S., Srivastava, P., Coecke, S., Smith, A., et al. (2023). Advances in animal models and cutting-edge research in alternatives: Proceedings of the third International Conference on 3Rs Research and Progress, Vishakhapatnam, 2022. Altern. Lab. Anim. 51, 263–288. doi:10.1177/02611929231180428
Nakamura, M., Morita, A., Seité, S., Haarmann-Stemmann, T., Grether-Beck, S., and Krutmann, J. (2015). Environment-induced lentigines: formation of solar lentigines beyond ultraviolet radiation. Exp. Dermatol. 24, 407–411. doi:10.1111/exd.12690
Nakamura, M., Nishida, E., and Morita, A. (2016). Action spectrum of melanoblast maturation and involvement of the aryl hydrocarbon receptor. Exp. Dermatol. 25 (3), 41–44. doi:10.1111/exd.13088
Nakamura, M., Ueda, Y., Hayashi, M., Kato, H., Furuhashi, T., and Morita, A. (2013). Tobacco smoke-induced skin pigmentation is mediated by the aryl hydrocarbon receptor. Exp. Dermatol. 22, 556–558. doi:10.1111/exd.12170
National Institute of Standards and Technology (NIST) (2023). Standard reference materials. Available at: https://www.nist.gov/srm (Accessed on June 26, 2023).
Nebert, D. W. (2017). Aryl hydrocarbon receptor (AHR): "pioneer member" of the basic-helix/loop/helix per-Arnt-sim (bHLH/PAS) family of "sensors" of foreign and endogenous signals. Prog. Lipid. Res. 67, 38–57. doi:10.1016/j.plipres.2017.06.001
Nel, A., Xia, T., Mädler, L., and Li, N. (2006). Toxic potential of materials at the nanolevel. Science 311, 622–627. doi:10.1126/science.1114397
Nkengne, A., Robic, J., and Lua, B. L. (2021). The effect of air pollution on the skin colour and tone of Chinese women: A multicentre cohort study. Skin. Res. Technol. 27, 428–434. doi:10.1111/srt.12953
Noakes, R. (2015). The aryl hydrocarbon receptor: A review of its role in the physiology and pathology of the integument and its relationship to the tryptophan metabolism. Int. J. Tryptophan Res. 8, 7–18. doi:10.4137/IJTR.S19985
Nusse, R., and Clevers, H. (2017). Wnt/β-catenin signaling, disease, and emerging therapeutic modalities. Cell 169, 985–999. doi:10.1016/j.cell.2017.05.016
Ono, Y., Torii, K., Fritsche, E., Shintani, Y., Nishida, E., Nakamura, M., et al. (2013). Role of the aryl hydrocarbon receptor in tobacco smoke extract-induced matrix metalloproteinase-1 expression. Exp. Dermatol. 22, 349–353. doi:10.1111/exd.12148
Ostrowski, S. M., and Fisher, D. E. (2019). “Chapter 20: pigmentation and melanocyte biology,” in Fitzpatrick’s Dermatology, 9e. Editors S. Kang, M. Amagai, A. L. Bruckner, A. H. Enk, D. J. Margolis, A. J. McMichaelet al. (McGraw Hill).
Pageon, H., Zucchi, H., Rousset, F., Girardeau-Hubert, S., Tancrede, E., and Asselineau, D. (2017). Glycation stimulates cutaneous monocyte differentiation in reconstructed skin in vitro. Mech. Ageing Dev. 162, 18–26. doi:10.1016/j.mad.2017.02.001
Park, H. Y., Perez, J. M., Laursen, R., Hara, M., and Gilchrest, B. A. (1999). Protein kinase C-beta activates tyrosinase by phosphorylating serine residues in its cytoplasmic domain. J. Biol. Chem. 274, 16470–16478. doi:10.1074/jbc.274.23.16470
Park, H. Y., Wu, C., Yonemoto, L., Murphy-Smith, M., Wu, H., Stachur, C. M., et al. (2006). MITF mediates cAMP-induced protein kinase C-beta expression in human melanocytes. Biochem. J. 395, 571–578. doi:10.1042/BJ20051388
Passeron, T., Valencia, J. C., Bertolotto, C., Hoashi, T., Le Pape, E., Takahashi, K., et al. (2007). SOX9 is a key player in ultraviolet B-induced melanocyte differentiation and pigmentation. Proc. Natl. Acad. Sci. U. S. A. 104, 13984–13989. doi:10.1073/pnas.0705117104
Patatian, A., Delestre-Delacour, C., Percoco, G., Ramdani, Y., Di Giovanni, M., Peno-Mazzarino, L., et al. (2021). Skin biological responses to urban pollution in an ex vivo model. Toxicol. Lett. 348, 85–96. doi:10.1016/j.toxlet.2021.05.003
Peng, F., Xue, C. H., Hwang, S. K., Li, W. H., Chen, Z., and Zhang, J. Z. (2017). Exposure to fine particulate matter associated with senile lentigo in Chinese women: A cross-sectional study. J. Eur. Acad. Dermatol. Venereol. 31, 355–360. doi:10.1111/jdv.13834
Pentland, A. P., and Mahoney, M. G. (1990). Keratinocyte prostaglandin synthesis is enhanced by IL-1. J. Invest. Dermatol. 94, 43–46. doi:10.1111/1523-1747.ep12873337
Pentland, A. P., Mahoney, M., Jacobs, S. C., and Holtzman, M. J. (1990). Enhanced prostaglandin synthesis after ultraviolet injury is mediated by endogenous histamine stimulation. A mechanism for irradiation erythema. J. Clin. Invest. 86, 566–574. doi:10.1172/JCI114746
Percoco, G., Patatian, A., Eudier, F., Grisel, M., Bader, T., Lati, E., et al. (2021). Impact of cigarette smoke on physical-chemical and molecular proprieties of human skin in an ex vivo model. Exp. Dermatol. 30, 1610–1618. doi:10.1111/exd.14192
Pillaiyar, T., Manickam, M., and Jung, S. H. (2017). Recent development of signaling pathways inhibitors of melanogenesis. Cell Signal 40, 99–115. doi:10.1016/j.cellsig.2017.09.004
Pitman, S. (2017). Euromonitor: The evolution of antipollution in europe and north America: Part I; cosmetics design USA.com. West Sussex, UK: William Reed Business Media SAS.
Pittayapruek, P., Meephansan, J., Prapapan, O., Komine, M., and Ohtsuki, M. (2016). Role of matrix metalloproteinases in photoaging and photocarcinogenesis. Int. J. Mol. Sci. 17, 868. doi:10.3390/ijms17060868
Portugal-Cohen, M., Oron, M., Cohen, D., and Ma'or, Z. (2017). Antipollution skin protection - a new paradigm and its demonstration on two active compounds. Clin. Cosmet. Investig. Dermatol. 10, 185–193. doi:10.2147/CCID.S129437
Prieux, R., Eeman, M., Rothen-Rutishauser, B., and Valacchi, G. (2020). Mimicking cigarette smoke exposure to assess cutaneous toxicity. Toxicol. Vitro 62, 104664. doi:10.1016/j.tiv.2019.104664
Procházková, J., Kabátková, M., Bryja, V., Umannová, L., Bernatík, O., Kozubík, A., et al. (2011). The interplay of the aryl hydrocarbon receptor and β-catenin alters both AhR-dependent transcription and Wnt/β-catenin signaling in liver progenitors. Toxicol. Sci. 122, 349–360. doi:10.1093/toxsci/kfr129
Randhawa, M., Huff, T., Valencia, J. C., Younossi, Z., Chandhoke, V., Hearing, V. J., et al. (2009). Evidence for the ectopic synthesis of melanin in human adipose tissue. FASEB J. 23, 835–843. doi:10.1096/fj.08-116327
Rasmussen, C., Gratz, K., Liebel, F., Southall, M., Garay, M., Bhattacharyya, S., et al. (2010). The StrataTest® human skin model, a consistent in vitro alternative for toxicological testing. Toxicol. Vitro. 24, 2021–2029. doi:10.1016/j.tiv.2010.07.027
Rothhammer, V., and Quintana, F. J. (2019). The aryl hydrocarbon receptor: an environmental sensor integrating immune responses in health and disease. Nat. Rev. Immunol. 19, 184–197. doi:10.1038/s41577-019-0125-8
Russell, W. M. S., and Burch, R. L. (1959). (as reprinted 1992). The principles of humane experimental technique. Wheathampstead (UK): Universities Federation for Animal Welfare.
Salminen, A. (2022). Aryl hydrocarbon receptor (AhR) reveals evidence of antagonistic pleiotropy in the regulation of the aging process. Cell. Mol. Life Sci. 79, 489. doi:10.1007/s00018-022-04520-x
Sasaki, M., Horikoshi, T., Uchiwa, H., and Miyachi, Y. (2000). Up-regulation of tyrosinase gene by nitric oxide in human melanocytes. Pigment. Cell Res. 13, 248–252. doi:10.1034/j.1600-0749.2000.130406.x
Schallreuter, K. U., Wazir, U., Kothari, S., Gibbons, N. C., Moore, J., and Wood, J. M. (2004). Human phenylalanine hydroxylase is activated by H2O2: A novel mechanism for increasing the L-tyrosine supply for melanogenesis in melanocytes. Biochem. Biophys. Res. Commun. 322, 88–92. doi:10.1016/j.bbrc.2004.07.082
Schallreuter, K. U., Kothari, S., Chavan, B., and Spencer, J. D. (2008). Regulation of melanogenesis--controversies and new concepts. Exp. Dermatol. 17, 395–404. doi:10.1111/j.1600-0625.2007.00675.x
Schallreuter, K. U., Wood, J. M., Lemke, R., LePoole, C., Das, P., Westerhof, W., et al. (1992). Production of catecholamines in the human epidermis. Biochem. Biophys. Res. Commun. 189, 72–78. doi:10.1016/0006-291x(92)91527-w
Schecter, A., Birnbaum, L., Ryan, J. J., and Constable, J. D. (2006). Dioxins: an overview. Environ. Res. 101, 419–428. doi:10.1016/j.envres.2005.12.003
Schmitz, J., Owyang, A., Oldham, E., Song, Y., Murphy, E., McClanahan, T. K., et al. (2005). IL-33, an interleukin-1-like cytokine that signals via the IL-1 receptor-related protein ST2 and induces T helper type 2-associated cytokines. Immunity 23, 479–490. doi:10.1016/j.immuni.2005.09.015
Scott, G., Jacobs, S., Leopardi, S., Anthony, F. A., Learn, D., Malaviya, R., et al. (2005). Effects of PGF2alpha on human melanocytes and regulation of the FP receptor by ultraviolet radiation. Exp. Cell. Res. 304, 407–416. doi:10.1016/j.yexcr.2004.11.016
Scott, G., Leopardi, S., Printup, S., Malhi, N., Seiberg, M., and Lapoint, R. (2004). Proteinase-activated receptor-2 stimulates prostaglandin production in keratinocytes: analysis of prostaglandin receptors on human melanocytes and effects of PGE2 and PGF2alpha on melanocyte dendricity. J. Invest. Dermatol. 122, 1214–1224. doi:10.1111/j.0022-202X.2004.22516.x
Serre, C., Busuttil, V., and Botto, J. M. (2018). Intrinsic and extrinsic regulation of human skin melanogenesis and pigmentation. Int. J. Cosmet. Sci. 40, 328–347. doi:10.1111/ics.12466
Serri, R., Romano, M. C., and Sparavigna, A. (2010). Quitting smoking rejuvenates the skin": results of a pilot project on smoking cessation conducted in milan, Italy. Skinmed 8, 23–29.
Seurat, E., Verdin, A., Cazier, F., Courcot, D., Fitoussi, R., Vié, K., et al. (2021). Influence of the environmental relative humidity on the inflammatory response of skin model after exposure to various environmental pollutants. Environ. Res. 196, 110350. doi:10.1016/j.envres.2020.110350
Shi, Y., Zeng, Z., Liu, J., Pi, Z., Zou, P., Deng, Q., et al. (2021). Particulate matter promotes hyperpigmentation via AhR/MAPK signaling activation and by increasing α-MSH paracrine levels in keratinocytes. Environ. Pollut. 278, 116850. doi:10.1016/j.envpol.2021.116850
Shin, H., Hong, S. D., Roh, E., Jung, S. H., Cho, W. J., Park, S. H., et al. (2015). cAMP-dependent activation of protein kinase A as a therapeutic target of skin hyperpigmentation by diphenylmethylene hydrazinecarbothioamide. Br. J. Pharmacol. 172, 3434–3445. doi:10.1111/bph.13134
Spencer, N. (2016). Cosmetics design-asia.com. West Sussex, UK: William Reed Business Media SAS.What does pollution mean for your skin
Stanisz, H., Stark, A., Kilch, T., Schwarz, E. C., Müller, C. S., Peinelt, C., et al. (2012). ORAI1 Ca(2+) channels control endothelin-1-induced mitogenesis and melanogenesis in primary human melanocytes. J. Invest. Dermatol. 132, 1443–1451. doi:10.1038/jid.2011.478
Starner, R. J., McClelland, L., Abdel-Malek, Z., Fricke, A., and Scott, G. (2010). PGE(2) is a UVR-inducible autocrine factor for human melanocytes that stimulates tyrosinase activation. Exp. Dermatol. 19, 682–684. doi:10.1111/j.1600-0625.2010.01074.x
Steingrímsson, E., Copeland, N. G., and Jenkins, N. A. (2004). Melanocytes and the microphthalmia transcription factor network. Annu. Rev. Genet. 38, 365–411. doi:10.1146/annurev.genet.38.072902.092717
Stout, P. R., and Ruth, J. A. (1999). Deposition of [3H]cocaine, [3H]nicotine, and [3H]flunitrazepam in mouse hair melanosomes after systemic administration. Drug Metab. Dispos. 27, 731–735.
Summerfield, A., Meurens, F., and Ricklin, M. E. (2015). The immunology of the porcine skin and its value as a model for human skin. Mol. Immunol. 66, 14–21. doi:10.1016/j.molimm.2014.10.023
Suo, D., Zeng, S., Zhang, J., Meng, L., and Weng, L. (2020). PM2.5 induces apoptosis, oxidative stress injury and melanin metabolic disorder in human melanocytes. Exp. Ther. Med. 19, 3227–3238. doi:10.3892/etm.2020.8590
Szparaga, M., Świercz, R., and Stępnik, M. (2021). Review of data on chemical content in an aerosol resulting from heating a tobacco or a solution used in e-cigarettes and in the smoke generated from the reference cigarettes. Toxicol. Mech. Methods. 31, 323–333. doi:10.1080/15376516.2021.1884922
Szüts, T., Olsson, S., Lindquist, N. G., Ullberg, S., Pilotti, A., and Enzell, C. (1978). Long-term fate of [14C]nicotine in the mouse: retention in the bronchi, melanin-containing tissues and urinary bladder wall. Toxicology 10, 207–220. doi:10.1016/0300-483x(78)90072-0
Takeda, K., Yasumoto, K., Takada, R., Takada, S., Watanabe, K., Udono, T., et al. (2000). Induction of melanocyte-specific microphthalmia-associated transcription factor by Wnt-3a. J. Biol. Chem. 275, 14013–14016. doi:10.1074/jbc.c000113200
Tamai, Y., Tsuji, M., Wada, K., Nakamura, K., Hayashi, M., Takeda, N., et al. (2014). Association of cigarette smoking with skin colour in Japanese women. Tob. Control 23, 253–256. doi:10.1136/tobaccocontrol-2012-050524
Taylor, S., Grimes, P., Lim, J., Im, S., and Lui, H. (2009). Postinflammatory hyperpigmentation. J. Cutan. Med. Surg. 13, 183–191. doi:10.2310/7750.2009.08077
Thiele, J. J., Traber, M. G., Tsang, K., Cross, C. E., and Packer, L. (1997). In vivo exposure to ozone depletes vitamins C and E and induces lipid peroxidation in epidermal layers of murine skin. Free Radic. Biol. Med. 23, 385–391. doi:10.1016/s0891-5849(96)00617-x
Thong, H. Y., Jee, S. H., Sun, C. C., and Boissy, R. E. (2003). The patterns of melanosome distribution in keratinocytes of human skin as one determining factor of skin colour. Br. J. Dermatol. 149, 498–505. doi:10.1046/j.1365-2133.2003.05473.x
Todo, H. (2017). Transdermal permeation of drugs in various animal species. Pharmaceutics 9, 33. doi:10.3390/pharmaceutics9030033
Toll, R., Jacobi, U., Richter, H., Lademann, J., Schaefer, H., and Blume-Peytavi, U. (2004). Penetration profile of microspheres in follicular targeting of terminal hair follicles. J. Invest. Dermatol. 123, 168–176. doi:10.1111/j.0022-202X.2004.22717.x
Toyofuku, K., Wada, I., Valencia, J. C., Kushimoto, T., Ferrans, V. J., and Hearing, V. J. (2001). Oculocutaneous albinism types 1 and 3 are ER retention diseases: mutation of tyrosinase or Tyrp1 can affect the processing of both mutant and wild-type proteins. FASEB J. 15, 2149–2161. doi:10.1096/fj.01-0216com
Tsuji, G., Takahara, M., Uchi, H., Takeuchi, S., Mitoma, C., Moroi, Y., et al. (2011). An environmental contaminant, benzo(a)pyrene, induces oxidative stress-mediated interleukin-8 production in human keratinocytes via the aryl hydrocarbon receptor signaling pathway. J. Dermatol. Sci. 62, 42–49. doi:10.1016/j.jdermsci.2010.10.017
Tsujimoto, A., Nakashima, T., Tanino, S., Dohi, T., and Kurogochi, Y. (1975). Tissue distribution of ['H]nicotine in dogs and rhesus monkeys. Toxicol. Appl. Pharmacol. 32, 21–31. doi:10.1016/0041-008x(75)90191-x
van den Bogaard, E. H., Bergboer, J. G., Vonk-Bergers, M., van Vlijmen-Willems, I. M., Hato, S. V., van der Valk, P. G., et al. (2013). Coal tar induces AHR-dependent skin barrier repair in atopic dermatitis. J. Clin. Invest. 123, 917–927. doi:10.1172/JCI65642
Van't Hof, A. E., Campagne, P., Rigden, D. J., Yung, C. J., Lingley, J., Quail, M. A., et al. (2016). The industrial melanism mutation in British peppered moths is a transposable element. Nature 534, 102–105. doi:10.1038/nature17951
Vierkötter, A., Schikowski, T., Ranft, U., Sugiri, D., Matsui, M., Krämer, U., et al. (2010). Airborne particle exposure and extrinsic skin aging. J. Invest. Dermatol. 130, 2719–2726. doi:10.1038/jid.2010.204
Villano, C. M., Murphy, K. A., Akintobi, A., and White, L. A. (2006). 2,3,7,8-tetrachlorodibenzo-p-dioxin (TCDD) induces matrix metalloproteinase (MMP) expression and invasion in A2058 melanoma cells. Toxicol. Appl. Pharmacol. 210, 212–224. doi:10.1016/j.taap.2005.05.001
Visconti, M. A., Castrucci, A. M., and Valente, D. (1984). A study on melanophore receptors of Papiliochromis ramirezi (Teleostei, Cichlidae). Comp. Biochem. Physiol. C. Comp. Pharmacol. Toxicol. 77, 161–165. doi:10.1016/0742-8413(84)90146-4
Vo, D. H., Hartig, R., Weinert, S., Haybaeck, J., and Nass, N. (2019). G-Protein-Coupled Estrogen Receptor (GPER)-specific agonist G1 induces ER stress leading to cell death in MCF-7 cells. Biomolecules 9, 503. doi:10.3390/biom9090503
Vogel, C. F., Sciullo, E., Li, W., Wong, P., Lazennec, G., and Matsumura, F. (2007). RelB, a new partner of aryl hydrocarbon receptor-mediated transcription. Mol. Endocrinol. 21, 2941–2955. doi:10.1210/me.2007-0211
Wallström, M., Sand, L., Nilsson, F., and Hirsch, J. M. (1999). The long-term effect of nicotine on the oral mucosa. Addiction 94, 417–423. doi:10.1046/j.1360-0443.1999.94341711.x
Whitehouse, L. (2016). Antipollution and the beauty industry: Part I. Cosmetics design-europ.com. West Sussex, UK: William Reed Business Media SAS.
Wilson, S. R., Joshi, A. D., and Elferink, C. J. (2013). The tumor suppressor Kruppel-like factor 6 is a novel aryl hydrocarbon receptor DNA binding partner. J. Pharmacol. Exp. Ther. 345, 419–429. doi:10.1124/jpet.113.203786
World Health Organization (WHO). (2021). WHO global air quality guidelines. Available at: https://apps.who.int/iris/bitstream/handle/10665/345329/9789240034228-eng.pdf on 18 June 2023
Wu, Q., Bai, P., Xia, Y., Lai, Q. W. S., Guo, M. S. S., Dai, K., et al. (2020). Solar light induces expression of acetylcholinesterase in skin keratinocytes: signalling mediated by activator protein 1 transcription factor. Neurochem. Int. 141, 104861. doi:10.1016/j.neuint.2020.104861
Wu, Q., Xia, Y., Dai, K., Bai, P., Kwan, K. K. L., Guo, M. S. S., et al. (2020a). Solar light induces the release of acetylcholine from skin keratinocytes affecting melanogenesis. FASEB J. 34, 8941–8958. doi:10.1096/fj.202000708R
Xu, G., Li, Y., Yoshimoto, K., Wu, Q., Chen, G., Iwata, T., et al. (2014). 2,3,7,8-Tetrachlorodibenzo-p-dioxin stimulates proliferation of HAPI microglia by affecting the Akt/GSK-3β/cyclin D1 signaling pathway. Toxicol. Lett. 224, 362–370. doi:10.1016/j.toxlet.2013.11.003
Yamada, T., Hasegawa, S., Iwata, Y., Arima, M., Kobayashi, T., Numata, S., et al. (2019). UV irradiation-induced DNA hypomethylation around WNT1 gene: implications for solar lentigines. Exp. Dermatol. 28, 723–729. doi:10.1111/exd.13949
Yamaguchi, Y., and Hearing, V. J. (2009). Physiological factors that regulate skin pigmentation. Biofactors 35, 193–199. doi:10.1002/biof.29
Yang, A. Y., Tang, M. L., Hamilton, M., Oddos, T., Martin, K., Southall, M., et al. (2020). 18274 Particulate matter <2.5 μm as a model of pollution induces hyperpigmentation in melanocytes potentially via oxidative stress. J. Am. Acad. Dermatol. 83, AB208. doi:10.1016/j.jaad.2020.06.921
Yang, X., Peng, F., Huang, J., Chen, Z., and Zhang, J. (2022). Particulate matter 2.5 induced hyperpigmentation in reconstructed human epidermis model (MelaKutis®). Chin. Med. J. Engl. 135, 502–504. doi:10.1097/CM9.0000000000001934
Yarden, Y., Kuang, W. J., Yang-Feng, T., Coussens, L., Munemitsu, S., Dull, T. J., et al. (1987). Human proto-oncogene c-kit: A new cell surface receptor tyrosine kinase for an unidentified ligand. EMBO J. 6, 3341–3351. doi:10.1002/j.1460-2075.1987.tb02655.x
Yazdanparast, T., Hassanzadeh, H., Nasrollahi, S. A., Seyedmehdi, S. M., Jamaati, H., Naimian, A., et al. (2019). Cigarettes smoking and skin: A comparison study of the biophysical properties of skin in smokers and non-smokers. Tanaffos 18, 163–168.
Ye, M., Zhang, Y., Gao, H., Xu, Y., Jing, P., Wu, J., et al. (2018). Activation of the Aryl Hydrocarbon Receptor leads to resistance to EGFR TKIs in non-small cell lung cancer by activating Src-mediated bypass signaling. Clin. Cancer Res. 24, 1227–1239. doi:10.1158/1078-0432.CCR-17-0396
Yerger, V. B., and Malone, R. E. (2006). Melanin and nicotine: A review of the literature. Nicotine Tob. Res. 8, 487–498. Erratum in: Nicotine Tob. Res. 2012 Jun;14(6):766. doi:10.1080/14622200600790039
Yin, L., Morita, A., and Tsuji, T. (2003). Tobacco smoke extract induces age-related changes due to modulation of TGF-beta. Exp. Dermatol. Suppl. 2, 51–56. doi:10.1034/j.1600-0625.12.s2.8.x
Zhou, J., Ling, J., Wang, Y., Shang, J., and Ping, F. (2016). Cross-talk between interferon-gamma and interleukin-18 in melanogenesis. J. Photochem. Photobiol. B 163, 133–143. doi:10.1016/j.jphotobiol.2016.08.024
Zhou, J., Shang, J., Song, J., and Ping, F. (2013). Interleukin-18 augments growth ability of primary human melanocytes by PTEN inactivation through the AKT/NF-κB pathway. Int. J. Biochem. Cell Biol. 45, 308–316. doi:10.1016/j.biocel.2012.11.008
Zhou, J., Song, J., Ping, F., and Shang, J. (2014). Enhancement of the p38 MAPK and PKA signaling pathways is associated with the pro-melanogenic activity of Interleukin 33 in primary melanocytes. J. Dermatol. Sci. 73, 110–116. doi:10.1016/j.jdermsci.2013.09.005
Zoio, P., Ventura, S., Leite, M., and Oliva, A. (2021). Pigmented full-thickness human skin model based on a fibroblast-derived matrix for long-term studies. Tissue Eng. Part. C. Methods. 27, 433–443. doi:10.1089/ten.TEC.2021.0069
Keywords: air pollution, tobacco smoke, skin pigmentation, New Approach Methodologies, skin aging
Citation: Bouchard KV and Costin G-E (2023) Promoting New Approach Methodologies (NAMs) for research on skin color changes in response to environmental stress factors: tobacco and air pollution. Front. Toxicol. 5:1256399. doi: 10.3389/ftox.2023.1256399
Received: 10 July 2023; Accepted: 25 August 2023;
Published: 10 October 2023.
Edited by:
Christophe Rousselle, Agence Nationale de Sécurité Sanitaire de l’Alimentation, de l’Environnement et du Travail (ANSES), FranceReviewed by:
Shamshad Alam, University at Buffalo, United StatesMaria Pilar Vinardell, University of Barcelona, Spain
Copyright © 2023 Bouchard and Costin. This is an open-access article distributed under the terms of the Creative Commons Attribution License (CC BY). The use, distribution or reproduction in other forums is permitted, provided the original author(s) and the copyright owner(s) are credited and that the original publication in this journal is cited, in accordance with accepted academic practice. No use, distribution or reproduction is permitted which does not comply with these terms.
*Correspondence: Gertrude-Emilia Costin, ZWNvc3RpbkBpaXZzLm9yZw==
†These authors have contributed equally to this work