- 1Water Research Group, School of Biological Sciences, North-West University, Potchefstroom, South Africa
- 2Department of Zoology, University of Johannesburg, Johannesburg, South Africa
Introduction: The bioaccumulation and differential effects of cadmium tellurium quantum dot (CdTe QDs) nanomaterials with different functional groups are poorly understood in aquatic organisms. This study aimed to investigate the metal uptake, developmental effects, and respiratory effects of CdTe QDs with different functional groups (COOH, NH3, and PEG) on zebrafish embryos.
Methods: Zebrafish embryos were exposed to carboxylate (COOH), ammonia (NH3), and polyethylene glycol (PEG) functionalized CdTe QDs at nominal concentrations of 0.5, 2, 4, 6, and 20 mg QDs/L. The materials were characterized in E3 exposure media and the metal uptake, developmental effects, and respiratory effects of zebrafish embryos were recorded.
Results: The total Cd or Te concentrations in the larvae could not be explained by the metal concentrations or dissolution of the materials in the exposure media. The metal uptake in the larvae was not dose-dependent, except for the QD-PEG treatment. The QD-NH3 treatment caused respiration inhibition at the highest exposure concentration and hatching delays and severe malformations at low concentrations. The toxicities observed at low concentrations were attributed to particles crossing the pores in the chorion, and toxicities at higher concentrations were linked to the aggregation of particle agglomerates to the surface of the chorion impairing respiration. Developmental defects were recorded following exposure to all three functional groups, but the QD-NH3 group had the most severe response. The LC50 values for embryo development of QD-COOH and QD-PEG groups were higher than 20 mg/L, and the LC50 of the QD-NH3 group was 20 mg/L.
Discussion: The results of this study suggest that CdTe QDs with different functional groups have differential effects on zebrafish embryos. The QD-NH3 treatment caused the most severe effects, including respiration inhibition and developmental defects. These findings provide valuable information for understanding the effects of CdTe QDs on aquatic organisms and highlight the need for further investigation.
1 Introduction
Quantum dots (QDs) are fluorescent nanocrystals with a semiconductor metal core, which is usually comprised of CdSe or CdTe (King-Heiden et al., 2009; Zheng et al., 2021). Due to their small size and unique composition, they absorb and emit light at different wavelengths making them ideal as contrasting agents for bio-imaging applications (Michalet et al., 2005). Besides cellular imaging, QDs can be used for a wide range of other applications, such as fixed tissue analysis, spectral encoding of microparticles, and quantitative analysis of ions (Åkerman et al., 2002; Jason et al., 2009). Even though QDs have many advantageous properties they are essentially inorganic semiconductor materials that can be toxic to living organisms (Jason et al., 2009). Many studies report no or little toxicity to living organisms (Jaiswal et al., 2003; Larson et al., 2003; Chen and Gerion, 2004; Voura et al., 2004; Zhang et al., 2013), however, some studies have seen cytotoxicity and other toxic outcomes from exposure to QDs leading to the development of surface coatings and functionalization to minimize toxicity (Derfus et al., 2004; Lovrić et al., 2005; Jason et al., 2009; Zhang et al., 2012b; Hsu et al., 2012). Surface functionalization with organic molecules increases their dispensability in water, making them more biologically compatible and prevents metal core leaching (Medintz et al., 2005; Michalet et al., 2005; Ron, 2006; King-Heiden et al., 2009).
Given their wide range of applications, the substantial growth in production volumes of QDs is predicted and thus entry of QDs and their by-products into waterways is likely (Singh et al., 2020). Even though the exact concentration of QDs in the environment is unknown, this potentially poses risks to aquatic biota. The coatings that make them unique and useful could also be their weakness. As they end up in the environment the coating could become compromised and reveal a possibly toxic core or cause the dissolution of metals from the core (Ron, 2006; Rocha et al., 2017). As QDs degrade they could also react in undesirable ways in vivo. It is said that the estimated environmental residence times for QDs range from months to years (Ron, 2006). From this, it is evident that their physicochemical properties and surface functionalization are fundamental in understanding their potential toxicity.
In the aquatic environment processes such as agglomeration patterns, settling, oxidation, interaction with organic material and biotransformation can influence the fate, behaviour and uptake of any nanomaterial (Rocha et al., 2017; Mishra et al., 2021). These aspects are determined by the physicochemical properties of the environment, particle size, shape, coating, and functionalization. Studies have found that particle size and functionalization determine the interaction with biological membranes (such as cell membranes as well as the chorion of an embryo) and biodistribution in the organism (Paatero et al., 2017). Not many studies have looked at the effect of QDs with the same core size and composition but different functionalization on their toxicity, especially in zebrafish (Rocha et al., 2017; Gallud et al., 2020). The zebrafish (Danio rerio) is an established bioindicator to study nanoparticle toxicity (Zhu et al., 2007; Martinez et al., 2019; Mishra et al., 2021). The specie is small in size, has good reproducibility and has a fast embryonic development. The embryo is transparent and thus the development of organs can be visualized during development (Wang et al., 2012; Paatero et al., 2017). Different parameters can be assessed, such as hatching, organ development, respiration, behaviour, immunotoxicity, and genotoxicity, in addition to reproductive toxicity and mortality.
Accordingly, the present study aimed to determine the embryotoxicity induced by three CdTe QDs functionalized with carboxylate (COOH), ammonia (NH3), and polyethylene glycol (PEG) using zebrafish embryo test (ZET) as a model. The authors conducted a sequence of assessments for embryonic developmental toxicity including embryonic mortality, hatching rate, malformation percentage, respiration rates and Cd/Te uptake from QDs exposure. Combining embryonic toxicity, metal body burden and respiration rates as indicators of evaluating QDs toxicity will give a clearer indication of the toxicity (nano-specific or metal-based) which is important for safety by design for biomedical application.
2 Materials and methods
2.1 Chemicals
Quantum dot nanopowders with 3–5 nm primary particle size were produced by PlasmaChem GmbH (Lot# YF140402) and supplied by the Nanosolutions EU FP7 project (Project ID: 309329). The stock suspensions (100 mg/L) were freshly prepared by directly dispersing the three types of QDs (5 mg) in 50 mL of ultrapure water individually (18.2 MΩ/cm, Millipore, Billerica, MA). The 50 mL nano stock solutions were sonicated at 60°C in a bath ultra-sonicator (Model E-UC6-HD-D, Scientech, India) for 1 h at 20 Hz prior to dilution in E3 media for exposure and characterization.
2.2 Preparation and characterization of quantum dots
Characterization was done in two batches. Firstly, the 100 mg/L NM stocks in ultrapure were characterised by the University of Plymouth as part of the Nanosolutions project. Secondly, QDs in E3 media at concentrations used for this study was characterized at the North-West University.
Characterization of 100 mg/L stock CdTe QDs data were obtained from the Nanosolutions project data sheets since they were the same batches that were used in this study (Nanosolutions., 2013; Vassallo et al., 2018; Tatsi et al., 2020). Briefly the hydrodynamic size distribution of stock solutions was determined by Nanoparticle Tracking Analysis (NTA) using Nanosight LM 10 (Nanosight, Salisbury, United Kingdom, laser output set at 30 mW at 640 nm) and methods described by Besinis et al. (2014). Material dissolution was measured using dialysis bags containing 700 μL of the appropriate stock solution. Briefly, 8 mL of a sonicated stock of each material was added to a dialysis tube (product code: D9777, cellulose membrane with molecular weight cut off at 12,000 Da, Sigma-Aldrich Ltd., Dorset, United Kingdom) which was then secured on both ends using cable ties to prevent leakage. Each bag was then placed in a beaker containing 492 mL of ultrapure water. Samples (15 mL) of the external water from each beaker was taken after 24 h. Each material was analysed in duplicate on a shaker (IKA Labortechnik, KS250 basic). Samples of 15 mL where acidified with 300 μL of 65% nitric acid. Metal concentrations were measured by inductively coupled plasma optical emission spectrophotometry (ICP-OES, ICAP 7400) and inductively coupled plasma optical mass spectrophotometry (ICP-MS, Thermoscientific, X Series 2).
The primary particle diameter of initial nanomaterial particles was determined using transmission electron microscopy (TEM) (FEI Tecnai G2). One drop of 100 mg/L solution of each individual nanomaterial was added to a carbon grid separately, allowed to settle and dried, thereafter it was examined at high resolution (200 kV).
For exposures, standard E3 media was used. The media was constituted by dissolving 34.8 g NaCl, 1.6 g KCl, 5.8 g CaCl2.2H2O, and 9.78 g MgCl2.6H2O in 2 L reverse osmosis water. The pH of approximately 7.6 remained stable at 7.65. To make up the desired exposure concentrations the relevant volumes of sonicated nanomaterial stock solutions (100 mg/L) were added to the exposure media. Six different concentrations were selected for each nanomaterial with 5 replicates of each. Nominal exposure concentrations were 0, 0.5, 2, 4, 6, and 20 mg/L QDs.
Further characterization of exposure solutions in E3 media was conducted at the North-West University. Hydrodynamic size distribution in E3 media was measured using Dynamic Light Scattering (DLS) and stability were examined with a zeta-sizer (Malvern, Zetasizer Nano-ZS). The total metal content of solutions was determined by acidifying solutions (10 μL 65% HNO3 per 10 mL) and measuring Cd and Te concentrations using standard ICP-MS techniques.
2.3 Zebrafish maintenance and embryo exposure
Long-fin wild-type adult D. rerio breeding stocks were kept in a ZebTEC recirculating aquarium system in an environmentally controlled room (28°C). Adult D. rerio breeding stocks were bred in Tecniplast iSpawn spawning chambers (Tecniplast, Italy) in a ratio of two males to three females. After approximately 2 h post fertilization (hpf) eggs were collected and placed in an incubator at 27°C for another 2 h (Martinez et al., 2019; Kumar et al., 2020). The D. rerio embryo acute toxicity test (OECD 236—OECD, 2013) was carried out using 4 hpf embryos. Viable fertilized eggs were sorted using a stereo microscope and separated from unhealthy eggs. Healthy fertilized eggs were then treated with QD-NH3, QD-PEG, and QD-COOH (0.5, 2, 4, 6, and 20 mg/L) respectively. Test concentrations of each material were prepared by adding the required amount of the stock solution to each well in a 6-well plate and filling it to 5 mL with E3 media. Negative controls consisted of E3 media with no material added. Five viable eggs were then transferred to each well, thus for each treatment consisted of four replicates of five eggs (n = 20). The six-well plates were sealed with self-adhesive, oxygen-permeable sealing film (BRAND®, Sigma Aldrich, Merck, Darmstadt, Germany) to prevent evaporation from taking place. Unless otherwise stated, data for the QDs are shown as a nominal mass concentration of QD per unit volume and not normalized to the concentration of metal in the particle. This is because the dissolution rates of QDs were very low and therefore the particles were the predominant form in which the metal occurred in the media throughout the exposures. The plates were then incubated for 96 h at 27°C at a 12:12 dark-light cycle. Mortality, hatching rate and malformations were noted every 24 h. Observations performed on each tested embryo included: Coagulation of embryos, lack of somite formation, non-detachment of the tail, spinal deformation, pericardial edema and lack of heartbeat.
2.4 Embryo respiration
Respiration was measured at 24 h and 48 h by removing embryos from exposure media, washing them twice with clean E3 media and placing 5 embryos per replicate in each well of a 12-well Loligo® microplate system containing 80 µL of aerated E3 media. Three replicates were assessed at each concentration (n = 3). Measurements were taken every 5 s for at least 30 min, where after embryos were carefully removed from the wells and returned to the exposure media and incubated to continue with the test. Data are expressed as mg oxygen per embryo per minute.
2.5 Metal uptake in larvae
A parallel exposure was carried out concomitantly in E3 media to obtain enough biomass for metal analysis. Fertilised eggs were exposed in groups of 20 in 30 mL of exposure media. After 96 h, larvae were collected and larvae that were not hatched yet were manually dechorionated using a pair of forceps to eliminate possible QDs adhering to the chorion surface. Larvae were humanely killed in ice water, washed twice in ultrapure water and 10 individuals were pooled to represent a replicate sample. A minimum of three replicates per treatment was immediately frozen at −20°C until analyses.
Thawed samples were weighed and digested for 24 h in a Teflon heatblock with 1 mL nitric acid (65%, suprapure, Merck) at 65°C. Metal concentrations were measured using standard ICP-MS. Untreated organisms were used as negative controls and the natural background concentrations were subtracted from the concentrations measured in the exposed organisms. Concentrations are expressed as the total metal concentration on a wet weight basis. Analytical quality control was assured using certified reference materials (DORM-4 fish tissue) and is presented with instrumental detection limits in Table 1.

TABLE 1. Recovery rates (%) and detection limits of cadmium and tellurium from larval tissue and E3 media samples.
2.6 Statistical analysis
The dose-response function, namely, effect concentration where 50% of the population died (LC50 values) was calculated using non-linear regression analysis in ToxRat® software. Effect data are expressed as mean ± standard error. Significance between controls and treatments and functionalised groups were determined by using One-way analysis of variance (ANOVA) and Tukey’s post hoc test. All data were tested for normality using the Shapiro-Wilk test. Data that did not meet the normality assumption were log-transformed prior to analyses. Differences were considered significant at p < 0.05.
3 Results
3.1 Characterization
The primary particle size of all three QDs was 3–5 nm as described by the manufacturers. Nanoparticle tracking analysis performed at the University of Plymouth (as part of the Nanosolutions project) on 100 mg/L stock solutions indicated that QD-PEG had the largest hydrodynamic size distribution in ultra-pure water (Table 3).
Dynamic light scattering analysis of exposure concentration in E3 media conducted at the North-West University indicated that QD-COOH produced the largest hydrodynamic size distribution in E3 media and a decline in hydrodynamic size distribution as concentrations increased could be seen in all three materials. There was also a time-dependent agglomeration of particles (Table 2). Large agglomerates were observed settling out in exposure vessels at the 20 mg/L concentration. Hydrodynamic particle size was smaller in ultrapure water compared to concentrations made in E3 media (Tables 2, 3). The zeta potential of QDs were measured in E3 media to provide quantitative information on the stability of QDs in this study. Overall no significant effects on zeta potential were observed. Dissolution was also measured as part of the Nanosolutions project, and it was established that all three groups had less than 4% metal dissolution in ultra-pure water, only the QD-NH3 group had a larger dissolution of Te at 23.69% (Table 3). Based on the total metal content of each concentration of QDs the Cd concentration was between 3.8 and 5.5-fold higher than the Te concentration in QD-COOH and QD-PEG but between 28 and 55-fold higher in QD-NH3. The QD-COOH group has the highest overall metal content and the QD-NH3 group the lowest. There was also an increase in total metal content as exposure concentrations increased.
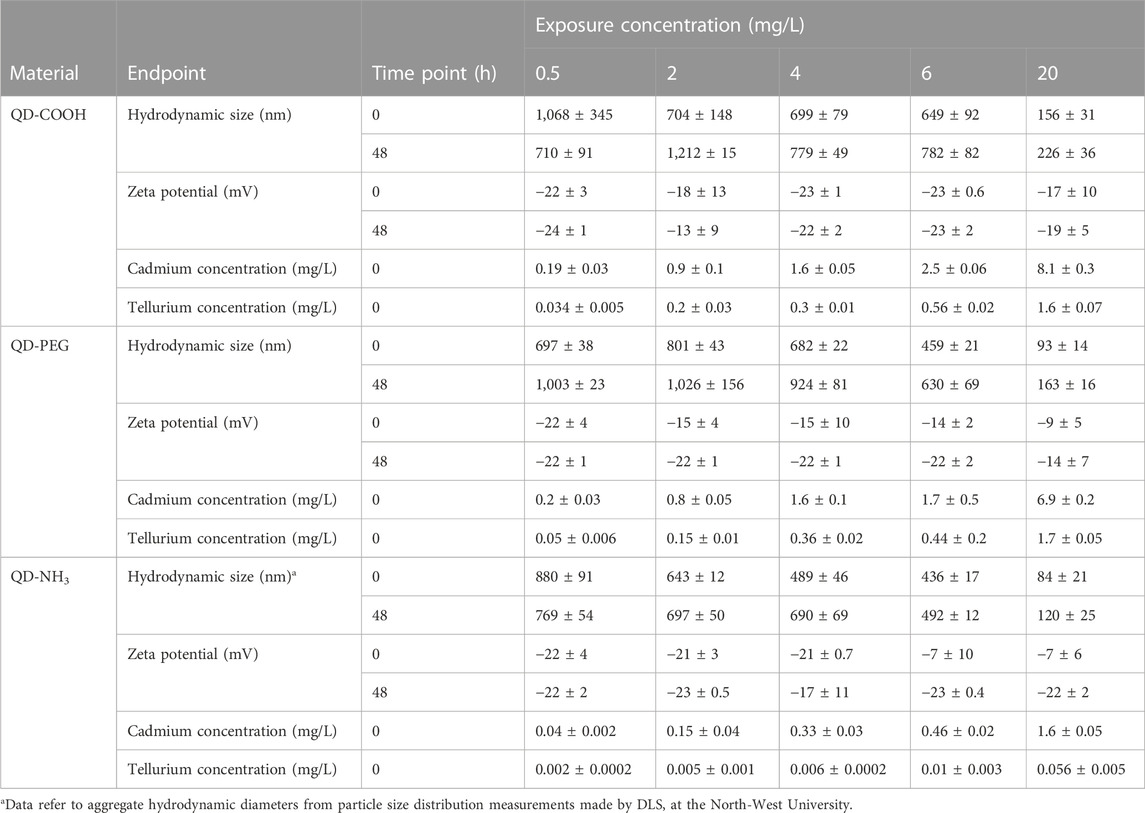
TABLE 2. Hydrodynamic size (nm) distribution, zeta potential (mV) and metal concentrations (mg/L) of CdTe QDs in E3 media (±SD) measured at the North-West University.

TABLE 3. Hydrodynamic size (nm) distribution and metal dissolution (mg/L) of 100 mg/L CdTe QDs stock solutions after 24 h in ultrapure water as analysed by Plymouth University for the Nanosolutions project (±SD).
3.2 Metal uptake
Particle agglomerates adhered to the surface of the embryo chorion in only the QD-NH3 group (Figure 1). Thus, for metal uptake, only hatched larvae after 96 h of exposure were used to avoid any metal from the chorion surface being included in uptake results.
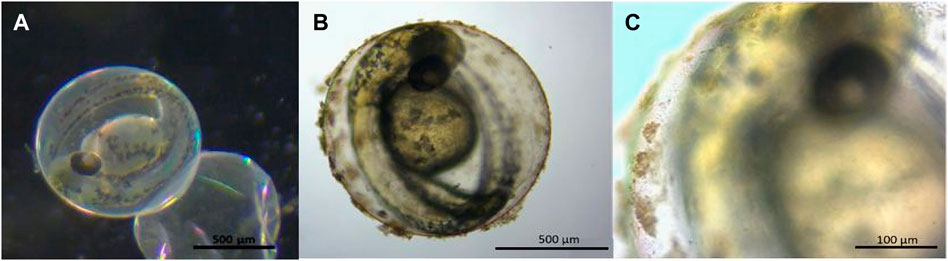
FIGURE 1. Stereo microscope images of 48 hpf Danio rerio embryos indicating no particle agglomerates on the chorion of the control (A) and QD agglomerates covering the outside of the chorion (B, C) during exposure to QD-NH3 20 mg/L.
Body burden of Cd after 96 h of exposure showed no significant uptake of Cd from the QD-COOH group and the QD-PEG group showed a concentration dependant increase in Cd uptake with the only significant increase compared to control was seen at the highest exposure concentration (20 mg/L) (Figure 2). The QD-NH3 group showed no dose-dependent increase in Cd uptake. The only two exposure concentrations that showed significant uptake was 2 mg/L and 20 mg/L.
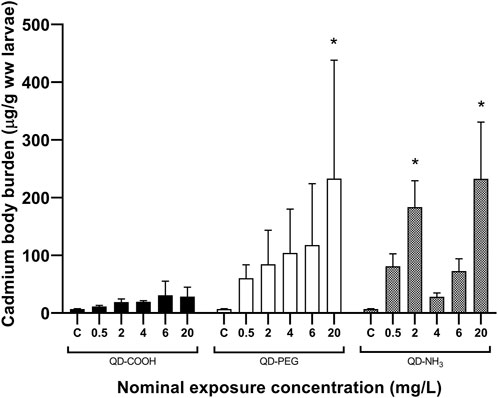
FIGURE 2. Total Cd concentrations (mean ± standard error expressed as µg/g ww larvae) in Danio rerio larvae after exposure to increasing concentrations of QD-COOH, QD-PEG and QD-NH3. Asterisk (*) indicates significance from control (p < 0.05, T-tests).
Similar uptake patterns were seen for Te but at lower concentrations (Figure 3). A significant uptake of 70 μg/g was found in the 20 mg/L exposure concentration of the QD-COOH group and the QD-NH3 group caused uptake of 94 μg/g and 60 μg/g in the 2 and 20 mg/L exposure concentrations.
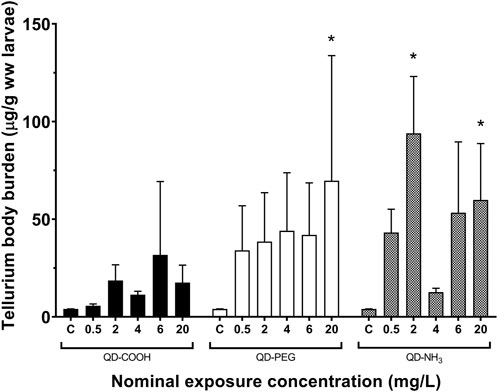
FIGURE 3. Total Te concentrations (mean ± standard error expressed as µg/g ww larvae) in Danio rerio larvae after exposure to increasing concentrations of QD-COOH, QD-PEG and QD-NH3. Single asterisk (*) indicates significance from control (p < 0.05, T-tests).
3.3 Respiration
After 24 h there was no significant effect on the respiration of embryos (Figure 4). However, after 48 h the highest concentration of the QD-NH3 group caused a significant decrease in respiration compared to the control. The highest QD-COOH exposure group concentration (20 mg/L) resulted in a significant increase in respiration compared to the control.
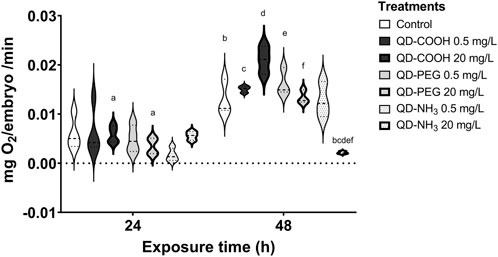
FIGURE 4. Respiration of Danio rerio embryos 24 hpf and 48 hpf. The graph represents the replicates and mean of each treatment. The same letters in superscript denotes a significant difference between treatments (p < 0.05, T-tests).
3.4 Developmental toxicity
Exposure to QD-COOH and QD-PEG groups did not produce any significant decrease in embryo survival (Figure 5). It was therefore not possible to calculate LC50 values for these groups, however the LOEC was calculated as ≥ 20 mg/L. Exposure to the QD-NH3 group caused significant mortality (i.e., > 50%) at the low concentrations of 0.5, 2, and 4 mg/L, while no mortality was observed at the higher exposure concentrations.
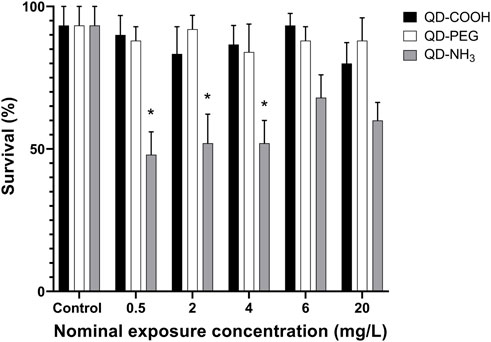
FIGURE 5. Survival of Danio rerio larvae after 96 h of exposure to QD-COOH, QD-PEG and QD-NH3. Error bars represent standard deviation. Single asterisk (*) indicates differences from control (p < 0.05).
Two low concentrations (2 and 4 mg/L) of the QD-NH3 group caused a significant (p < 0.05) delay in the hatching of surviving embryos (Figure 6). No significant effect on hatching success during the exposure period was observed at the other exposure concentrations.
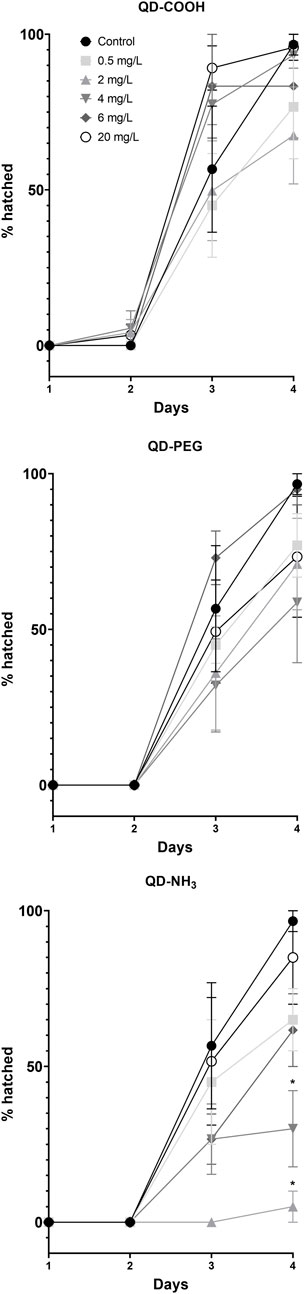
FIGURE 6. Percentage hatched Danio rerio larvae at different time points during the exposure to QD-COOH, QD-PEG and QD-NH3. Error bars represent standard deviation. Single asterisk (*) differences from control (p < 0.05, T-tests).
Exposure to all concentrations, except for the highest exposure (20 mg/L), of QD-PEG and QD-NH3 resulted in significant increases in pericardial edemas (Figure 7A). All concentrations of the QD-COOH group also resulted in significant pericardial edemas (Figure 7A). No significant occurrence of spinal deformations was observed in embryos exposed to QD-COOH (Figure 7B). The QD-PEG group showed significant spinal deformations compared to the control at all exposure concentrations except he 4 mg/L exposure. The QD-NH3 group also showed a significant occurrence of spinal deformations at all concentrations, with the lowest concentration (0.5 mg/L) having the highest effect.
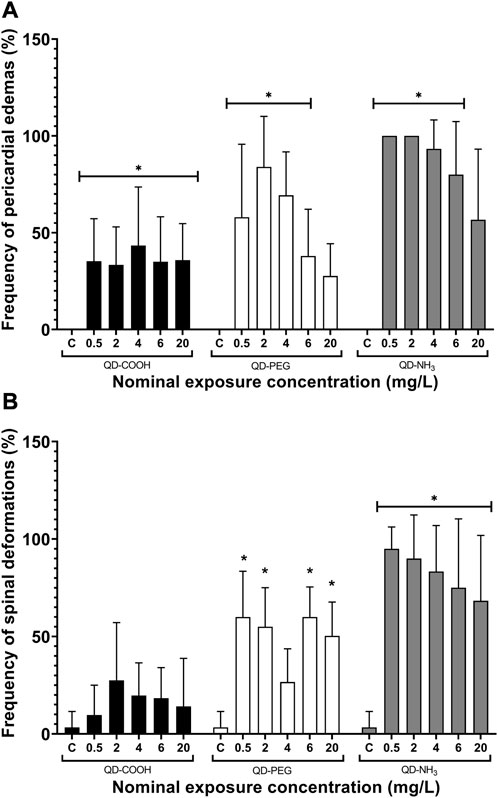
FIGURE 7. Spinal deformations (A) and pericardial edemas (B) of Danio rerio embryos exposed to QD-COOH, QD-PEG, and QD-NH3 expressed as a percentage of surviving embryos. Error bars represent standard deviation. Single asterisk (*) denotes significant differences from control (p < 0.05, T-tests).
4 Discussion
Nanomaterials have many beneficial properties and as their daily use is increasing it is inevitable that this will lead to the exposure of humans and other organisms to these materials (Ghobadian et al., 2015). The size and functionalization of these materials influence their toxicity and thus it is important to investigate and compare the toxicity of different functionalized QDs with the same primary particle size. In this study, we showed that there are clear differences of particle behaviour in exposure media influencing toxicity, metal uptake and respiration effects to the zebrafish embryo’s during exposure. Overall, the QD-NH3 group resulted in the most significant negative effects to the developing embryos causing severe malformations, hatching delay and mortality at low concentrations, and respiration inhibition at the highest concentration (20 mg/L). This contrasts with findings by Zheng et al. (2021) that showed exposure to CdSe/ZnS QDs with COOH functionalization was more toxic to developing zebrafish than NH3 functionalized material. The QD-PEG group caused significant edema and spinal deformations in a bimodal pattern, caused no respiration effects and no significant mortality, while the QD-COOH group showed the least toxic effects with only significant cardiac edema and increased respiration rate the lowest exposure concentration.
4.1 Dissolution and colloidal activity of particles
The colloidal activity of these particles can be described by the DLVO theory. This suggests that both van der Waals forces and electrostatic forces cause aggregation of particles in a suspension (Adair et al., 2001). At higher concentrations van der Waals forces between the large number of particles cause higher aggregation of nanoparticles. At a certain point the aggregates and agglomerates become too large to remain in suspension causing them to settle out. This makes them less bioavailable and influences toxicity. In the present study it was seen that QD-COOH caused the largest aggregates in the exposure media. This can be attributed to Mg and Ca ions in the media that reduce the electrostatic double layer initiating binding with the negatively charged COOH chain on the nanomaterial (Deerfield et al., 1989). However, these interactions are dynamic and size, temperature, time and concentration dependent. Thus, bioavailability and toxicity and change over time and concentration, leading to the bimodal and effects at lower concentrations seen in the present study.
Dissolution is a fundamental parameter to be considered when nanomaterial toxicity is assessed, as it can lead to highly toxic ions interacting with target sites (Zolotarev et al., 2012). The QDs used in this study have a very low dissolution potential (<4%) in ultra-pure water and decreases in solutions with higher pH such as E3 media that was used in this study (Boyle et al., 2020). Thus, the effects of dissolution metal ions from these materials were not considered to be significant. The highest dissolution of Te was from the QD-NH3 group (i.e., 23%) meaning that there would only be 13 μg/L Te ions in the highest exposure concentration of 20 mg/L. This is less than 10% of the NOEC derived for Te in algae and freshwater fish (ECHA, 2022). Total body metal concentrations for both Cd and Te increased in a dose-dependent manner only in the QD-PEG group. There were however no clear dose-response relationships between the body burdens and the effects observed, suggesting mechanisms other than dissolved metal content and total internal exposure (as reflected in the body burdens).
However, it may be possible that despite the insignificant dissolution of metals in exposure media, in vivo QD degradation may expose embryos to Cd and Te (Gao et al., 2019). This would be expected to produce endpoints of Cd toxicity in zebrafish embryos exposed to sublethal QD concentrations. King-Heiden et al. (2009) found that CdSe QDs with different chain lengths did degrade internally into Cd2+ ions. The only toxicity parameter that can be linked to total metal uptake in our study was hatching success. A study by Zolotarev et al. (2012) also found hatching success impacted by Cd2+ ions dissociating from Cdse/Cds/Zns/S,S-Dihydrolipoic acid/Polyacrylic acid QDs, but also found edemas and spinal deformations associated with Cd2+ ions. Boyle et al. (2020) and Vicario-Parés et al. (2014) suggest that metal ions from nanomaterials bind to the hatching enzyme sites resulting in delayed or non-hatching of embryos. Delayed hatching in the present study (in the case of QD-NH3 exposures) also lead to decreased interaction of the larvae with the media thereby decreased uptake of nanomaterials due to the prolonged protection of the chorion; this was seen for the 4 mg/L concentration but not for the 2 mg/L concentration in the present study.
4.2 Embryo developmental toxicity
Hatching success has been linked to increased occurrence of developmental defects following exposure to metal nanomaterials (King-Heiden et al., 2009; Asharani et al., 2011; Zhao et al., 2013; Ghobadian et al., 2015; Zheng et al., 2021). In this study malformations were more prevalent in the QD-NH3 group at the lower exposure concentrations. This contrasts with studies by Duan et al. (2013), that found a very strong dose-response relationship between exposure concentrations and developmental toxicity. These contrasting findings are probably related to the difference in materials with Duan et al. (2013) using water-soluble CdTe QDs as opposed to the three functionalised groups used in this study. Pericardial edemas were the most common malformation observed, consistent with previous results (Zhang et al., 2012a; Xu et al., 2012; Duan et al., 2013; Jin et al., 2017). Cellular studies have also shown that NH3 functionalized graphene oxide nanoparticles were more cytotoxic than pristine particles and mammalian studies exploring PEG functionalized gold nanoparticles found that these PEGylated particles were toxic to rats when injected intravenously (Cho et al., 2009; Keremidarska-Markova et al., 2018). Tang et al. (2013) reported that QDs enter cells through endocytosis and cause the formation of reactive oxygen species (ROS), which result in ROS-mediated genotoxicity leading to malformations. Studies have also concluded that QDs and Cd ions cause pericardial edemas and in turn, impaired cardiac function via bradycardia (Zhu et al., 2009; Duan et al., 2013; Krzykwa et al., 2019; Zheng et al., 2021).
Since the cardiac function influences the respiration rate, we established whether exposure to the three different functionalised QDs influenced the oxygen consumption of zebrafish embryos. Data showed that the embryo oxygen consumption displayed a different trend from the other effects recorded. The QD-NH3 group had the greatest effects but only at the highest exposure concentration (20 mg/L). Large agglomerates formed and adhered to the chorion of the embryos. This was also reported in other studies (Sun et al., 2013; Petushkova et al., 2015; Zheng et al., 2021). The agglomerations were the result of increased ZP at the highest exposure concentration and settling out of suspension. Lower dispersions ZP values will lead to aggregation, coagulation, or flocculation due to van der Waals interparticle attraction (Joseph and Singhvi, 2019; Shnoudeh et al., 2019), as is the case in the present study where all ZP values were in the non-stable range.
The chorion consists of two membranes, namely, the chorion membrane and the vitelline, it also has pores running through it to assist in gas exchange, as gills only develop after 2 weeks (Pelster and Bagatto, 2010). The pore canals are between 0.5 and 0.7 µm in diameter, this means that small particles can permeate these canals and enter the larvae. However, larger agglomerates can also block these canals causing hypoxic conditions for the embryo (Lee et al., 2007; Vicario-Parés et al., 2014; Samaee et al., 2015; Lacave et al., 2016). Even though embryos were washed in clean media before respiration measurements were taken there still could have been residual agglomerates blocking pore canals and impairing respiration in the 20 mg/L QD-NH3 treatment embryos. Increased oxygen consumption is most likely due to stress, increased activity or increased metabolic activity seen in the 20 mg/L QD-COOH group, as no agglomerates on the embryo surface were observed in this treatment. Increased oxygen requiring activities were observed as severe hyperactivity in larvae exposed to low doses (2.5 nM) of uncoated CdTe QDs (Duan et al., 2013), as well as anxiety-related swimming behaviour changes after exposure to CdSe QDs (Zonouzi-Marand et al., 2022).
By comparing responses of zebrafish embryos following exposure to different functional groups QDs, it was evident that developmental and respiratory effects could not solely be attributed to metal ion toxicity. This supports findings by Tang et al. (2013) that reported effects of free Cd could not adequately explain QD toxicity in zebrafish embryos. Endpoints assessed in the present study also indicated a clear difference in the severity of responses between functionalized groups. Bimodal responses in metal uptake and malformations were observed for QD-PEG and QD-COOH treatments. This is most likely attributed to smaller NMs crossing the chorion membrane and eliciting direct toxicological responses, whereas larger agglomerates at higher exposure concentrations blocking the membrane pores and eliciting indirect toxicity. Bioaccumulation and elimination rates are important parameters in QD toxicokinetics and merit examination (King-Heiden et al., 2009). The QD-COOH and QD-PEG groups may be less bioavailable compared to the QD-NH3 group. Work by King-Heiden et al. (2009) found that QD-PEG with different chain lengths did exhibit toxicity towards zebrafish embryos but these effects were less severe than non-functionalized QDs. Several studies suggest that the terminal functional group of QDs and surface chemistry influences tissue distribution (Hardman, 2006). Thus, it is likely that surface chemistry, surface charge, and particle size influenced particle bioavailability and mode of toxicity, accounting for the differences observed in this study.
5 Conclusion
In summary, this study demonstrates that CdTe QDs cause developmental embryonic toxicity, resulting in persistent effects on larval development and respiration. We also established that different functionalized CdTe QDs materials tended to agglomerate and react differently in exposure media, which in turn influenced the toxicity. The QD-NH3-were the most toxic of the three tested QDs in this study. It was also seen that lower exposure concentrations were more toxic to survival and spinal deformations compared to high exposure concentrations, and no dose dependent effects were observed for all three materials in this study regardless of metal uptake. This was attributed to changes in the agglomerate sizes and colloidal behaviour of the particles in the media. This was evident in larger agglomerates forming at the high exposure concentrations, which resulted in decreased respiration rates, such as for the QD-NH3 group. It is thus concluded that three differently functionalized quantum dots with the same primary particle size can have different toxicities due to the particles characteristics, and that the toxicity observed is not solely due to metal body burden but rather a combination of particle specific effects due to aggregation, such as respiration impairment and metal uptake at lower concentrations. This highlights the need for further investigation.
Data availability statement
The raw data supporting the conclusion of this article will be made available by the authors, without undue reservation.
Ethics statement
The animal study was reviewed and approved by NWU-Animcare animal research ethics committee, North-West University, South Africa. Study NWU-00173-18-A5.
Author contributions
SB, TLB, and VW contributed to the conception and design of the study. SB wrote the first draft of the manuscript and performed the statistical analysis. All authors contributed to the manuscript revision, and read, and approved the submitted version.
Funding
The study was funded through the Health, Safety and Environmental Risk of Nanotechnology research platform of the South African Department of Science and Innovation (DSI). Funding for SBM through the National Research Foundation (NRF) (UID: 131561) is acknowledged. Opinions expressed and conclusions arrived at, are those of the authors and are not necessarily to be attributed to the NRF.
Acknowledgments
This is contribution number 754 of the North-West University (NWU) Water Research Group.
Conflict of interest
The authors declare that the research was conducted in the absence of any commercial or financial relationships that could be construed as a potential conflict of interest.
Publisher’s note
All claims expressed in this article are solely those of the authors and do not necessarily represent those of their affiliated organizations, or those of the publisher, the editors and the reviewers. Any product that may be evaluated in this article, or claim that may be made by its manufacturer, is not guaranteed or endorsed by the publisher.
References
Adair, J., Suvaci, E., and Sindel, J. (2001). Surface and colloid chemistry. Encycl. Mater. Sci. Technol. (2), 1–10. doi:10.1016/b0-08-043152-6/01622-3
Åkerman, M. E., Chan, W. C. W., Laakkonen, P., Bhatia, S. N., and Ruoslahti, E. (2002). Nanocrystal targeting in vivo. Proc. Natl. Acad. Sci. 99, 12617–12621. doi:10.1073/pnas.152463399
Asharani, P. V., Lianwu, Y., Gong, Z., and Valiyaveettil, S. (2011). Comparison of the toxicity of silver, gold and platinum nanoparticles in developing zebrafish embryos. Nanotoxicology 5, 43–54. doi:10.3109/17435390.2010.489207
Besinis, A., Van Noort, R., and Martin, N. (2014). Remineralization potential of fully demineralized dentin infiltrated with silica and hydroxyapatite nanoparticles. Dent. Mat. 30, 249–262. doi:10.1016/j.dental.2013.11.014
Boyle, D., Clark, N. J., and Handy, R. D. (2020). Toxicities of copper oxide nanomaterial and copper sulphate in early life stage zebrafish: Effects of pH and intermittent pulse exposure. Ecotoxicol. Environ. Saf. 190, 109985. doi:10.1016/j.ecoenv.2019.109985
Chen, F., and Gerion, D. (2004). Fluorescent CdSe/ZnS nanocrystal−peptide conjugates for long-term, nontoxic imaging and nuclear targeting in living cells. Nano Lett. 4, 1827–1832. doi:10.1021/nl049170q
Cho, W. S., Cho, M., Jeong, J., Choi, M., Cho, H. Y., Han, B. S., et al. (2009). Acute toxicity and pharmacokinetics of 13 nm-sized PEG-coated gold nanoparticles. Toxicol. Appl. Pharmacol. 236, 16–24. doi:10.1016/j.taap.2008.12.023
Deerfield, D. W., Lapadat, M. A., Spremulli, L. L., Hiskey, R. G., and Pedersen, L. G. (1989). The role of hydrated divalent metal ions in the bridging of two anionic groups. An ab initio quantum chemical and molecular mechanics study of dimethyl phosphate and formate bridged by calcium and magnesium ions. J. Biomol. Struct. Dyn. 6 (6), 1077–1091. doi:10.1080/07391102.1989.10506538
Derfus, A. M., Chan, W. C. W., and Bhatia, S. N. (2004). Probing the cytotoxicity of semiconductor quantum dots. Nano Lett. 4, 11–18. doi:10.1021/nl0347334
Duan, J., Yu, Y., Li, Y., Yu, Y., Li, Y., Huang, P., et al. (2013). Developmental toxicity of CdTe QDs in zebrafish embryos and larvae. J. Nanoparticle Res. 15, 1700. doi:10.1007/s11051-013-1700-8
ECHA (2022). Tellurium European chemicals agency (ECHA) registration dossier. Available at: https://echa.europa.eu/fr/registrationdossier/-/registered-dossier/14525/6/2/2
Gallud, A., Delaval, M., Kinaret, P., Marwah, V. S., Fortino, V., Ytterberg, J., et al. (2020). Multiparametric profiling of engineered nanomaterials: Unmasking the surface coating effect. Adv. Sci. 7, 2002221. doi:10.1002/advs.202002221
Gao, Y., Xie, Z., Feng, J., Ma, W., and Zhu, L. (2019). Different factors determined the toxicokinetics of organic chemicals and nanomaterials exposure to zebrafish (Danio Rerio). Ecotoxicol. Environ. Saf. 186, 109810. doi:10.1016/j.ecoenv.2019.109810
Ghobadian, M., Nabiuni, M., Parivar, K., Fathi, M., and Pazooki, J. (2015). Toxic effects of magnesium oxide nanoparticles on early developmental and larval stages of zebrafish (Danio rerio). Ecotoxicol. Environ. Saf. 122, 260–267. doi:10.1016/j.ecoenv.2015.08.009
Hardman, R. (2006). A toxicologic review of quantum dots: Toxicity depends on physicochemical and environmental factors. Environ. Health Perspect. 114, 165–172. doi:10.1289/ehp.8284
Hsu, P. C., O'Callaghan, M., Al-Salim, N., and Hurst, M. R. (2012). Quantum dot nanoparticles affect the reproductive system of Caenorhabditis elegans. Environ. Toxicol. Chem. 31, 2366–2374. doi:10.1002/etc.1967
Jaiswal, J. K., Mattoussi, H., Mauro, J. M., and Simon, S. M. (2003). Long-term multiple color imaging of live cells using quantum dot bioconjugates. Nat. Biotechnol. 21, 47–51. doi:10.1038/nbt767
Jason, R. E. S., Jennifer, A. N., and Maureen, A. W. (2009). Quantum dots for live cell and in vivo imaging. Int. J. Mol. Sci. 10, 441–491. doi:10.3390/ijms10020441
Jin, Y., Wu, S., Zeng, Z., and Fu, Z. (2017). Effects of environmental pollutants on gut microbiota. Environ. Pollut. 222, 1–9. doi:10.1016/j.envpol.2016.11.045
Joseph, E., and Singhvi, G. (2019). “Chapter 4 - multifunctional nanocrystals for cancer therapy: A potential nanocarrier,” in Nanomaterials for drug delivery and therapy. Editor A. M. Grumezescu (William Andrew Publishing).
Keremidarska-Markova, M., Hristova, K., Andreeva, T., Speranza, G., Wang, D., and Krasteva, N. (2018). Cytotoxicity evaluation of ammonia-modified graphene oxide particles in lung cancer cells and embryonic stem cells. Adv. Condens. Matter Phys., 2018, 1–11. doi:10.1155/2018/9571828
King-Heiden, T. C., Wiecinski, P. N., Mangham, A. N., Metz, K. M., Nesbit, D., Pedersen, J. A., et al. (2009). Quantum dot nanotoxicity assessment using the zebrafish embryo. Environ. Sci. Technol. 43, 1605–1611. doi:10.1021/es801925c
Krzykwa, J. C., Saeid, A., and Jeffries, M. K. S. (2019). Identifying sublethal endpoints for evaluating neurotoxic compounds utilizing the fish embryo toxicity test. Ecotoxicol. Environ. Saf. 170, 521–529. doi:10.1016/j.ecoenv.2018.11.118
Kumar, N., Willis, A., Satbhai, K., Ramalingam, L., Schmitt, C., Moustaid-Moussa, N., et al. (2020). Developmental toxicity in embryo-larval zebrafish (Danio rerio) exposed to strobilurin fungicides (azoxystrobin and pyraclostrobin). Chemosphere 241, 124980. doi:10.1016/j.chemosphere.2019.124980
Lacave, J. M., Retuerto, A., Vicario-Parés, U., Gilliland, D., Oron, M., Cajaraville, M. P., et al. (2016). Effects of metal-bearing nanoparticles (Ag, Au, CdS, ZnO, SiO2) on developing zebrafish embryos. Nanotechnology 27, 325102. doi:10.1088/0957-4484/27/32/325102
Larson, D. R., Zipfel, W. R., Williams, R. M., Clark, S. W., Bruchez, M. P., Wise, F. W., et al. (2003). Water-soluble quantum dots for multiphoton fluorescence imaging in vivo. Science 300, 1434–1436. doi:10.1126/science.1083780
Lee, K. J., Nallathamby, P. D., Browning, L. M., Osgood, C. J., and Xu, X.-H. N. (2007). In vivo imaging of transport and biocompatibility of single silver nanoparticles in early development of zebrafish embryos. ACS Nano 1, 133–143. doi:10.1021/nn700048y
Lovrić, J., Bazzi, H. S., Cuie, Y., Fortin, G. R., Winnik, F. M., and Maysinger, D. (2005). Differences in subcellular distribution and toxicity of green and red emitting CdTe quantum dots. J. Mol. Med. Berl. 83, 377–385. doi:10.1007/s00109-004-0629-x
Martinez, C. S., Igartua, D. E., Czarnowski, I., Feas, D. A., Alonso, S. D., and Prieto, M. J. (2019). Biological response and developmental toxicity of zebrafish embryo and larvae exposed to multi-walled carbon nanotubes with different dimension. Heliyon 5, e02308. doi:10.1016/j.heliyon.2019.e02308
Medintz, I. L., Uyeda, H. T., Goldman, E. R., and Mattoussi, H. (2005). Quantum dot bioconjugates for imaging, labelling and sensing. Nat. Mater. 4, 435–446. doi:10.1038/nmat1390
Michalet, X., Pinaud, F. F., Bentolila, L. A., Tsay, J. M., Doose, S., Li, J. J., et al. (2005). Quantum dots for live cells, in vivo imaging, and diagnostics. Science 307, 538–544. doi:10.1126/science.1104274
Mishra, Y. K., Verma, S. K., Nandi, A., Sinha, A., Patel, P., Jha, E., et al. (2021). Zebrafish (Danio rerio) as an ecotoxicological model for nanomaterial induced toxicity profiling. Precis. Nanomedicine 4 (1), 750–782. doi:10.33218/001c.21978
NANOSOLUTIONS (2013). Biological foundation for the safety classification of engineered nanomaterials (ENM): Systems biology approaches to understand interactions of enm with living organisms and the environment. D3.2 Particle behaviour in exposure media (report on task 3.4). Project ID: 309329. Funded under: FP7-NM. 2013-04-01 to 2017-03-31.
Paatero, I., Casals, E., Niemi, R., Özliseli, E., Rosenholm, J. M., and Sahlgren, C. (2017). Analyses in zebrafish embryos reveal that nanotoxicity profiles are dependent on surface-functionalization controlled penetrance of biological membranes. Sci. Rep. 7, 8423. doi:10.1038/s41598-017-09312-z
Pelster, B., and Bagatto, B. (2010). “7 - respiration,” in Fish physiology. Editors S. F. Perry, M. Ekker, A. P. Farrell, and C. J. brauner (Academic Press).
Petushkova, N. A., Kuznetsova, G. P., Larina, O. V., Kisrieva, Y. S., Samenkova, N. F., Trifonova, O. P., et al. (2015). One-dimensional proteomic profiling of Danio rerio embryo vitellogenin to estimate quantum dot toxicity. Proteome Sci. 13, 17. doi:10.1186/s12953-015-0072-7
Rocha, T. L., Mestre, N. C., Sabóia-Morais, S. M. T., and Bebianno, M. J. (2017). Environmental behaviour and ecotoxicity of quantum dots at various trophic levels: A review. Environ. Int. 98, 1–17. doi:10.1016/j.envint.2016.09.021
Ron, H. (2006). A toxicologic review of quantum dots: Toxicity depends on physicochemical and environmental factors. Environ. Health Perspect. 114, 165–172. doi:10.1289/ehp.8284
Samaee, S.-M., Rabbani, S., Jovanović, B., Mohajeri-Tehrani, M. R., and Haghpanah, V. (2015). Efficacy of the hatching event in assessing the embryo toxicity of the nano-sized TiO2 particles in zebrafish: A comparison between two different classes of hatching-derived variables. Ecotoxicol. Environ. Saf. 116, 121–128. doi:10.1016/j.ecoenv.2015.03.012
Shnoudeh, A. J., Hamad, I., Abdo, R. W., Qadumii, L., Jaber, A. Y., Surchi, H. S., et al. (2019). “Chapter 15 - synthesis, characterization, and applications of metal nanoparticles,” in Biomaterials and bionanotechnology. Editor R. K. Tekade (Academic Press).
Singh, S., Dhawan, A., Karhana, S., Bhat, M., and Dinda, A. K. (2020). Quantum dots: An emerging tool for point-of-care testing. Micromachines 11 (12), 1058. doi:10.3390/mi11121058
Sun, J., Zhang, Q., Wang, Z., and Yan, B. (2013). Effects of nanotoxicity on female reproductivity and fetal development in animal models. Int. J. Mol. Sci. 14, 9319–9337. doi:10.3390/ijms14059319
Tang, S., Cai, Q., Chibli, H., Allagadda, V., Nadeau, J. L., and Mayer, G. D. (2013). Cadmium sulfate and CdTe-quantum dots alter DNA repair in zebrafish (Danio rerio) liver cells. Toxicol. Appl. Pharmacol. 272, 443–452. doi:10.1016/j.taap.2013.06.004
Tatsi, K., Hutchinson, T. H., and Handy, R. D. (2020). Consequences of surface coatings and soil ageing on the toxicity of cadmium telluride quantum dots to the earthworm Eisenia fetida. Ecotoxicol. Environ. Saf. 201, 110813. doi:10.1016/j.ecoenv.2020.110813
Vassallo, J., Besinis, A., Boden, R., and Handy, R. D. (2018). The minimum inhibitory concentration (MIC) assay with Escherichia coli: An early tier in the environmental hazard assessment of nanomaterials? Ecotoxicol. Environ. Saf. 162, 633–646. doi:10.1016/j.ecoenv.2018.06.085
Vicario-Parés, U., Castañaga, L., Lacave, J. M., Oron, M., Reip, P., Berhanu, D., et al. (2014). Comparative toxicity of metal oxide nanoparticles (CuO, ZnO and TiO2) to developing zebrafish embryos. J. Nanoparticle Res. 16, 2550. doi:10.1007/s11051-014-2550-8
Voura, E. B., Jaiswal, J. K., Mattoussi, H., and Simon, S. M. (2004). Tracking metastatic tumor cell extravasation with quantum dot nanocrystals and fluorescence emission-scanning microscopy. Nat. Med. 10, 993–998. doi:10.1038/nm1096
Wang, J., Zhu, X., Chen, Y., and Chang, Y. (2012). “Application of embryonic and adult zebrafish for nanotoxicity assessment,” in Nanotoxicity. Methods in molecular biology (methods and protocols). Editor J. Reineke (Totowa, NJ: Humana Press).
Xu, Z., Zhang, Y.-L., Song, C., Wu, L.-L., and Gao, H.-W. (2012). Interactions of hydroxyapatite with proteins and its toxicological effect to zebrafish embryos development. PLOS ONE 7, e32818. doi:10.1371/journal.pone.0032818
Zhang, W., Lin, K., Miao, Y., Dong, Q., Huang, C., Wang, H., et al. (2012). Toxicity assessment of zebrafish following exposure to CdTe QDs. J. Hazard Mater 213-214, 413–420. doi:10.1016/j.jhazmat.2012.02.014
Zhang, X.-Q., Xu, X., Bertrand, N., Pridgen, E., Swami, A., and Farokhzad, O. C. (2012). Interactions of nanomaterials and biological systems: Implications to personalized nanomedicine. Adv. Drug Deliv. Rev. 64, 1363–1384. doi:10.1016/j.addr.2012.08.005
Zhang, S., Jiang, Y., Chen, C.-S., Creeley, D., Schwehr, K. A., Quigg, A., et al. (2013). Ameliorating effects of extracellular polymeric substances excreted by Thalassiosira pseudonana on algal toxicity of CdSe quantum dots. Aquat. Toxicol. 126, 214–223. doi:10.1016/j.aquatox.2012.11.012
Zhao, X., Wang, S., Wu, Y., You, H., and Lv, L. (2013). Acute ZnO nanoparticles exposure induces developmental toxicity, oxidative stress and DNA damage in embryo-larval zebrafish. Aquat. Toxicol. 136-137, 49–59. doi:10.1016/j.aquatox.2013.03.019
Zheng, N., Yan, J., Qian, W., Song, C., Zuo, Z., and He, C. (2021). Comparison of developmental toxicity of different surface modified CdSe/ZnS QDs in zebrafish embryos. J. Environ. Sci. 100, 240–249. doi:10.1016/j.jes.2020.07.019
Zhu, X., Zhu, L., Li, Y., Duan, Z., Chen, W., and Alvarez, P. J. (2007). Developmental toxicity in zebrafish (Danio rerio) embryos after exposure to manufactured nanomaterials: Buckminsterfullerene aggregates (nC60) and fullerol. Environ. Toxicol. Chem. 26, 976–979. doi:10.1897/06-583.1
Zhu, X., Wang, J., Zhang, X., Chang, Y., and Chen, Y. (2009). The impact of ZnO nanoparticle aggregates on the embryonic development of zebrafish (Danio rerio). Nanotechnology 20, 195103. doi:10.1088/0957-4484/20/19/195103
Zolotarev, K., Kashirtseva, V., Mishin, A., Belyaeva, N., Medvedeva, N., and Ipatova, O. (2012). Assessment of toxicity of Cdse/Cds/Zns/S,S-Dihydrolipoic Acid/Polyacrylic acid quantum dots at Danio rerio embryos and larvae. ISRN Nanotechnol., 2012, 1, 5. doi:10.5402/2012/914636
Keywords: metal uptake, oxygen consumption, carboxylate (COOH), ammonia (NH3), polyethylene glycol (PEG)
Citation: Bosch S, Botha TL and Wepener V (2023) Influence of different functionalized CdTe quantum dots on the accumulation of metals, developmental toxicity and respiration in different development stages of the zebrafish (Danio rerio). Front. Toxicol. 5:1176172. doi: 10.3389/ftox.2023.1176172
Received: 28 February 2023; Accepted: 11 April 2023;
Published: 02 May 2023.
Edited by:
Iseult Lynch, University of Birmingham, United KingdomReviewed by:
Andi Alijagic, Örebro University, SwedenChangjian Xie, Shandong University of Technology, China
Copyright © 2023 Bosch, Botha and Wepener. This is an open-access article distributed under the terms of the Creative Commons Attribution License (CC BY). The use, distribution or reproduction in other forums is permitted, provided the original author(s) and the copyright owner(s) are credited and that the original publication in this journal is cited, in accordance with accepted academic practice. No use, distribution or reproduction is permitted which does not comply with these terms.
*Correspondence: Suanne Bosch, Ym9zY2guc3Vhbm5lQGdtYWlsLmNvbQ==