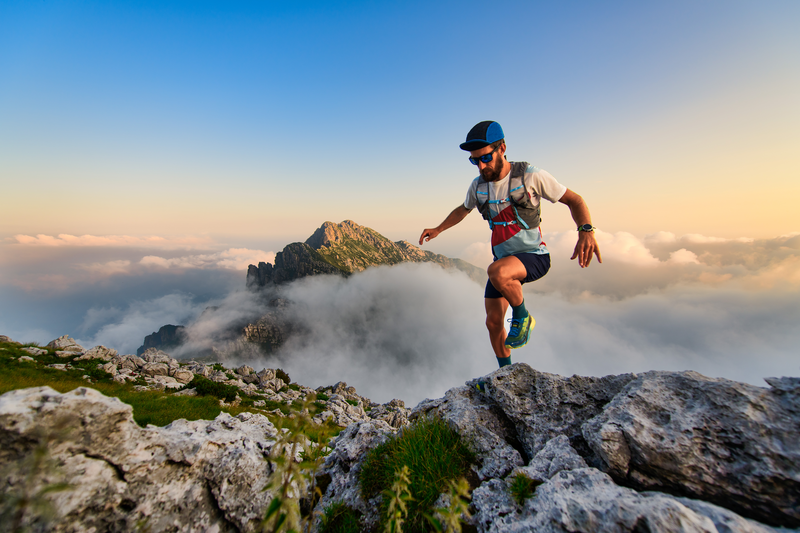
95% of researchers rate our articles as excellent or good
Learn more about the work of our research integrity team to safeguard the quality of each article we publish.
Find out more
ORIGINAL RESEARCH article
Front. Toxicol. , 02 May 2022
Sec. In Vitro Toxicology
Volume 4 - 2022 | https://doi.org/10.3389/ftox.2022.832780
Introduction: Many people living with HIV/AIDS (PLHIV) in Uganda widely use herbal medicines. However, their toxicity and safety have not been investigated. The use of these plants can potentially cause harmful effects to the health of patients. The purpose of this study was to determine the cytotoxicity of some commonly used medicinal plant species used by PLHIV.
Methods: The cytotoxicity of the plant extracts was determined with the AlamarBlue cell viability assay using the human glioblastoma cell line U87.CD4.CXCR4. The cells were treated with varying concentrations of extracts of Warburgia ugandensis, Erythrina abyssinica, Cryptolepis sanguinolenta, Albizia coriaria, Psorospermum febrifugium, Gymnosporia senegalensis, Zanthoxylum chalybeum, Securidaca longipendunculata, Vachellia hockii, Gardenia ternifolia, and Bridelia micrantha reconstituted with ethanol and dimethyl sulfoxide (DMSO). Using regression analysis, the half maximal cytotoxic concentration (CC50) of the plant extracts were calculated from exponential curve fits, since they provided the highest coefficient of determination, R2.
Results: The ethanol extracts of W. ugandensis (CC50 = 7.6 μg/ml) and A. coriaria (CC50 = 1.5 μg/ml) as well as the DMSO-reconstituted extracts of W. ugandensis (CC50 = 6.4 μg/ml) and A. coriria (CC50 = < 4 μg/ml) were highly cytotoxic. The cytotoxicity of W. ugandensis and A. coriaria compared well with the indigenous traditional knowledge of the toxic effects experienced when the plants were not used correctly. However, the cytotoxicity of most of the plant extracts (15/22) was low to moderate (CC50 = 21–200 μg/ml).
Conclusion: Most of the plant species tested in this study had low to moderate cytotoxicity against U87.CD4.CXCR4 cells, except W. ugandensis and A. coriria which were highly cytotoxic.
Traditional medicines are very popular and are widely used among people living with HIV/AIDS (PLHIV) (Ahmad, 2005; Mills et al., 2005; Anywar et al., 2020a, 2020b, 2020c). AIDS is the world’s commonest immunosuppressive disease. It is caused by the human immunodeficiency virus (Bedoya et al., 2002; Gea-Banacloche, 2006). There are more than 37.6 million PLHIV worldwide, with 61% of total in Sub Saharan Africa (UNAIDS, 2021). The HIV prevalence among adults in Uganda was 5.4%, though higher in females (6.8%) (UNAIDS, 2020). In Uganda, more than 90% of the population, especially in rural areas, rely on traditional herbal remedies to manage their daily health care needs (Kamatenesi-Mugisha and Oryem-Origa, 2007). This is largely attributed to the accessibility, affordability, and cultural appropriateness of the herbal medicine.
Traditional medicinal practitioners (TMP) in Uganda use over 235 medicinal plant species for treating PLHIV (Anywar et al., 2020a; Anywar et al., 2020b). We selected 11 popular medicinal plant species for cytotoxicity analysis from a previous ethnobotanical study conducted by Anywar et al. (2020a), on medicinal plant species used to treat PLHIV in Uganda. Most of the plants are boiled as decoctions or concoctions.
The use of traditional medicine has been commonly associated with severe side effects. In South Africa, for instance, high rates of dehydration, vomiting, diarrhoea, and altered mental status have been observed in patients who reported recent use of traditional medicines (Luyckx et al., 2004). Similarly, traditional herbal treatment has been associated with dysfunctions of the liver and kidneys resulting in high patient mortality (Luyckx et al., 2004). Acute renal failure is one of the most severe, but under-recognized, complications of traditional medicine use and yet it forms an important aetiology of renal diseases in routine clinical practice (Luyckx et al., 2004; Luyckx, 2012). About 35% of all cases of acute renal failure in Africa have been associated with the use of herbal remedies. Moreover, this figure is thought to be an underestimate because of the secrecy surrounding traditional health practices and the use of traditional remedies (Isnard Bagnis et al., 2004; Luyckx et al., 2004).
Contamination from ingestion of potentially toxic medicinal herbs, incorrect substitution of required medicine with toxic herbs, adulteration, herb-drug interactions, the use of suboptimal un-standardized herbal medicines and lack of regulations or their enforcement are some of the main challenges that plague the use of herbal medicines (Ernst, 2002; Liwa and Jaka, 2016). It is also known that the source, composition, and preparation of herbal remedies vary with prevailing local healing practices and level of knowledge and skill of the herbalist (Jha and Rathi, 2008; Anywar et al., 2020a).
Many herbs contain active compounds. Only a few of them have been evaluated for efficacy and safety, even when various reports have implicated many of them in toxicity (Jha & Rathi, 2008). The identities of toxic substances contained in African herbal medicines, their toxicology and pathogenesis are largely unknown (Luyckx et al., 2002; Anywar et al., 2021). This makes patients with pre-existing renal insufficiency or at risk of impairment more predisposed to kidney insults from such herbal remedies (Luyckx, 2012).
Cytotoxicity tests are useful to screen chemicals for their intrinsic and relative toxicities. This helps in determining the potential toxic or harmful effect of such compounds to human health that may occur inadvertently during use (Castaño and Gómez-Lechón, 2005; Schultz et al., 2020a). It is therefore important to establish their safety. The purpose of this study was to determine the cytotoxicity of some commonly used medicinal plant species treat people living with HIV/AIDS in Uganda.
Plant extraction and preparation were done at the Fraunhofer Institute for Cell Therapy & Immunology (IZI), in Leipzig, Germany. The cytotoxicity assays were conducted at the Institute for Medical Microbiology & Virology, University Clinics & Faculty of Medicine, University of Leipzig, Leipzig, Germany, between June and December 2019.
Eleven medicinal plant species were collected from different parts of Uganda between March and September 2017. The medicinal plant species administered were all used by herbalists to treat PLHIV in Uganda (Anywar et al., 2020a). Voucher specimens of the plant species were collected using standard procedures described in Martin (1995) and deposited in the Makerere University herbarium for identification. The identified specimens were classified using the Kew Medicinal Plant Names Services (MPNS), https://mpns.science.kew.org/mpns-portal/, on 9th December 2021. The plant families were checked against the Angiosperm Phylogeny Group IV. The plant species selected and their voucher numbers were: Warburgia ugandensis Sprague (AG383), Erythrina abyssinica Lam. (AG608), Cryptolepis sanguinolenta (Lindl.) Schltr., (AG480), Albizia coriaria Welw. ex Oliv., (AG366), Psorospermum febrifugium Spach (AG483), Gymnosporia senegalensis (Lam.) Loes. (AG462), Zanthoxylum chalybeum Engl. (AG632), Securidaca longipendunculata Fresen. (AG363), Vachellia hockii (De Wild.) Seigler and Ebinger. (AG428), Gardenia ternifolia Schumach. and Thonn., (AG453) and Bridelia micrantha (Hochst.) Baill (AG452).
Except for C. sanguinolenta where the root or root bark was used, all the other plant extracts were obtained from the barks of the respective plant species. Fresh material from all plant species were chipped and air-dried for 3–4 weeks at room temperature in a well-ventilated room. The dried plant material was ground into fine powder using an electric mill. The plant materials were stored in air tight containers to protect them from oxidation following the World Health Organization guidelines on good agricultural and collection practices (GACP) for medicinal plants (WHO, 2003).
The plant material was extracted in 80% ethanol using the following procedure. About 1 g of pulverized plant material from each species was put in Eppendorf tubes. Then 10 ml of 80% ethanol was added to each of the tubes and vortexed to dissolve the plant material. The tubes were slowly and gently heated in a thermocycler to evaporate off the excess solvent at 35°C. All the extracts were made up to a concentration of 30 mg/ml by redissolving them in both DMSO and Ethanol. The Eppendorf tubes with the extracts were then labelled and stored in a refrigerator at 4°C for further tests.
Cell viability was measured using the human glioblastoma cell line U87.CD4.CXCR4 obtained through the National Institute for Biological Standards and Control (NIBSC) Centre for AIDS reagents, United Kingdom. The U87.CD4.CXCR4 cell-line was selected for this study as the most appropriate because the plant extracts would ultimately have to be tested for antiviral activity against HIV-1. U87 glioma cell lines are stably transfected with CD4 and CCR5 or CXCR4, which they express (Bjorndal et al., 1997; Princen et al., 2004). Additionally, U87 cells have an infinite lifespan and are easy to handle (Allen et al., 2016). The U87 are a human primary glioblastoma cell line that is one of the most widely studied and commonly used cell lines (Westermark et al., 1973; Clark et al., 2010). The malignant glioma is an aggressive type of primary brain cancer in adults (Pollard et al., 2009) with a very poor survival rate of 36.5% after 1 year (Ostrom et al., 2014).
The cells were prepared following the laboratory’s standard operating procedures and instructions from the manufactures. The cells were retrieved from storage under vapour phase of liquid nitrogen at −196°C. Then a 1 ml cell aliquot was quickly thawed in a water bath at 37°C. The cells were transferred to 9 ml warm Dulbecco’s Modified Eagles Medium (DMEM) supplemented with 10% foetal calf serum (FCS) and 1% antibiotics (100 U/ml Penicillin, 100 μg/ml Streptomycin (Gibco, Thermofisher, Life Technologies Ltd., United Kingdom) in a conical 15 ml tube and gently mixed. The cells were centrifuged at 1,200 rpm for 5 min to cause them to pellet. The medium was discarded and the cell pellet was gently resuspended in 10 ml of fresh warm medium.
The cells were divided into two T25 flasks containing 5 ml warm medium and incubated (Panasonic CO2 incubator, Model MCO-20AIC-PE, Japan) for 48 h at 37°C in a humidified atmosphere at 5% CO2, after which fresh medium was added. After the cells reached a confluence of 80–90%, they were split in a ratio of 1:4 to 1:10. The culture medium was removed and discarded. The cells were briefly rinsed with an equal volume of phosphate-buffered saline (PBS, pH 7.4) (Gibco/Invitrogen) which was discarded. The cells were then detached from the surface of the culture flask with 3 ml 0.25% (w/v) trypsin +0.53 mM EDTA, Gibco/Invitrogen, United States). The detached cells were observed under an inverted microscope. Then 10 ml of growth medium was added and cells were collected by gently pipetting. Different aliquots of the cell suspension were added to new culture vessels. The cells were incubated and harvested during the exponential growth phase. The viability of the cells was determined by trypan blue exclusion using a Neubauer counting chamber (Altman et al., 1993; Tran et al., 2011).
The cytotoxicity of the plant extracts was determined using the AlamarBlue® (Thermo Scientific, United States) cell viability assay following the manufacturer’s manual. AlamarBlue is a water-soluble resazurin based reagent added to the cell culture that changes its colour upon chemical reduction by viable cells. Inhibition of cell metabolism, cell proliferation and destruction of cells by cytotoxic agents slows down the change of colour. Resazurin was used to quantitatively measure the viability of mammalian cell lines as a rapid, non-toxic, sensitive, and reliable growth indicator (O’Brien et al., 2000; Schoonen et al., 2012). Different concentrations of the plant extracts were tested in comparison to ethanol.
The plant extracts were prepared from a stock solution of 30 mg/ml in Eppendorf tubes. The extracts were pre-diluted 1:30 to a concentration of 1 mg/ml. Ethanol and DMSO were pre-diluted in medium to 6.2%. Then 100 µl of medium was added to each well of a 96-well flat-bottomed plate, followed by 100 µl of plant extract in the second row in duplicate. The medium and extracts were mixed in the wells followed by the transfer of 100 µl to the next row. The process was repeated with all diluted plant extracts. The carryover in the last row of 100 μl was discarded. Then 100 µl of cells was added to each well leading to plant extract concentrations starting with the undiluted row with the highest concentration of 250 μg/ml right through 125, 62.5, 31.3, 15.6, 8, and 4 μg/ml which was the lowest concentration. The 6.2% ethanol and DMSO were diluted in the same way. In the last pipetting phase, 20 μl of AlamarBlue reagent was added to each of the wells. The optical density (OD) was measured spectrophotometrically using an automatic plate reader (Tecan, Sunrise-Basic Tecan, Austria, GmbH, model 16039400) with a test wavelength of 570 nm and a reference wavelength of 600 nm. The readings were taken at 1, 3, 5, 24, and 50 h, respectively. Higher OD values indicated viable, metabolically active cells. Low OD values indicated cytotoxicity. The readings taken after 24 h of incubation were used to calculate the degree of metabolic activity in the presence of different concentrations of plant extract using the formula:
Regression analysis was performed using Microsoft Excel 2011. The 50% cytotoxicity values (CC50) of the plant extracts and the coefficient of determination R2 were calculated. The CC50 values represent the concentration that reduced the chemical reduction potential of the cell culture by 50%. The coefficient of determination R2 indicates the goodness of fit of the measured values and the regression curve. To determine CC50 values, a best curve fit analysis was performed with linear, logarithmic, exponential, and polynomic curve equations offered by the Microsoft Excel computer spreadsheet program. Exponential curves provided the highest coefficient of determination, R2, in most cases. Therefore, exponential curve equations were used to calculate the CC50 values.
The National Cancer Institute (NCI) classified cytotoxic compounds as: 1) highly active if IC50 ≤ 20 μg/ml, 2) moderately active if IC50 = 21–200 μg/ml, 3) weakly active if IC50 = 201–500 μg/ml and inactive if IC50 = > 501 μg/ml (Geran, 1972). Using the NCI system with a slight modification to replace activity with cytotoxicity, we categorized both the ethanol and DMSO-reconstituted extracts of A. coriaria and W. ugandensis as highly cytotoxic. Generally, there was a dose dependent increase in cytotoxicity with all the plant extracts tested.
Extracts from V. hockii, B. micrantha, E. abyssinica, G. ternifolia Subsp. jovis-tonantis, P. febrifugium, G. senegalensis, C. sanguinolenta (DMSO extract), S. longipendunculata (DMSO extract), and Z. chalybeaum (EtOH extract) were moderately cytotoxic. Extracts from C. sanguinolenta (EtOH extract), S. longipendunculata (EtOH extract), and Z. chalybeaum (DMSO extract) were weakly cytotoxic. Both ethanol and DMSO did affect cell growth by less than 10% at a concentration of 0.83 %v/v which was the maximum concentration of extractant present in the tested plant extracts (Table 1).
The aim of the examination was to determine the toxicity of ethanol and DMSO extracts of selected medicinal plants in a cell culture model. The glioblastoma cell line U87 was chosen for this analysis because the existence of a U87 model for examining the inhibition of HIV infection in vitro (Bjorndal et al., 1997; Princen et al., 2004). The data on inhibition of HIV is still under review. Several methods are in use to examine the toxicity of medicinal compounds in cell culture (Cost et al., 2002; Auld et al., 2008; Wang et al., 2014). Repeated measurements of cytotoxic effects at several time points were done. Above pH 6.5, resazurin stains the cell culture medium purple. Upon chemical reduction, the colour of the cell culture medium changes to pink. Resazurin reduction occurs in viable, untreated cells. The colour of the cell cultures were measured spectrophotometrically. High absorbance of light (optical density) at 570 nm (pink) indicated viable cells whereas low optical density values (purple) indicated cytotoxicity. We monitored the metabolism of the cells for 5–50 h. The 24 h values were chosen for the calculation of the CC50, because untreated cells reached maximum pink colour intensity at this time point.
Cytotoxicity was examined with twofold dilutions of the extracts. A concentration range between 4 and 250 μg/ml was optimal for most of the extracts. The coefficients of determination were greater than 0.7 in all but one instance indicating that the calculated CC50 values were fairly precise. At 250 μg/ml diluted extracts contained 0.83% EtOH and DMSO. At this concentration, EtOH and DMSO alone showed less than 10% cytotoxicity indicating that cytotoxicity of the extractants was negligeable (Table 1 and Supplementary Figure S1).
Herbalists generally prepare the medicinal plant extracts using water. However, in our experiments, ethanol and DMSO were used for the extraction. The ethanol extracts were generally less cytotoxic than the DMSO extracts. A compound is regarded to be cytotoxic if it prevents cellular attachment, causes dramatic morphological changes, adversely affects replication rate, or leads to a reduction in overall viability (Horvath, 1980). It is worth noting that the expression of these effects depends on the length of time of exposure of the cells to the particular compounds, mechanism of cytotoxicity and the kind of compound under test (Riss and Moravec, 2004; Di Nunzio et al., 2017).
Other studies have also shown W. ugandensis and A. coriaria, the most highly cytotoxic plant species in our study to be cytotoxic in other cell lines. Mwitari et al. (2013) showed the ethanol extracts of W. ugandensis were cytotoxic for intestinal epithelial cells IEC-6, (IC50 < 50 μg/ml). W. ugandensis contains cytotoxic sesquiterpenes called muzigadials (Olila et al., 2001) and causes toxic side effects when the recommended dosage is exceeded as shown in Table 2. However, water extracts of W. ugandensis extracts were considered nontoxic in Vero E6 cells (CC50 > 250 μg/ml) and in BALB/c mice (LD50 > 5000 mg/kg body weight, Karani et al., 2013). Both the DMSO-reconstituted and ethanol extracts of A. coriaria from this study were highly cytotoxic. Kigondu et al. (2009) showed that the methanol and aqueous extracts of A. coriaria had very low cytotoxicity (CC50 > 500 μg/ml) against human embryonic lung fibroblast cells (HELF). The methanol, ethanol, ethyl acetate and diethyl ether extracts of A. coriaria were not toxic for the human keratinocyte cell line HaCaT (IC50 > 512) (Schultz et al., 2020b). Albizia spp. contains a toxic compound, 4-methoxypyridoxine that is a vitamin B6 antagonist (Botha and Penrith, 2008). A. coriaria and W. ugandensis are widely used in traditional herbal preparations as well as commercial herbal preparations in Uganda (Anywar et al., 2020a; Kaggwa et al., 2022).
TABLE 2. A comparison of cytotoxicity and medical indication, use and traditional knowledge on toxicity of A. coriaca and W. ugandensis
B. micrantha showed moderate cytotoxicity in our study. In other studies, B. micrantha was cytotoxic to HeLa cells (IC50 = 8.9 μg/ml), human breast cells (MCF-12A, IC50 = 24.2 μg/ml) (Steenkamp et al., 2009), CCRF-CEM leukaemia cells (IC50 = 9.43 μg/ml and 23.5 μg/ml) for leaf and bark extract in dichloromethane and methanol, respectively (Omosa et al., 2016). B. micrantha has also been shown to be toxic to brine shrimp (50% lethal concentration, LC50 = 77 μg/m) for the water stem bark extract (Osebe et al., 2016) but mildly toxic for the ethanol root extract (LC50 = 30 μg/ml) (Moshi et al., 2010).
The ethanol extracts of P. febrifugium exhibited significant in vivo cytotoxicity against P-388 lymphocytic leukaemia cells (3 PS) in mice (Abou-shoer et al., 1989). Psorospermin is a cytotoxic dihydrofuranoxanthone first isolated from the roots of P. febrifugum (Gebre-Mariam et al., 2006). Psorospermin showed significant activity against human colon adenocarcinoma (HT-29), human lung carcinoma (A-549), and human breast adenocarcinoma (MCF-7) cell lines (Cassady et al., 1990).
The ethanol root extract of S. longipendunculata significantly inhibited proliferation of U87 cells (IC50 = 20.54 μg/ml) (Ngulde et al., 2019). The chloroform root extract of S. longipedunculata was preferentially cytotoxic to PANC-1 human pancreatic cancer cells. The aqueous root bark extract was toxic to Ehrlich ascites tumour cells with a cytotoxicity of 82.5% at 1000 μg/ml, and an IC50 of 67 μg/ml (Lawal et al., 2012). The methanol root extract of S. longipendunculata is relatively toxic to brine shrimp with a LC50 of 77.1 μg/ml (Moshi et al., 2006), LC50 of 74.18 μg/ml (Adiele et al., 2013). The extract was also toxic in albino mice (LD50 = 1.74 g/kg and 0.02 g/kg) when administered orally and intraperitoneally, respectively (Adeyemi et al., 2010). Toxic saponins and methyl salicylate, a suspected nephrotoxin from S. longipendunculata can cause renal damage (Colson and De Broe, 2005). Xanthones from S. longipedunculata exhibited potent and selective cytotoxicity against PANC-1 human pancreatic cancer cells (Dibwe et al., 2013). The aqueous root extract of C. sanguinolenta exhibited potent toxicity to a variety of mammalian cells in vitro including the Chinese hamster lung fibroblast cell line V79-MZ) (Ansah and Gooderham, 2002). C. sanguinolenta contains the alkaloid cryptolepine and isocryptolepine (Grellier et al., 1996; Bonjean et al., 1998). Cryptolepine was cytotoxic to B16 melanoma cells (IC50 = 0.3 μg/ml) (Bonjean et al., 1998). Skilled herbalists normally administer known toxic medicinal plants albeit in low doses and in polyherbal preparations (Anywar et al., 2020a). This can potentially neutralize the toxicity of some medicinal plant compounds. However, polyherbal preparations can also have unpredictable and complicated effects arising from the various interactions that can occur among individual components (Che et al., 2013; Di Nunzio et al., 2017). The cytotoxicity data on the two highly cytotoxic plants: W. ugandensis and A. coriaria corresponds to the traditional knowledge of herbalists from our earlier study (Anywar et al., 2020a) about the toxic side effects experienced with the incorrect use of these plants. Although single species plant extracts were tested, it is common for the herbalists to use mixtures of multiple plant species for treatment. Therefore, the corresponding cytotoxicities of standard plant extract mixtures need to be investigated.
Most of the plant species extracts tested in this study had low to moderate cytotoxicities against U87.CD4.CXCR4 cells in vitro, except for W. ugandensis and A. coriria extracts which were both highly cytotoxic. The high in vitro cytotoxicity levels of W. ugandensis and A. coriria correspond well with the toxic effects experienced by people who incorrectly used these herbs as reported by herbalists. Therefore, the correct use of herbal medicines is important in mitigating toxic side effects.
The datasets presented in this study can be found in online repositories. The names of the repository/repositories and accession number(s) can be found below: https://data.mendeley.com/datasets/25kdprt3ch/2, doi: 10.17632/25kdprt3ch.2.
GA conceptualised the study and designed the methods with CJ. GA conducted the experiments under the guidance of CJ and AS. GA analysed the data and wrote the manuscript with CJ and AS. CJ, HO-O, and EK helped in developing and writing the manuscript. All authors read and reviewed the manuscript. All authors read and approved the final manuscript.
This research was supported by the Consortium for Advanced Research Training in Africa (CARTA). CARTA is jointly led by the African Population and Health Research Center and the University of the Witwatersrand and funded by the Carnegie Corporation of New York (Grant No—G-19-57145), Sida (Grant No:54100113), Uppsala Monitoring Centre and the DELTAS Africa Initiative (Grant No: 107768/Z/15/Z). The DELTAS Africa Initiative is an independent funding scheme of the African Academy of Sciences (AAS)’s Alliance for Accelerating Excellence in Science in Africa (AESA) and supported by the New Partnership for Africa’s Development Planning and Coordinating Agency (NEPAD Agency) with funding from the Wellcome Trust (UK) and the UK government. The statements made and views expressed are solely the responsibility of the fellow/researchers.
The authors declare that the research was conducted in the absence of any commercial or financial relationships that could be construed as a potential conflict of interest.
All claims expressed in this article are solely those of the authors and do not necessarily represent those of their affiliated organizations, or those of the publisher, the editors and the reviewers. Any product that may be evaluated in this article, or claim that may be made by its manufacturer, is not guaranteed or endorsed by the publisher.
We acknowledge the technical assistance of both CJ and Katrin Bräutigam. We are grateful to Protase Rwaburindori for taxonomic expertise, the Traditional Medicine Practitioners who gave their consent to take part in this study and to share their knowledge with us and the Directorate of Research & Graduate Training, Makerere University.
The Supplementary Material for this article can be found online at: https://www.frontiersin.org/articles/10.3389/ftox.2022.832780/full#supplementary-material
Abou-shoer, M., Suwanborirux, K., Chang, C.-j., and Cassady, J. M. (1989). Novel Tetrahydrofurobenzofuranoxanthones from Psorospermamum febrifugium. Tetrahedron Lett. 30, 3385–3388. doi:10.1016/s0040-4039(00)99251-7
Adeyemi, O. O., Akindele, A. J., Yemitan, O. K., Aigbe, F. R., and Fagbo, F. I. (2010). Anticonvulsant, Anxiolytic and Sedative Activities of the Aqueous Root Extract of Securidaca longepedunculata Fresen. J. Ethnopharmacology 130, 191–195. doi:10.1016/j.jep.2010.04.028
Adiele, R., Fakae, B., and Isuzu, I. (2013). Anthelmintic Activity of Securidaca longepedunculata (Family: Polygalaceae) Root Extract in Mice, In Vitro and In Vivo. Asian Pac. J. Trop. Med. 6, 841–846. doi:10.1016/s1995-7645(13)60150-9
Ahmad, K. (2005). Herbal Treatment for HIV/AIDS Not Recommended. Lancet Infect. Dis. 5, 537. doi:10.1016/s1473-3099(05)70205-x
Allen, M., Bjerke, M., Edlund, H., Nelander, S., and Westermark, B. (2016). Origin of the U87MG Glioma Cell Line: Good News and Bad News. Sci. Transl Med. 8, 354re3. doi:10.1126/scitranslmed.aaf6853
Altman, S. A., Randers, L., and Rao, G. (1993). Comparison of Trypan Blue Dye Exclusion and Fluorometric Assays for Mammalian Cell Viability Determinations. Biotechnol. Prog. 9, 671–674. doi:10.1021/bp00024a017
Ansah, C., and Gooderham, N. J. (2002). The Popular Herbal Antimalarial, Extract of Cryptolepis Sanguinolenta, Is Potently Cytotoxic. Toxicol. Sci. 70, 245–251. doi:10.1093/toxsci/70.2.245
Anywar, G., Kakudidi, E., Byamukama, R., Mukonzo, J., Schubert, A., and Oryem-Origa, H. (2020a). Indigenous Traditional Knowledge of Medicinal Plants Used by Herbalists in Treating Opportunistic Infections Among People Living with HIV/AIDS in Uganda. J. Ethnopharmacology 246, 112205. doi:10.1016/j.jep.2019.112205
Anywar, G., Kakudidi, E., Byamukama, R., Mukonzo, J., Schubert, A., and Oryem-Origa, H. (2020b). Medicinal Plants Used by Traditional Medicine Practitioners to Boost the Immune System in People Living with HIV/AIDS in Uganda. Eur. J. Integr. Med. 35, 101011. doi:10.1016/j.eujim.2019.101011
Anywar, G., Kakudidi, E., Byamukama, R., Mukonzo, J., Schubert, A., and Oryem-Origa, H. (2020c). Data on Medicinal Plants Used by Herbalists for Boosting Immunity in People Living with HIV/AIDS in Uganda. Data in Brief 29, 105097. doi:10.1016/j.dib.2019.105097
Anywar, G., Kakudidi, E., Byamukama, R., Mukonzo, J., Schubert, A., Oryem-Origa, H., et al. (2021). A Review of the Toxicity and Phytochemistry of Medicinal Plant Species Used by Herbalists in Treating People Living with HIV/AIDS in Uganda. Front. Pharmacol. 12, 435. doi:10.3389/fphar.2021.615147
Auld, D. S., Thorne, N., Nguyen, D.-T., and Inglese, J. (2008). A Specific Mechanism for Nonspecific Activation in Reporter-Gene Assays. ACS Chem. Biol. 3, 463–470. doi:10.1021/cb8000793
Bedoya, L. M., Palomino, S. S., Abad, M. J., Bermejo, P., and Alcami, J. (2002). Screening of Selected Plant Extracts for In Vitro Inhibitory Activity on Human Immunodeficiency Virus. Phytother. Res. 16 (6), 550–554. doi:10.1002/ptr.992
Björndal, A., Deng, H., Jansson, M., Fiore, J. R., Colognesi, C., Karlsson, A., et al. (1997). Coreceptor Usage of Primary Human Immunodeficiency Virus Type 1 Isolates Varies According to Biological Phenotype. J. Virol. 71, 7478–7487. doi:10.1128/JVI.71.10.7478-7487.1997
Bonjean, K., De Pauw-Gillet, M. C., Defresne, M. P., Colson, P., Houssier, C., Dassonneville, L., et al. (1998). The DNA Intercalating Alkaloid Cryptolepine Interferes with Topoisomerase II and Inhibits Primarily Dna Synthesis in B16 Melanoma Cells. Biochemistry 37, 5136–5146. doi:10.1021/bi972927q
Botha, C. J., and Penrith, M.-L. (2008). Poisonous Plants of Veterinary and Human Importance in Southern Africa. J. Ethnopharmacology 119, 549–558. doi:10.1016/j.jep.2008.07.022
Cassady, J. M., Baird, W. M., and Chang, C.-J. (1990). Natural Products as a Source of Potential Cancer Chemotherapeutic and Chemopreventive Agents. J. Nat. Prod. 53, 23–41. doi:10.1021/np50067a003
Castaño, A., and Gómez-Lechón, M. J. (2005). Comparison of Basal Cytotoxicity Data between Mammalian and Fish Cell Lines: A Literature Survey. Toxicol. Vitr. 19, 695–705.
Che, C.-T., Wang, Z., Chow, M., and Lam, C. (2013). Herb-Herb Combination for Therapeutic Enhancement and Advancement: Theory, Practice and Future Perspectives. Molecules 18, 5125–5141. doi:10.3390/molecules18055125
Clark, M. J., Homer, N., O'Connor, B. D., Chen, Z., Eskin, A., Lee, H., et al. (2010). U87MG Decoded: the Genomic Sequence of a Cytogenetically Aberrant Human Cancer Cell Line. Plos Genet. 6, e1000832. doi:10.1371/journal.pgen.1000832
Colson, C. R. D., and De Broe, M. E. (2005). Kidney Injury from Alternative Medicines. Adv. Chronic Kidney Dis. 12, 261–275. doi:10.1016/j.ackd.2005.03.006
Cos, P., Hermans, N., De Bruyne, T., Apers, S., Sindambiwe, J. B., Witvrouw, M., et al. (2002). Antiviral Activity of Rwandan Medicinal Plants against Human Immunodeficiency Virus Type-1 (HIV-1). Phytomedicine 9, 62–68. doi:10.1078/0944-7113-00083
Di Nunzio, M., Valli, V., Tomás-Cobos, L., Tomás-Chisbert, T., Murgui-Bosch, L., Danesi, F., et al. (2017). Is Cytotoxicity a Determinant of the Different In Vitro and In Vivo Effects of Bioactives? BMC Complement. Altern. Med. 17, 453–448. doi:10.1186/s12906-017-1962-2
Dibwe, D. F., Awale, S., Kadota, S., Morita, H., and Tezuka, Y. (2013). Heptaoxygenated Xanthones as Anti-austerity Agents from Securidaca longepedunculata. Bioorg. Med. ChemistryBioorg Med 451 Chem 21, 7663–7668. doi:10.1016/j.bmc.2013.10.027
Ernst, E. (2002). Toxic Heavy Metals and Undeclared Drugs in Asian Herbal Medicines. Trends Pharmacol. Sci. 23, 136–139. doi:10.1016/S0165-6147(00)01972-6
Gea-Banacloche, J. C. (2006). “Immunomodulation,” in Principles of Molecular Medicine (Springer), 893–904.
Gebre-Mariam, T., Neubert, R., Schmidt, P. C., Wutzler, P., and Schmidtke, M. (2006). Antiviral Activities of Some Ethiopian Medicinal Plants Used for the Treatment of Dermatological Disorders. J. Ethnopharmacology 104, 182–187. doi:10.1016/j.jep.2005.08.071
Geran, R. I. (1972). Protocols for Screening Chemical Agents and Natural Products against Animal Tumors and Other Biological Systems. Cancer Chemother. Rep. 3, 17–27.
Grellier, P., Ramiaramanana, L., Millerioux, V., Deharo, E., Schrével, J., Frappier, F., et al. (1996). Antimalarial Activity of Cryptolepine and Isocryptolepine, Alkaloids Isolated from. Cryptolepsis sanguinolenta Phyther. Res. 10, 469 317–321. doi:10.1002/(sici)1099-1573(199606)10:4<317::aid-ptr858>3.0.co;2-0
Horváth, S. (1980). Cytotoxicity of Drugs and Diverse Chemical Agents to Cell Cultures. Toxicology 16, 59–66. doi:10.1016/0300-483x(80)90110-9
Isnard Bagnis, C., Deray, G., Baumelou, A., Le Quintrec, M., and Vanherweghem, J. L. (2004). Herbs and the Kidney. Am. J. Kidney Dis. 44, 1–11. doi:10.1053/j.ajkd.2004.02.009
Jha, V., and Rathi, M. (2008). Natural Medicines Causing Acute Kidney Injury. Semin. Nephrol. 28, 416–428. doi:10.1016/j.semnephrol.2008.04.010
Kaggwa, B., Kyeyune, H., Munanura, E. I., Anywar, G., Lutoti, S., Aber, J., et al. (2022). Safety and Efficacy of Medicinal Plants Used to Manufacture Herbal Products with Regulatory Approval in Uganda: A Cross-Sectional Study. Evid. Based Complementary Altern. Med. 2022, 1304839.
Kamatenesi-Mugisha, M., and Oryem-Origa, H. (2007). Medicinal Plants Used to Induce Labour during Childbirth in Western Uganda. J. Ethnopharmacol 109, 1–9. doi:10.1016/j.jep.2006.06.011
Karani, L. W., Tolo, F. M., Karanja, S. M., and Khayeka−Wandabwa, C. (2013). Safety of Prunus africana and Warburgia ugandensis in Asthma Treatment. South Afr. J. Bot. 88, 183–190. doi:10.1016/j.sajb.2013.07.007
Kigondu, E. V. M., Rukunga, G. M., Keriko, J. M., Tonui, W. K., Gathirwa, J. W., Kirira, P. G., et al. (2009). Anti-parasitic Activity and Cytotoxicity of Selected Medicinal Plants from Kenya. J. Ethnopharmacology 123, 504–509. doi:10.1016/j.jep.2009.02.008
Lawal, H. O., Adewuyi, G. O., Fawehinmi, A. B., and Etatuvie, S. O. (2012). Chemical Evaluation of Mosquito Repellent Formulation Prepared from Essential Oils of Plants. J. Nat. Prod. 6.
Liwa, C. A., and Jaka, H. M. (2016). Renal Diseases and Use of Medicinal Herbal Extracts: A Concise Update of Reported Literature in Africa. J. Nephrol. Ren. Ther. 2. doi:10.24966/nrt-7313/100008
Luyckx, V. A., Ballantine, R., Claeys, M., Cuyckens, F., Van den Heuvel, H., Cimanga, R. K., et al. (2002). Herbal Remedy-Associated Acute Renal Failure Secondary to Cape Aloes. Am. J. Kidney Dis. 39, E13. 503. doi:10.1053/ajkd.2002.31424
Luyckx, V. A., Steenkamp, V., Rubel, J. R., and Stewart, M. J. (2004). Adverse Effects Associated with the Use of South African Traditional Folk Remedies. Cent. Afr. J. Med. 50, 46–51.
Luyckx, V. A. (2012). Nephrotoxicity of Alternative Medicine Practice. Adv. Chronic Kidney Dis. 19, 129–141. doi:10.1053/j.ackd.2012.04.005
Maroyi, A. (2017). Ethnopharmacology and Therapeutic Value of Bridelia Micrantha (Hochst.) Baill. In Tropical Africa: A Comprehensive Review. Molecules 22 (9), 1493. doi:10.3390/molecules22091493
Martin, G. J. (1995). Ethnobotany: A Methods Manual. London: Hall, 510. doi:10.1007/978-1-4615-2496-0Ethnobotany
Mills, E., Cooper, C., and Kanfer, I. (2005). Traditional African Medicine in the Treatment of HIV. Lancet Infect. Dis. 5, 465–467. doi:10.1016/S1473-3099(05)70172-9
Moshi, M. J., van den Beukel, C. J., Hamza, O. J., Mbwambo, Z. H., Nondo, R. O., Masimba, P. J., et al. (2006). Brine Shrimp Toxicity Evaluation of Some Tanzanian Plants Used Traditionally for the Treatment of Fungal Infections. Afr. J. Tradit Complement. Altern. Med. 4, 219–225. doi:10.4314/ajtcam.v4i2.31211
Moshi, M. J., Innocent, E., Magadula, J. J., Otieno, D. F., Weisheit, A., Mbabazi, P. K., et al. (2010). Brine Shrimp of Some Plants Used as Traditional Medicine in Kagera Region, Northest West Tanzania. Tanzan. J. Health Res. 12, 521. doi:10.4314/thrb.v12i1.56287
Mwitari, P. G., Ayeka, P. A., Ondicho, J., Matu, E. N., and Bii, C. C. (2013). Antimicrobial Activity and Probable Mechanisms of Action of Medicinal Plants of Kenya: Withania somnifera, Warbugia ugandensis, Prunus africana and Plectrunthus barbatus. PLoS One 8, 532–e65619. doi:10.1016/j.jep.2004.11.015
Ngulde, S. I., Sandabe, U. K., Abounader, R., Dawson, T. K., Zhang, Y., Iliya, I., et al. (20192019). Ethanol Extract of Securidaca longipedunculata Induces Apoptosis in Brain Tumor (U87) Cells. Biomed. Res. Int. 2019, 9826590. doi:10.1155/2019/9826590
O'Brien, J., Wilson, I., Orton, T., and Pognan, F. (2000). Investigation of the Alamar Blue (Resazurin) Fluorescent Dye for the Assessment of Mammalian Cell Cytotoxicity. Eur. J. Biochem. 267, 5421–5426. doi:10.1046/j.1432-1327.2000.01606.x
Olila, D., Opuda-Asibo, J., and Olwa-Odyek, O. (2001). Bioassay-guided Studies on the Cytotoxic and In Vitro Trypanocidal Activities of a Sesquiterpene (Muzigadial) Derived from a Ugandan Medicinal Plant (Warburgia ugandensis). Afr. Health Sci. 1, 12–15.
Omosa, L. K., Midiwo, J. O., Masila, V. M., Gisacho, B. M., Munayi, R., Francisca-Kamakama, K., et al. (2016). Cytotoxicity of 91 Kenyan Indigenous Medicinal Plants towards Human CCRF-CEM Leukemia Cells. J. Ethnopharmacology 179, 177–196. doi:10.1016/j.jep.2015.12.028
Osebe, T., Mbaria, J., Yole, D., Odongo, D., Nderitu, J., and Ochanda, H. (2016). Bioactivity and Toxicity of Bridelia micrantha, Chenopodium ambrosoides and Ocimum americanum Plant Extracts. Int. J. Basic Clin. Pharmacol. 6, 5. doi:10.18203/2319-2003.ijbcp20164753
Ostrom, Q. T., Gittleman, H., Liao, P., Rouse, C., Chen, Y., Dowling, J., et al. (2014). CBTRUS Statistical Report: Primary Brain and central Nervous System Tumors Diagnosed in the United States in 2007-2011. Neuro Oncol. 16 (Suppl. 4), iv1–63. iv1-63. doi:10.1093/neuonc/nou223
Pollard, S. M., Yoshikawa, K., Clarke, I. D., Danovi, D., Stricker, S., Russell, R., et al. (2009). Glioma Stem Cell Lines Expanded in Adherent Culture Have Tumor-specific Phenotypes and Are Suitable for Chemical and Genetic Screens. Cell Stem Cell 4, 568–580. 567. doi:10.1016/j.stem.2009.03.014
Princen, K., Hatse, S., Vermeire, K., De Clercq, E., and Schols, D. (2004). Establishment of a Novel CCR5 and CXCR4 Expressing CD4+ Cell Line Which Is Highly Sensitive to HIV and Suitable for High-Throughput Evaluation of CCR5 and CXCR4 Antagonists. Retrovirology 1, 2. 571 Retrovirology 1. doi:10.1186/1742-4690-1-2
Riss, T. L., and Moravec, R. A. (2004). Use of Multiple Assay Endpoints to Investigate the Effects of Incubation Time, Dose of Toxin, and Plating Density in Cell-Based Cytotoxicity Assays. ASSAY Drug Development Tech. 2, 51–62. doi:10.1089/154065804322966315
Schoonen, W. G. E. J., Stevenson, J. C. R., Westerink, W. M. A., and Horbach, G. J. (2012). Cytotoxic Effects of 109 Reference Compounds on Rat H4IIE and Human HepG2 Hepatocytes. III: Mechanistic Assays on Oxygen Consumption with MitoXpress and NAD(P)H Production with Alamar Blue. Toxicol. Vitro 26, 511–525. doi:10.1016/j.tiv.2012.01.004
Schultz, F., Anywar, G., Tang, H., Chassagne, F., Lyles, J. T., Garbe, L.-A., et al. (2020b). Targeting ESKAPE Pathogens with Anti-infective Medicinal Plants from the Greater Mpigi Region in Uganda. Sci. Rep. 10, 11935. doi:10.1038/s41598-020-67572-8
Schultz, F., Anywar, G., Wack, B., Quave, C. L., and Garbe, L.-A. 2020a. Ethnobotanical Study of Selected Medicinal Plants Traditionally Used in the Rural Greater Mpigi Region of Uganda. J. Ethnopharmacology 256, 112742. doi:10.1016/j.jep.2020.112742
Steenkamp, V., Mokoele, T. L., and Rensburg, C. E. J. V. (2009). Toxicity Testing of Two Medicinal Plants, Bridelia Micrantha and Antidesma venosum. Totoxij 3, 35–38. doi:10.2174/1874340400903010035
Tran, S. L., Puhar, A., Ngo-Camus, M., and Ramarao, N. (2011). Trypan Blue Dye Enters Viable Cells Incubated with the Pore-Forming Toxin HlyII of Bacillus Cereus. PLoS One 6, e22876. doi:10.1371/journal.pone.0022876
UNAIDS (2020). Country Fact Sheet: Uganda. Available at: https://www.unaids.org/en/regionscountries/countries/uganda.
UNAIDS (2021). Fact Sheet 2021. Preliminary UNAIDS 2021 Epidemiological Estimates. Available at: https://www.unaids.org/sites/default/files/media_asset/UNAIDS_FactSheet_en.pdf.
Wang, C. R., Zhou, R., Ng, T. B., Wong, J. H., Qiao, W. T., and Liu, F. (2014). First Report on Isolation of Methyl Gallate with Antioxidant, Anti-HIV-1 and HIV-1 Enzyme Inhibitory Activities from a Mushroom (Pholiota adiposa). Environ. Toxicol. Pharmacol. 37, 626–637. doi:10.1016/j.etap.2014.01.023
Westermark, B., Pontén, J., and Hugosson, R. (1973). Determinants for the Establishment of Permanent Tissue Culture Lines from Human Gliomas. Acta Pathol. Microbiol. Scand. A. 81, 791–805. doi:10.1111/j.1699-0463.1973.tb03573.x
Keywords: cytotoxicity, glioblastoma cell-line, medicinal plants, herbalists, antiviral, HIV/AIDS, cytotoxic concentration, Uganda
Citation: Anywar GU, Kakudidi E, Oryem-Origa H, Schubert A and Jassoy C (2022) Cytotoxicity of Medicinal Plant Species Used by Traditional Healers in Treating People Suffering From HIV/AIDS in Uganda. Front. Toxicology 4:832780. doi: 10.3389/ftox.2022.832780
Received: 10 December 2021; Accepted: 24 March 2022;
Published: 02 May 2022.
Edited by:
Siddhartha Kumar Mishra, Chhatrapati Shahu Ji Maharaj University, IndiaReviewed by:
Arpita Roy, Sharda University, IndiaCopyright © 2022 Anywar, Kakudidi, Oryem-Origa, Schubert and Jassoy. This is an open-access article distributed under the terms of the Creative Commons Attribution License (CC BY). The use, distribution or reproduction in other forums is permitted, provided the original author(s) and the copyright owner(s) are credited and that the original publication in this journal is cited, in accordance with accepted academic practice. No use, distribution or reproduction is permitted which does not comply with these terms.
*Correspondence: Godwin Upoki Anywar, Z29kd2luYW55d2FyQGdtYWlsLmNvbQ==, Z2FueXdhckBjYXJ0YWZyaWNhLm9yZw==, Z29kd2luLmFueXdhckBtYWsuYWMudWc=
Disclaimer: All claims expressed in this article are solely those of the authors and do not necessarily represent those of their affiliated organizations, or those of the publisher, the editors and the reviewers. Any product that may be evaluated in this article or claim that may be made by its manufacturer is not guaranteed or endorsed by the publisher.
Research integrity at Frontiers
Learn more about the work of our research integrity team to safeguard the quality of each article we publish.