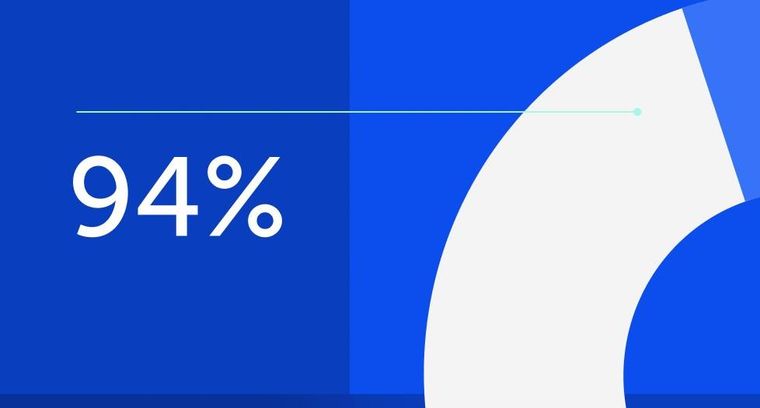
94% of researchers rate our articles as excellent or good
Learn more about the work of our research integrity team to safeguard the quality of each article we publish.
Find out more
EDITORIAL article
Front. Therm. Eng., 24 March 2025
Sec. Heat Transfer Mechanisms and Applications
Volume 5 - 2025 | https://doi.org/10.3389/fther.2025.1591428
This article is part of the Research TopicBio-thermal Medical Devices, Methods, and Models: New Developments and AdvancesView all 5 articles
Editorial on the Research Topic
Bio-thermal medical devices, methods, and models: new developments and advances
Recent advancements in medical imaging techniques have greatly enhanced the ability to capture anatomically precise and highly detailed vascular structures within biological tissues Singh 2024. This progress is particularly significant for bioheat transfer modeling, where an accurate representation of the vascular network is essential for understanding heat exchange, blood perfusion dynamics, and thermal responses in both healthy and pathological conditions. The integration of high-resolution, three-dimensional geometries extracted from medical imaging data, often at the voxel level, enables more precise simulations, improving the predictive accuracy of thermal treatments and physiological responses Singh 2024. More recently, research efforts have continued to develop anatomically accurate models from medical imaging and develop physics and physiology-based models Singh et al., 2024. On contrary, voxel-based domains generated from medical image are crucial for bioheat transfer modeling; however, a key challenge lies in voxel resolution limitations. Due to the small dimensional scale of blood vessels, not all vessels are captured within a given voxel resolution, resulting in discontinuities in vascular segmentation. Also, pre-capillary vessels such as arterioles, which play a critical role in regulating blood flow resistance, are often modeled within the tissue as a porous domain (Xuan and Roetzel, 1997). Such simplification leads to a loss of critical vascular information, potentially affecting the accuracy of bioheat transfer simulations. Additionally, magnetic particle imaging (MPI) has emerged as a powerful tool for tracking magnetic nanoparticles used in hyperthermia-based cancer treatments. By combining mathematical modeling with MPI, researchers are optimizing nanoparticle-induced hyperthermia to improve therapeutic outcomes while minimizing unintended thermal damage to surrounding healthy tissues Singh et al., 2020; Singh et al., 2021; Singh 2023.
In this Research Topic, Pawar et al. conducted a sensitivity analysis to assess the impact of the spatial distribution of magnetic iron oxide nanoparticles (MIONs) on tumor temperature. Their study utilized co-registered magnetic resonance (MR)/computed tomography (CT) imaging alongside magnetic particle imaging (MPI) to derive in vivo MION distribution, which was then compared to mathematically generated uniform and Gaussian distributions. Theoretical predictions were based on the Pennes bioheat transfer equation Pennes 1948, incorporating the dynamic influence of temperature on blood perfusion. To enhance accuracy, they employed a piecewise function to model the degree of vascular stasis (collapse of vasculature), as previously quantified by Singh 2022 in the context of magnetic hyperthermia. This approach provided valuable insights into optimizing MION distribution for more effective magnetic hyperthermia treatments. It should be noted that thermal therapy, commonly known as hyperthermia is not a recently developed method. Its application in tumor treatment dates back at least 4,000 years, with evidence suggesting its use in tumor ablation even earlier Hornback 1989; Glazer and Curley, 2010. One promising thermal therapy technique involves the use of laser energy to selectively induce the “suffocation” of superficial tumors without requiring the insertion of plasmonic nanoparticles directly into human tissues. Instead of directly heating the tumor, this indirect method targets the surrounding biological tissue, causing localized heating that reduces or completely cuts off the oxygen supply to the tumor cells, ultimately leading to their destruction Dombrovsky et al. 2012; Dombrovsky 2022. Pawar et al. mentioned an early version of the energy equation (Pennes bioheat equation) for heat transfer in human tissues. However, this equation does not account for arterial blood cooling and can only be used to estimate volumetric heat transfer in organs containing large blood vessels. A more comprehensive approach to modeling heat transfer in human tissues should be based on two coupled energy equations—one for the biological tissue and another for arterial blood—while account for spatial and temporal variations in arterial blood temperature. A model of this type was discussed in detail in the works of Dombrovsky et al. 2012 and any other modifications to traditional Pennes bioheat equation is summarized in more detail in Bhowmik et al. 2025.
In another article of this Research Topic, Amare et al. highlighted the challenges involved in extracting the small blood vessels due to limited resolution of voxels obtained from image data. Their approach clearly provides evidence that mathematical representations of unsegmented blood vessels can approximate the thermal resistance and reduced the need for high-resolution imaging. In addition, their proposed methodology provides a computationally efficient alternative to high-resolution imaging, making it a valuable tool for future applications in biomedical modeling and thermal therapy planning.
Besides the above numerical work, Pioletti presented an intriguing and innovative perspective on the role of self-heating in soft tissues, specifically in cartilage, because of mechanical stimulation induced heat effect. The core idea discussed in this work is that temperature changes induced by mechanical activity might be necessary for cartilage maintenance−introduces a potential paradigm shift in how we think about the physiological effects of mechanical loading on musculoskeletal tissues.
In addition to the perspective article, Li et al. conducted a bibliometric analysis to assess studies on hypothermia-related injuries, treatment strategies, and underlying mechanisms. This study provides a comprehensive summary of hypothermia’s impact on human health and the therapeutic applications of moderate hypothermia. By mapping research trends, frontiers, and key focus areas, the analysis offers valuable insights into the current landscape and future directions of hypothermia research. Additionally, it highlights the distinctions and interconnections between therapeutic and severe hypothermia, offering a clearer understanding of advancements and emerging trends in the field.
This Research Topic presents a Research Topic of two research articles, a perspective paper, and a review paper, each showcasing novel discoveries, state-of-the-art advancements, and future directions in the interdisciplinary field of computational modeling in biomedical engineering. These studies emphasize multiscale, multiphysics, and medical imaging-assisted approaches, highlighting their integration and applications. We believe that the insights shared in this Research Topic will pave the way for groundbreaking research in bioheat transfer, accelerating innovations in medical device development.
MS: Writing – original draft, Writing – review and editing. AB: Writing – review and editing. RR: Writing – review and editing. KM: Writing – review and editing.
The author(s) declare that no financial support was received for the research and/or publication of this article.
The authors declare that the research was conducted in the absence of any commercial or financial relationships that could be construed as a potential conflict of interest.
The author(s) declared that they were an editorial board member of Frontiers, at the time of submission. This had no impact on the peer review process and the final decision.
The author(s) declare that no Generative AI was used in the creation of this manuscript.
All claims expressed in this article are solely those of the authors and do not necessarily represent those of their affiliated organizations, or those of the publisher, the editors and the reviewers. Any product that may be evaluated in this article, or claim that may be made by its manufacturer, is not guaranteed or endorsed by the publisher.
Bhowmik, A., Singh, M., and Repaka, R. (2025). “Bioheat transfer models: theory and applications in bioengineering,” in Biofluid dynamics of human body systems: expanded and revised edition. Apple Academic Press, 163–277. doi:10.1201/9781003604310
Dombrovsky, L. A. (2022). Laser-induced thermal treatment of superficial human tumors: an advanced heating strategy and non-Arrhenius law for living tissues. Front. Therm. Eng. 1, 807083. doi:10.3389/fther.2021.807083
Dombrovsky, L. A., Timchenko, V., and Jackson, M. (2012). Indirect heating strategy for laser induced hyperthermia: an advanced thermal model. Int. J. Heat. Mass Transf. 55 (17-18), 4688–4700. doi:10.1016/j.ijheatmasstransfer.2012.04.029
Glazer, E. S., and Curley, S. A. (2010). The ongoing history of thermal therapy for cancer. Surg. Oncol. Clin. N. Am. 20 (2), 229–235. doi:10.1016/j.soc.2010.11.001
Hornback, N. B. (1989). Historical aspects of hyperthermia in cancer therapy. Radiol. Clin. N. Am. 27 (3), 481–488. doi:10.1016/S0033-8389(22)02133-9
Pennes, H. H. (1948). Analysis of Tissue and arterial blood Temperatures in the Resting human Forearm. J. Appl. Physiol. 1 (2), 93–122. doi:10.1152/jappl.1948.1.2.93
Singh, M. (2022). Incorporating vascular-stasis based blood perfusion to evaluate the thermal signatures of cell-death using modified Arrhenius equation with regeneration of living tissues during nanoparticle-assisted thermal therapy. Int. Commun. Heat. Mass Transf. 135, 106046. doi:10.1016/j.icheatmasstransfer.2022.106046
Singh, M. (2023). Biological heat and mass transport mechanisms behind nanoparticles migration revealed under microCT image guidance. Int. J. Therm. Sci. 184, 107996. doi:10.1016/j.ijthermalsci.2022.107996
Singh, M. (2024). Modified Pennes bioheat equation with heterogeneous blood perfusion: a newer perspective. Int. J. Heat. Mass Transf. 218, 124698. doi:10.1016/j.ijheatmasstransfer.2023.124698
Singh, M., Gu, Q., Ma, R., and Zhu, L. (2020). Heating protocol design affected by nanoparticle re-distribution and thermal damage model in magnetic nanoparticle hyperthermia for cancer treatment. ASME J. Heat. Transf. 142 (7), 072501. doi:10.1115/1.4046967
Singh, M., Reifman, J., and Rubio, J. E. (2024). A 3-D whole-body human thermoregulatory model to simulate cold-induced vasodilation in the hands and feet. Comput. Biol. Med. 180, 108935. doi:10.1016/j.compbiomed.2024.108935
Singh, M., Soni, S., and Singh, T. (2021). Pre-operative assessment of ablation margins for variable blood perfusion metrics in a magnetic resonance imaging based complex breast tumour anatomy: simulation paradigms in thermal therapies. Comput. Methods Programs Biomed. 198, 105781. doi:10.1016/j.cmpb.2020.105781
Keywords: bioheat and mass transfer, thermal physiology, mathematical modeling, thermal therapy, medical device design, hypothermia, medical image 3D reconstruction
Citation: Singh M, Bhowmik A, Repaka R and Mitra K (2025) Editorial: Bio-thermal medical devices, methods, and models: new developments and advances. Front. Therm. Eng. 5:1591428. doi: 10.3389/fther.2025.1591428
Received: 11 March 2025; Accepted: 19 March 2025;
Published: 24 March 2025.
Edited and reviewed by:
Leonid A. Dombrovsky, Joint Institute for High Temperatures (RAS), RussiaCopyright © 2025 Singh, Bhowmik, Repaka and Mitra. This is an open-access article distributed under the terms of the Creative Commons Attribution License (CC BY). The use, distribution or reproduction in other forums is permitted, provided the original author(s) and the copyright owner(s) are credited and that the original publication in this journal is cited, in accordance with accepted academic practice. No use, distribution or reproduction is permitted which does not comply with these terms.
*Correspondence: Manpreet Singh, bXNpbmdoNkB1bWJjLmVkdQ==; Arka Bhowmik, YXJrYWJob3dtaWtAeWFob28uY28udWs=; Ramjee Repaka, cmFtamVlLnJlcGFrYUBnbWFpbC5jb20=; Kunal Mitra, a21pdHJhQGZpdC5lZHU=
Disclaimer: All claims expressed in this article are solely those of the authors and do not necessarily represent those of their affiliated organizations, or those of the publisher, the editors and the reviewers. Any product that may be evaluated in this article or claim that may be made by its manufacturer is not guaranteed or endorsed by the publisher.
Research integrity at Frontiers
Learn more about the work of our research integrity team to safeguard the quality of each article we publish.