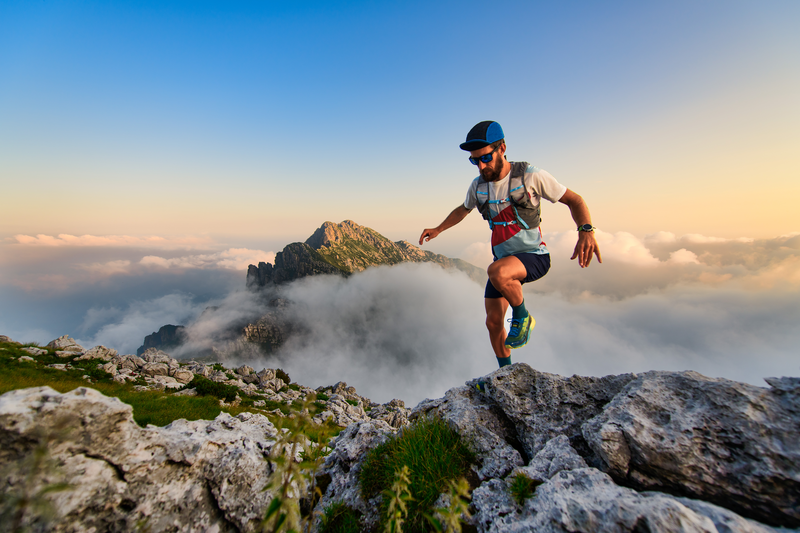
94% of researchers rate our articles as excellent or good
Learn more about the work of our research integrity team to safeguard the quality of each article we publish.
Find out more
ORIGINAL RESEARCH article
Front. Surg. , 21 January 2025
Sec. Neurosurgery
Volume 11 - 2024 | https://doi.org/10.3389/fsurg.2024.1513899
This article is part of the Research Topic Improving neurosurgical precision: leveraging technology for enhanced visualization and navigation of complex neuroanatomy View all 4 articles
Background: External ventricular drain (EVD) placement is a routine neurosurgical procedure used to treat acute hydrocephalus and monitor intracranial pressure. Kocher's point is the most commonly used anatomical landmark, but other entry points can be challenging even for experienced neurosurgeons. Augmented reality (AR) may enhance the accuracy and safety of these procedures. Previous studies demonstrated improved ventriculostomy accuracy using AR among novices. This study evaluates AR's impact on EVD placement accuracy performed by experienced neurosurgeons.
Methods: Eighteen neurosurgical experts performed ventriculostomies on a Styrofoam head model using Kaufman's, Keen's, Kocher's, and Frazier's points. Punctures were performed freehand (Freehand group) and with AR assistance (AR group). Post-procedure CT scans were used to compare the actual catheter tip positions with the ideal positions. Accuracy was assessed by the distance between real and ideal catheter tips and by Kakarla grading.
Results: The AR group had a mean tip distance of 16.93 ± 9.38 mm compared to 21.71 ± 9.69 mm in the Freehand group (p = 0.003). The AR group also showed better Kakarla grading outcomes (Grade 1: n = 26, Grade 3: n = 26) vs. the Freehand group (Grade 1: n = 7, Grade 3: n = 53; p < 0.0001). Neurosurgeons with ≥7 years of experience demonstrated higher accuracy across both methods (p = 0.040).
Conclusion: AR significantly enhances the accuracy of EVD placement, particularly using Kaufman's, Keen's, Kocher's, and Frazier's points, with experienced neurosurgeons benefiting the most from AR assistance.
Placement of external ventricular drainage (EVD) is a basic neurosurgical procedure performed on daily basis. Because of its crucial importance in patient care, the safety and accuracy is of paramount importance (1). In case of procedure-related complications such as misplacement or bleeding, the consequences might offset the benefits and even result in poor long-term prognosis (2). The contemporary standard of EVD placement is placement according to anatomical landmarks (3). In case of free-hand placement, increasing midline shift and left-sided placement of the catheter significantly worsen the accuracy according to Kakarla grade (4, 5). Kocher's point is the standard anatomical landmark used for the EVD placement. However, in case of slit ventricles or atypical ventricle configuration, accuracy decreases even in the hand of more experienced professionals (6). Occasionally (7), other entry points to the ventricles are used in order to safely perform the placement (8). We hypothesized that augmented reality (AR) might be a useful tool to help neurosurgeons identify the correct trajectory and navigate the puncture in order to reach the optimal catheter tip position. To perform the ventriculostomy with neuronavigation, acquisition costs can range from $350,000 up to $690,000. AR-guided placement might be a tool to provide much cheaper and effective solutions if high-end neuronavigation is not available (9). IMAGINER was an experimental study, which proved that neurosurgical-naïve medical students have higher accuracy in catheter placement if AR was used to aid the procedure (8). Based on the results of IMAGINER (10), we designed an experiment which compares the EVD placement performed according to anatomical landmarks (Freehand group) and with the AR (AR group).
We used Styrofoam heads for the experiment. This allowed us to avoid the use of cadavers or an animal model. The target points and optimal trajectories were virtually planned using Brainlab software (Brainlab AG, Munich). The planning was based on cranial magnetic resonance imaging (cMRI) scan of one of the authors (FK). After trajectory-planning was performed, an STL-file was obtained. This original file was then optimized in FREECad 1.0 software and uploaded to the Microsoft Hololens 2 (Microsoft Corporation, Redmond). The virtual model from this planning was then projected onto the Styrofoam heads. For the virtual model projection, the application was developed by our research team. Accurate surface registration was necessary to ensure precise overlay of the virtual model onto the Styrofoam heads. Using the Hololens 2, the manual surface-matching registration was performed with the Styrofoam head, similarly to our previous study IMAGINER (10).
One side of the Styrofoam head was punctured using Microsoft Hololens (AR group), while the other side was punctured without AR assistance (Freehand group). The participants were allowed to choose the sides freely. Entry points for the puncture were anteroposteriorly: Kaufmann's point, Kocher's point, Keen's point and Frazier's point (8). Kocher's point was anatomically defined as 12 cm superior and posterior from the nasion and 2.5–3 cm lateral to midline. Kaufmann's point placed 5 cm superior to the nasion and 3 cm lateral to midline. Keen's point 3 cm superior and posterior to the pinna of the ear, and Frazier's point 6 cm superior to the inion and 3 to 4 cm left or right to the midline (8).
Before the experiment, all neurosurgeons were given access to the MRI scan of the author to enhance their free-hand EVD placement. For AR-guided punctures, manual surface matching was performed using the Styrofoam head. The trajectories and entry points were pre-planned and projected onto the Styrofoam head. For anatomical punctures, neurosurgeons were allowed to reference the anatomical article describing the entry points and trajectories (8) prior to performing the puncture. Additionally, they were permitted to use a tape measure to identify the entry point.
We used steel needles to replace the EVD-catheters to perform the puncture of the Styrofoam model. The needles remained placed in the head for subsequent computed tomography (CT) scans. Before each puncture, the exact depth of needle insertion was determined due to missing haptic feedback. After the puncture was performed, we used plaster to permanently fix the needle in place and to make the face of the Styrofoam head recognizable in the CT scans.
After performing the punctures, all model heads with inserted needles were scanned using CT (Figure 1). The resulting data were segmented using Brainlab software, identifying needles and skin based on grayscale values. The plaster represented the skin, and the needles indicated the actual trajectories.
An expected-actual comparison was conducted using ZEISS Inspect (Carl Zeiss GOM Metrology GmbH, Braunschweig). The virtual planning model (expected) was compared with the segmented Styrofoam model (actual). The evaluated data included the calculated trajectory deviation—distance between the expected and actual points measured in millimeters (mm).
Subsequently, we evaluated each trajectory (see Figure 2) separately according to Kakarla grading as 1-optimal placement, 2- suboptimal placement, in the wall of the ventricle with intraventricular tip, 3-extraventricular placement. For the sake of the analysis, we dichotomized the results as good (Kakarla 1) and suboptimal (Kakarla 2 and 3) (5).
Figure 2. (A) Comparison of distance between catheter tips, (B) Kakarla evaluation of AR-group, (C) Kakarla evaluation of freehand group. This analysis focused on clinical aspect of the placement with main endpoint being the optimal intraventricular placement, ignoring the exact geometrical place of the target point.
Finally, we divided neurosurgeons in experienced (>7 years of experience) and less experienced (≤7 years of experience).
Data were organized and analyzed using SPSS for Windows (version 29.0; IBM Corp., Armonk, NY, USA). We compared the means and standard-deviation of the geometrical placement using student t-test. All values with p < 0.05 were considered significant. For Data visualization, Raincloud-plot was constructed using R version 4.3.1 (R Foundation, Vienna, Austria) including the package ggrain.
18 neurosurgical experts took part in our experiment. Cumulatively, 144 punctures on 18 Styrofoam heads were performed. The participants had free choice of the side to use the AR, however, only one participant decided for the left-sided free-hand catheter placement.
The distance between the real and ideal catheter tip was 16.93 ± 9.38 mm in the AR group, compared to 21.71 ± 9.69 mm in the Freehand group, with a mean difference of 4.77 mm (see Figure 3, p = 0.003).
Figure 3. Rainbow-plot demonstrating the differences between the freehand and AR group based on trajectory deviation (mm).
We subsequently compared all trajectories separately. The only statistically significant difference was observed in Keen's point trajectory (15.29 ± 7.27 mm vs. 23.48 ± 11.22 mm, p < 0.01). Punctions over Kocher's point showed no significant difference (16.33 ± 7.33 mm vs. 20.60 ± 6.88 mm, p < 0.08). All other trajectories showed no significant difference between AR group and Freehand group. For detailed information, see Table 1.
AR group showed significantly better results compared to Freehand group (p = 0.00001). After dividing the results in good (Kakarla = 1) and suboptimal (Kakarla = 2 and 3), AR group also shows superior results compared to Freehand group (Table 2, p = 0.0027).
We divided the trajectories in those performed by more experienced neurosurgeons (>7 years of experience) and less experienced neurosurgeons (≤7 years of experience). Both groups showed better results with AR (>7 years of experience, p < 0.0001, ≤7 years of experience p = 0.005).
Comparing all the trajectories cumulatively according to the experience, the experienced neurosurgeons performed better (p = 0.040). Table 3 summarizes the results.
We performed an experiment evaluating Ventriculostomies on Styrofoam head performed by 18 neurosurgical experts using Kaufman's, Keen's, Kocher's and Frazier's point as entry point. We observed higher accuracy according to both trajectory deviation and Kakarla grading, if augmented reality was used.
We compared a ventriculostomy solely by neurosurgical experts. Because of the freedom to choose the side of the puncture, we have gathered very reliable data, as the majority preferred the right-sided placement of the needle. According to the published data, years of experience do impact the overall accuracy of the puncture. According to O'Neil et al. (11), senior residents and experienced neurosurgeons require less attempts in order to successfully place the catheter. Our data support the thesis that experienced surgeons not only perform better in overall evaluations regardless of the technique used but also benefit more significantly from AR support compared to their less experienced colleagues, particularly in achieving clinically optimal placement (Kakarla Grade 1). Specifically, experienced neurosurgeons achieved Kakarla Grade 1 placement in 21 cases (AR: n = 17; Freehand: n = 4), whereas less-experienced neurosurgeons achieved this in 12 cases (AR: n = 9; Freehand: n = 3).
As experience poses a significant limitation to the experiment, it is important to note that Kocher's point is the preferred entry point in our department. Additionally, two neurosurgeons with prior pediatric experience routinely use Frazier's point.
The Styrofoam head models offered several advantages. They did not cause any CT-artefacts and have no restriction in availability compared to animal models or cadaveric heads. Furthermore, the material is easy to puncture and provides simultaneously sufficient support for the needle so that it does not accidentally dislocate.
Several disadvantages of the model presented themselves in the course of the experiment. The missing haptic feedback of ventricle puncture might have been a strong limitation (12). In case of successful ventricular puncture, a loss of resistance followed by CSF flow is a sign of success (13). Styrofoam head does not offer this feedback. If loss of resistance and CSF flow are missing in real ventriculostomies, it usually triggers a new puncture under alternated trajectory. This fact cannot be reflected in our experiment, as it only offers one single try without the possibility of correcting the needle trajectory.
We observed a strong preference among neurosurgeons to right-sided anatomical placement of the catheter. This reflects the daily clinical practice, as the right-sided puncture is often the preferred approach (14). This preference should theoretically strengthen the results and supports higher accuracy in the Freehand group. However, we noted a very high number of Kakarla Grade 3 catheter tips. This suggests, that despite of the complexity and obvious disadvantage of left-sided puncture, AR guided catheter placement may be superior to Freehand group, even in hands of experienced professionals (15, 16).
We performed two types of evaluation; trajectory deviation and target point evaluation according to Kakarla grading. Both have some pros and cons.
Trajectory deviation offers a comparison with ideal trajectory as planned preoperatively. This offers an exact comparison of catheter tip distance to preoperatively planned target point. However, it misses the clinical critically important information—the position of the catheter according to the ventricular system.
Evaluation according to Kakarla scale (5) presents clinically valuable evaluation of actual catheter tip placement in relation to the ventricle. On the other hand, it omits the geometry and does not evaluate the deviation from the ideal trajectory.
Several possibilities to navigate the EVD placement have already been analyzed (17). The complexity of use and mainly retrospective, single center reports with strongly biased data limit the routine use of this navigation techniques. Under such circumstances, a simple AR-based tools might offer the most straightforward solution.
The experiment-design had several limitations. The absence of haptic feedback and CSF flow, which in real-life puncture would prompt the neurosurgeon to correct the trajectory and perform a new puncture, likely contributed to higher rates of Kakarla Grade 3 (intraparenchymal) placements. In real-life scenarios, the missing CSF flow and loss of resistance usually indicate a suboptimal catheter tip position, triggering a new attempt with a corrected trajectory. The second factor contributing to high grade of Kakarla 3 rate is the fact that a model MRI used for the experiment design was performed on young individual without any sign of hydrocephalus and radiologically relatively slit ventricle-system. For the detailed image of the MRI, please see the Supplementary File.
Our study experimentally demonstrates higher accuracy in EVD placement using Kaufman's, Keen's, Kocher's and Frazier's points when AR was used. However, despite good results, we still see a high number of missed attempts in both groups. In the future, further development and simplification of the tools might play a crucial role in order to minimize missed target points.
The original contributions presented in the study are included in the article/Supplementary Material, further inquiries can be directed to the corresponding author.
MV: Conceptualization, Data curation, Formal Analysis, Investigation, Methodology, Writing – original draft, Writing – review & editing. FK: Methodology, Project administration, Resources, Software, Writing – original draft. DW: Supervision, Writing – review & editing. EG: Supervision, Writing – review & editing. RG: Conceptualization, Methodology, Software, Supervision, Writing – review & editing. JW: Conceptualization, Formal Analysis, Investigation, Methodology, Supervision, Visualization, Writing – original draft, Writing – review & editing.
The author(s) declare that no financial support was received for the research, authorship, and/or publication of this article.
The graphical abstract of the present manuscript was created with BioRender.com.
The authors declare that the research was conducted in the absence of any commercial or financial relationships that could be construed as a potential conflict of interest.
The author(s) declare that no Generative AI was used in the creation of this manuscript.
All claims expressed in this article are solely those of the authors and do not necessarily represent those of their affiliated organizations, or those of the publisher, the editors and the reviewers. Any product that may be evaluated in this article, or claim that may be made by its manufacturer, is not guaranteed or endorsed by the publisher.
The Supplementary Material for this article can be found online at: https://www.frontiersin.org/articles/10.3389/fsurg.2024.1513899/full#supplementary-material
1. Todnem N, Nguyen KD, Reddy V, Grogan D, Waitt T, Alleyne CH. A simple and cost-effective model for ventricular catheter placement training: technical note. J Neurosurg. (2020) 134(5):1640–3. doi: 10.3171/2020.2.JNS19161
2. Fried HI, Nathan BR, Rowe AS, Zabramski JM, Andaluz N, Bhimraj A, et al. The insertion and management of external ventricular drains: an evidence-based consensus statement: a statement for healthcare professionals from the neurocritical care society. Neurocrit Care. (2016) 24(1):61–81. doi: 10.1007/s12028-015-0224-8
3. Brenke C, Fürst J, Katsigiannis S, Carolus AE. High accuracy of external ventricular drainage placement using anatomical landmarks. Neurochirurgie. (2020) 66(6):435–41. doi: 10.1016/j.neuchi.2020.09.009
4. Maher Hulou M, Maglinger B, McLouth CJ, Reusche CM, Fraser JF. Freehand frontal external ventricular drain (EVD) placement. Accuracy and complications. J Clin Neurosci. (2022) 97:S7–11. doi: 10.1016/j.jocn.2021.12.036
5. Kakarla UK, Kim LJ, Chang SW, Theodore N, Spetzler RF. Safety and accuracy of bedside external ventricular drain placement. Neurosurgery. (2008) 63(1 Suppl 1):ONS162–6; discussion ONS166–7. doi: 10.1227/01.neu.0000335031.23521.d0
6. Dossani RH, Patra DP, Terrell DL, Willis B. Placement of an external ventricular drain. N Engl J Med. (2021) 384(2):e3. doi: 10.1056/NEJMvcm1805314
7. Ashir Shafique M, Saqlain Mustafa M, Haseeb A, Mussarat A, Arham Siddiq M, Faheem Iqbal M, et al. Trapped temporal horn: from theory to practice, a systematic review of current understanding and future perspectives. World Neurosurg X. (2024) 23:100345. doi: 10.1016/j.wnsx.2024.100345
8. Morone PJ, Dewan MC, Zuckerman SL, Tubbs RS, Singer RJ. Craniometrics and ventricular access: a review of Kocher’s, Kaufman’s, Paine’s, Menovksy’s, Tubbs’, Keen’s, Frazier’s, Dandy’s, and Sanchez’s points. Oper Neurosurg. (2020) 18(5):461–9. doi: 10.1093/ons/opz194
9. Grunert R, Winkler D, Wach J, Kropla F, Scholz S, Vychopen M, et al. IMAGINER: improving accuracy with a mixed reality navigation system during placement of external ventricular drains. A feasibility study. Neurosurg Focus. (2024) 56(1):E8. doi: 10.3171/2023.10.FOCUS23554
10. Rossi VJ, Wells-Quinn TA, Malham GM. Negotiating for new technologies: guidelines for the procurement of assistive technologies in spinal surgery: a narrative review. J Spine Surg. (2022) 8(2):254–65. doi: 10.21037/jss-21-107
11. O'Neill BR, Velez DA, Braxton EE, Whiting D, Oh MY. A survey of ventriculostomy and intracranial pressure monitor placement practices. Surg Neurol. (2008) 70(3):268–73; discussion 273. doi: 10.1016/j.surneu.2007.05.007
12. Pollock JR, Hayward RD. Adverse operative events in neurosurgical training: incidence, trends and proposals for prevention. Br J Neurosurg. (2001) 15(4):312–8. doi: 10.1080/02688690120072450
13. Abinaya P, Manivannan M. Haptic based fundamentals of laparoscopic surgery simulation for training with objective assessments. Front Robot AI. (2024) 11:1363952. doi: 10.3389/frobt.2024.1363952
14. Pulcinelli M, Antoni F D, Presti DL, Schena E, Carassiti M, De Tommasi F, et al. Combining fiber bragg grating and artificial intelligence technologies for supporting epidural procedures. IEEE Trans Biomed Eng. (2024) 71(11):3213–20. doi: 10.1109/TBME.2024.3412215
15. Becker A, Kuhnt D, Bakowsky U, Nimsky C. Contrast-enhanced ultrasound ventriculography. Neurosurgery. (2012) 71(2 Suppl Operative):ons296–301; discussion ons301. doi: 10.1227/NEU.0b013e31826a8a97
16. Mortazavi MM, Adeeb N, Griessenauer CJ, Sheikh H, Shahidi S, Tubbs RI, et al. The ventricular system of the brain: a comprehensive review of its history, anatomy, histology, embryology, and surgical considerations. Childs Nerv Syst. (2014) 30(1):19–35. doi: 10.1007/s00381-013-2321-3
Keywords: external ventricular drain, augmented reality, Kaufman's point, Keen's point, Kocher's point, Frazier's points
Citation: Vychopen M, Kropla F, Winkler D, Güresir E, Grunert R and Wach J (2025) IMAGINER 2—improving accuracy with augmented realIty navigation system during placement of external ventricular drains over Kaufman's, Keen's, Kocher's and Frazier's point. Front. Surg. 11:1513899. doi: 10.3389/fsurg.2024.1513899
Received: 19 October 2024; Accepted: 26 December 2024;
Published: 21 January 2025.
Edited by:
Aristotelis Kalyvas, University of Toronto, CanadaReviewed by:
Nicole A Terpolilli, Ludwig Maximilian University of Munich, GermanyCopyright: © 2025 Vychopen, Kropla, Winkler, Güresir, Grunert and Wach. This is an open-access article distributed under the terms of the Creative Commons Attribution License (CC BY). The use, distribution or reproduction in other forums is permitted, provided the original author(s) and the copyright owner(s) are credited and that the original publication in this journal is cited, in accordance with accepted academic practice. No use, distribution or reproduction is permitted which does not comply with these terms.
*Correspondence: Martin Vychopen, bWFydGluLnZ5Y2hvcGVuQG1lZGl6aW4udW5pLWxlaXB6aWcuZGU=
†These authors have contributed equally to this work
Disclaimer: All claims expressed in this article are solely those of the authors and do not necessarily represent those of their affiliated organizations, or those of the publisher, the editors and the reviewers. Any product that may be evaluated in this article or claim that may be made by its manufacturer is not guaranteed or endorsed by the publisher.
Research integrity at Frontiers
Learn more about the work of our research integrity team to safeguard the quality of each article we publish.