- 1Sidney Kimmel Medical College, Thomas Jefferson University, Philadelphia, PA, United States
- 2School of Medicine, Georgetown University, Washington, DC, United States
- 3Department of Neurosurgery, Perelman School of Medicine, University of Pennsylvania, Philadelphia, PA, United States
- 4Department of Radiology, Perelman School of Medicine, University of Pennsylvania, Philadelphia, PA, United States
Background: Extended reality (XR) includes augmented reality (AR), virtual reality (VR), and mixed reality (MR). Endovascular neurosurgery is uniquely positioned to benefit from XR due to the complexity of cerebrovascular imaging. Given the different XR modalities available, as well as unclear clinical utility and technical capabilities, we clarify opportunities and obstacles for XR in training vascular neurosurgeons.
Methods: A systematic review following the Preferred Reporting Items for Systematic Reviews and Meta-Analyses (PRISMA) guidelines was conducted. Studies were critically appraised using ROBINS-I.
Results: 19 studies were identified. 13 studies used VR, while 3 studies used MR, and 3 studies used AR. Regarding specific educational applications, VR was used for simulation in 10 studies and anatomical modeling in 3 studies. AR was only used for live intra-operative guidance (n = 3 studies). MR was only used for modeling and intra-operative teaching. Considering disease-specific uses, XR enhanced trainee understanding of intracranial aneurysms (n = 12 studies) and stroke (n = 7). XR trained surgeons in diverse neurosurgical procedures, including aneurysm coiling (n = 5 studies), diagnostic angiography (n = 5), and thrombectomy (n = 5).
Conclusions: Anatomical modeling with VR and MR enhances neurovascular anatomy education with patient-specific, 3-D models from imaging data. AR and MR enable live intra-operative guidance, allowing experienced surgeons to remotely instruct novices, potentially improving patient care and reducing geographic disparities. AR overlays enhance instruction by allowing the surgeon to highlight key procedural aspects during training. Inaccurate tracking of surgical tools is an XR technological barrier for modeling and intra-operative training. Importantly, the most reported application of XR is VR for simulation–using platforms like the Mentice VIST and Angio Mentor. 10 studies examine VR for simulation, showing enhanced procedural performance and reduced fluoroscopy use after short training, although long-term outcomes have not been reported. Early-stage trainees benefited the most. Simulation improved collaboration between neurosurgeons and the rest of the surgical team, a promising role in interprofessional teamwork. Given the strength of VR for simulation, MR for simulation is an important gap in the literature for future studies. In conclusion, XR holds promise for transforming neurosurgical education and practice for simulation, but technological research is needed in modeling and intra-procedural training.
1 Background
Over the past century, the expansion of neurosurgical approaches has been driven by advancements in imaging technology. Because fluoroscopic imaging in endovascular procedures offers only biplane views, vascular neurosurgery is uniquely positioned to benefit greatly from advanced imaging projections. For example, augmented fluoroscopy—which combines 3-D rotational angiography with 2-D fluoroscopy—has greatly improved endovascular neurosurgery. This progress highlights the potential advantages of further advancements in imaging technology, such as extended reality. Extended reality (XR) is an umbrella term for mixed reality (MR), virtual reality (VR), and augmented reality (AR); it has already found applications across an array of fields, including education, military training, and surgery (1–4).
XR technologies have distinct mechanisms: VR constructs entirely computer-generated environments that change in real-time with input from the surgeon and the physical environment. Instead of projections in a computer-generated environment, AR overlays virtual objects (like 3-D representations of neurovasculature) onto the surgeon's real-world environment. In contrast, MR builds upon AR by allowing physical and virtual elements to interact for example, a surgeon manipulating a 3-D brain model on a table.
What utility might XR provide for neurosurgical training? In a survey of 233 neurosurgeons from 38 countries, 67.4% had simulator access as a trainee, but only 15.3% used it frequently. Notably, the survey responses indicate that the realism and convenience of an improved virtual simulator would increase usage–something newer XR technology makes possible (5, 6). The proposed utility of XR for neurosurgery is broad: one review coins the term “future surgery” to describe the magnitude of XR's impact on a new era of neurosurgery (7, 8). First, XR can create immersive, risk-free environments where trainees can practice complex procedures, improving their skills and confidence before performing on patients. Similarly, XR can also potentially curb expenses and waste in surgical training by offering an alternative to costly simulation environments–reducing the use of cadavers or animal models. Moreover, AR, VR, and MR can provide an enhanced educational experience by improved anatomy visualizations (9, 10). It has been suggested that XR could be useful for tele-proctoring for attendings in remote areas that are not comfortable with advanced vascular procedures, but are the only surgeons available (11, 12). However, while these applications have been proposed, these commentaries lack data-driven evidence that accurately assesses the current state of XR. In a strong state-of-the-art published in Frontiers in Surgery, Cannizzaro et al. discuss the great promise that AR holds for neurosurgery. While an extremely robust review, several questions remain unanswered after their work 3 years ago. First, their review only assesses AR, not VR or MR. In addition, their broad approach to general neurosurgery is limited in its ability to provide a detailed analysis of the implications of specific technological advances; this lack of detail is partially because they examine all of neurosurgery, while we will focus on endovascular neurosurgery (13). Given the diversity of neurosurgical procedures, focusing on a subspecialty allows for a standardized collection of outcomes. This focused methodology has been applied to the subspecialty of spinal neurosurgery, but the devices examined in the literature are used for screw placement and discectomy and have little overlap with the devices used in vascular neurosurgery, such as catheters and guidewires (14). Most importantly, among all neurosurgical subspecialties, vascular neurosurgery stands out with the most comprehensive reports in XR, showing significant potential for a systematic review (15).
Here, we aim to provide an unprecedented, detailed, analysis with specific attention to key technical advancements and novel clinical applications of XR for endovascular neurosurgery training. By doing so, we will highlight areas that warrant further study and key strengths to capitalize on, to ultimately facilitate the future integration of AR, VR, and MR into the future of neurosurgery education.
2 Materials and methods
This systematic review complied with the Preferred Reporting Items for Systematic Reviews and Meta-Analyses (PRISMA) guidelines (16). The five research databases for this review were PubMed, Scopus, Ovid, EMBASE, and Clarivate Web of Science. The search queries were crafted to cover a comprehensive range of studies related to the use of AR, MR and VR in endovascular neurosurgery. As an example query, we searched PubMed with the following terms: ((vascular neurosurgery[Title/Abstract]) OR (neurovascular[Title/Abstract])) OR (neuro-vascular[Title/Abstract]) OR (neuro-endovascular[Title/Abstract]) OR (interventional neurorad*[Title/Abstract]) OR (neurointervention*[Title/Abstract]) OR (neuro-intervention*[Title/Abstract]) OR (neuro intervention*[Title/Abstract])) AND ((augmented reality[Title/Abstract]) OR (AR[Title/Abstract]) OR (virtual reality[Title/Abstract]) OR (VR[Title/Abstract])). The queries were syntactically modified for the other four databases (Supplementary Data S1). To make sure we did not miss any cutting-edge, recently published studies, our search was last repeated in April 2024.
Next, the titles and abstracts retrieved from these databases were screened for relevance to the research question. Then, articles with ambiguous relevance based on their titles and abstracts were scrutinized further by full text examination. Studies were excluded if they did not mention endovascular neurosurgery, were unrelated to the research question, or were not written in English. Two independent reviewers (S.A.P., M.C.), did all the screening with a third reviewer (S.P.) to resolve conflicts. Reviews and commentaries were excluded so that only papers reporting defined outcomes would be included.
Data was systematically extracted from each selected study, capturing key information such as the type of technology (AR, MR, or VR), its specific application in endovascular neurosurgery, pathologies examined, procedures trained, the experience and education of trainees taught in each study, and the main outcomes and limitations reported. This structured data extraction process facilitated the synthesis of summary figures and enabled a comprehensive comparison of the studies included in the review.
ROBINS-I was used to critically appraise the studies for bias across 7 categories: confounding, selection bias, bias in the classification of interventions, bias due to deviations from the intended interventions, bias due to missing data, bias in the measurement of outcomes, and bias in the selection of reported results (17).
3 Results
The search strategy identified 408 unique publications, 85 of which underwent full-text review. 19 studies from 2001 to 2023 were included in the final analysis (Figure 1). 13 studies used VR, while 3 studies used MR, and 3 used AR (Table 1). AR was only used for live intra-operative training. MR was only used for live intra-operative training or modeling. VR was used for modeling or simulation (Figure 2). Intracranial aneurysm was the most common pathology that XR was used to teach, followed by stroke (Figure 3A). Coiling (n = 5 studies), diagnostic angiography (n = 5) and thrombectomy were the most common applications of XR to procedural training (Figure 3B).
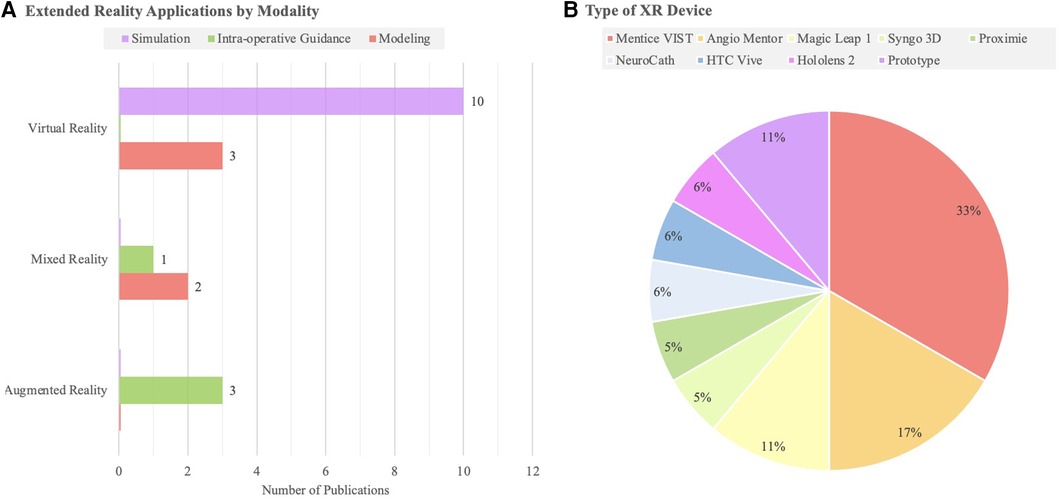
Figure 2. (A) A bar chart highlighting the number of studies of each extended reality modality (AR, MR, VR) by educational domain. (B) Types of XR devices reported in each study identified.
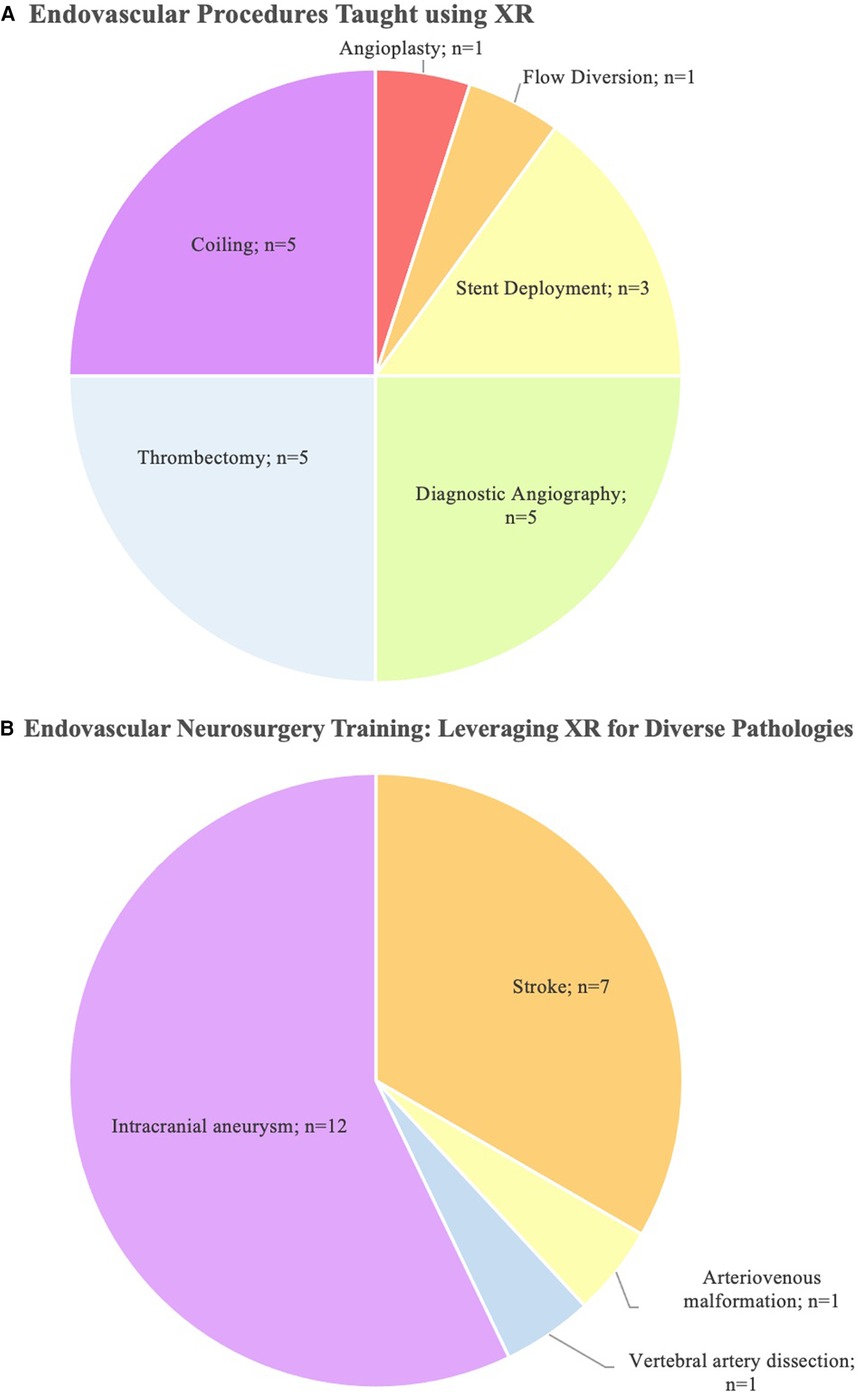
Figure 3. (A) A pie chart highlighting the procedural applications of XR in the literature and (B) pathologies that were taught with the use of XR. Note that some studies examine multiple procedures or pathologies.
4 Discussion
This systematic review synthesizes the current evidence regarding XR in vascular neurosurgery. We identify several domains of XR technology used to train vascular neurosurgeons across various conditions. In addition, we discuss the opportunities available for clinicians, engineers, and innovators interested in advancing neurosurgical education.
4.1 Modeling with XR
XR can be used to generate models of neurovasculature. Interactive modeling is particularly strong at introducing novice surgeons and medical students to neurovascular anatomy. Here, XR models can be generated based on anatomical diagrams, as a supplement–or replacement–to textbooks and cadavers. Many medical schools already use VR or AR to teach anatomy–including but not limited to neuroanatomy–which was particularly useful for remote education during the COVID-19 pandemic (34, 35). While this is well-established, our review demonstrates that XR shows promise not just for early-stage trainees, but also for advanced surgeons. In 2013, a commentary discussed the advantages of augmented fluoroscopy over 2-D biplane angiography. It paves the way for AR in 3-D intra-operative navigation, aiding surgeons in identifying crucial functional brain areas (36). Rather than modeling pre-programmed anatomy, custom XR models can be generated based on a patient's individual anatomy–highlighting morphological differences and anatomical variations. Preoperative planning remains a cornerstone of successful surgical intervention in endovascular neurosurgery. Surgeons can now create patient-specific, three-dimensional models derived from high-resolution imaging modalities, which can be examined and manipulated in XR environments. While pre-programmed models could help medical students develop a general, foundational, anatomical understanding, customizable models help advanced surgeons teach surgical approaches on patients with more complex anatomy. In a comparison of 3-D VR constructions of aneurysms with traditional CTA, 10 neurosurgeons were shown a CTA, provided an assessment and were then shown a 3-D VR construction of the patient's anatomy and asked to reassess the case. In the 26 patients examined, this modality significantly influenced detection of the vascular structure around the aneurysm, ideal head positioning, and neurosurgical approach (37). In 2018, using the HoloLens2 (Microsoft Corporation, Washington, United States), MR was shown to avoid the need for multiple fluoroscopy images by generating a volumetric representation of the vasculature the neurosurgeon can rotate. The device is designed such that the color of the text of the C-arm angle changes based on whether the angle is achievable by the gantry. This interface that can help trainees understand how to approach procedures. In addition, the neurosurgeon can manipulate the system through either hand gestures, eye gaze or voice control (24).
However, there are some technological limitations to customizable models. In 2004, a novel method was developed for translation of biplane angiography into personalized VR 3-D models of patient neurovasculature. While the authors hoped to apply the technology for endovascular neurosurgery in stroke, the VR system still requires up to 6 h to generate a model (33). Recently in 2023, VR was used to assist in stent deployment for anterior circulation aneurysms of 27 patients. While the virtual stent underestimated the length and diameter of the stent, the authors anticipate its application for pre-operative planning with further technological advancement (18). Another study describes the experience using the MxR Magic Leap One (Magic Leap, Florida, United States) for the pre-procedural planning of neurosurgical aneurysm treatment. Similar to the HoloLens 2, hand gestures were used to examine the aneurysm and surrounding neurovasculature; this intuitive user interface highlights an advantage of MR over VR and AR (4).
Customizable AR and VR models have shown clinical promise in small studies. Great progress has been made with the technological capabilities themselves, patient specific models need to be revisited. Future studies should investigate outcomes prospectively and with larger sample sizes. Neurosurgical innovators should also focus on using MR technology over VR and AR for pre-operative planning, due to strengths inherent to the technology's interface.
4.2 Live intra-operative and tele-education
In addition to teaching anatomy and explaining cases, we uncover a second key educational role of XR: live intra-operative guidance, where neurosurgeons guide trainees while performing a procedure. Overlays can help a surgeon understand how to approach a procedure–such as the gantry angle feature of the HoloLens 2 described previously (24). In addition to overlays, an important use case of XR is live tele-proctoring, where an experienced specialist can educate a less-experienced surgeon in a remote area on performing a procedure.
Proximie (Proximie, United Kingdom), is an AR platform enabling users to intra-operatively guide trainees. In a series of 10 cases, an attending surgeon used the device to communicate with neurosurgical fellows and provide immediate feedback. In this setting, intra-operative imaging and a video feed of the operating fellow were live-streamed to an attending surgeon in a different geographic location. In addition to a video feed, the surgeon also used AR illustrations to communicate with the trainee in the angiography suite. No complications or deaths were recorded across a variety of procedures, including diagnostic angiograms, balloon angioplasty, and stent deployment. While fluoroscopy time and contrast use were lower compared to in-person supervision, the difference was not statistically significant (12, 19). In Woven EndoBridge device deployment, another AR device called Magic Leap-1 (Magic Leap Incorporated, Florida, United States) guided a trainee over either a cellular data hotspot or a Wi-Fi-based network (11). The minimal latency observed in the study shows promise, especially for education in remote areas or low-income settings with less stable connections to the Internet. XR may enhance surgical training across diverse geographic regions, enhancing collaboration and reducing disparities due to lack of training. However, these resource-limited settings often have little access to novel technologies, and efforts must be made to ensure this is not a barrier as well. However, further studies with much larger sample sizes are needed to demonstrate the long-term benefits of XR for intra-operative neurosurgical education.
Importantly, XR should allow the supervising surgeon to clearly see and understand the actions of the operating trainee, even from a remote location, so advancements in intra-operative education have emphasized improved monitoring technology. A team at Notre Dame Hospital in Montreal, Canada, developed an algorithm for guidewire tracking in endovascular neurosurgery. In this process, the guidewire needs to be manually segmented on a still image before separate algorithms track the lateral and anteroposterior movement. Notably, they achieve a significantly lower error for guidewire tracking than previous papers, and perform the first testing for neurosurgical interventions (32, 38). Gao et al. have proposed a VR system that not only accounts for the displacement of the catheter as it inserts/retracts and rotates but also has haptic feedback when the catheter contacts a blood vessel wall, something a separate commentary from neurosurgeons in 2013 called for (15, 39). Haptic feedback, where touch and physical pressure are electronically transmitted, allows for a more intuitive experience for the surgeon. By augmenting XR with the sensation of touch, technical advancements in endovascular tool-artery interaction paved the way for haptic feedback technology in neurointervention. In 2007, an endovascular neurosurgery-specific catheter-artery interaction database was reported, pioneering haptic feedback technology (40). Additionally, a VR device has been developed called the NeuroCath (Neuroradiology Catheterization Simulator, Poland) that provides 3-D fluoroscopic as well as haptic feedback (8, 9, 41). In addition to haptic feedback, another key augmentation is multiple visualization modes; a team at Johns Hopkins Hospital report how the HTC Vive (New Taipei City, Taiwan) provided an opaque display mode that was helpful to evaluate morphological parameters, while a translucent projection was useful when evaluating inflow and outflow tracts or visualizing overlapping anatomical structures (28).
Clinical studies examining XR in live education are promising and show strong technological foundations. Further study is warranted because of a large potential to improve the quality of neurosurgical education globally and reduce disparities due to poor training/clinical exposure. Notably, clinical studies on existing devices all examine only AR, while designs focus on VR devices–an important niche for future MR research to fill (Figure 2). We note that all studies examining live intra-operative education have an experienced surgeon use XR to guide an inexperienced one. However, we propose that XR technology could also be used in the opposite way: to have novice surgeons to virtually observe more experienced surgeons. Operative videos are popular in leading neurosurgical journals, but XR technology may provide a superior, more immersive, experience than videos. Recently, a team at Mount Sinai Hospitals in New York published a VR arteriovenous malformation resection (42). We hope to see further clinical studies comparing operative videos with XR experiences in the coming years.
4.3 Neurosurgical simulation
Lastly, we address the use of XR in simulations and whether it provides a promising educational approach that improves surgical performance. Several reviews and commentaries discuss using XR to teach neurosurgical procedures through simulation (3, 6, 43–47).
The most frequently identified platform to aid in neurosurgical simulation was the Mentice Vascular Intervention Simulation Trainer (Figure 2). The Mentice Vascular Intervention Simulation Trainer (VIST; Mentice, Sweden) is a metric-based VR simulator that is able to accept real materials, including catheters, coils, and stents, to assess trainee skills and rehearse procedures (31). Neuroradiologists who trained with the Mentice VIST improved operative speed and reduced contrast use in the simulation of cerebral aneurysm embolization (30). Kreiser et al. found that the Mentice VIST decreased total procedure duration and fluoroscopy time during diagnostic angiography simulations, but only in inexperienced beginner surgeons (22). In contrast, among interventional neuroradiology attendings and residents in mechanical thrombectomy simulations, utilizing the Mentice VIST improved handling errors, reduced contrast volume, and reduced fluoroscopy time. Each neuroradiologist in this study reported positive attitudes toward the simulator (20, 21).
In addition to the Mentice VIST, the Angio Mentor (Simbionix Surgical Science, Sweden), a commercially available simulation learning platform, improves a mentor's ability to provide haptic feedback following simulated endovascular procedures with catheter-based systems and enhances trainees' understanding of cerebrovascular anatomy (23). In endovascular neurosurgery trainees, the Angio Mentor has decreased time to every procedural benchmark for simulated revascularization of a middle cerebral artery thrombosis (25). This platform has demonstrated similar results in diagnostic cerebral angiogram simulations (5). Simulations using the Magic Leap 1 and Snygo 3-D showed educational benefits when simulations were performed across a variety of device types, including the Woven EndoBridge (MicroVention, California, USA), flow diverter P64, the flow diverter P48 (Phenox, Germany), and the pipeline embolization device (Medtronic, California, USA) (11, 18).
Unexpectedly, XR in endovascular neurosurgery was useful to non-surgical specialties as well. A team of vascular surgery, anesthesiology, and radiology team members were led by a neurosurgeon in drafting carotid artery stenting protocols. These protocols were tested in a VR environment, and participants reported an increased understanding of what to do during stent deployment, and another similar study reported near-perfect survey scores from participants in the categories of realism, technical issues, teamwork, and communication (27, 48).
VR technology from ImmersiveTouch (Illinois, USA) has shown promise in ventriculostomy, lumbar puncture, and trigeminal rhizotomy simulations, but have not published results on vascular neurosurgical procedures. Notably, the team behind this project has received almost half a million dollars in grant funding for their work examining a VR haptic model of an MCA bifurcation aneurysm from the United States National Institutes of Health. This information is publicly available to anyone by accessing the National Institutes of Health grant reporter page (49).
Despite the apparent benefits of augmented reality (AR) and virtual reality (VR) in endovascular neurosurgery, a detailed review and formal risk-of-bias assessment using ROBINS-I revealed several opportunities for growth in the current evidence base (Figure 4). There is substantial heterogeneity in the XR outcome measures reported, which range from subjective user satisfaction scores to objective measures of task performance. Most importantly, we identify a gap in the literature on long-term outcomes, limiting our understanding of the lasting impact and retention of skills or benefits imparted by XR. In addition, whether this technology is cost-effective should be examined.
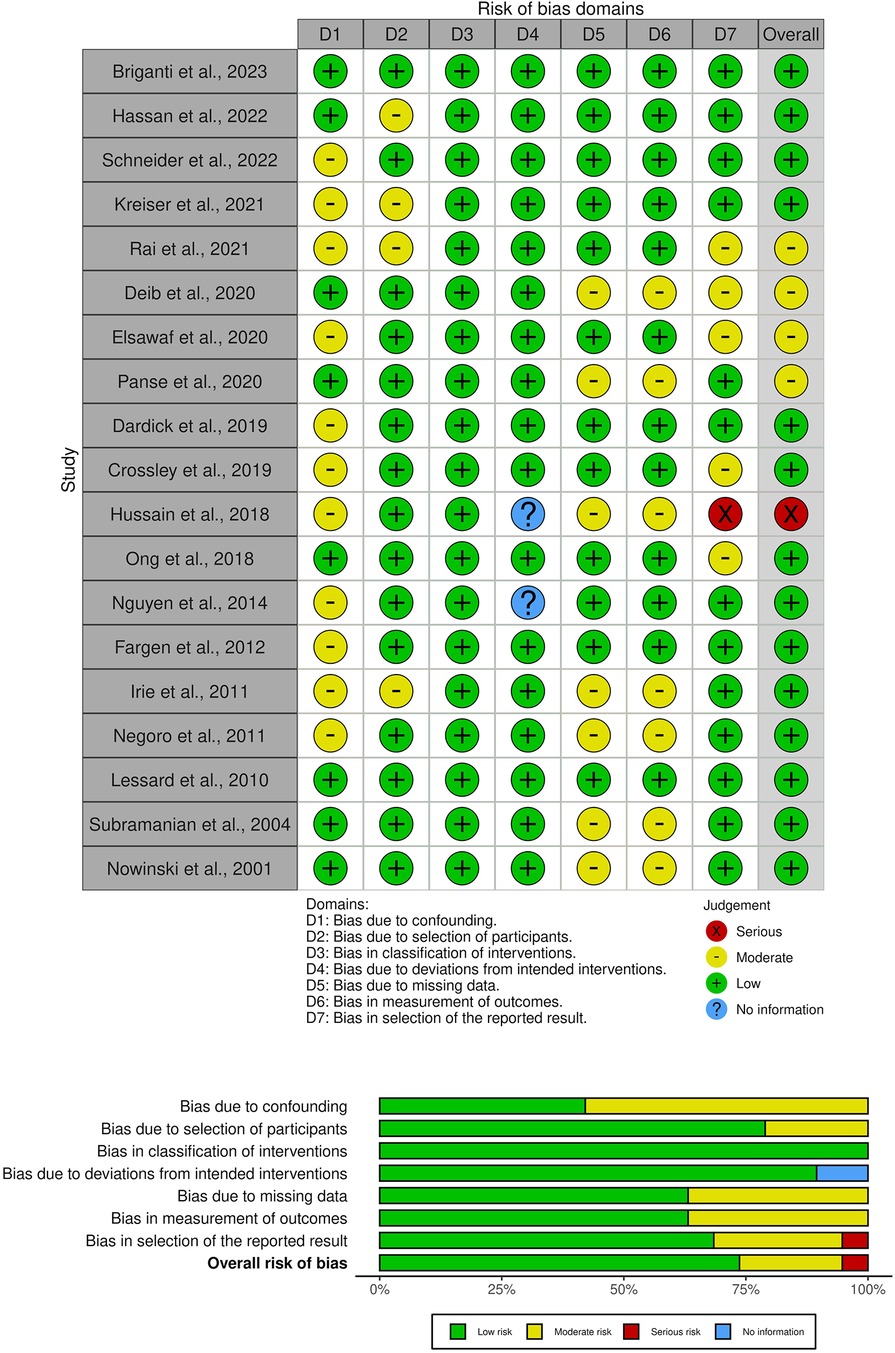
Figure 4. A risk-of-bias visualization was generated using robvis: An R package and shiny web app for visualizing risk-of-bias assessments. Studies on the left axis are classified into major sub-topics.
5 Conclusions
This systematic review synthesizes the current evidence regarding the use of XR technology in vascular neurosurgery, identifying various domains where XR is employed to train surgeons and highlighting opportunities for future innovation. While the role of XR in teaching anatomy is well-established for medical students and junior trainees, we reveal that XR technology offers significant benefits for advanced surgeons in preoperative planning through the creation of patient-specific, three-dimensional models derived from high-resolution imaging modalities. These customizable models, which can be manipulated in XR environments, provide critical insights into individual anatomical variations, enhancing the precision of surgical interventions. However, technological limitations persist, such as the lengthy time required to generate simulations and the occasional inaccuracies in patient-specific models. Despite these technological barriers, preliminary studies demonstrate the clinical promise of XR, suggesting its potential to transform neurosurgical training and planning.
In addition to pre-operative education, XR shows great potential in live intra-operative guidance, where experienced surgeons can remotely oversee and instruct trainees. Platforms like Proximie and the Magic Leap-1 have facilitated remote guidance with minimal latency–even over cellular data hotspots–which could prove especially valuable in resource-limited settings. However, we call for further large-scale studies to substantiate these benefits and explore new applications, such as our novel proposal to use XR to allow novice surgeons to virtually observe experienced colleagues.
Finally, VR simulations, like the Mentice VIST and Angio Mentor, have demonstrated efficacy in improving procedural performance and reducing training times. These tools also show promise in enhancing interdisciplinary education. While AR and VR simulation consistently show benefits across many studies and diverse metrics, there remains a need for rigorous evaluation of XR's impact on long-term skill acquisition. Reports on long-term outcomes, implementation in low-resource settings, technological improvements, and cost-effectiveness are still needed, but augmented, mixed, and virtual reality together hold promise for a paradigm shift in endovascular neurosurgery training.
Author contributions
SP: Conceptualization, Data curation, Formal Analysis, Investigation, Methodology, Project administration, Supervision, Validation, Visualization, Writing – original draft, Writing – review & editing. MC: Conceptualization, Data curation, Formal Analysis, Investigation, Validation, Visualization, Writing – original draft, Writing – review & editing. SP: Conceptualization, Data curation, Formal Analysis, Investigation, Validation, Visualization, Writing – original draft, Writing – review & editing. SK: Project administration, Resources, Software, Supervision, Validation, Visualization, Writing – original draft, Writing – review & editing. SKP: Conceptualization, Data curation, Formal Analysis, Investigation, Validation, Visualization, Writing – original draft, Writing – review & editing, Software, Supervision. AG: Conceptualization, Data curation, Formal Analysis, Investigation, Validation, Visualization, Writing – original draft, Writing – review & editing. OS: Conceptualization, Methodology, Project administration, Resources, Software, Supervision, Validation, Writing – review & editing. GS: Conceptualization, Methodology, Project administration, Resources, Software, Supervision, Validation, Writing – review & editing. AD: Methodology, Project administration, Resources, Software, Supervision, Validation, Writing – review & editing. TG: Investigation, Methodology, Project administration, Resources, Software, Supervision, Validation, Writing – review & editing. J-KB: Investigation, Methodology, Project administration, Resources, Supervision, Writing – review & editing. VS: Conceptualization, Investigation, Methodology, Project administration, Resources, Software, Supervision, Validation, Writing – review & editing.
Funding
The author(s) declare no financial support was received for the research, authorship, and/or publication of this article.
Conflict of interest
The authors declare that the research was conducted in the absence of any commercial or financial relationships that could be construed as a potential conflict of interest.
Publisher's note
All claims expressed in this article are solely those of the authors and do not necessarily represent those of their affiliated organizations, or those of the publisher, the editors and the reviewers. Any product that may be evaluated in this article, or claim that may be made by its manufacturer, is not guaranteed or endorsed by the publisher.
Supplementary material
The Supplementary Material for this article can be found online at: https://www.frontiersin.org/articles/10.3389/fsurg.2024.1440228/full#supplementary-material
Abbreviations
XR, extended reality, MR, mixed reality, AR, augmented reality; VR, virtual reality; Mentice VIST, Mentice vascular intervention simulation trainer.
References
1. Khoong YM, Luo S, Huang X, Li M, Gu S, Jiang T, et al. The application of augmented reality in plastic surgery training and education: a narrative review. J Plast Reconstr Aesthet Surg. (2023) 82:255–63. doi: 10.1016/j.bjps.2023.04.033
2. Jean WC, Britz GW, DiMeco F, Elmi-Terander A, McIntyre C. Introduction. Virtual and augmented reality in neurosurgery: a timeline. Neurosurg Focus. (2021) 51(2):E1. doi: 10.3171/2021.5.FOCUS21313
3. Kersten-Oertel M, Gerard I, Drouin S, Mok K, Sirhan D, Sinclair DS, et al. Augmented reality in neurovascular surgery: feasibility and first uses in the operating room. Int J Comput Assist Radiol Surg. (2015) 10(11):1823–36. doi: 10.1007/s11548-015-1163-8
4. Deib G, Smith D, Chaudhary B, Boo S, Tarabishy A, Carpenter J, et al. A mixed reality spatial computing framework for preprocedural evaluation of cerebral aneurysms: approach and preliminary results. J Neurointerv Surg. (2020):12. ((Deib G.; Boo S.; Tarabishy A.; Carpenter J.; Rai A.) Neuroradiology, West Virginia University, Morgantown, WV, United States(Smith D.; Chaudhary B.) Reed College of Media, West Virginia University, Morgantown, WV, United States):A134–5.
5. Nguyen N, Eagleson R, Boulton M, De Ribaupierre S. Realism, criterion validity, and training capability of simulated diagnostic cerebral angiography. Stud Health Technol Informatics. (2014) 196:297–303. Available online at: https://www.scopus.com/inward/record.uri?eid=2-s2.0-84897748807&doi=10.3233%2f978-1-61499-375-9-297&partnerID=40&md5=213d86671b9f88c6ac9602c0859c8b2c
6. Chui CK, Li Z, Anderson JH, Murphy K, Venbrux A, Ma X, et al. Training and pretreatment planning of interventional neuroradiology procedures-initial clinical validation. Stud Health Technol Informatics. (2002) 85:96–102. Available online at: https://www.scopus.com/inward/record.uri?eid=2-s2.0-4544322568&doi=10.3233%2f978-1-60750-929-5-96&partnerID=40&md5=b9e9c6108a8495f695a81fa032b23592
7. Nowinski WL. Virtual Reality in Brain Intervention: Models and Applications. Shanghai: Institute of Electrical and Electronics Engineers Inc (2005). p. 4164–7.
8. Nowinski WL. Virtual reality in brain intervention. Int J on Artif Intell Tools. (2006) 15(5):741–52. doi: 10.1142/S0218213006002916
9. Nowinski WL, Chui CK. Simulation of interventional neuroradiology procedures. In Institute of Electrical and Electronics Engineers Inc.; (2001). p. 87–94. Available online at: https://www.scopus.com/inward/record.uri?eid=2-s2.0-84964495012&doi=10.1109%2fMIAR.2001.930269&partnerID=40&md5=4f3ea2075e9ec677cf2759b3ce960f72 (Accessed June 15, 2023).
10. Lu Y, Ma X, Loe K, Chui C, Nowinski WL. Haptic vascular modeling and visualization in web-enabled interventional neuroradiology simulation system. In Diego San, CA; (2004). p. 201–8. Available online at: https://www.scopus.com/inward/record.uri?eid=2-s2.0-5644235416&doi=10.1117%2f12.535253&partnerID=40&md5=29fa0afe61025e3dddd8fbb2b6c47421 (Accessed July 14, 2023).
11. Rai AT, Deib G, Smith D, Boo S. Teleproctoring for neurovascular procedures: demonstration of concept using optical see-through head-mounted display, interactive mixed reality, and virtual space sharing-A critical need highlighted by the COVID-19 pandemic. Am J Neuroradiol. (2021) 42(6):1109–15. doi: 10.3174/ajnr.A7066
12. Hassan A, Desai S, Georgiadis A, Tekle W. Single center experience utilizing a novel augmented reality platform to teleproctor a neuroendovascular fellow. J Neurointerv Surg. 2021;13(SUPPL 2):A15. doi: 10.1136/neurintsurg-2020-016045
13. Cannizzaro D, Zaed I, Safa A, Jelmoni AJM, Composto A, Bisoglio A, et al. Augmented reality in neurosurgery, state of art and future projections. A systematic review. Front Surg. (2022) 9:864792. doi: 10.3389/fsurg.2022.864792. Available online at: https://www.frontiersin.org/articles/10.3389/fsurg.2022.864792 (cited May 22, 2024).35360432
14. McCloskey K, Turlip R, Ahmad HS, Ghenbot YG, Chauhan D, Yoon JW. Virtual and augmented reality in spine surgery: a systematic review. World Neurosurg. (2023) 173:96–107. doi: 10.1016/j.wneu.2023.02.068
15. Schirmer CM, Mocco J, Elder JB. Evolving virtual reality simulation in neurosurgery. Neurosurgery. (2013) 73(SUPPL. 4):S127–37. doi: 10.1227/NEU.0000000000000060
16. Page MJ, McKenzie JE, Bossuyt PM, Boutron I, Hoffmann TC, Mulrow CD, et al. The PRISMA 2020 statement: an updated guideline for reporting systematic reviews. Br Med J. (2021) 372:n71. doi: 10.1136/bmj.n71
17. Sterne JA, Hernán MA, Reeves BC, Savović J, Berkman ND, Viswanathan M, et al. ROBINS-I: a tool for assessing risk of bias in non-randomised studies of interventions. Br Med J. (2016) 355:i4919. doi: 10.1136/bmj.i4919
18. Briganti F, Tortora M, Loiudice G, Tarantino M, Guida A, Buono G, et al. Utility of virtual stenting in treatment of cerebral aneurysms by flow diverter devices. Radiol Med. (2023) 128(4):480–91. doi: 10.1007/s11547-023-01620-x
19. Hassan AE, Desai SK, Georgiadis AL, Tekle WG. Augmented reality enhanced tele-proctoring platform to intraoperatively support a neuro-endovascular surgery fellow. Interv Neuroradiol. (2022) 28(3):277–82. doi: 10.1177/15910199211035304
20. Schneider MS, Sandve KO, Kurz KD, Dalen I, Ospel J, Goyal M, et al. Metric based virtual simulation training for endovascular thrombectomy improves interventional neuroradiologists’ simulator performance. Interv Neuroradiol. (2023) 29(5):577–82. doi: 10.1177/15910199221113902
21. Schneider M, Sandve K, Kurz K, Advani R, Kurz M, Fjetland L. Metric based virtual simulation training for mechanical thrombectomy improves interventional neuroradiologists simulator performance. Eur Stroke J. (2021) 6(1 SUPPL):324. doi: 10.1177/15910199221113902
22. Kreiser K, Strober L, Gehling K, Schneider F, Kohlbecher S, Schulz C, et al. Simulation training in neuroangiography-validation and effectiveness. Clin Neuroradiol. (2021) 31(2):465–73. doi: 10.1007/s00062-020-00902-5
23. Elsawaf Y, Rennert RC, Steinberg JA, Santiago-Dieppa DR, Olson SE, Khalessi AA, et al. Simulator training for endovascular neurosurgery. J Visualized Exp. (2020) (159):e60923. doi: 10.3791/60923-v
24. Panse A, Flexman M, Mory B, Webb P, Keenan P. Optimal projection angle planning tool for carm in augmented reality. J Neurointerv Surg. (2020):12. ((Panse A.; Flexman M.; Mory B.; Webb P.; Keenan P.) Philips Research, Cambridge, MA, United States):A117.
25. Dardick J, Allen S, Scoco A, Zampolin RL, Altschul DJ. Virtual reality simulation of neuroendovascular intervention improves procedure speed in a cohort of trainees. Surg Neurol Int. (2019) 10:184. doi: 10.25259/SNI_313_2019
26. Crossley R, Liebig T, Holtmannspoetter M, Lindkvist J, Henn P, Lonn L, et al. Validation studies of virtual reality simulation performance metrics for mechanical thrombectomy in ischemic stroke. J Neurointerv Surg. (2019) 11(8):775–80. doi: 10.1136/neurintsurg-2018-014510
27. Hussain M, Abdallah F, Grunwald I. The role of simulation training in the endovascular management of stroke. Neuroradiology. (2018):60. ((Hussain M.) Queen’s Hospital, Department of Neurosurgery, Romford, United Kingdom(Abdallah F.) Kasr AlAiny Medical Scool, Cairo University, Department of Neurology, Cairo, Egypt(Grunwald I.) Southend University Hospital, Department of Neuroradiology, Sou):S510–1.
28. Ong CS, Deib G, Yesantharao P, Qiao Y, Pakpoor J, Hibino N, et al. Virtual reality in neurointervention. J Vasc Interv Neurol. (2018) 10(1):17–22. PMID: 29922399.
29. Fargen K, Siddiqui A, Veznedaroglu E, Turner R, Ringer A, Mocco J. Simulator based angiography education in neurosurgery: results of a pilot educational program. J Neurointerv Surg. (2012) 4(6):438–41. doi: 10.1136/neurintsurg-2011-010128
30. Medical Advisory Secretariat. Coil embolization for intracranial aneurysms: an evidence-based analysis. Ont Health Technol Assess Ser. (2006) 6(1):1–114. PMID: 23074479.
31. Negoro M, Ikeda S, Arai F, Fukuda T, Irie K, Fukasaku K. Simulation-based training system for neurointervention. Interv Neuroradiol. (2011):17. ((Negoro M.; Irie K.) Fujita Health University, Toyoake-Nagoya, Japan(Ikeda S.; Arai F.; Fukuda T.) Nagoya University, School of Engineering, Nagoya, Japan(Fukasaku K.) Riken, Wako-Tokyo, Japan):87.21561554
32. Lessard S, Lau C, Chav R, Soulez G, Roy D, de Guise JA. Guidewire tracking during endovascular neurosurgery. Med Eng Phys. (2010) 32(8):813–21. doi: 10.1016/j.medengphy.2010.05.006
33. Subramanian N, Kesavadas T, Hoffmann KR. A prototype virtual reality system for preoperative planning of neuro-endovascular interventions. Stud Health Technol Inform. (2004) 98:376–81.15544308
34. Abundez Toledo M, Ghanem G, Fine S, Weisman D, Huang YM, Rouhani AA. Exploring the promise of virtual reality in enhancing anatomy education: a focus group study with medical students. Front Virtual Real. (2024):5. doi: 10.3389/frvir.2024.1369794
35. Sinou N, Sinou N, Filippou D. Virtual reality and augmented reality in anatomy education during COVID-19 pandemic. Cureus. (2023) 15(2):e35170. doi: 10.7759/cureus.35170
36. Anxionnat R, Berger MO, Kerrien E. Time to go augmented in vascular interventional neuroradiology? LNCS, Lect Notes Comput Sci Nice. (2013) 7815:3. doi: 10.1007/978-3-642-38085-3_2
37. Zawy Alsofy S, Sakellaropoulou I, Nakamura M, Ewelt C, Salma A, Lewitz M, et al. Impact of virtual reality in arterial anatomy detection and surgical planning in patients with unruptured anterior communicating artery aneurysms. Brain Sci. (2020) 10(12):963. doi: 10.3390/brainsci10120963
38. Baert SAM, Viergever MA, Niessen WJ. Guide-wire tracking during endovascular interventions. IEEE Transactions on Medical Imaging. (2003) 22(8):965–72. doi: 10.1109/TMI.2003.815904
39. Gao B, Guo S, Hu K. Diagnosis and Treatment of Navigation Technology Based on the Multi-Modality Image Fusion for Angioneoplasm. Tianjin: IEEE Computer Society (2014). p. 565–70. Available online at: https://www.scopus.com/inward/record.uri?eid=2-s2.0-84906974888&doi=10.1109%2fICMA.2014.6885759&partnerID=40&md5=a38f8a181fe7734ddb636e62ecd590e9
40. Sengupta A, Kesavadas T, Hoffmann KR, Baier RE, Schafer S. Evaluating tool-artery interaction force during endovascular neurosurgery for developing haptic engine. Stud Health Technol Informatics (2007) 125:418–20. Available online at: https://www.scopus.com/inward/record.uri?eid=2-s2.0-34248585745&partnerID=40&md5=6b4fb6ca84780c8e36d740b6f6ea8330
41. Ma X. Latest development of an interventional radiology training simulation system: neuroCath. In: Duffy VG, editor. Digital Human Modeling. Berlin, Heidelberg: Springer (2007). p. 684–93.
42. Rossitto CP, Yudkoff C, Youssef D, Carrasquilla A, Carr MT, Oemke H, et al. Resection of arteriovenous malformation with sonolucent cranioplasty: 2-dimensional operative video with 360° virtual reality fly-through. Oper Neurosurg (Hagerstown). (2023) 25(1):e38. doi: 10.1227/ons.0000000000000686
43. Patel E, Aydin A, Cearns M, Dasgupta P, Ahmed K. A systematic review of simulation-based training in neurosurgery, part 2: spinal and pediatric surgery, neurointerventional radiology, and nontechnical skills. World Neurosurg. (2020) 133:E874–92. doi: 10.1016/j.wneu.2019.08.263
44. Kerray FM, Tambyraja AL. Simulated reality. Eur J Vasc Endovasc Surg. (2021) 62(6):999. doi: 10.1016/j.ejvs.2021.09.035
45. Tomlinson S, Hendricks B, Cohen-Gadol A. Immersive three-dimensional modeling and virtual reality for enhanced visualization of operative neurosurgical anatomy. World Neurosurg. (2019) 131:313–20. doi: 10.1016/j.wneu.2019.06.081
46. Elder JB, Hoh DJ, Oh BC, Heller AC, Liu CY, Apuzzo MLJ. The future of cerebral surgery: a kaleidoscope of opportunities. Neurosurgery. (2008) 62(6 SUPPL.):SHC1555–79. doi: 10.1227/01.NEU.0000316426.13241.A9
47. McGuire L, Fuentes A, Alaraj A. Three-Dimensional modeling in training, simulation, and surgical planning in open vascular and endovascular neurosurgery: a systematic review of the literature. World Neurosurg. (2021) 154:53–63. doi: 10.1016/j.wneu.2021.07.057
48. European Stroke Conference. 26th conference, Berlin, Germany, May 24–26, 2017: abstract e-Book. Cerebrovasc Dis. (2017) 43(Suppl 1):I–II. doi: 10.1159/000471872
49. National Institutes of Health. RePORT ⟩ RePORTER. Available online at: https://reporter.nih.gov/search/GW36fskc5k2QuWqSsnT2Yw/project-details/8410568 (cited May 24, 2024).
Keywords: virtual reality, augmented reality, mixed reality, endovascular, neurosurgery
Citation: Patel SA, Covell MM, Patel S, Kandregula S, Palepu SK, Gajjar AA, Shekhtman O, Sioutas GS, Dhanaliwala A, Gade T, Burkhardt J-K and Srinivasan VM (2024) Advancing endovascular neurosurgery training with extended reality: opportunities and obstacles for the next decade. Front. Surg. 11:1440228. doi: 10.3389/fsurg.2024.1440228
Received: 29 May 2024; Accepted: 12 August 2024;
Published: 27 August 2024.
Edited by:
Cesare Zoia, San Matteo Hospital Foundation (IRCCS), ItalyReviewed by:
Prajwal Ghimire, King’s College Hospital NHS Foundation Trust, United KingdomVittorio Ricciuti, University of Milano Bicocca, Italy
Copyright: © 2024 Patel, Covell, Patel, Kandregula, Palepu, Gajjar, Shekhtman, Sioutas, Dhanaliwala, Gade, Burkhardt and Srinivasan. This is an open-access article distributed under the terms of the Creative Commons Attribution License (CC BY). The use, distribution or reproduction in other forums is permitted, provided the original author(s) and the copyright owner(s) are credited and that the original publication in this journal is cited, in accordance with accepted academic practice. No use, distribution or reproduction is permitted which does not comply with these terms.
*Correspondence: Visish M. Srinivasan, visish.srinivasan@pennmedicine.upenn.edu