- 1Department of Anesthesiology, Affiliated Shanghai Sixth People's Hospital, Shanghai Jiao Tong University, Shanghai, China
- 2Department of ICU, Affiliated Shanghai Sixth People's Hospital, Shanghai Jiao Tong University, Shanghai, China
Introduction: Sepsis delays wound healing owing to uncontrolled inflammation. A single perioperative dose of dexamethasone is widely used because of its anti-inflammatory effects. However, the effects of dexamethasone on wound healing in sepsis remain unclear.
Methods: We discuss the methods to obtain dose curves and explore the safe dosage range for wound healing in mice with or without sepsis. A saline or LPS intraperitoneal injection was applied to C57BL/6 mice. After 24 hours, the mice received a saline or DEX intraperitoneal injection and full-thickness, dorsal wounding operation. Wound healing was observed by image record, immunofluorescence and histological staining. Inflammatory cytokines and M1/M2 macrophages in wounds were determined by ELISA and immunofluorescence, respectively.
Results: Dose-response curves reflected the safe dosage range of DEX in mice with or without sepsis, from 0.121 to 2.03 mg/kg and from 0 to 0.633 mg/kg, respectively. we found that a single dose of dexamethasone (1 mg/kg, i.p.) promoted wound healing in septic mice, but delayed wound healing in normal mice. In normal mice, dexamethasone delays inflammation, resulting in an insufficient number of macrophages during the healing process. In septic mice, dexamethasone alleviated excessive inflammation and maintained the balance of M1/M2 macrophages in the early and late healing process.
Discussion: In summary, the safe dosage range of dexamethasone in septic mice is wider than that in normal mice. A single dose of dexamethasone (1 mg/kg) increased wound healing in septic mice, but delayed it in normal mice. Our findings provide helpful suggestions for the rational use of dexamethasone.
1. Introduction
Wound healing is a well-orchestrated process involving many tightly controlled factors that work in concert (1). The healing process consists of four distinct yet overlapping stages: haemostasis, inflammation, proliferation, and remodelling. Many risk factors hamper the tight control of this process, including steroids, systemic inflammation status, and comorbidities.
Sepsis is characterised by a severe systemic inflammation due to an imbalance in the body’s response to infection (2, 3). Intra-abdominal infection has the highest mortality rate (30.7%) (4), and surgery is the most viable therapeutic measure to control such infections (5). However, sepsis has been proven in basic research and clinical trials to delay wound healing (6, 7), and patients with sepsis may suffer from impaired healing of the surgical incision.
Dexamethasone (DEX) has potent anti-inflammatory and immunosuppressive effects. The clinical outcomes of corticosteroid treatment in patients with sepsis or septic shock are associated with dosage. While low-dose dexamethasone has been reported to reduce mortality risk in patients with sepsis, high-dose dexamethasone may result in more harm than benefits (8). However, few studies have compared the effects of dexamethasone on wound healing in septic and non-septic conditions.
A perioperative single dose of dexamethasone is widely used to prevent postoperative nausea and vomiting (PONV) (9). However, the routine perioperative use of dexamethasone is still controversial (10, 11). Dexamethasone did not increase the incidence of surgical-site infection within 30 days after nonurgent noncardiac surgery (12) when compared with placebo controls. However, it is uncertain whether dexamethasone affects wound healing in the general surgical population (13).
Therefore, we hypothesised that dexamethasone (1 mg/kg, i.p.) may have different effects on wound healing in septic mice than in normal mice. We also explored the possible mechanism of action of dexamethasone in wound healing in the context of sepsis. Of note, we depicted the differences between the two dose–response curves, which explored the safe dosage range of dexamethasone in mice with or without sepsis.
2. Materials and methods
2.1. Dose–response curves
A total of 260 mice were allocated to two groups and received an intraperitoneal injection of dexamethasone at doses ranging from 0 to 5 mg/kg (9–11 doses per experiment). For normal mice, the complete range of dexamethasone doses was 0, 0.125, 0.25, 0.375, 0.5, 1, 1.25, 2.5, and 5 mg/kg (six mice per dose). Dexamethasone was administered to septic mice at 0, 0.125, 0.25, 0.5, 1, 1.25, 1.6, 2, 2.5, 3, and 5 mg/kg (6–10 mice per dose).
2.2. LPS sepsis model and medication procedure
Male C57BL/6 mice (6–8 weeks) were housed 3–5 per cage and had free access to water and food throughout the experiment; the padding was replaced at least three times a week. The protocol complied with the NIH Guide for the Care and Use of Laboratory Animals (NIH Publications No. 8023, revised 1978) and was approved by the Animal Ethics Committee of the Sixth People’s Hospital Affiliated to Shanghai Jiao Tong University, and was reported in accordance with the ARRIVE guidelines.
The mice were randomly separated into four experimental groups: control, DEX, sepsis, and sepsis + DEX group (Figure 1). The control and DEX groups received an intraperitoneal (i.p.) injection of saline solution, and the other groups received lipopolysaccharide (LPS) (O55:B5, Sigma Aldrich, St. Louis, MO, United States) injection (i.p.) at a dose of 10 mg/kg (14–17). After 24 h, mice with signs of lethargy, piloerection, and tachypnoea were diagnosed with sepsis (6). Dexamethasone (Sigma Aldrich, St. Louis, MO, United States), 1 mg/kg, was injected intraperitoneally into mice in the DEX group and sepsis + DEX group. The control and sepsis groups were injected with saline solution (i.p.).
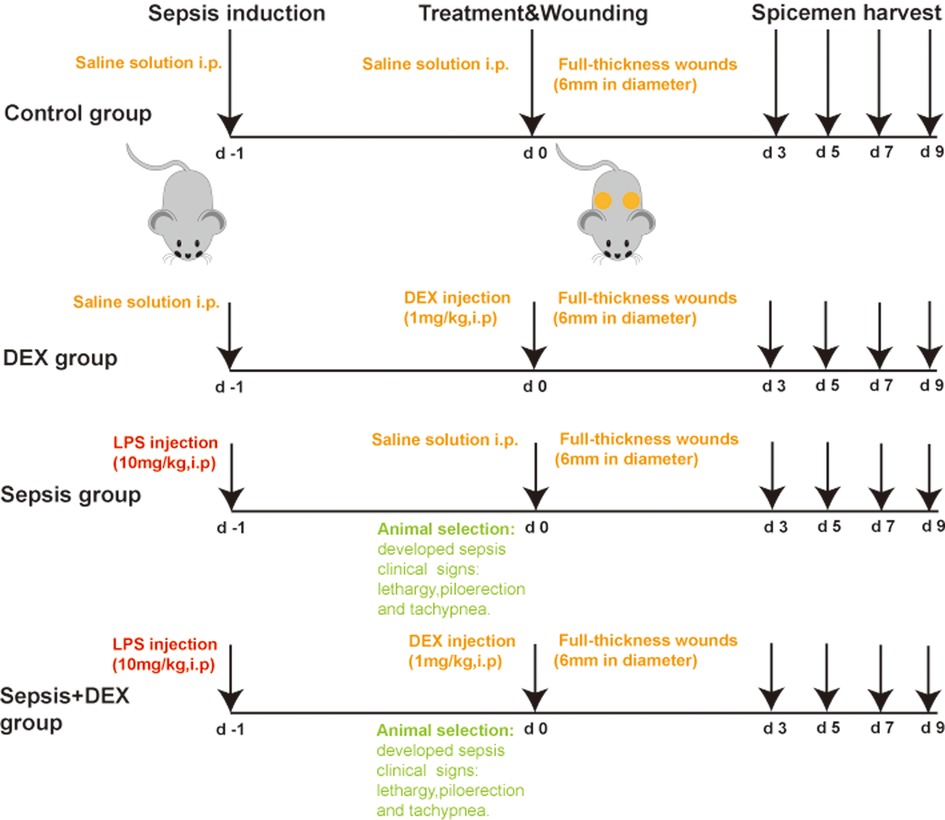
Figure 1. LPS sepsis model and medication procedure in the sepsis + DEX group. Medication procedure in 4 groups.
2.3. Skin wound model and evaluation of wound closure
A mouse full-thickness wound model was generated and all the experiments were performed at 0, 3, 5, 7, and 9 days after the operation.
Before the experiment, the mice were anaesthetised by intraperitoneal administration of 3% pentobarbital sodium, and their back hair was removed. After that, their skin was disinfected with 75% alcohol. Two full-thickness wounds (6 mm diameter) were created on each side of the dorsal midline using a sterilised biopsy punch. The centres of the wounds were located 28 mm cranial from the beginning of the tail and 8 mm lateral to the spine.
Images of the wounds were captured using a digital camera. A ruler was used to standardise the measurements, and the results were quantified using ImageJ software (version 2.0.0, United States). The wound closure% was calculated using the equation (A0 − An)/A0 × 100, where A0 is the original wound area and An is the wound area on days 3, 5, 7, or 9 post-surgery. At different time points, the mice were euthanised following the approved euthanasia protocol, and the original wound area and 2 mm-wide slices of skin around the wound surface were harvested and sectioned for further investigation.
2.4. Histological analysis
For morphometric analysis, the wound specimens were sectioned, stained with haematoxylin and eosin (H&E) according to the manufacturer’s protocol, and observed by light microscopy (Leica DM IL LED, Buffalo Grove, IL, United States). The thickness of granulation tissue was defined as the distance from the wounded dermal upper margin to the bottom of the area that is rich in cellular infiltration and revascularisation (18) (five measurement points per view). The thickness of the epidermis (imaged at ×200), granulation tissue (imaged at ×50), and the number of blood vessels (imaged at ×200) and follicles (imaged at ×50) were calculated in three randomly selected views per specimen using ImageJ software.
2.5. Immunofluorescence analysis
Immunofluorescence staining was performed on the tissue sections to assess vessel regeneration and granulation tissue with the expression of CD31 and α-smooth muscle actin, respectively. Cryosections of the tissue were fixed in paraformaldehyde (4%) for 15 min, washed with phosphate buffered saline (PBS) for 15 min, and blocked with 1% goat serum for 1 h before staining with primary antibodies overnight. The slices were incubated with the following primary antibodies: anti-mouse CD31 antibody (BD Pharmingen, clone 550274) 1:100 and anti-mouse α-smooth muscle actin (Cell Signalling Technology, clone 19245) 1:100.
To detect the effect of dexamethasone on macrophage polarisation in wounds, skin slices were processed by immunofluorescence staining with anti-mouse iNOS (Abcam, clone ab178945) 1:50 and anti-mouse Arg-1 (Santa Cruz Biotechnology, sc-271430) 1:500. After the primary antibodies were removed, Alexa Fluor 488- or Alexa Fluor 594-conjugated secondary antibodies (Cell Signalling Technology, MA, United States) 1:500 were used, followed by staining with DAPI (SouthernBiotech, Birmingham, United States).
2.6. Enzyme-linked immunosorbent assay
Wound samples collected on days 3, 5, 7, and 9 were frozen at −80°C. Frozen samples were carefully minced with sharp scissors and homogenised in cold PBS containing protease inhibitors (NCM Biotech, Suzhou, China) at a weight-to-volume ratio of 1 mg/mL. The samples were centrifuged at 2,500 rpm for 10 min at 4°C. The supernatant was analysed using a BCA Protein Assay Kit (Beyotime Biotechnology, Shanghai, China). Tissue levels of IL-6, TNF-α, and IL-10 were measured using enzyme-linked immunosorbent assay (ELISA) kits (Neobioscience Technology, Beijing, China), following the manufacturer’s instructions.
2.7. Statistics
Data are presented as the mean ± SEM. All statistical analyses were performed using Prism 9 (GraphPad, San Diego, CA, United States). For the comparison between normal and septic mice or between dexamethasone-treated normal mice and dexamethasone-treated septic mice, a two-way ANOVA followed by Tukey’s post-hoc test was used. For the safe dosage range of dexamethasone, the dose–response curve in normal mice was generated using a four-parameter nonlinear regression, and the curve in septic mice was generated using a bell-shaped nonlinear regression. Correlations of the dose–response curve in normal mice were assessed with the Pearson correlation coefficient. In all cases, statistical significance was set at P < 0.05.
3. Results
3.1. Dose curves of DEX for wound healing in mice with and without sepsis
It is challenging to obtain the dose–response curve of dexamethasone for wound healing. The dose variable is always quantitative, and the crux depends on the forms of the dependent variable. Table 1 shows a comparison of the response variables. In plan A, the dependent variable is the event incidence, whereas it is the relative wound closure% in plan B. Usually, the relationship between x and y in a dose–response curve is described by nonlinear regression models. Taking the four-parameter logistic regression model as an example [Equation (1)]
where y is the response variable and x is the dose variable. If y decreases as the dose increases, l is the lower limit of the outcome variable at an infinitely large dose and u represents the upper limit of the outcome variable when the dose is zero. Parameter e indicates the dose inducing a response halfway between u and l parameters (19). In general (e.g., the dependent variable is cell viability), the value of u does not exceed 100% (the formula for cell viability determines the range of this value). Similarly, l was not less than 0% (20, 21). In plan A (Table 1), u and l satisfy the conditions in the model (u ≤ 100% and l ≥ 0%). There is only one new parameter to be defined: a certain value of the delayed-healing threshold. In our study, the average wound closure% of the control group was taken as the threshold value, which was used to normalise the data in experimental groups in previous studies (19, 20). However, u and l in plan B (the dependent variable was normalised by the control group) failed to meet the application conditions. Thus, Plan A (Table 1) was a better choice.
To determine the association between dosing and the different effects of dexamethasone mentioned above, we performed a medium-safe dose experiment in mice with and without sepsis (Figure 2A). Taking the average wound closure% (94.8% ± 4.73%) of the control group on day 9 as the standard (when the wound closure% was less than this value, it was determined as delayed healing), we obtained the dose curves (Figures 2B,C). The dexamethasone dose at which delayed healing of 50% of the mice occurred was calculated as 0.633 mg/kg in dexamethasone-treated normal mice, while for dexamethasone-treated septic mice it ranged from 0.121 to 2.03 mg/kg (Figures 2B,C, Tables 2, 3). The safe dosage range for wound healing in mice without sepsis was from 0 to 0.633 mg/kg (Figure 2B), and that in mice with sepsis was from 0.121 to 2.03 mg/kg (Figure 2C). There was a positive correlation between the dose of dexamethasone and the incidence of delayed wound healing (R2 = 0.665, P = 0.007) in wounds without sepsis.
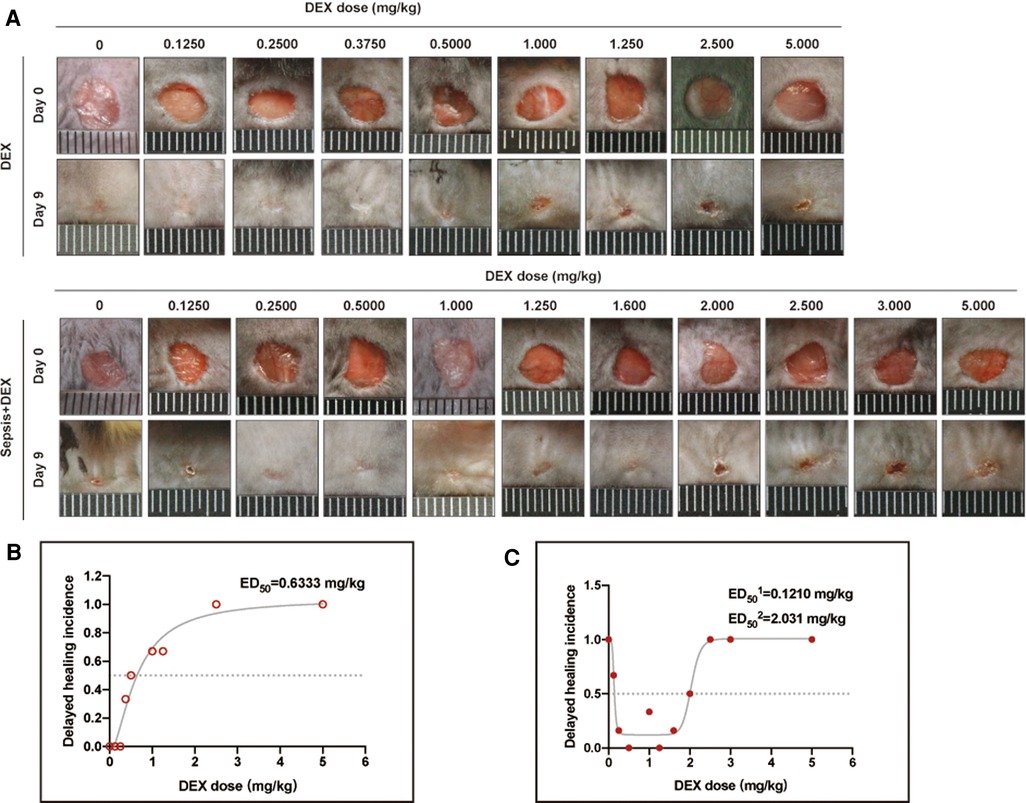
Figure 2. Dose curves of dexamethasone for wound healing in mice with and without sepsis. (A) Appearance of wounds on days 0 and 9 for DEX and sepsis + DEX groups. (B) Dose curve of dexamethasone for wound healing in normal mice. R2 is 0.959. (C) Dose curve of dexamethasone for wound healing in septic mice. R2 is 0.958. In (B,C), the non-healing wound was defined as that wound closure rate on day 9 was less than 90.1%. Parameter values are presented in Tables 2, 3.
3.2. Intraperitoneal injection of dexamethasone (1 mg/kg) promoted wound healing in septic mice but delayed it in normal mice
To explore whether a single dose of dexamethasone (1 mg/kg, i.p.) has different effects on wound healing in normal and septic mice, we compared wound states among four groups of mice (six mice per group): control (intraperitoneal injection of saline solution to C57BL/6 mice), DEX (DEX, i.p.), sepsis (LPS, i.p.), and sepsis + DEX (septic mice with DEX, i.p.) groups. As shown in Figure 3, dexamethasone delayed wound healing from day 3 after wounding in normal mice but accelerated the healing process in septic mice from day 7 (Figure 3E), which was visualised by image records and a schematic diagram (Figures 3A,D). By immunofluorescence staining, we observed less granulation tissue (revealed by αSMA-positive areas) and blood vessels (indicated by CD31 distribution) in the DEX group on day 9 after wounding than in the control group (Figures 3B,C). In contrast, more granulation tissue and blood vessels were seen in the sepsis + DEX group than in the sepsis group.
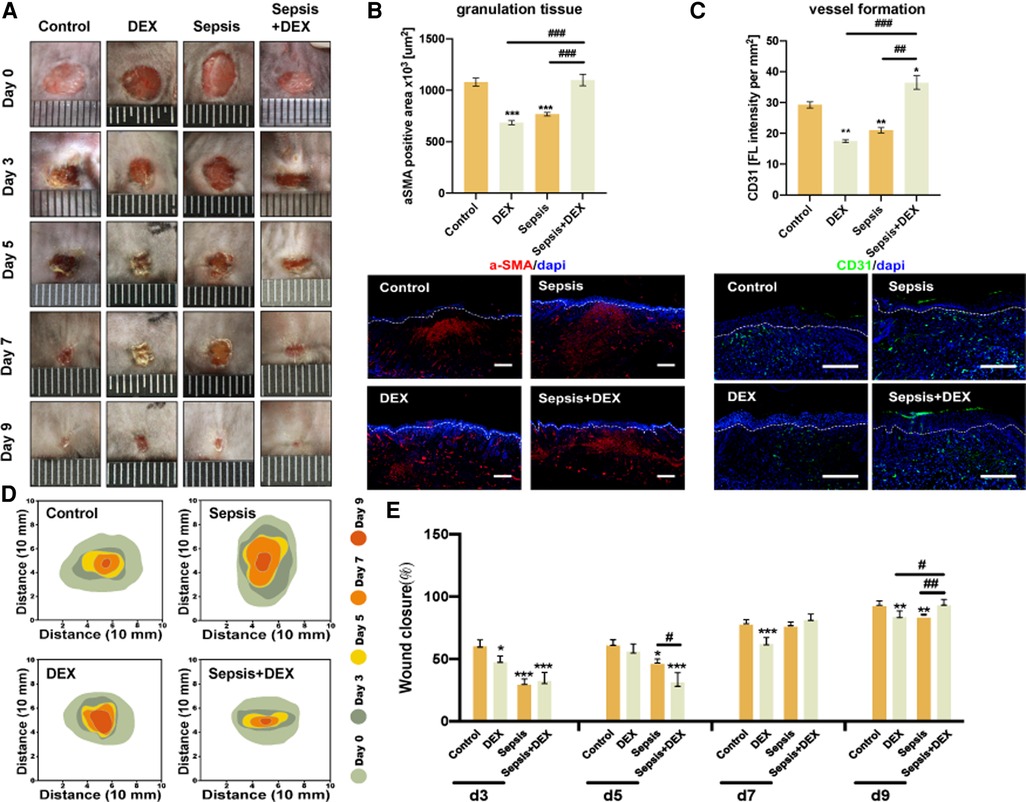
Figure 3. Intraperitoneal injection of dexamethasone (1 mg/kg) promoted wound healing in septic mice but delayed that in normal mice. Mice received intraperitoneal LPS (10 mg/kg) injection (sepsis and sepsis + DEX groups) or saline solution (control and DEX groups). After 24 h, dexamethasone was injected (1 mg/kg, i.p.) (DEX and sepsis + DEX groups) or normal saline (control and sepsis groups). Then, all groups had the wounding operation. Observation period included days 0, 3, 5, 7, and 9 after wounding. (A) Appearance of wounds on days 0, 3, 5, 7, and 9 for control, sepsis, DEX, and sepsis + DEX groups. (B) Granulation on day 9 after wounding. αSMA-IF (scale bar 500 μm), granulation tissue = αSMA-positive area (mean ± SEM, n = 6 per group). (C) Vessel formation on the 9th day after wounding. CD31-IF (scale bar 500 μm), angiogenesis = CD31 distribution/wound area (mean ± SEM, n = 3 per group). (D) Schematic diagram of wound area during 9 days for control, sepsis, DEX, and sepsis + DEX groups. Visualised by Adobe Illustrator. (E) Wound closure for each group. Quantitative data of image records (mean ± SEM, n = 6 per group). Wound closure rate was measured by the equation: (A0 − An)/A0 × 100, where A0 is the wound area on day 0, and An is the wound area of days 3, 5, 7, or 9 post-wounding. *P < 0.05, **P < 0.01, ***P < 0.001, vs. control group; #P < 0.05, ##P < 0.01, ###P < 0.001, vs. other groups. IF, immunofluorescence; αSMA, α-smooth muscle actin.
3.3. Intraperitoneal injection of dexamethasone (1 mg/kg) improved tissue regeneration in septic mice
Next, we assessed the quality of the regenerated tissue in the wounds by histological analysis (Figure 4). Mice in the DEX group expressed thinner epidermis on day 9 than those in the control group (Figures 4A,B). However, wounds in the sepsis + DEX group had thicker epidermis than those in the sepsis group, which indicated that dexamethasone improved epidermal regeneration in septic mice.
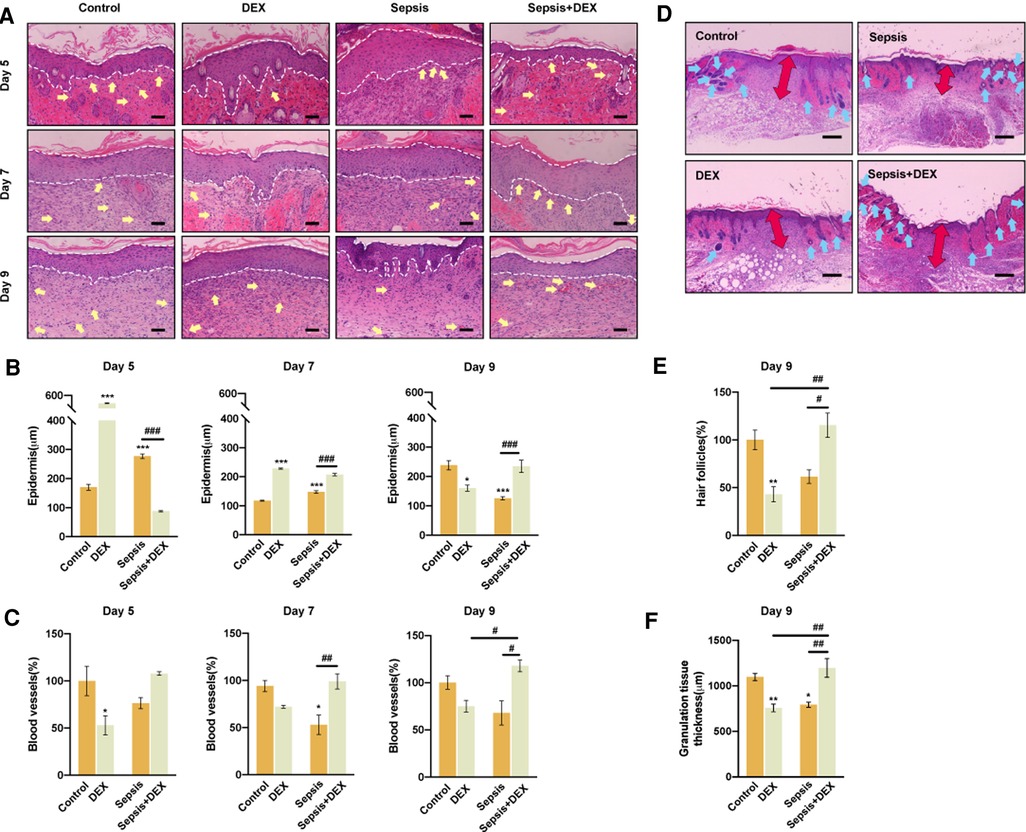
Figure 4. Intraperitoneal injection of dexamethasone (1 mg/kg) improved tissue regeneration in septic mice. (A) Wound H&E staining for control, sepsis, DEX, and sepsis + DEX on the 5th, 7th, and 9th day (blood vessels: yellow arrows, hair follicles: blue arrows, boundary of epithelium: white lines). Scale bar: 100 µm. (B) Epidermis thickness assessments for different groups on the 5th, 7th, and 9th day. (C) Blood vessels regeneration on the 5th, 7th, and 9th day. Quantified data were presented by relative number percentage. Control group on 5th day was set as 100%. (D) Granulation tissue (red arrows) thickness and amount of hair follicles (blue arrows) for different groups on the 9th day. Scale bar: 500 µm. (E) Hair follicles for different groups on day 9 post-wounding. Data were shown in relative number percentage. Control group was set as 100%. (F) Quantitative data of granulation tissue thickness. Data are shown as mean ± SEM, n = 3.*P < 0.05, **P < 0.01, ***P < 0.001, vs. control group; #P < 0.05, ##P < 0.01, ###P < 0.001, vs. other groups.
Soon after the injury, the fibrin clot is replaced by blood vessel-rich granulation tissue. In general, new vessel formation is essential for tissue repair by delivering nutrients and oxygen to injured tissue and removing waste products and carbon dioxide (22). Thus, blood vessel and granulation tissue conditions are closely related to the healing situation and are often used as evaluation parameters (23, 24). During the entire observation period, dexamethasone promoted angiogenesis and granulation tissue formation in wounds of the sepsis + DEX group, exhibiting a larger number of blood vessels and thicker granulation tissue than those in the sepsis group. However, dexamethasone suppressed vessel regeneration in the DEX group (Figures 4A,C).
The hair follicle is another regeneration parameter (as it is rich in several kinds of skin stem cells, which improves wound healing in re-epithelialisation and angiogenesis) (25–28), which was significantly increased by dexamethasone in the sepsis + DEX group compared with that in the DEX group on day 9 (Figure 4E). These results demonstrate that dexamethasone improved wound healing only in septic mice, but not in normal mice, in terms of enhancing angiogenesis and the formation of granulation and follicles.
3.4. Intraperitoneal injection of dexamethasone impaired the initiation of inflammation in wounds of normal mice but not in septic mice
Accumulating evidence shows that the effect of dexamethasone on wound healing is accompanied by changes in inflammatory cytokines (29–31). To reveal the association between the inflammatory response and the different effects of dexamethasone (1 mg/kg, i.p.) on wound healing in septic and normal mice, we assessed the expression of pro-inflammatory cytokines (IL-6 and TNF-α) and anti-inflammatory cytokine (IL-10) in the wound healing process (3–9 days).
As shown in the ELISA analysis (Figure 5), early in wound healing (days 3 and 5), dexamethasone decreased the expression of inflammatory cytokines in the DEX group (compared to the control group) and the sepsis + DEX group (compared to the sepsis group). Later in the healing process, the DEX group showed persistent inflammation with a higher level of IL-6 on day 7 than that in the control group. In addition, more TNF-α was secreted in the DEX group on days 7 and 9, although there were no statistically significant differences between the DEX and control groups. These results were different in the sepsis + DEX group. Dexamethasone suppressed the secretion of pro-inflammatory cytokines (IL-6 and TNF-α) during the early stages of wound healing. A higher level of IL-6 on day 7 and a lower level of inflammation (both IL-6 and TNF-α) on day 9 were observed in the Sepsis + DEX group than in the sepsis group.
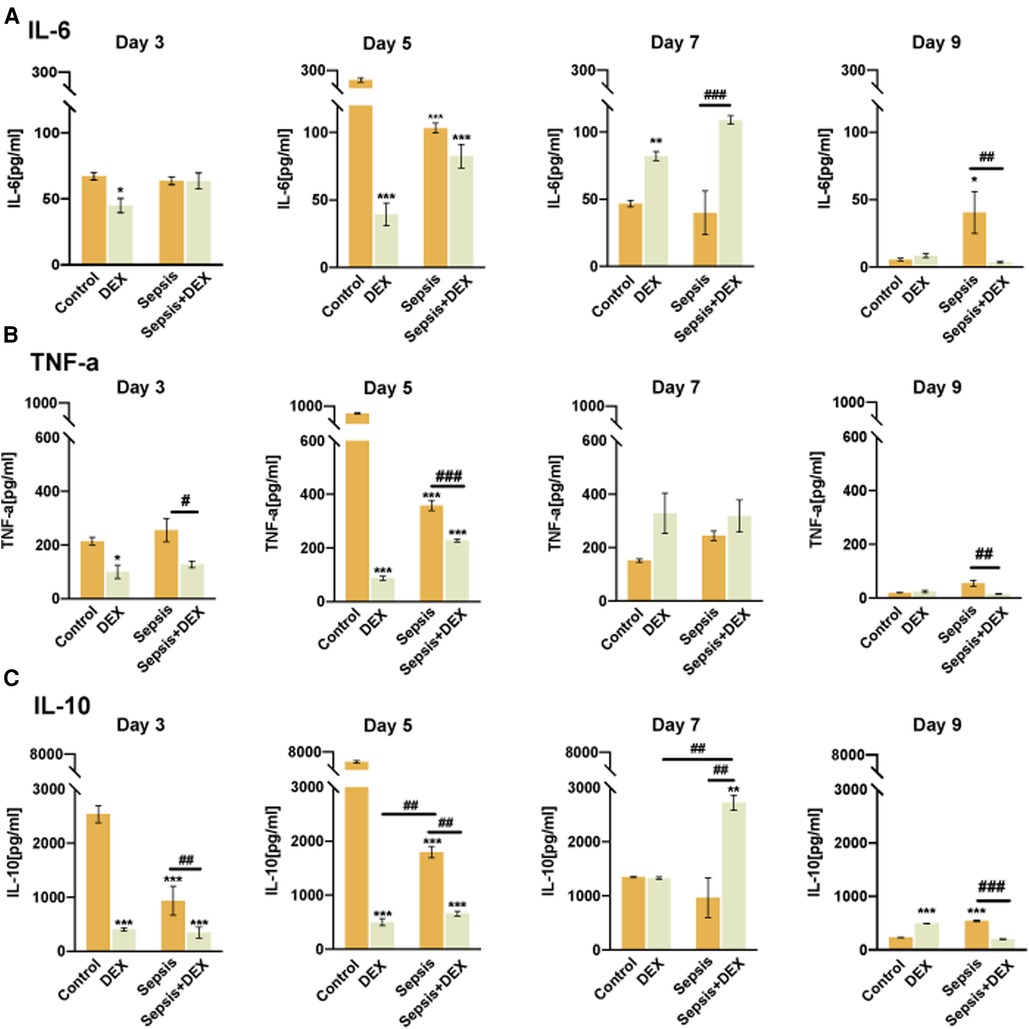
Figure 5. Intraperitoneal injection of dexamethasone impaired the initiation of inflammation in wounds of normal mice but not in septic mice. ELISA analysis of IL-6, TNF-α, and IL-10 in wounds. (A) The concentration of IL-6 in skin defects among control, DEX, sepsis, and sepsis + DEX groups on the 3rd, 5th, 7th, and 9th days after wounds creation. (B) TNF-α expression in tissue among the four groups on the 3rd, 5th, 7th, and 9th day after wounding. (C) IL-10 expression of wounds among different groups on the 3rd, 5th, 7th, and 9th day after the operation. All values represent mean ± SEM, n = 3.*P < 0.05, **P < 0.01, ***P < 0.001, vs. control group; #P < 0.05, ##P < 0.01, ###P < 0.001, vs. other groups. TNF-α, tumour necrosis factor-α; ELISA, enzyme-linked immunosorbent assay.
Of note, IL-10 showed a trend of changes, which were lower on days 3 and 5 in the DEX-treated groups than in the saline groups, and then reached a peak on day 7 but declined again on day 9.
3.5. The initiation of inflammation and subsequent macrophage polarisation towards M2 relied on adequate M1 macrophages in early wound healing
Macrophages play a prominent role in tissue repair by balancing the pro- and anti-inflammatory responses. Early in wound repair, M1 macrophages participate in pro-inflammatory activities and have traditionally been marked with iNOS. M2 macrophages, which promote inflammation resolution and tissue repair during the later stages of wound healing, are marked with Arg-1. To study how dexamethasone affects inflammation progression, we checked the number of macrophages (M1 + M2) and the M1/M2 ratio in wound sites using immunofluorescence staining (Figure 6).
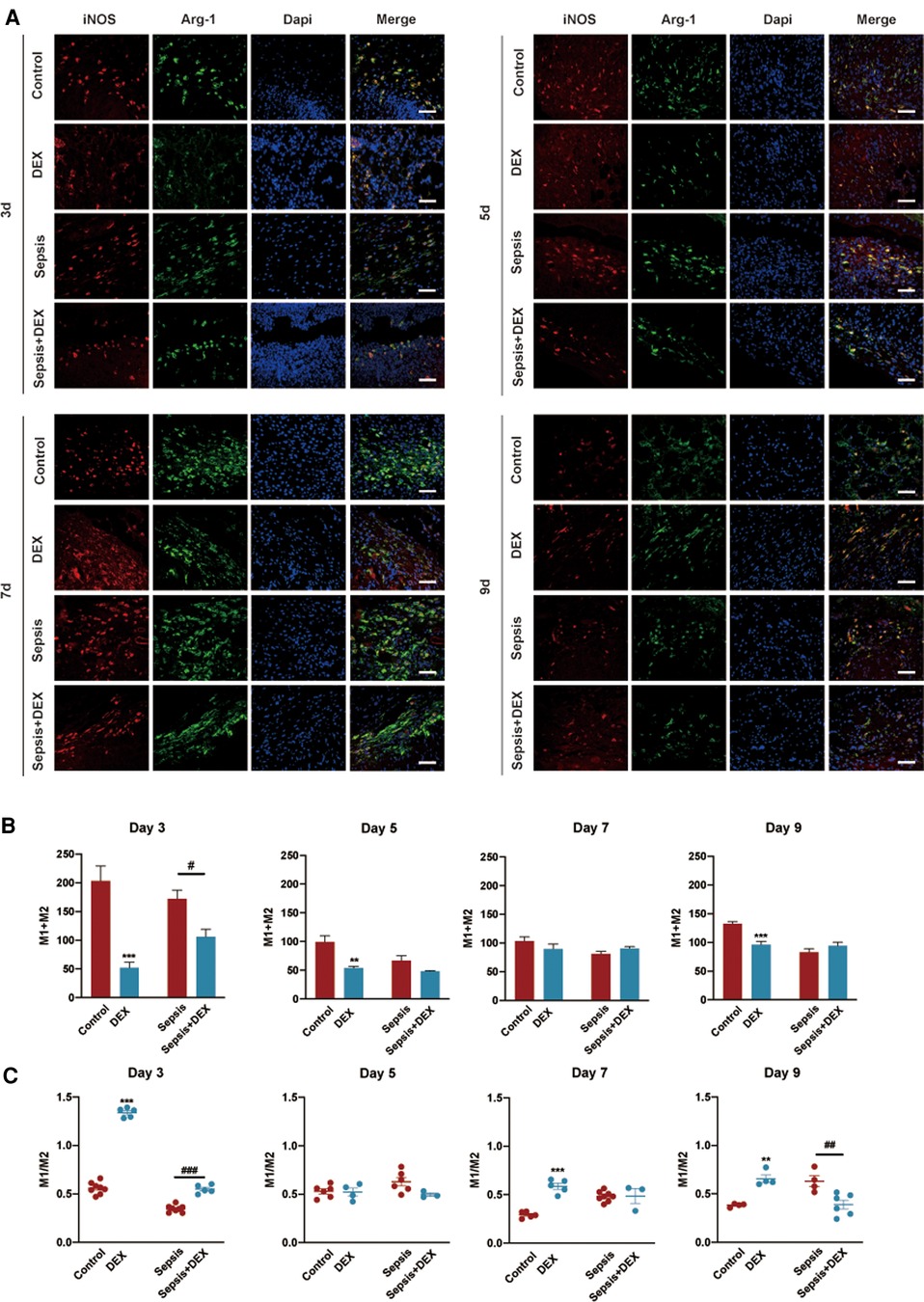
Figure 6. The initiation of inflammation and subsequent macrophage polarisation towards M2 relied on adequate M1 macrophages in early wound healing. Immunofluorescence staining analysis of M1 and M2 macrophages in wounds in various groups on the 3rd, 5th, 7th, and 9th day. (A) Representative histological slices of wounds stained by immunofluorescence: red (iNOS, M1 macrophages marker), green (Arg-1, M2 macrophages marker), and blue (DAPI). Scale bar: 100 µm. (B) Number of M1 and M2 macrophages. (C) M1/M2 ratio. (B,C) are quantised data of (A). All values represent mean ± SEM, n > 3.*P < 0.05, **P < 0.01, ***P < 0.001, vs. control group; #P < 0.05, ##P < 0.01, ###P < 0.001 vs. sepsis group. iNOS, inducible nitric oxide synthase; Arg-1, arginase-1.
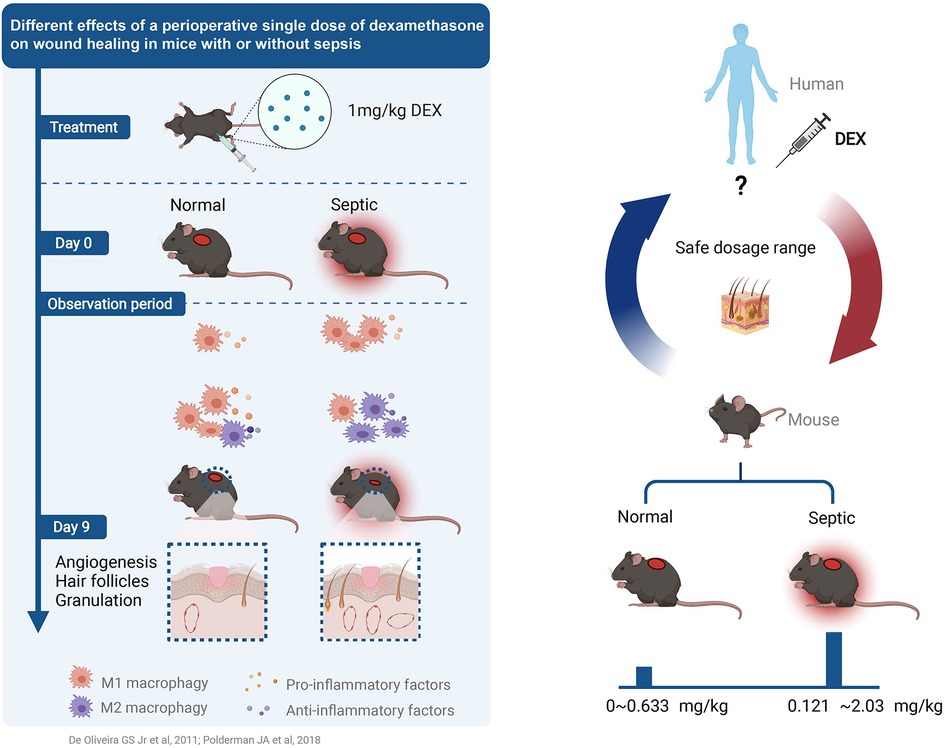
Figure 7. Different effects of a perioperative single dose of dexamethasone on wound healing in mice with or without sepsis (50, 51).
Throughout the wound healing process, mice in the DEX group had fewer macrophages (M1 + M2) than those in the control group. On day 3, more macrophages were observed in the sepsis group, but fewer were observed in the sepsis + DEX group. There were no significant differences in the number of M1 + M2 macrophages between the sepsis and Sepsis + DEX groups on days 5, 7, and 9.
The wounds in the DEX group displayed a higher M1/M2 ratio than those in the control group on day 3. During later tissue repair, a higher M1/M2 ratio was observed in the DEX group than in the control group.
On the other hand, there was a higher M1/M2 ratio in wounds of the sepsis + DEX group than in the sepsis group on day 3. Wounds in the sepsis + DEX group had a lower M1/M2 ratio on day 9 than those in the sepsis group.
These results indicate that dexamethasone decreased M1 macrophages in early wound healing, which may result in the phenotypic switch of macrophages from M1 to M2 being delayed or failing to occur.
4. Discussion
Dexamethasone, an approved corticosteroid medication, is widely used for prophylaxis and treatment of PONV. However, the safe dosage of dexamethasone for wound healing in the perioperative period, particularly in patients with sepsis, remains unknown.
Herein, we found that the differences between normal and septic mice were dose-related and described them in the dose–response curves. By comparing the incidence of delayed healing, we demonstrated that the therapeutic window of dexamethasone in septic mice was broader. Identification of the relationship between inflammatory response and wound healing may provide a plausible explanation for dose-related discrepancies.
Significantly, these curves may be beneficial for a rational plan of dexamethasone use. The differences between them highlight some important considerations regarding perioperative dexamethasone administration, namely, dosing and disease of the body.
By observing the dose–response curves, we found that intraperitoneal injection of dexamethasone (1 mg/kg) played a positive role in wound repair in septic mice but impaired it in normal mice. Previous studies have focused on either the association of wound healing and sepsis (6) or wound healing and dexamethasone (32); however, few investigations have focused on the impact of single-dose dexamethasone on wound repair under septic status. This is, so far as we know, the first study to explore the single dose of dexamethasone (1 mg/kg, i.p.) on wound healing in mice with and without sepsis and found the different effects of dexamethasone. Durmus et al. (32) identified that a single dose of dexamethasone at 1 mg/kg may have negative effects on wound healing in a prospective, randomised, experimental rat model. Our experiments confirmed these findings, and we found that a low dose of dexamethasone may benefit wound healing in septic mice.
Here, we monitored the progression of wound healing in two aspects: wound performance and the quality of tissue regeneration, and evaluated them using diverse parameters: wound closure, epidermal proliferation, angiogenesis, and the formation of granulation tissue and follicles. Moreover, we compared the results with those of their septic counterparts and found different effects of dexamethasone, which were visualised by multiple experimental methods: image recording, H&E staining, and immunofluorescence staining.
The dose–response curve in normal mice was generated using a four-parameter nonlinear regression; Carvalho et al. (33) and Ginosar et al. (34) used this model to describe the relationship between dose and response in dose–response curves, and correlations were assessed with the use of linear regression in their studies. For wounds without sepsis, there was a positive correlation between the dose of dexamethasone and the incidence of delayed wound healing in our study. The curve in septic mice was generated using a bell-shaped nonlinear regression; Owen et al. (35) and Zhu et al. (36) used this model to describe the relationship between dose and response in dose–response curves. They performed statistical analyses of the dose–response curves, However, the forms of the response in our study are different from theirs, and the bell-shaped model is the sum of two dose–response curves; the relationship between the dose of dexamethasone and the incidence of delayed wound healing is not suitable to describe with simple positive/negative correlation. If we perform statistical analyses of the dose–response curves, the other form of the response (relative wound closure rate) will be chosen, which may produce more problems that we discuss in Table 4.
The process of wound healing involves a programmed local inflammatory reaction that requires precise coordination and is dictated mainly by macrophages in response to tissue damage. Early in wound healing, the initial inflammatory response is characterised by macrophage tissue destruction, production of inflammatory cytokines, and clearance of pathogens and debris. This is followed by the resolution of inflammation and initiation of tissue repair. The timing of both initiation and resolution of inflammation is essential for restoring tissue integrity after injury. Macrophages, which initiate and resolve inflammation, play an indispensable role in wound healing and contribute to tissue regeneration. The transition from pro-inflammatory to reparative phenotypes is required for effective wound healing in an orderly manner (32, 37). However, in the pathological status, the phenotypic switch of macrophages can be perturbed, resulting in the insufficiency and imbalance of pro-inflammatory and anti-inflammatory macrophages, preventing wound repair (36, 38, 39).
Our study demonstrated that a single dose of dexamethasone reduced pro-inflammatory cytokine levels and the number of macrophages in the wounds of normal mice in the early healing stage. This failure to generate an initial inflammatory response negatively impacts the downstream orchestration of subsequent phases, resulting in ongoing inflammation instead of proceeding into the proliferative phase (39, 40).
Our data suggest that dexamethasone impairs the initiation of inflammation in normal mice by reducing the number of macrophages, which may result from the inhibition of macrophage polarisation towards the M1 phenotype.
Unlike in normal mice, dexamethasone decreased the inflammatory response in the early healing of septic mice, and in the later stage, the wounds expressed a lower level of inflammatory cytokines. During the wound healing process, the total number of M1 and M2 macrophages was equal in the sepsis and sepsis + DEX groups. However, we found that there were sufficient M1 macrophages in the early stages and M2 macrophages in later wound healing in dexamethasone-treated septic mice, exhibiting a higher M1/M2 ratio and a lower M1/M2 ratio, respectively. This ensures that macrophages function in both pro-inflammatory and reparative phenotypes (41–43). These data indicate that dexamethasone alleviated the degree of inflammation rather than impairing the initiation of inflammation in wounds of dexamethasone-treated septic mice in the early stages; thus, healing progressed with resolving inflammation.
During wound healing, the local inflammatory response to injury may be influenced by dysregulation of systemic inflammation. In the current study, we found that dexamethasone reduced excessive inflammation by reducing the number of macrophages but maintained the balance between M1 and M2 macrophages in wounds in the context of sepsis. This may be because of the following reasons. First, DEX may disturb macrophage polarisation in wounds. Indeed, using in vitro and in vivo models, prior studies have demonstrated that glucocorticoids have an inhibitory effect on the polarisation of macrophages towards a pro-inflammatory phenotype (44–46). Second, DEX may perturb the migration of macrophages from the circulation; however, this hypothesis contradicts the observations of Chatzopoulou et al. (47) and Xie et al. (44) that glucocorticoids have a limited effect on the migration of macrophages in the zebrafish tail amputation model.
In the peritonitis model, a systemic inflammatory response occurs with large numbers of M1 macrophages (44, 48), which may provide a pre-inflammatory environment. After wounding, large numbers of monocytes are recruited from circulation into the wound bed, and such monocytes may differentiate into pro-inflammatory macrophages in response to the pre-inflammatory environment. Dexamethasone treatment, however, reduced the expression of inflammatory cytokines and the polarisation of macrophages in early stages (29, 49) and had limited effects on specific macrophages (pre-inflammatory environment maker), which maintained the coordination of the initiation and resolution of inflammation in wound healing. However, the effect of dexamethasone may be conditional, and further investigation is warranted.
Our study offers insight into the rational use of dexamethasone. However, this study has some limitations that must be addressed. Since we know that the effects of DEX on wound healing are associated with macrophages, further studies are necessary to clarify the regulatory mechanism by which DEX affects phenotypic changes in macrophages during the wound healing process in sepsis. In addition, rodent models have limitations in the evaluation of the effects of drugs in clinical practice and do not completely reflect human disease; thus, more forms of disease models and injections should be investigated.
In conclusion, wound healing was promoted in septic mice but delayed in normal mice following a single-dose intraperitoneal injection of dexamethasone. Dexamethasone reduced inflammation without impairing the M1-induced initiation of inflammation and polarisation towards M2 in septic mice but impaired inflammation in normal mice. The two curves reflect the dose-related differences, which may provide considerable suggestions for the rational use of dexamethasone.
Data availability statement
The original contributions presented in the study are included in the article/Supplementary Material, further inquiries can be directed to the corresponding author/s.
Ethics statement
The animal study was reviewed and approved by Animal Ethics Committee of the Sixth People’s Hospital Affiliated to Shanghai Jiao Tong University.
Author contributions
YC designed the research and wrote the article. YC and XC acquired and analysed data. QZ supervised the entire research. All authors contributed to the article and approved the submitted version.
Funding
This work was supported by the National Natural Science Foundation of China (81771933).
Acknowledgments
We thank Chengyu Wang for his helpful suggestions about dose curves.
Conflict of interest
The authors declare that the research was conducted in the absence of any commercial or financial relationships that could be construed as a potential conflict of interest.
Publisher's note
All claims expressed in this article are solely those of the authors and do not necessarily represent those of their affiliated organizations, or those of the publisher, the editors and the reviewers. Any product that may be evaluated in this article, or claim that may be made by its manufacturer, is not guaranteed or endorsed by the publisher.
References
1. De Simone B, Sartelli M, Coccolini F, Ball CG, Brambillasca P, Chiarugi M, et al. Intraoperative surgical site infection control and prevention: a position paper and future addendum to WSES intra-abdominal infections guidelines. World J Emerg Surg. (2020) 15(1):10. doi: 10.1186/s13017-020-0288-4
2. Liao Y, Wang R, Wen F. Diagnostic and prognostic value of long noncoding RNAs in sepsis: a systematic review and meta-analysis. Expert Rev Mol Diagn. (2022) 22(8):821–31. doi: 10.1080/14737159.2022.2125801
3. He S, Leng W, Du X, He Y, Zhao Y, Wang Y, et al. Diagnostic significance of heart-type fatty acid-binding protein as a potential biomarker to predict the mortality rate of patients with sepsis: a systematic review and meta-analysis. Expert Rev Mol Diagn. (2022) 22(3):379–86. doi: 10.1080/14737159.2022.2046464
4. Vandewalle J, Libert C. Glucocorticoids in sepsis: to be or not to be. Front Immunol. (2020) 11:1318. doi: 10.3389/fimmu.2020.01318
5. Sartelli M, Coccolini F, Kluger Y, Agastra E, Abu-Zidan FM, Abbas AES, et al. WSES/GAIS/SIS-E/WSIS/AAST global clinical pathways for patients with intra-abdominal infections. World J Emerg Surg. (2021) 16(1):49. doi: 10.1186/s13017-021-00387-8
6. Davis FM, Schaller MA, Dendekker A, Joshi AD, Kimball AS, Evanoff H, et al. Sepsis induces prolonged epigenetic modifications in bone marrow and peripheral macrophages impairing inflammation and wound healing. Arterioscler Thromb Vasc Biol. (2019) 39(11):2353–66. doi: 10.1161/ATVBAHA.119.312754
7. Koskela M, Gäddnäs F, Ala-Kokko TI, Laurila JJ, Saarnio J, Oikarinen A, et al. Epidermal wound healing in severe sepsis and septic shock in humans. Crit Care. (2009) 13(3):R100. doi: 10.1186/cc7932
8. Son J-Y, Shin S, Choi YJ. New evidence of potential benefits of dexamethasone and added on therapy of fludrocortisone on clinical outcomes of corticosteroid in sepsis patients: a systematic review and meta-analysis. J Pers Med. (2021) 11(6):544. doi: 10.3390/jpm11060544
9. Gan TJ, Diemunsch P, Habib AS, Kovac A, Kranke P, Meyer TA, et al. Consensus guidelines for the management of postoperative nausea and vomiting. Anesth Analg. (2014) 118(1):689. doi: 10.1213/ANE.0000000000000002
10. Kakodkar PS. Routine use of dexamethasone for postoperative nausea and vomiting: the case for. Anaesthesia. (2013) 68(9):889–91. doi: 10.1111/anae.12308
11. Bartlett R, Hartle AJ. Routine use of dexamethasone for postoperative nausea and vomiting: the case against. Anaesthesia. (2013) 68(9):892–6. doi: 10.1111/anae.12309
12. Corcoran TB, Myles PS, Forbes AB, Cheng AC, Bach LA, O'Loughlin E, et al. Dexamethasone and surgical-site infection. N Engl J Med. (2021) 384(18):1731–41. doi: 10.1056/NEJMoa2028982
13. Polderman JA, Farhang-Razi V, Van Dieren S, Kranke P, DeVries JH, Hollmann MW, et al. Adverse side effects of dexamethasone in surgical patients. Cochrane Database Syst Rev. (2018) 8:CD011940. doi: 10.1002/14651858.CD011940.pub2
14. de Pádua Lúcio K, Rabelo ACS, Araújo CM, Brandão GC, de Souza GHB, da Silva RG, et al. Anti-inflammatory and antioxidant properties of black mulberry (Morus nigra L.) in a model of LPS-induced sepsis. Oxid Med Cell Longev. (2018) 2018:5048031. doi: 10.1155/2018/5048031
15. Li N, Wang W, Zhou H, Wu Q, Duan M, Liu C, et al. Ferritinophagy-mediated ferroptosis is involved in sepsis-induced cardiac injury. Free Radic Biol Med. (2020) 160:303–18. doi: 10.1016/j.freeradbiomed.2020.08.009
16. Song P, Shen D-F, Meng Y-Y, Kong C-Y, Zhang X, Yuan Y-P, et al. Geniposide protects against sepsis-induced myocardial dysfunction through AMPKα-dependent pathway. Free Radic Biol Med. (2020) 152:186–96. doi: 10.1016/j.freeradbiomed.2020.02.011
17. Wang Z, Kong L, Tan S, Zhang Y, Song X, Wang T, et al. Zhx2 accelerates sepsis by promoting macrophage glycolysis via Pfkfb3. J Immunol. (2020) 204(8):2232–41. doi: 10.4049/jimmunol.1901246
18. van de Vyver M, Boodhoo K, Frazier T, Hamel K, Kopcewicz M, Levi B, et al. Histology scoring system for murine cutaneous wounds. Stem Cells Dev. (2021) 30(23):1141–52. doi: 10.1089/scd.2021.0124
19. Keshtkar E, Kudsk P, Mesgaran MB. Perspective: common errors in dose-response analysis and how to avoid them. Pest Manag Sci. (2021) 77(6):2599–608. doi: 10.1002/ps.6268
20. Jimenez-Toro I, Rodriguez CA, Zuluaga AF, Otalvaro JD, Vesga O. A new pharmacodynamic approach to study antibiotic combinations against enterococci in vivo: application to ampicillin plus ceftriaxone. PLoS One. (2020) 15(12):e0243365. doi: 10.1371/journal.pone.0243365
21. Preez GD, Fourie H, Daneel M, Miller H, Höss S, Ricci C, et al. Oxygen consumption rate of Caenorhabditis elegans as a high-throughput endpoint of toxicity testing using the seahorse Xfe96 extracellular flux analyzer. Sci Rep. (2020) 10(1):4239. doi: 10.1038/s41598-020-61054-7
22. Bissell MJ, Hall HG, Parry G. How does the extracellular matrix direct gene expression? J Theor Biol. (1982) 99(1):31–68. doi: 10.1016/0022-5193(82)90388-5
23. Ferrer RA, Saalbach A, Grünwedel M, Lohmann N, Forstreuter I, Saupe S, et al. Dermal fibroblasts promote alternative macrophage activation improving impaired wound healing. J Invest Dermatol. (2017) 137(4):941–50. doi: 10.1016/j.jid.2016.11.035
24. Liang Y, Zhao X, Hu T, Chen B, Yin Z, Ma PX, et al. Adhesive hemostatic conducting injectable composite hydrogels with sustained drug release and photothermal antibacterial activity to promote full-thickness skin regeneration during wound healing. Small. (2019) 15(12):e1900046. doi: 10.1002/smll.201900046
25. Ito M, Yang Z, Andl T, Cui C, Kim N, Millar SE, et al. Wnt-dependent de novo hair follicle regeneration in adult mouse skin after wounding. Nature. (2007) 447(7142):316–20. doi: 10.1038/nature05766
26. Yang C-C, Cotsarelis G. Review of hair follicle dermal cells. J Dermatol Sci. (2010) 57(1):2–11. doi: 10.1016/j.jdermsci.2009.11.005
27. Chou WC, Takeo M, Rabbani P, Hu H, Lee W, Chung YR, et al. Direct migration of follicular melanocyte stem cells to the epidermis after wounding or UVB irradiation is dependent on Mc1r signaling. Nat Med. (2013) 19(7):924–9. doi: 10.1038/nm.3194
28. Paus R. Migrating melanocyte stem cells: masters of disaster? Nat Med. (2013) 19(7):818–9. doi: 10.1038/nm.3264
29. Hübner G, Brauchle M, Smola H, Madlener M, Fässler R, Werner S. Differential regulation of pro-inflammatory cytokines during wound healing in normal and glucocorticoid-treated mice. Cytokine. (1996) 8(7):548–56. doi: 10.1006/cyto.1996.0074
30. Desgeorges T, Caratti G, Mounier R, Tuckermann J, Chazaud B. Glucocorticoids shape macrophage phenotype for tissue repair. Front Immunol. (2019) 10:1591. doi: 10.3389/fimmu.2019.01591
31. Tu H, Zhang D, Barksdale AN, Wadman MC, Muelleman RL, Li Y-L. Dexamethasone improves wound healing by decreased inflammation and increased vasculogenesis in mouse skin frostbite model. Wilderness Environ Med. (2020) 31(4):407–17. doi: 10.1016/j.wem.2020.07.003
32. Durmus M, Karaaslan E, Ozturk E, Gulec M, Iraz M, Edali N, et al. The effects of single-dose dexamethasone on wound healing in rats. Anesth Analg. (2003) 97(5):1377–80. doi: 10.1213/01.ANE.0000080611.29106.9E
33. Carvalho B, Durbin M, Drover DR, Cohen SE, Ginosar Y, Riley ET. The Ed50 and Ed95 of intrathecal isobaric bupivacaine with opioids for cesarean delivery. Anesthesiology. (2005) 103(3):606–12. doi: 10.1097/00000542-200509000-00025
34. Ginosar Y, Mirikatani E, Drover DR, Cohen SE, Riley ET. Ed50 and Ed95 of intrathecal hyperbaric bupivacaine coadministered with opioids for cesarean delivery. Anesthesiology. (2004) 100(3):676–82. doi: 10.1097/00000542-200403000-00031
35. Owen SC, Doak AK, Ganesh AN, Nedyalkova L, McLaughlin CK, Shoichet BK, et al. Colloidal drug formulations can explain “bell-shaped” concentration-response curves. ACS Chem Biol. (2014) 9(3):777–84. doi: 10.1021/cb4007584
36. Zhu Z, Ding J, Ma Z, Iwashina T, Tredget EE. Alternatively activated macrophages derived from thp-1 cells promote the fibrogenic activities of human dermal fibroblasts. Wound Repair Regen. (2017) 25(3):377–88. doi: 10.1111/wrr.12532
37. d'Emmanuele di Villa Bianca R, Lippolis L, Autore G, Popolo A, Marzocco S, Sorrentino L, et al. Dexamethasone improves vascular hyporeactivity induced by LPS in vivo by modulating ATP-sensitive potassium channels activity. Br J Pharmacol. (2003) 140(1):91–6. doi: 10.1038/sj.bjp.0705406
38. Campbell L, Saville CR, Murray PJ, Cruickshank SM, Hardman MJ. Local arginase 1 activity is required for cutaneous wound healing. J Invest Dermatol. (2013) 133(10):2461–70. doi: 10.1038/jid.2013.164
39. Landén NX, Li D, Ståhle M. Transition from inflammation to proliferation: a critical step during wound healing. Cell Mol Life Sci. (2016) 73(20):3861–85. doi: 10.1007/s00018-016-2268-0
40. Jayme TS, Leung G, Wang A, Workentine ML, Rajeev S, Shute A, et al. Human interleukin-4-treated regulatory macrophages promote epithelial wound healing and reduce colitis in a mouse model. Sci Adv. (2020) 6(23):eaba4376. doi: 10.1126/sciadv.aba4376
41. Boniakowski AE, Kimball AS, Jacobs BN, Kunkel SL, Gallagher KA. Macrophage-mediated inflammation in normal and diabetic wound healing. J Immunol. (2017) 199(1):17–24. doi: 10.4049/jimmunol.1700223
42. Han G, Ceilley R. Chronic wound healing: a review of current management and treatments. Adv Ther. (2017) 34(3):599–610. doi: 10.1007/s12325-017-0478-y
43. Li C, Xu MM, Wang K, Adler AJ, Vella AT, Zhou B. Macrophage polarization and meta-inflammation. Transl Res. (2018) 191:29–44. doi: 10.1016/j.trsl.2017.10.004
44. Xie Y, Tolmeijer S, Oskam JM, Tonkens T, Meijer AH, Schaaf MJM. Glucocorticoids inhibit macrophage differentiation towards a pro-inflammatory phenotype upon wounding without affecting their migration. Dis Model Mech. (2019) 12(5):dmm037887. doi: 10.1242/dmm.037887
45. Sacta MA, Tharmalingam B, Coppo M, Rollins DA, Deochand DK, Benjamin B, et al. Gene-specific mechanisms direct glucocorticoid-receptor-driven repression of inflammatory response genes in macrophages. eLife. (2018) 7:e34864. doi: 10.7554/eLife.34864
46. Tu G-W, Shi Y, Zheng Y-J, Ju M-J, He H-Y, Ma G-G, et al. Glucocorticoid attenuates acute lung injury through induction of type 2 macrophage. J Transl Med. (2017) 15(1):181. doi: 10.1186/s12967-017-1284-7
47. Chatzopoulou A, Heijmans JPM, Burgerhout E, Oskam N, Spaink HP, Meijer AH, et al. Glucocorticoid-induced attenuation of the inflammatory response in zebrafish. Endocrinology. (2016) 157(7):2772–84. doi: 10.1210/en.2015-2050
48. Mehta A, Brewington R, Chatterji M, Zoubine M, Kinasewitz GT, Peer GT, et al. Infection-induced modulation of M1 and M2 phenotypes in circulating monocytes: role in immune monitoring and early prognosis of sepsis. Shock. (2004) 22(5):423–30. doi: 10.1097/01.shk.0000142184.49976.0c
49. Vettorazzi S, Bode C, Dejager L, Frappart L, Shelest E, Klaßen C, et al. Glucocorticoids limit acute lung inflammation in concert with inflammatory stimuli by induction of Sphk1. Nat Commun. (2015) 6:7796. doi: 10.1038/ncomms8796
50. De Oliveira GS, Almeida MD, Benzon HT, McCarthy RJ. Perioperative single dose systemic Dexamethasone for postoperative pain: a meta-analysis of randomized controlled trials. Anesthesiology. (2011) 115(3):575–88. doi: 10.1097/ALN.0b013e31822a24c2
Keywords: dexamethasone, dose–response curve, macrophage, sepsis, wound healing
Citation: Chen Y, Chen X and Zhou Q (2023) Different effects of a perioperative single dose of dexamethasone on wound healing in mice with or without sepsis. Front. Surg. 10:927168. doi: 10.3389/fsurg.2023.927168
Received: 23 April 2022; Accepted: 16 March 2023;
Published: 11 April 2023.
Edited by:
Gabriel Sandblom, Karolinska Institutet (KI), SwedenReviewed by:
Magnus Ågren, University of Copenhagen, DenmarkYi Liao, Affiliated Hospital of Southwest Medical University, China
© 2023 Chen, Chen and Zhou. This is an open-access article distributed under the terms of the Creative Commons Attribution License (CC BY). The use, distribution or reproduction in other forums is permitted, provided the original author(s) and the copyright owner(s) are credited and that the original publication in this journal is cited, in accordance with accepted academic practice. No use, distribution or reproduction is permitted which does not comply with these terms.
*Correspondence: Quanhong Zhou zhouanny@hotmail.com
†These authors have contributed equally to this work and share first authorship
Specialty Section: This article was submitted to Visceral Surgery, a section of the journal Frontiers in Surgery