- 1Department of Neurosurgery, IRCCS Neuromed, Pozzilli, Italy
- 2Department of Neuroscience, Sapienza University, Rome, Italy
Introduction: Neurosurgery is one of the most complex surgical disciplines where psychomotor skills and deep anatomical and neurological knowledge find their maximum expression. A long period of preparation is necessary to acquire a solid theoretical background and technical skills, improve manual dexterity and visuospatial ability, and try and refine surgical techniques. Moreover, both studying and surgical practice are necessary to deeply understand neuroanatomy, the relationships between structures, and the three-dimensional (3D) orientation that is the core of neurosurgeons' preparation. For all these reasons, a microsurgical neuroanatomy laboratory with human cadaveric specimens results in a unique and irreplaceable training tool that allows the reproduction of patients' positions, 3D anatomy, tissues' consistencies, and step-by-step surgical procedures almost identical to the real ones.
Methods: We describe our experience in setting up a new microsurgical neuroanatomy lab (IRCCS Neuromed, Pozzilli, Italy), focusing on the development of training activity programs and microsurgical milestones useful to train the next generation of surgeons. All the required materials and instruments were listed.
Results: Six competency levels were designed according to the year of residency, with training exercises and procedures defined for each competency level: (1) soft tissue dissections, bone drilling, and microsurgical suturing; (2) basic craniotomies and neurovascular anatomy; (3) white matter dissection; (4) skull base transcranial approaches; (5) endoscopic approaches; and (6) microanastomosis. A checklist with the milestones was provided.
Discussion: Microsurgical dissection of human cadaveric specimens is the optimal way to learn and train on neuroanatomy and neurosurgical procedures before performing them safely in the operating room. We provided a “neurosurgery booklet” with progressive milestones for neurosurgical residents. This step-by-step program may improve the quality of training and guarantee equal skill acquisition across countries. We believe that more efforts should be made to create new microsurgical laboratories, popularize the importance of body donation, and establish a network between universities and laboratories to introduce a compulsory operative training program.
Introduction
Neurosurgery is one of the most complex and highly demanding surgical disciplines. Intensive and long training is required to acquire a solid theoretical background, deep anatomical knowledge, hand–eye coordination, manual dexterity, and complex technical skills, in addition to controlling physiologic and psychological tremors. Young neurosurgeons must be aware of the intricate three-dimensional neuroanatomy (3D), the psychomotor abilities, the fatigue related to long operative surgeries, and accurate and safe manipulation in deep and narrow operative corridors, which can affect the surgical performance and results (1–3). For all these reasons, neurosurgical laboratories with human cadaveric specimens result in a unique and irreplaceable training tool for developing and refining anatomical knowledge, dexterity, technical skills, and surgical procedures before performing them on a living patient (4–6). The use of a real human specimen gives the possibility to reproduce the actual procedure, starting from the head positioning to the step-by-step surgery facing narrow corridors, fragile neurovascular structures, and a delicate brain surface, which can give an idea of how gentle and accurate the dissection has to be during the live surgery (4, 6, 7). Nevertheless, permanent neurosurgical laboratories are not widespread all over the world due to strict requirements and permissions, ethical and legal issues, and the high costs related to equipment, materials, and specimens. On the other hand, in the last few decades, medicolegal issues in the medical field and, in particular, neurosurgical practice have increased (8, 9). For these reasons, neurosurgical training has become crucial, with increasing interest in it, to train the next generation of neurosurgeons before practicing on living patients to reduce perioperative complications (10, 11). In this scenario, in recent years, the European Union has increased its financial support to settle up new anatomical and surgical laboratories, and, in parallel, the Italian government has unlocked some restrictions on body donation (12, 13). Convinced of the irreplaceable value of a cadaver lab, in this article we describe our experience in setting up a new microsurgical neuroanatomy lab (IRCCS Neuromed, Pozzilli, Italy), focusing on the development of training activity programs and microsurgical milestones useful to train the next generation of surgeons.
Materials and methods
We set up a microsurgical neuroanatomy laboratory, “Laboratorio di neuroanatomia G. Cantore” (Centro di Medicina Necroscopica-Unità di Chirurgia Formativa), at Parco Tecnologico IRCCS Neuromed in Pozzilli (Italy), according to the Italian requirements (Law no. 10, 10 February 2020; G.U., 4 March 2020). The laboratory and all its activities on human and animal specimens were approved by the ethical committee of IRCCS Neuromed. Embalmed and latex-injected human cadaveric heads have been used for dissections, while the human placenta has been furnished by the Obstetrics and Gynecology Department of the Istituto Clinico Mediterraneo (ICM, Salerno, Italy) as a scientific donation after birth, and Sprague–Dawley rats were provided by the Neuromed stabularium according to the Italian law on laboratory animal welfare (D.lgs. 26/2014). The human placenta was prepared as reported elsewhere (14). A dedicated veterinarian took care of the rodents, proceeding with anesthesia, analgesia, and immobilization during the courses. All the required materials and instruments are listed in Table 1. Moreover, a permanent neuronavigation system (Treon, Medtronic) is present in the lab for measurements and anatomical verification during research activities.
The training program for neurosurgical residents has been developed considering the progressive competency acquired in each year of residency, from basic techniques and procedures to complex anatomy and approaches. Four types of dissection courses have already been organized at our laboratory for neurosurgical residents and young neurosurgeons: (a) basic techniques and approaches using the microscope; (b) the 3D exoscope; (c) transcranial and endoscopic skull base approaches; and (d) microvascular anastomosis on the human placenta and rats. Furthermore, an ongoing anatomical research activity is currently being performed.
A review of the literature on anatomy and dissection guides has been done to provide a list of “suggested references” for the preparation of trainees.
Results
Six competency levels were designed according to the year of residency (PGY, from 1 to 5): (1) soft tissue dissections, bone drilling, and microsurgical suturing; (2) basic craniotomies and neurovascular anatomy; (3) white matter dissection; (4) skull base transcranial approaches; (5) endoscopic approaches; (6) vessel preparation on placenta and rats; and (7) microanastomosis. For each level, a checklist with milestones is provided in Table 2. Figure 1 shows the organization of our lab.
Level 1: soft tissue dissections, bone drilling, and microsurgical suturing (PGY 1–2)
Level 1 represents the surgical basics for PGY1. The junior resident should know the different myocutaneous layers, their relationship with nerves and vessels, craniometric points, and differences between cortical and cancellous bones. At this level, the resident must become familiar with the Mayfield head holder, macroscopic instruments, dissection techniques, engine functioning, bone drilling, and macro and microscopic suture techniques.
Level 2: basic craniotomies and neurovascular anatomy (PGY 2–3)
Level 2 corresponds to PGY2 and PGY3. The resident should be able to perform basic craniotomies, that is, pterional, subtemporal, parasagittal, retrosigmoid, and suboccipital approaches. They must perform Sylvian and interhemispheric fissure openings and know the target anatomy exposed during each approach. The resident must become familiar with bony drilling without injuring neurovascular structures.
Level 3: white matter dissection (PGY 3–4)
Level 3 represents the anatomical knowledge of white matter for PGY3 and PGY4. Knowledge of the anatomy of the cerebral surface, white matter fibers, deep nuclei, brainstem, and cerebellum is mandatory. At this level, the resident becomes confident enough in white matter dissection to dissect the main structures.
Level 4: skull base transcranial approaches (PGY 4–5)
Level 4 represents the advanced transcranial craniotomies for skull-base pathologies. This level is addressed to PGY4 and PGY5. The resident should be able to perform those approaches and recognize the extra- and intradural anatomies. Gentle interdural dissection, bony drilling in deep and narrow fields, and cutting the tent without injuring neurovascular structures are important technical skills.
Level 5: endoscopic transnasal approaches (PGY 4–5)
Level 5 is addressed to PGY4 and PGY5 and corresponds to endoscopic transnasal approaches. The resident should learn the anatomy of the nasal cavity and its relationship with the maxillary, orbital, ethmoidal, sphenoidal, clival, and craniocervical junction (CCJ) compartments. Preparation of the nasoseptal flap and the transsphenoidal approach to the sella is the first step, followed by extended medial and lateral approaches. The resident must become familiar with the endoscope and one-hand dissection. Finally, an endoscopic mucosal suture should be attempted.
Figure 2 shows the arrangement of the stations during our microsurgical and endoscopic courses.
Level 6: vessel preparation and microanastomosis on inert materials, chicken wings, human placenta, human cadavers, and rats (PGY 5)
Level 6 is addressed to PGY 5 and represents one of the most difficult skills to acquire in neurosurgery, namely vascular anastomosis. Starting from inert materials such as gauze and silicon tubes, the resident should train in simple knots and end-to-end, end-to-side, and side-to-side microanastomoses. Figure 3 shows the organization of the stations. Chicken wings and human placenta are used to mimic the consistency of the vessels and to train with vessel dissection. As described elsewhere (14), the human placenta is prepared and injected continuously with red and blue saline solution by cannulating the umbilical arteries and veins (Figure 4). The following step is using human cadaveric heads and in vivo rats. In particular, the human specimen allows for the replication of a real surgical procedure for bypass, dissecting vessels, and performing microanastomoses in deep fields. On the other side, the in vivo rat is the most realistic model through which it is possible to simulate bloody dissection, blood pulsation, empathy, and anxiety (Figure 5).
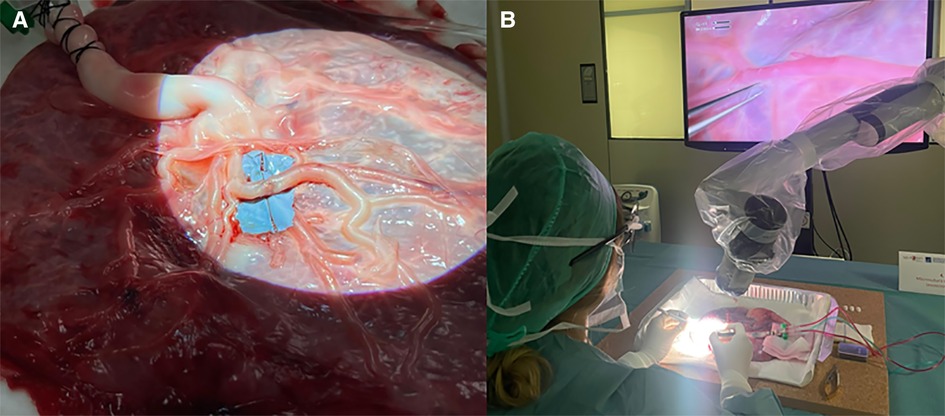
Figure 4. (A) Preparation of human placenta for microanastomosis with cannulation of the umbilical arteries and vein. After washing arteries and veins with clean water to remove blood clots, colored saline solution is used to continuously fill the vessels mimicking the blood. (B) Vascular dissection and microanastomosis on human placenta using a 3D exoscope.
Finally, in Table 3, we reported a list of some useful books and articles’ references for each level of competency to guide the residents during their studies and cadaveric dissections.
Discussion
The education and training needed to become a neurosurgeon consist of a long and demanding program that consists of acquiring a solid theoretical background and clinical and surgical experience. Residents in neurosurgery have to spend a lot of time in the operating room to become familiar with surgical anatomy and techniques and to develop practical skills (1–3). Although intraoperative surgical exposure represents the fundamental way to train surgeons, several drawbacks are encountered in every country. Differences between departments, hospitals, and nations are wide, and residents often do not have the same opportunity in their education and operation room in particular (101–107). Considering the European centers, recent surveys among neurosurgical residents report very low satisfaction rates for the theoretical and practical aspects of training in some countries (101). Moreover, novel regulations on reducing working hours for residents reduce the possibilities for practical and psychophysical training, affecting the quality of the residency and prolonging the learning curve (108, 109). These controversial aspects are also critical for the increasing medicolegal issues affecting medical practice in the last few decades (8, 9). Accordingly, the impossibility to try or repeat surgical procedures makes neurosurgical training more difficult. For all these reasons, training on human cadaveric specimens results in an essential and irreplaceable tool for residents and fully-trained neurosurgeons (10). Similarly, the evolution of simulation technology applied to surgery has assumed an important role in learning anatomy, completing procedural tasks, and improving accuracy and hand–eye coordination. In recent years, 3D inert models and virtual and augmented reality have been popularized among universities and through courses to train young residents (110, 111). Nevertheless, the use of a real human specimen gives the possibility to reproduce the real procedure starting from the head positioning to the step-by-step surgery facing narrow corridors, fragile neurovascular structures, and a delicate brain surface that can give an idea of how gentle and accurate the dissection has to be during the live surgery. Last but not least, training on human cadavers differs from inert materials or simulation tools because the trainer must also manage the emotional counterpart, empathy, and respect for the human body that can approximate real surgery.
Laboratory setup
After the introduction in Italy of Law no. 10 of 10 February 2020 entitled “Rules regarding the disposition of one's body and postmortem tissues for study, training, and scientific research purposes,” our Neurosurgical Department and IRCCS Neuromed have worked together to set up a new microsurgical neuroanatomy laboratory called “Laboratorio di neuroanatomia G. Cantore” (Centro di Medicina Necroscopica—Unità di Chirurgia Formativa) at Parco Tecnologico Neuromed in Pozzilli (Italy). Finally, our laboratory has been accredited as a no-profit laboratory for body donation with other centers in Italy. This lab has been created based on our previous experiences with neurosurgical dissections in several laboratories around the world to offer educational opportunities and training activities for residents and neurosurgeons from Italy and abroad and establish research fellowships and permanent surgical research. After the initial funding for no-profit research, a policy of reuse has been applied in our no-profit lab. The necessary equipment has been found through the recovery of disused microscopes and instruments, a donation from retired surgeons, and second-hand instruments left in the lab after courses. Expired or nonsterile materials are also routinely brought into the operative room (OR) of our hospital.
For research fellows (two for each semester) and residents, a “neurosurgical booklet,” represented in Table 2 as a checklist, is furnished to give a progressive training guide. The concept is to take all the time to perform slow and gentle step-by-step dissection to preserve tissues and understand the 3D surgical anatomy and intricate relationships between structures. Meticulous work and a sound state of mind are essential to preparing the fellow for the accuracy necessary to be a neurosurgeon. Moreover, it is essential to acquire strong neuroanatomical knowledge through transcranial and endoscopic dissections to understand the anatomy from different perspectives directly on the specimen, which is the basis for performing their research.
In parallel, fellows can observe surgical procedures to treat skull base pathologies, gliomas, or epilepsy in our department, taking inspiration to learn more about a procedure directly on the specimen or to develop new anatomical research or approaches.
Courses
During the first year of activity, we organize six courses for neurosurgical residents and young neurosurgeons, ranging from basic to more complex: (a) basic techniques and approaches using the microscope and (b) the exoscope, (c) transcranial and endoscopic skull base approaches, and (d) microvascular anastomosis on the human placenta and rats. The costs for human specimens and organization are supported by sponsors and participation fees. Each specimen preserved in a solution of alcohol, water, and softener can be reused for different courses, lowering the final cost. Considering the aforementioned checklist (Table 2), we have organized these courses taking into account the year of residency and related neurosurgical milestones. Each course included theoretical lectures and practical lab sessions.
Basic techniques and approaches
The goal of these courses is to introduce the young resident to the basics of neurosurgery. Milestones of these courses include soft tissue dissection preserving the facial nerve and superficial temporal artery (STA), drilling techniques, basic craniotomies, sinus exposure, dura opening, interhemispheric and Sylvian fissure dissections, relevant intradural anatomy, and dura closure with a patch. During these courses, residents become familiar with instruments and techniques, can perform more common approaches such as pterional, parasagittal, and retrosigmoid approaches, and study surgical anatomy. Given the technological advancements in the neurosurgical field and the recent introduction of 3D exoscopes in surgical practice (112), we organize courses that not only use microscopes, but also hybrid microexoscopes and only exoscopes. The use of a 3D exoscope during a cadaveric course has gained widespread approval from trainees due to the limited presence of this technology in Italian hospitals.
Transcranial and endoscopic skull base approaches
These courses are addressed to senior residents and neurosurgeons and consist of lectures and practice on skull base anatomy, pathologies, surgical strategies, and both transcranial and endoscopic approaches. Great emphasis is given to extradural anatomy and corridors. Participants exercise accurate drilling techniques and gentle intradural dissection in narrow and deep corridors. A combination of transcranial and endoscopic approaches can provide a 360° view of anatomy.
Microvascular anastomosis on inert materials, placenta, and rats
The course is aimed at senior residents and neurosurgeons who want to learn the techniques of microsuturing and train their skills. Sutures, dedicated instruments, and step-by-step techniques are illustrated during lectures. In the lab, each participant has their own station with microscopes, instruments, and sutures (from 6.0 to 10.0). Starting from inert materials, like gauze and synthetic vessels, the participant can practice different anastomotic techniques on fresh human placenta, which simulates the vessels, arachnoid, and pia mater. The placenta is cannulated and perfused first with water and then with a colored saline solution (red for arteries and blue for veins). After practicing on ex vivo specimens and trying to perform a patent and functional anastomosis, the participant could perform it on in vivo rats under sedation. It is the most realistic model through which it is possible to simulate bloody dissection, blood pulsation, empathy, and anxiety with the aim of not killing the specimen.
Depending on specific areas of training, these presented milestones could be fine-tuned and expanded by training experts.
Universities and international networks
Considering the aforementioned drawbacks, limitations, and differences among the neurosurgical training centers, in particular, in Europe and especially in our country (101, 109), that affect the learning curve of young neurosurgeons and ultimately the quality of medical assistance, the authors believe that it is time to renovate the residency systems. As previously proposed by Stienen et al., European standard guidelines for neurosurgical training could help further harmonize training among European countries and facilitate exchange (101). As regards the last decade, great work has been done by the European and Italian Neurosurgical Societies (EANS, SINCH) to contribute to the improvement of neurosurgical education through EANS training courses, the EANS spine diploma, SINCH basic courses for young residents, and hands-on courses. Nevertheless, all these opportunities remain at the discretion of the single resident and professor, are limited in time, and are affected by economical and organizational issues. The authors believe that the same compulsory theoretical program has to be suggested for all European countries during all the years of residency, in addition to being a parallel compulsory operative training plan that sustains and reinforces the surgical training in the OR with a uniform and verified number of procedures performed by the resident. Acquiring 3D anatomical knowledge, technical skills, manual dexterity, visuospatial ability, and surgical procedures should not be a choice for a neurosurgical resident, but rather a mandatory requirement to become a neurosurgeon. For this reason, the authors believe that each resident of all residency programs must have the opportunity to spend a period during each year of their residency in a cadaveric laboratory with a precise step-by-step “neurosurgical training checklist” with progressive milestones to improve the quality of operative training and guarantee equal skill acquisition all over the world.
However, high costs, difficult human specimen acquisition, and bureaucracy issues make this goal impossible. As reported by Italian anatomists (13), although Italian law is now more permissive about body donation, the number of centers performing anatomical dissections for the benefit of medical students and residents has decreased in favor of the few universities that can afford it.
Limitations
Although human cadaveric specimens and fixed brain hemispheres are the most reliable models for reproducing anatomy and surgery on a living patient, technical limitations are not negligible. If embalmed specimens can be easily conserved, their soft tissue and brain stiffen, affecting the qualitative and quantitative study of the approach. On the contrary, fresh specimens overcome this issue, resulting in a greater similarity to the living one at the cost of limited conservation and use, increasing the costs. Second, the absence of the cerebrospinal fluid or the pathology of interest, such as tumors and vascular malformations, makes the cadaver defective and far from a real model.
Furthermore, the resident who spends some time in the laboratory should not forget that their experience in the OR is essential and irreplaceable for the emotional counterpart and the anxiety for responsibility related to the patient’s expectations.
Future directions
As neurosurgeons are involved in intense cadaveric activities, we believe that more efforts should be made to create collaboration between universities and laboratories to optimize human, material and financial resources with the aim of establishing a solid and equitable operative training program. For this purpose, the provided “neurosurgery booklet” could be a starting point to be spread among universities and hands-on courses. The usefulness of this milestone approach should be discussed in the neurosurgical community and validated through questionnaires given to faculty experts and trainees. In this regard, we are formulating dedicated surveys for each level of competency to administer to participants of our cadaveric courses and research fellows in our lab. Similarly, training protocols on cadaveric specimens could be developed for spinal and peripheral neurosurgery. Although the higher costs of specimens and instrumentation could limit the possibilities, permanent laboratories for spinal and peripheral nerve surgery should be developed.
On another aspect, despite the great knowledge of neuroanatomy and surgical advances in the last few decades, descriptive neurosurgical anatomy is continually evolving through multidisciplinary description, new surgical corridors, and the improvement of minimally invasive skull base approaches (15). In this scenario, cadaver laboratories play a fundamental role in enhancing neuroanatomical knowledge and surgical outcomes. Different skull base research projects could be performed in the lab: description of anatomy with the integration of radiological examinations, development of three-dimensional models for surgical and training purposes, description of minimally invasive corridors, and comparison between surgical approaches. For this reason, a permanently equipped laboratory with research fellows is imperative.
Conclusion
Microsurgical dissection of human cadaveric specimens is the optimal way to learn and train on neuroanatomy and neurosurgical procedures before safely performing them in the operating room. The authors believe that the neurosurgical preparation has to be integrated with a compulsory operative training program as a complementary activity during all the years of residency. The goals are acquiring three-dimensional anatomical knowledge, technical skills, manual dexterity, visuospatial ability, and surgical procedures. We provided a “neurosurgery training checklist” with progressive milestones for neurosurgical residents. This step-by-step operational program may improve the quality of training and guarantee equal skill acquisition across countries. We believe that more efforts should be made to create new microsurgical laboratories, popularize the importance of body donation, and encourage collaboration between universities and laboratories.
Data availability statement
The original contributions presented in the study are included in the article/Supplementary Material; further inquiries can be directed to the corresponding author.
Ethics statement
The animal study was reviewed and approved by the Ethics Committee of IRCCS Neuromed.
Author contributions
Conception and design: AF, PdR, and VE. Data: AF, PdR, NG, MDA, and VE. Drafting: AF. Critical revision of the article: PdR, NG, MDA, and VE. All authors contributed to the article and approved the submitted version.
Funding
This study was supported by the Ministero della Salute (Ricerca Corrente 2023).
Conflict of interest
The authors declare that the research was conducted in the absence of any commercial or financial relationships that could be construed as a potential conflict of interest.
Publisher's note
All claims expressed in this article are solely those of the authors and do not necessarily represent those of their affiliated organizations, or those of the publisher, the editors and the reviewers. Any product that may be evaluated in this article, or claim that may be made by its manufacturer, is not guaranteed or endorsed by the publisher.
References
1. Yaşargil MG. A legacy of microneurosurgery: memoirs, lessons, and axioms. Neurosurgery. (1999) 45(5):1025–92. doi: 10.1097/00006123-199911000-00014
2. Yadav YR, Parihar V, Ratre S, Kher Y, Iqbal M. Microneurosurgical skills training. J Neurol Surg A Cent Eur Neurosurg. (2016) 77(2):146–54. doi: 10.1055/s-0034-1376190
3. Belykh E, Onaka NR, Abramov IT, Yağmurlu K, Byvaltsev VA, Spetzler RF, et al. Systematic review of factors influencing surgical performance: practical recommendations for microsurgical procedures in neurosurgery. World Neurosurg. (2018) 112:e182–207. doi: 10.1016/j.wneu.2018.01.005
4. Aboud E, Al-Mefty O, Yaşargil MG. New laboratory model for neurosurgical training that simulates live surgery. J Neurosurg. (2002) 97(6):1367–72. doi: 10.3171/jns.2002.97.6.1367
5. Moon K, Filis AK, Cohen AR. The birth and evolution of neuroscience through cadaveric dissection. Neurosurgery. (2010) 67(3):710–99. doi: 10.1227/01.NEU.0000383135.92953.A3
6. Matsushima T, Matsushima K, Kobayashi S, Lister JR, Morcos JJ. The microneurosurgical anatomy legacy of Albert L. Rhoton Jr. MD: an analysis of transition and evolution over 50 years. J Neurosurg. (2018) 129(5):1331–41. doi: 10.3171/2017.7.JNS17517
7. Hernesniemi J, Niemelä M, Karatas A, Kivipelto L, Ishii K, Rinne J, et al. Some collected principles of microneurosurgery: simple and fast, while preserving normal anatomy: a review. Surg Neurol. (2005) 64(3):195–200. doi: 10.1016/j.surneu.2005.04.031
8. Steele L, Mukherjee S, Stratton-Powell A, Anderson I, Timothy J. Extent of medicolegal burden in neurosurgery: an analysis of the national health service litigation authority database. Br J Neurosurg. (2015) 29(5):622–9. doi: 10.3109/02688697.2015.1054362
9. Lefever D, Esfahani DR, Kandregula S, Trosclair K, Demand A, Vega A, et al. The medical legal environment in neurosurgery: an informative overview of the stages of litigation and distinct challenges. World Neurosurg. (2021) 151:370–4. doi: 10.1016/j.wneu.2021.03.039
10. Bernardo A. Establishment of next-generation neurosurgery research and training laboratory with integrated human performance monitoring. World Neurosurg. (2017) 106:991–1000. doi: 10.1016/j.wneu.2017.06.160
11. Hoz SS, Al-Sharshahi ZF, Esene IN, Al-Awadi OM, Salih HR, Alrawi MA, et al. More laboratory simulations—fewer brain complications: prospects from the first neurosurgery laboratory in Iraq. World Neurosurg. (2022) 157:30–4. doi: 10.1016/j.wneu.2021.09.087
12. McHanwel S, Brenner E, Chirculescu ARM, Drukker J, van Mameren H, Mazzotti G, et al. The legal and ethical framework governing body donation in Europe: a review of current practice and recommendations for good practice. Eur J Anat. (2008) 12(1):1–24.
13. De Caro R, Boscolo-Berto R, Artico M, Bertelli E, Cannas M, Cappello F, et al. The Italian law on body donation: a position paper of the Italian college of anatomists. Ann Anat. (2021) 238:151761. doi: 10.1016/j.aanat.2021.151761
14. Del Maestro M, Rampini AD, Mauramati S, Giotta Lucifero A, Bertino G, Occhini A, et al. Dye-perfused human placenta for vascular microneurosurgery training: preparation protocol and validation testing. World Neurosurg. (2021) 146:e854–64. doi: 10.1016/j.wneu.2020.11.034
15. Meybodi AT, Benet A. Cerebrovascular anatomy. Neurosurg Clin NA. (2022) 33(4):505–15. doi: 10.1016/j.nec.2022.05.003
16. Rhoton AL Jr. The anterior and middle cranial base. Neurosurgery. (2002) 51(4 Suppl):S273–302. PMID: 12234451. doi: 10.1227/01.NEU.0000028087.76279.41
17. Rhoton AL Jr. The orbit. Neurosurgery. (2002) 51(4 Suppl):303–34. PMID: 12234452. doi: 10.1227/01.NEU.0000028329.38986.47
18. Rhoton AL. Jugular foramen: The posterior cranial fossa: Microsurgical anatomy and surgical approaches. Neurosurgery. (2007) 47 (Suppl. 3), S267–85. doi: 10.1227/01.NEU.0000296227.70319.6E
19. Rhoton AL Jr. Tentorial incisura. Neurosurgery. (2000) 47(3 Suppl):S131–53. doi: 10.1097/00006123-200009001-00015. PMID: 10983307.10983307
20. Rhoton J. The posterior cranial fossa: microsurgical anatomy and surgical approaches. Neurosurgery. (2000) 47(3 Suppl):5–6. doi: 10.1097/00006123-200009001-00005
21. Rhoton AL Jr. Cerebellum and fourth ventricle. Neurosurgery. (2000) 47(3 Suppl):S7–27. doi: 10.1097/00006123-200009001-00007. PMID: 10983303.10983303
22. Rhoton AL Jr. The cerebellar arteries. Neurosurgery. (2000) 47(3 Suppl):S29–68. doi: 10.1097/00006123-200009001-00010. PMID: 10983304.10983304
23. Rhoton AL Jr. The posterior fossa veins. Neurosurgery. (2000) 47(3 Suppl):S69–92. doi: 10.1097/00006123-200009001-00012. PMID: 10983305.10983305
24. Rhoton AL Jr. The posterior fossa cisterns. Neurosurgery. (2000) 47(3 Suppl):S287–97. doi: 10.1097/00006123-200009001-00029. PMID: 10983312.10983312
25. Rhoton AL Jr. The foramen magnum. Neurosurgery. (2000) 47(3):S155–93. doi: 10.1097/00006123-200009001-00017. PMID: 10983308.10983308
26. Rhoton AL Jr. The supratentorial arteries. Neurosurgery. (2002) 51(4 Suppl):S53–120. doi: 10.1227/01.NEU.0000028484.12422.23. PMID: 12234447.12234447
27. Rhoton AL Jr. The cerebral veins. Neurosurgery (2002) 51(4 Suppl):S159–205. doi: 10.1227/01.NEU.0000028228.51706.2D. PMID: 12234449.12234449
28. Rhoton AL Jr. The lateral and third ventricles. Neurosurgery. (2002) 51(4 Suppl):S207–71. doi: 10.1227/01.NEU.0000028161.91504.4F. PMID: 12234450.12234450
29. Rhoton AL Jr. The sellar region. Neurosurgery. (2002) 51(4 Suppl):S335–74. doi: 10.1227/01.NEU.0000028680.51299.00. PMID: 12234453.12234453
30. Rhoton AL. The cavernous sinus, the cavernous venous plexus, and the carotid collar. Neurosurgery. (2002) 51:375–410. doi: 10.1227/01.NEU.0000028833.01529.E7
31. Rhoton AL. The temporal bone and transtemporal approaches. Neurosurgery. (2000) 47(3):S211–65. doi: 10.1097/00006123-200009001-00023
32. Ammirati M, Bernardo A. Anatomical study of the superior orbital fissure as seen during a pterional approach. J Neurosurg. (2007) 106(1):151–6. doi: 10.3171/jns.2007.106.1.151
33. Chaddad-Neto F, Filho JMC, Dória-Netto HL, Faria MH, Ribas GC, Oliveira E. The pterional craniotomy: tips and tricks. Arq Neuropsiquiatr. (2012) 70(9):727–32. doi: 10.1590/S0004-282X2012000900015
34. Coscarella E, Vishteh AG, Spetzler RF, Seoane E, Zabramski JM. Subfascial and submuscular methods of temporal muscle dissection and their relationship to the frontal branch of the facial nerve. Technical note. J Neurosurg. (2000) 92(5):877–80. doi: 10.3171/jns.2000.92.5.0877
35. Oikawa S, Mizuno M, Muraoka S, Kobayashi S. Retrograde dissection of the temporalis muscle preventing muscle atrophy for pterional craniotomy: technical note. J Neurosurg. (1996) 84(2):297–9. doi: 10.3171/jns.1996.84.2.0297
36. Kadri PAS, Al-Mefty O. The anatomical basis for surgical preservation of temporal muscle. J Neurosurg. (2004) 100(3):517–22. doi: 10.3171/jns.2004.100.3.0517
37. Poblete T, Jiang X, Komune N, Matsushima K, Rhoton AL. Preservation of the nerves to the frontalis muscle during pterional craniotomy. J Neurosurg. (2015) 122(6):1274–82. doi: 10.3171/2014.10.JNS142061
38. Campero A, Villalonga JF, Elizalde RL, Ajler P. The nuchal lines as anatomic landmarks to dissect the muscles in the far lateral approach. World Neurosurg. (2018) 113:188–94. doi: 10.1016/j.wneu.2018.02.090
39. Rhoton J. The far-lateral approach and its transcondylar, supracondylar, and paracondylar extensions. Neurosurgery. (2000) 47(3 Suppl). doi: 10.1097/00006123-200009001-00020
40. Ribas GC, Rhoton AL, Cruz OR, Peace D. Suboccipital burr holes and craniectomies. Neurosurg Focus. (2005) 19(2):1–12. doi: 10.3171/foc.2005.19.2.2
41. Rhoton AL. The cerebellopontine angle and posterior fossa cranial nerves by the retrosigmoid approach. Neurosurgery. (2000) 47(Suppl 3):S93–129. doi: 10.1097/00006123-200009001-00013
42. Ugur HC, Dogan I, Kahilogullari G, Al-Beyati ESM, Ozdemir M, Kayaci S, et al. New practical landmarks to determine sigmoid sinus free zones for suboccipital approaches: an anatomical study. J Craniofac Surg. (2013) 24(5):1815–8. doi: 10.1097/SCS.0b013e3182997ff8
43. Savardekar A, Nagata T, Kiatsoontorn K, Terakawa Y, Ishibashi K, Goto T, et al. Preservation of labyrinthine structures while drilling the posterior wall of the internal auditory canal in surgery of vestibular schwannomas via the retrosigmoid suboccipital approach. World Neurosurg. (2014) 82(3):474–9. doi: 10.1016/j.wneu.2014.02.029
44. Raso JL, Silva Gusmão SN. A new landmark for finding the sigmoid sinus in suboccipital craniotomies. Neurosurgery. (2011) 68(Suppl 1):1–6. doi: 10.1227/NEU.0b013e3182082afc
45. Sampath P, Rini D, Long DM. Microanatomical variations in the cerebellopontine angle associated with vestibular schwannomas (acoustic neuromas): a retrospective study of 1006 consecutive cases. J Neurosurg. (2000) 92(1):70–8. doi: 10.3171/jns.2000.92.1.0070
46. Giammattei L, Starnoni D, Benes V, Froelich S, Cossu G, Borsotti F, et al. Extreme lateral supracerebellar infratentorial approach: surgical anatomy and review of the literature. World Neurosurg. (2021) 147:89–104. doi: 10.1016/j.wneu.2020.12.042
47. Campero A, Tróccoli G, Martins C, Fernandez-Miranda JC, Yasuda A, Rhoton ALJ. Microsurgical approaches to the medial temporal region: an anatomical study. Neurosurgery. (2006) 59(4 Suppl 2), discussion ONS307-8:ONS279-307, doi: 10.1227/01.NEU.0000223509.21474.2E.17041498
48. Voets NL, Bartsch A, Plaha P. Brain white matter fibre tracts: a review of functional neuro-oncological relevance. J Neurol Neurosurg Psychiatry. (2017) 88(12):1017–25. doi: 10.1136/jnnp-2017-316170
49. Tanriover N, Rhoton AL, Kawashima M, Ulm AJ, Yasuda A. Microsurgical anatomy of the insula and the Sylvian fissure. J Neurosurg. (2004) 100(5):891–922. doi: 10.3171/jns.2004.100.5.0891
50. Türe U, Yaşargil MG, Friedman AH, Al-Mefty O. Fiber dissection technique: lateral aspect of the brain. Neurosurgery. (2000) 47(2):417–26; discussion 426-7. doi: 10.1097/00006123-200008000-00028. PMID: 10942015.
51. Costa M, Braga VL, Yağmurlu K, Centeno RS, Cavalheiro S, Chaddad-Neto F. A technical guide for fiber tract dissection of the internal capsule. Turk Neurosurg. (2018) 28(6):934–9. doi: 10.5137/1019-5149.JTN.20884-17.1
52. Sincoff EH, Tan Y, Abdulrauf SI. White matter fiber dissection of the optic radiations of the temporal lobe and implications for surgical approaches to the temporal horn. J Neurosurg. (2004) 101(5):739–46. doi: 10.3171/jns.2004.101.5.0739
53. Serra C, Türe U, Krayenbühl N, Şengül G, Yaşargil DCH, Yaşargil MG. Topographic classification of the thalamus surfaces related to microneurosurgery: a white matter fiber microdissection study. World Neurosurg. (2017) 97:438–52. doi: 10.1016/j.wneu.2016.09.101
54. Martino J, Brogna C, Robles SG, Vergani F, Duffau H. Anatomic dissection of the inferior fronto-occipital fasciculus revisited in the lights of brain stimulation data. Cortex. (2010) 46(5):691–9. doi: 10.1016/j.cortex.2009.07.015
55. Kadri PAS, de Oliveira JG, Krayenbühl N, Türe U, de Oliveira EPL, Al-Mefty O, et al. Surgical approaches to the temporal horn: an anatomic analysis of white matter tract interruption. Oper Neurosurg. (2017) 13(2):258–70. doi: 10.1093/ons/opw011
56. Flores-Justa A, Baldoncini M, Pérez Cruz JC, Sánchez Gonzalez F, Martínez OA, González-López P, et al. White matter topographic anatomy applied to temporal lobe surgery. World Neurosurg. (2019) 132:e670–9. doi: 10.1016/j.wneu.2019.08.050
57. Fernández-Miranda JC, Rhoton ALJ, Kakizawa Y, Choi C, Alvarez-Linera J. The claustrum and its projection system in the human brain: a microsurgical and tractographic anatomical study. J Neurosurg. (2008) 108(4):764–74. doi: 10.3171/JNS/2008/108/4/0764
58. Di Carlo DT, Benedetto N, Duffau H, Cagnazzo F, Weiss A, Castagna M, et al. Microsurgical anatomy of the sagittal stratum. Acta Neurochir. (2019) 161(11):2319–27. doi: 10.1007/s00701-019-04019-8
59. Campero A, Tróccoli G, Martins C, Fernandez-Miranda JC, Yasuda A, Rhoton AL Jr. Microsurgical approaches to the medial temporal region: an anatomical study. Neurosurgery. (2006) 59(4 Suppl 2):ONS279–307; discussion ONS307-8. doi: 10.1227/01.NEU.0000223509.21474.2E. PMID: 17041498.17041498
60. Sarubbo S, De Benedictis A, Maldonado IL, Basso G, Duffau H. Frontal terminations for the inferior fronto-occipital fascicle: anatomical dissection, DTI study and functional considerations on a multi-component bundle. Brain Struct Funct. (2013) 218(1):21–37. doi: 10.1007/s00429-011-0372-3
61. García-Feijoo P, Reghin-Neto M, Holanda V, Rassi MS, Saceda-Gutierrez JM, Carceller-Benito FE, et al. 3-step didactic white matter dissection of human cerebellum: micro-neuroanatomical training. Neurocirugia. (2022) 33(2):61–70. doi: 10.1016/j.neucie.2021.04.003
62. Capilla-Guasch P, Quilis-Quesada V, Regin-Neto M, Holanda VM, González-Darder JM, de Oliveira E. White matter relationships examined by transillumination technique using a lateral transcortical parietal approach to the atrium: three-dimensional images and surgical considerations. World Neurosurg. (2019) 132:e783–94. doi: 10.1016/j.wneu.2019.08.018
63. Ribas GC. The cerebral sulci and gyri. Neurosurg Focus. (2010) 28(2):E2. doi: 10.3171/2009.11.FOCUS09245
64. Ribas GC, Yasuda A, Ribas EC, Nishikuni K, Rodrigues AJ. Surgical anatomy of microneurosurgical sulcal key points. Neurosurgery. (2006) 59(4 Suppl 2):177–211. doi: 10.1227/01.NEU.0000240682.28616.b2
65. Párraga RG, Ribas GC, Welling LC, Alves RV, De Oliveira E. Microsurgical anatomy of the optic radiation and related fibers in 3-dimensional images. Neurosurgery. (2012) 71(Suppl 1):160–72. doi: 10.1227/NEU.0b013e3182556fde
66. Peltier J, Verclytte S, Delmaire C, Pruvo JP, Godefroy O, Le Gars D. Microsurgical anatomy of the temporal stem: clinical relevance and correlations with diffusion tensor imaging fiber tracking—laboratory investigation. J Neurosurg. (2010) 112(5):1033–8. doi: 10.3171/2009.6.JNS08132
67. Goga C, Türe U. The anatomy of Meyer's loop revisited: changing the anatomical paradigm of the temporal loop based on evidence from fiber microdissection. J Neurosurg. (2015) 122:1253–62. doi: 10.3171/2014.12.JNS14281. PMID: 25635481.25635481
68. Cristina G, Klara BISF, Mena RR. The three-dimensional architecture of the internal capsule of the human brain demonstrated by fiber dissection technique. ARS Medica Tomitana. (2015) 20(3):115–22. doi: 10.2478/arsm-2014-0021
69. Bozkurt B, da Silva Centeno R, Chaddad-Neto F, da Costa MDS, Goiri MAA, Karadag A, et al. Transcortical selective amygdalohippocampectomy technique through the middle temporal gyrus revisited: an anatomical study laboratory investigation. J Clin Neurosci. (2016) 34:237–45. doi: 10.1016/j.jocn.2016.05.035
70. Tanriover N, Ulm AJ, Rhoton AL, Kawashima M, Yoshioka N, Lewis SB. One-piece versus two-piece orbitozygomatic craniotomy: quantitative and qualitative considerations. Neurosurgery. (2006) 58(Suppl 2):229–37. doi: 10.3171/jns.2004.100.5.0891
71. Shimizu S, Tanriover N, Rhoton AL, Yoshioka N, Fujii K. Maccarty keyhole and inferior orbital fissure in orbitozygomatic craniotomy. Neurosurgery. (2005) 57(1 Suppl):152–9. doi: 10.1227/01.NEU.0000163600.31460.D8
72. Froelich S, Aziz KA, Levine NB, Tew JM, Keller JT, Theodosopoulos PV. Extension of the one-piece orbitozygomatic frontotemporal approach to the glenoid fossa: cadaveric study. Neurosurgery. (2008) 62(5 Suppl 2):312–7. doi: 10.1227/01.NEU.0000297104.57378.72
73. Aziz KM, Froelich SC, Cohen PL, Sanan A, Keller JT, van Loveren HR. The one-piece orbitozygomatic approach: the Maccarty burr hole and the inferior orbital fissure as keys to technique and application. Acta Neurochir (Wien). 2002 144(1):15–24. doi: 10.1007/s701-002-8270-1. PMID: 11807643.11807643
74. Alaywan M, Sindou M. Fronto-temporal approach with orbito-zygomatic removal surgical anatomy. Acta Neurochir. (1990) 104(3–4):79–83. doi: 10.1007/BF01842824
75. Cappabianca P, Califano L, Iaconetta G. Cranial, craniofacial and skull base surgery (2010) Cranial, Craniofacial and Skull Base Surgery (pp. 1–350). Springer Milan. https://doi.org/10.1007/978-88-470-1167-0
76. Noguchi A, Balasingam V, Shiokawa Y, McMenomey SO, Delashaw JB. Extradural anterior clinoidectomy. Technical note. J Neurosurg. (2005) 102(5):945–50. doi: 10.3171/jns.2005.102.5.0945
77. Froelich SC, Aziz KMA, Levine NB, Theodosopoulos PV, Van Loveren HR, Keller JT. Refinement of the extradural anterior clinoidectomy: surgical anatomy of the orbitotemporal periosteal fold. Neurosurgery. (2007) 61(5 Suppl 2):179–86. doi: 10.1227/01.neu.0000303215.76477.cd
78. Coscarella E, Ba¸kaya MK, Morcos JJ, Rosenblatt S, Ciric IS, Sekhar LN, et al. An alternative extradural exposure to the anterior clinoid process: the superior orbital fissure as a surgical corridor. Neurosurgery. (2003) 53(1):162–7. doi: 10.1227/01.NEU.0000068866.22176.07
79. Fukuda H, Evins AI, Burrell JC, Iwasaki K, Stieg PE, Bernardo A. The meningo-orbital band: microsurgical anatomy and surgical detachment of the membranous structures through a frontotemporal craniotomy with removal of the anterior clinoid process. J Neurol Surg B Skull Base. (2014) 75(2):125–32. doi: 10.1055/s-0033-1359302
80. Fava A, di Russo P, Giammattei L, Froelich S. Endoscopic-assisted microsurgical resection of right recurrent Meckel’s cave meningioma extended to cavernous Sinus. J Neurol Surg B Skull Base. (2022) 83(Suppl 3):e632–4. doi: 10.1055/s-0041-1725934
81. Aziz KM, van Loveren HR, Tew JMJ, Chicoine MR. The Kawase approach to retrosellar and upper clival basilar aneurysms. Neurosurgery. (1999) 44(6):1225–6.10371621
82. Diaz Day J. The middle fossa approach and extended middle fossa approach: technique and operative nuances. Neurosurgery. (2012) 70(2 Suppl):192–201. doi: 10.1227/NEU.0b013e31823583a1
83. Fava A, di Russo P, Passeri T, Camara B, Paglia F, Matano F, et al. The mini-combined transpetrosal approach: an anatomical study and comparison with the combined transpetrosal approach. Acta Neurochir (Wien). (2022) 164(4):1079–93. doi: 10.1007/s00701-022-05124-x. PMID: 35230553. 35230553
84. Giammattei L, Passeri T, Abbritti R, Lieber S, Matano F, Le Van T, et al. Surgical morbidity of the extradural anterior petrosal approach: the Lariboisière experience. J Neurosurg. (2023) 138(1):276–86. doi: 10.3171/2022.3.JNS212962
85. Roche P-H, Lubrano VF, Noudel R. How I do it: epidural anterior petrosectomy. Acta Neurochir. (2011) 153(6):1161–7. doi: 10.1007/s00701-011-1010-9
86. Sincoff EH, McMenomey SO, Delashaw JB Jr. Posterior transpetrosal approach: less is more. Neurosurgery. (2007) 60(2 Suppl 1):ONS53-8; discussion ONS58-9. doi: 10.1227/01.NEU.0000249232.12860.A5. PMID: 17297365.17297365
87. Luzzi S, Giotta Lucifero A, Bruno N, Baldoncini M, Campero A, Galzio R. Far lateral approach. Acta Biomed. (2021) 92(2):e2021352. doi: 10.23750/abm.v92iS4.12823. PMID: 35441601; PMCID: PMC9179055.
88. Fava A, di Russo P, Tardivo V, Passeri T, Câmara B, Penet N, et al. Endoscope-assisted far-lateral transcondylar approach for craniocervical junction chordomas: a retrospective case series and cadaveric dissection. J Neurosurg. (2021):1–12. doi: 10.3171/2020.9.JNS202611. [Epub ahead of print]33799304
89. Giammattei L, di Russo P, Penet N, Froelich S. Endoscope-assisted anterolateral approach to a recurrent cervical spinal chordoma. Acta Neurochir. (2020) 162(2):443–7. doi: 10.1007/s00701-019-04194-8
90. Di Carlo DT, Voormolen EH, Passeri T, Champagne P-O, Penet N, Bernat AL, et al. Hybrid antero-lateral transcondylar approach to the clivus: a laboratory investigation and case illustration. Acta Neurochir. (2020) 162(6):1259–68. doi: 10.1007/s00701-020-04343-4
91. George B, Lot G, Sen C. Anterolateral and posterolateral approaches to the foramen magnum: technical description and experience from 97 cases. Skull Base Surg. (1995) 5(1):9–19. doi: 10.1055/s-2008-1058945
92. Cappabianca P, Cavallo LM, de Divitiis O, Esposito F. Midline skull base surgery. (2016):1–373. doi: 10.1007/978-3-319-21533-4. ISBN 978-3-319-21532-7
93. Stamm AC. Transnasal endoscopic skull base and brain surgery. (2019). Ed 2. (p. 1–690). Thieme Medical Publishers. ISBN 9781626237100
94. Kassam AB, Thomas A, Carrau RL, Snyderman CH, Vescan A, Prevedello D, et al. Endoscopic reconstruction of the cranial base using a pedicled nasoseptal flap. Neurosurgery. (2008) 63(1 Suppl), discussion ONS52-3. doi: 10.1227/01.neu.0000297074.13423.f5
95. Thomas R, Girishan S, Chacko AG. Endoscopic transmaxillary transposition of temporalis flap for recurrent cerebrospinal fluid leak closure. J Neurol Surg B Skull Base. (2016) 77(6):445–8. doi: 10.1055/s-0036-1581065
96. di Russo P, Fava A, Giammattei L, Passeri T, Okano A, Abbritti R, et al. The rostral mucosa: the door to open and close for targeted endoscopic endonasal approaches to the clivus. Oper Neurosurg. (2021):21(3):150–159. doi: 10.1093/ons/opab141. PMID: 34038940.
97. MacDonald JD. Learning to perform microvascular anastomosis. Skull Base. (2005) 15(3):229–40. doi: 10.1055/s-2005-872598
98. Belykh E, Lei T, Safavi-Abbasi S, Yagmurlu K, Almefty RO, Sun H, et al. Low-flow and high-flow neurosurgical bypass and anastomosis training models using human and bovine placental vessels: a histological analysis and validation study. J Neurosurg. (2016) 125(4):915–28. doi: 10.3171/2015.8.JNS151346
99. Tellioglu AT, Eker E, Cimen K, Comert A, Karaeminogullari G, Tekdemir I. Training model for microvascular anastomosis. J Craniofac Surg. (2009) 20(1):238–9. doi: 10.1097/SCS.0b013e3181843ade
100. Liu H-L. Microvascular anastomosis of submillimeter vessels—a training model in rats. J Hand Microsurg. (2013) 5(1):14–7. doi: 10.1007/s12593-013-0089-z
101. Stienen MN, Netuka D, Demetriades AK, Ringel F, Gautschi OP, Gempt J, et al. Neurosurgical resident education in Europe—results of a multinational survey. Acta Neurochir. (2016) 158(1):3–15. doi: 10.1007/s00701-015-2632-0
102. Calderon C, Dos Santos Rubio EJ, Negida A, Park KB. Neurosurgical training in the Caribbean. Brain Spine. (2022) 2:101691. doi: 10.1016/j.bas.2022.101691
103. Ekhator C, Rak R. The need for improved recruitment to neurosurgery training: a systematic review of enrollment strategies. Cureus. (2022) 14(6):e26212. doi: 10.7759/cureus.26212
104. Ferraris KP, Matsumura H, Wardhana DPW, Vesagas T, Seng K, Mohd Ali MR, et al. The state of neurosurgical training and education in east Asia: analysis and strategy development for this frontier of the world. Neurosurg Focus. (2020) 48(3):E7. doi: 10.3171/2019.12.FOCUS19814
105. Hoffman C, Härtl R, Shlobin NA, Tshimbombu TN, Elbabaa SK, Haglund MM, et al. Future directions for global clinical neurosurgical training: challenges and opportunities. World Neurosurg. (2022) 166:e404–18. doi: 10.1016/j.wneu.2022.07.030
106. Ogbu II, Kaliaperumal C. The future of neurosurgical training in the United Kingdom. World Neurosurg. (2022) 168:89–93. doi: 10.1016/j.wneu.2022.09.038
107. Wang A, Shlobin NA, DiCesare JAT, Holly LT, Liau LM. Diversity in neurosurgical recruitment and training in the United States: a systematic review. World Neurosurg. (2022) 162:111–117.e1. doi: 10.1016/j.wneu.2022.03.086
108. Cowie CJA, Pešić-Smith JD, Boukas A, Nelson RJ. Has the impact of the working time regulations changed neurosurgical trainees’ attitudes towards the European working time directive 5 years on? Br J Neurosurg. (2013) 27(5):580–5. doi: 10.3109/02688697.2013.834530
109. Maxwell AJ, Crocker M, Jones TL, Bhagawati D, Papadopoulos MC, Bell BA. Implementation of the European working time directive in neurosurgery reduces continuity of care and training opportunities. Acta Neurochir. (2010) 152(7):1207–10. doi: 10.1007/s00701-010-0648-z
110. Chawla S, Devi S, Calvachi P, Gormley WB, Rueda-Esteban R. Evaluation of simulation models in neurosurgical training according to face, content, and construct validity: a systematic review. Acta Neurochir. (2022) 164(4):947–66. doi: 10.1007/s00701-021-05003-x
111. Bernardo A. Virtual reality and simulation in neurosurgical training. World Neurosurg. (2017) 106:1015–29. doi: 10.1016/j.wneu.2017.06.140
Keywords: neurosurgical training, anatomical dissection, anatomy laboratory, cadaveric specimen, skull base technique, microanastomosis
Citation: Fava A, Gorgoglione N, De Angelis M, Esposito V and di Russo P (2023) Key role of microsurgical dissections on cadaveric specimens in neurosurgical training: Setting up a new research anatomical laboratory and defining neuroanatomical milestones. Front. Surg. 10:1145881. doi: 10.3389/fsurg.2023.1145881
Received: 16 January 2023; Accepted: 13 February 2023;
Published: 9 March 2023.
Edited by:
Giuseppe Emmanuele Umana, Cannizzaro Hospital, ItalyReviewed by:
Ali Tayebi Meybodi, Rutgers University, United StatesSamer Hoz, University of Cincinnati, United States
Jorge Lazareff, Angeles County, United States
© 2023 Fava, Gorgoglione, De Angelis, Esposito and di Russo. This is an open-access article distributed under the terms of the Creative Commons Attribution License (CC BY). The use, distribution or reproduction in other forums is permitted, provided the original author(s) and the copyright owner(s) are credited and that the original publication in this journal is cited, in accordance with accepted academic practice. No use, distribution or reproduction is permitted which does not comply with these terms.
*Correspondence: Arianna Fava arianna.f.90@gmail.com
Specialty Section: This article was submitted to Neurosurgery, a section of the journal Frontiers in Surgery