- 1Department of Orthopaedics, Hainan Hospital of Chinese PLA General Hospital, Sanya, China
- 2Department of Dermatology, Hainan Hospital of Chinese PLA General Hospital, Sanya, China
- 3Department of Anesthesiology, Wenchang People’s Hospital, Wenchang, China
- 4Senior Department of Orthopedics, The Fourth Medical Center of PLA General Hospital, Beijing, China
- 5Department of Orthopedics, The Eighth Medical Center of PLA General Hospital, Beijing, China
- 6National Clinical Research Center for Orthopedics, Sports Medicine & Rehabilitation, PLA General Hospital, Beijing, China
- 7Department of Pediatric, The First Medical Center of Chinese PLA General Hospital, Beijing, China
- 8Senior Department of Pediatric, The Seventh Medical Center of Chinese PLA General Hospital, Beijing, China
Background: Negative pressure wound therapy with instillation (NPWTi) is a novel method based on standard negative pressure wound therapy (NPWT). This study aimed to compare the effects of standard NPWT and NPWTi on bioburden and wound healing in a Staphylococcus aureus (S.aureus) infected porcine model.
Methods: Green fluorescent protein-labeled S.aureus infected wounds were created on the back of porcine. Wounds were treated with NPWT or NPWT with instillation (saline). The tissue specimens were harvested on days 0 (12 h after bacterial inoculation), 2, 4, 6, and 8 at the center of wound beds. Viable bacterial counts, laser scanning confocal microscopy, PCR, western blot, and histological analysis were performed to assess virulence and wound healing.
Results: The bacterial count in the NPWTi group was lower than that of the NPWT group and the difference was statistically significant on day 2, day 4, day 6, and day 8 (P < 0.05). The expression levels of agrA, Eap, Spa, and Hla genes of the NPWTi group were significantly lower than that of the NPWT group on day 8 (P < 0.05). The bacterial invasion depth of the NPWTi group was significantly lower than that of the NPWT group on day 2, day 4, day 6, and day 8 (P < 0.05). Though the NPWTi group showed a significantly increased expression of bFGF and VEGF than that of the NPWT group in the early time (P < 0.05), NPWTi cannot lead to better histologic parameters than the NPWT group (P > 0.05).
Conclusion: Our results demonstrated that NPWTi induced a better decrease in bacterial burden and virulence compared with standard NPWT. These advantages did not result in better histologic parameters on the porcine wound model.
Introduction
Treatment of infected wounds with soft tissue defects was very tricky. A heavy bacterial bioburden in the wound can negatively impact wound healing by stimulating a pro-inflammatory environment and encouraging the in-migration of monocytes, macrophages, and leucocytes (1). In addition, bacterial bioburden can also increase metabolic requirements and decrease blood flow to the wound, which delays wound healing (2, 3). Thus, effective control and clearance of infections are essential for normal wound healing. Staphylococcus aureus (S.aureus) is the most commonly gram-positive bacteria in infected wounds (4). S.aureus can express various virulence factors, such as extracellular adherence protein (Eap), staphylococcal protein A (Spa), and α-hemolysin (Hla) which play an important role in the pathogenesis of wound infection (5, 6). In addition, accessory gene regulator system (agr), a global regulatory system of S.aureus, plays an important role in the transcription and expression of virulence factors.
Negative pressure wound therapy (NPWT) is widely used for the management of both acute and chronic wounds (7). The benefit of NPWT has been proposed: fluid removal, macro-deformation or wound shrinkage, micro deformation at the foam-wound surface interface, and stabilization of the wound environment (8). In addition, other studies suggest that NPWT decreases edema, decreases bacterial bioburden, increases immune response, increases blood flow to the wound margins, and decreases the permeability of vessels (9–12). It has been reported that negative pressure can inhibit the growth, virulence, and biofilm formation of S.aureus and P.aeruginosa (13–15).
Negative pressure wound therapy with instillation (NPWTi) is a novel method based on standard negative pressure wound therapy, which combines NPWT and the timed delivery of topical irrigation solutions (saline, distilled water, or antimicrobials) to the wound bed (16). This method subjects the subcutaneous layer of the wound to irrigation and negative pressure, thus removing any exudates and irritants that can accumulate in the wound (17). Wolvos et al. showed that NPWTi may help with noncontaminated wound management (18). However, the possible mechanism of NPWTi on bacterial bioburden in soft tissue is unclear. Thus, we designed an animal study aimed at the comparison of the effects of NPWT and NPWTi in a porcine model of bacterial bioburden. We hypothesized that NPWTi would have beneficial effects on decreasing the number of bacteria, reducing the virulence of bacteria and the depth of bacterial invasion, which may help with wound healing.
Materials and methods
Ethical statement. All animal experiments in this study were approved by the Medical Ethics Committee of the Chinese PLA General Hospital (Beijing, China) and received humane care in compliance with the Guidelines for Care and Use of Animals in Research.
Animals. Ten healthy pigs of both sexes, with a mean body weight of 15 kg, were used for this study. All to the animals were free to access water in separate cages under constant temperature (22°C) and humidity (45%).
Bacterial strains and culture. S. aureus strain RN6390-GFP (constitutively expressing a green fluorescent protein, a gift from the People's Liberation Army Institute for Disease Control and Control) was utilized for wound inoculation. S. aureus was cultured in Luria broth at 37°C and grown overnight until the log phase. Bacteria were harvested and diluted with phosphate-buffered saline (PBS) until OD (optical density) 600 nm reached a value of 1.0, equivalent to 105 colony-forming units/µl empirically.
Wound protocol and bacterial inoculation. Pigs were anesthetized by intramuscular injection of ketamine (30 mg/kg) and midazolam (500 µg/kg). The dorsum of the animal was cleaned, treated with depilatory cream (Veet) for hair removal, sterilized twice with povidone and 70% ethanol, and covered with sterile surgical drapes. Two circular full-thickness 5 cm diameter excisional wounds to the muscle were created on the dorsum, with the epidermal, dermal, subdermal fat, and subcutaneous fat layers removed. Light pressure with sterile gauze was applied to stanch bleeding if necessary. Each wound was inoculated with 105 colony-forming units/µl S.aureus at a volume of 1 ml. Bacteria were allowed to proliferate for 12 h to ensure bacterial adhesion and colonization.
Treatment protocol. Overall, 20 acute S.aureus-contaminated wounds were created (2 wounds/pig). Five pigs were randomly assigned to the NPWT (Shandong, WEIGAO) group and the others were assigned to the NPWTi group. The wound was filled with black polyurethane foam. In the NPWT group, the foam was connected to a therapy unit set to deliver intermittent negative pressure, with each cycle consisting of 5 min at -125 mmHg followed by 2 min at 0 mmHg (19). In the NPWTi group, the treatment is programmed to cycle instill the saline for 45 s, follow by a 3 second hold time to allow the solution distribution over the wound bed, then deliver 2 h of negative pressure therapy same as the NPWT group (16). Animals were anesthetized before dressing change. Foams were checked daily and changed on postoperative days 2, 4, 6, and 8. Before dressing changes, the diameter of the wounds were measured and 5 mm punch biopsies were taken from the wound for analysis and detection. The detection was repeated 3 times. Animals were sacrificed on postoperative day 8 via over-dose of intravenous ketamine and midazolam.
Bacterial count. The tissue specimens were collected 12 h after bacterial inoculation and on postoperative days 2, 4, 6, and 8. Each specimen was weighed and homogenized under sterile conditions. The homogenates were serially diluted with sterile PBS, the concentration ranging from 10−1, 10−2 to 10−6 times, then plated on Staphylococcus Isolation Ager and incubated at 37°C for 24 h. A standard colony-counting method was conducted and the results were evaluated by a medical microbiologist. The colony-counting method was conducted and the results were expressed as the logarithm of CFUs/wound (20).
Quantitative RT-PCR. The primer sequences used in this study were listed in Table 1. Total RNA was extracted using an RNA prep Pure Kit (TIANGEN, China) according to the manufacturer's instructions. Total RNA was treated with Recombinant DNase I (TAKARA, Japan) and reverse-transcribed using the TIANScript RT Kit (TIANGEN) according to the manufacturer's instructions. Real-time PCR analyses using the SYBR FAST qPCR Kit Master Mix Universal (KAPA, United States) were performed with an ABI7900HT sequence detection system (ABI, United States). The reaction procedures were as follows: incubation at 95°C for 3 min, and then 40 cycles at 95°C for 3 s, 60°C for the 20 s, and one dissociation step at 95°C for 15 s, 60°C for 15 s, and 95 for 15 s. All samples were analyzed in triplicate and normalized against housekeeping gene β-actin expression.
Western blot analysis. The tissue specimens harvested above were homogenized and incubated on ice for 30 min in the presence of RadioImmuno Precipitation Assay (RIPA) buffer. Supernatants were collected by centrifugation at 13,000 rpm for 30 min (4°C). 25 ul of supernatant was loaded on 8% SDS-PAGE gels. Western Blot analysis for the detection of Eap, Spa, and a-toxin in wound extracts was performed as previously described (21). Antibodies for the detection of Eap, Spa, and a-toxin were purchased from Abcam.
Measurement of bacterial invasion depth. The muscle specimens were cut into 6 μm thick sections with the use of a cryostat and mounted on glass slides for viewing using an argon confocal laser scanning microscope (Olympus FV1000, Tokyo, Japan). S. aureus (constitutively expressing green fluorescent protein) was green under Olympus FV1000. To determine the invasion depth of S.aureus from the tissue boundary to the deepest location, digital images were captured with the speed set at 4 ms/pixel and measured by two blinded independent observers. The depths of the bacterial fluorescent signal from samples quantified by FV10-ASW 4.1 software embedded on Olympus FV-1,000 (Figure 1).
Histological analysis. Specimens were fixed in 10% neutral formalin. Samples were then embedded in paraffin, cut into 5 um sections, and stained for analysis. Hematoxylin and eosin (H & E) staining, immunohistochemistry (CD31), and Masson staining were performed respectively for further analysis. Image-Pro Plus version 6.0 software (Media Cybernetics, United States) was used to quantify the area of neovascularization and new granulation thickness.
Statistical analysis. Time course measurements were analyzed by two-way analysis of variance with repeated measures. To calculate significant differences at each time point between the two groups, a Student's t-test was applied. The statistical analysis was performed using SPSS software. Significant differences were defined at P < 0.05.
Results
Bacterial count
Before treatment, the bacterial count was 107 CFUs of g tissue in both groups (Figure 2). In the two groups, the count of bacteria decreased from postoperative day 2, and there was a significant difference between the two groups. In the NPWTi group, the bacterial count was 105 CFUs of g tissue on postoperative day 4, and less than 105 CFUs of g tissue on postoperative days 6 and 8. In the NPWT group, the bacterial count was less than 105 CFUs of g tissue only on postoperative day 8. Applying a two-sided t-test, the numbers of bacteria in the NPWT group compared with the NPWTi group was significantly different on a postoperative day 2, 4, 6, 8 (1.29 ± 0.12 × 107 vs. 7.26 ± 0.13 × 106, 1.02 ± 0.1 × 105 vs. 7.11 ± 1.58 × 104, 9.53 ± 0.62 × 105 vs. 2.30 ± 0.74 × 104, 2.58 ± 0.37 × 104 vs. 2.78 ± 0.49 × 103, all P < 0.05).
Virulence
Virulence factor regulated gene and agrA
Biopsies taken from postoperative days 2, 4, 6, and 8 were analyzed by quantitative RT-PCR. Expression levels of Eap, Spa, Hla, and agrA genes were measured. Data show no statistical difference before treatment in the two groups. Data showed a trend for a significant difference in expressions of Eap between NPWT and NPWTi groups on a postoperative day 4 and 8 (4.10 ± 0.29 vs. 3.09 ± 0.33, 1.99 ± 0.17 vs. 1.05 ± 0.15, all P < 0.05), despite no statistical difference on postoperative day 2 and 6 (3.98 ± 0.13 vs. 3.87 ± 0.38, 2.44 ± 0.14 vs. 2.34 ± 0.09, all P > 0.05) (Figure 3). In two groups, expression levels of Eap, Spa, Hla, and agrA on postoperative days 2, 4, and 6, were higher than before treatment (Figure 3). On postoperative day 8, expression levels of Spa were lower than before treatment in the NPWTi group but higher in the NPWT group. The expression levels of Spa, Hla, and agrA were significantly different on a postoperative day 2, 4, 6, and 8 between NPWT and NPWTi groups (Spa: 2.43 ± 0.10 vs. 1.22 ± 0.17, 1.71 ± 0.08 vs. 1.40 ± 0.21, 1.80 ± 0.20 vs. 1.51 ± 0.14, 1.05 ± 0.13 vs. 0.51 ± 0.16, all P < 0.05; Hla: 2.93 ± 0.13 vs. 1.26 ± 0.08, 2.21 ± 0.11 vs. 1.82 ± 0.32, 1.36 ± 0.14 vs. 1.16 ± 0.28, 1.25 ± 0.21 vs. 0.77 ± 0.18, all P < 0.05, agrA: 5.12 ± 0.25 vs. 1.65 ± 0.22, 3.75 ± 0.36 vs. 1.42 ± 0.15, 2.11 ± 0.14 vs. 1.48 ± 0.14, 1.94 ± 0.24 vs. 1.03 ± 0.18, all P < 0.05).
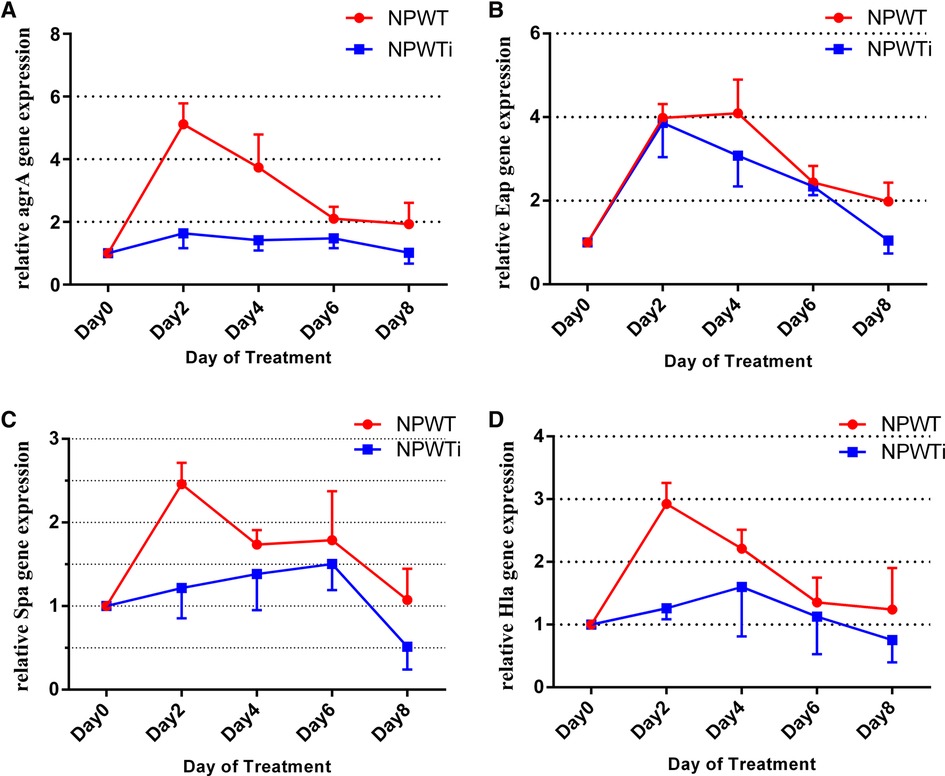
Figure 3. Differences in gene expression in the two treatment groups over time (fold change). (A) The difference of agrA expression; (B) The difference of Eap expression; (C) The difference of Spa expression; (D) The difference of Hla expression.
Virulence proteins
Western blot analysis was used to assess the production of Eap, Spa, and a-toxin proteins (Figure 4A). Data show no statistical difference before treatment in the two groups. The expression level of Eap protein was significantly lower in NPWTi groups than that in NPWT groups on postoperative days 4 and 8 (2.30 ± 0.10 vs. 4.43 ± 0.15 and 0.94 ± 0.15 vs. 2.03 ± 0.07, all P < 0.05). The expression level of Spa protein was significantly lower in NPWTi groups than that in NPWT groups on postoperative days 2 and 4 (2.33 ± 0.06 vs. 3.27 ± 0.12, 2.23 ± 0.06 vs. 4.43 ± 0.16, all P < 0.05). The expression level of a-toxin protein was significantly lower in NPWTi groups than that in NPWT groups on postoperative day 2 to 6 (2.47 ± 0.06 vs. 6.44 ± 0.06, 2.05 ± 0.05 vs. 3.58 ± 0.08, and 2.30 ± 0.10 vs. 2.97 ± 0.12, all P < 0.05), but higher in NPWTi groups than that in NPWT groups on postoperative day 8 (1.65 ± 0.05 vs. 1.48 ± 0.03, P < 0.05) (Figure 4B).
The depths of bacterial invasion
The depths of the bacterial fluorescent signal from samples before treatment and on postoperative days 2, 4, 6, and 8 were measured (Figure 5). The depth in the two groups increased on postoperative day 2 and decreased gradually from postoperative day 2 to 8. There was a significant difference on a postoperative day 2, 4, 6, and 8 between NPWT and NPWTi groups (1136.67 ± 60.28 um vs. 466.67 ± 20.82 um, 1053.33 ± 75.06 um vs. 418.33 ± 17.56 um, 633.33 ± 40.41 um vs. 206.67 ± 15.28 um, 183.33 ± 25.17 um vs. 153.33 ± 10.41 um, all P < 0.05) (Figure 7). The depth on a postoperative day 8 was no statistical difference compared with before treatment in the NPWT group, but a significant difference in the NPWTi group (P < 0.05).
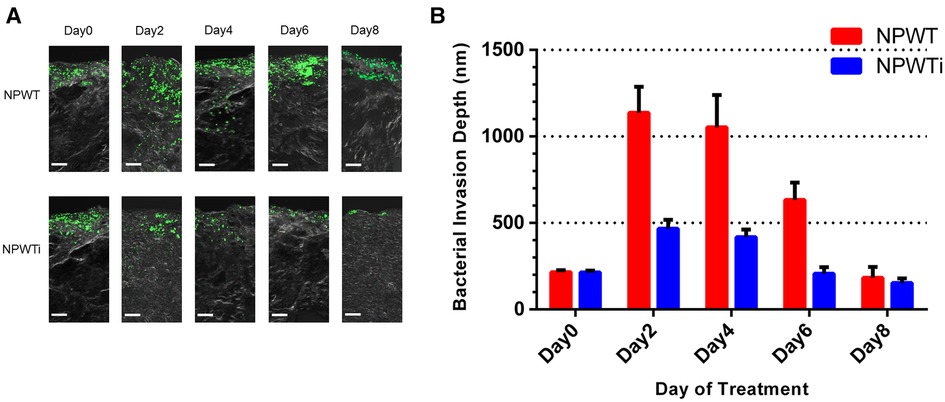
Figure 5. Bacterial invasion depth observed by laser scanning confocal microscopy. (A) This figure shows the depth of infection in NPWT and NPWTi on days 0, 2, 4, 6, and 8. (B) Bacterial invasion depth significantly descended in the NPWTi group compared with the NPWT group at day 2, 4, 6, and day 8 (*P < 0.001). Scale bar was 100 um.
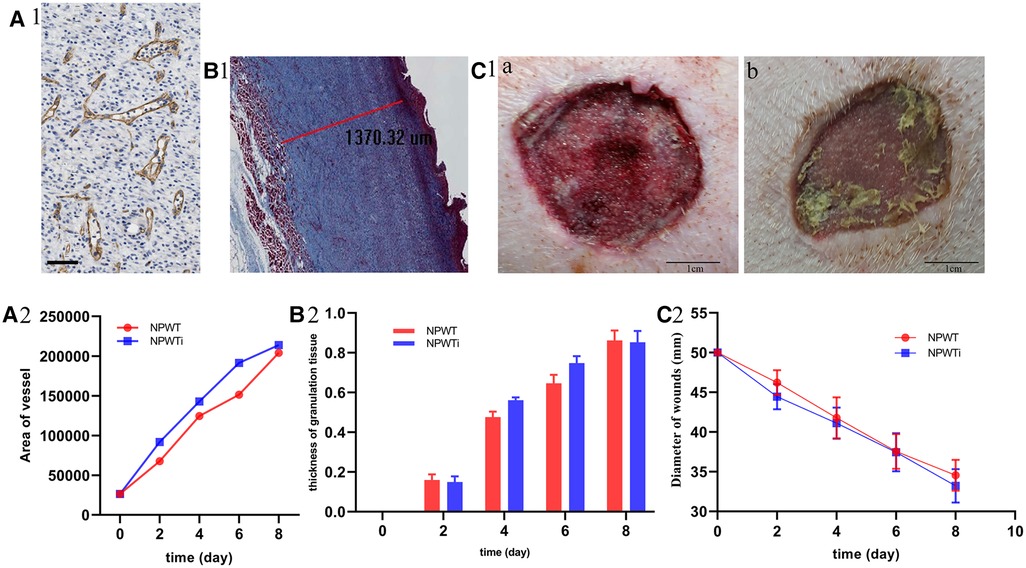
Figure 7. Histologic parameters and wound healing. (A) The difference of area of neovascularization between two groups and the scale bar was 100 um. (B) The difference of thickness of new granulation. (C) The difference of gap of wounds between two groups. Area of neovascularization and the thickness of new granulation tissue showed that it was significant larger in NPWTi group compared with NPWT on day4 and day6 (P < 0.05). No significant difference was observed between two groups on day 2, 4, 6, and day 8 with respect to wound gap (P > 0.05).
Histologic parameters and wound healing
Expression of VEGF and bFGF
In the two groups, the expression levels of VEGF and bFGF were increased gradually from postoperative day 2 to 8 (Figure 6). Expression levels of VEGF and bFGF on postoperative day 2, 4, and 6, were significantly higher in the NPWTi group than in that of the NPWT group (P < 0.05). However, expression levels of VEGF on postoperative day 8 were significant higher in the NPWT group. No significant difference was observed between the two groups on postoperative day 8 concerning expression levels of bFGF (P > 0.05).
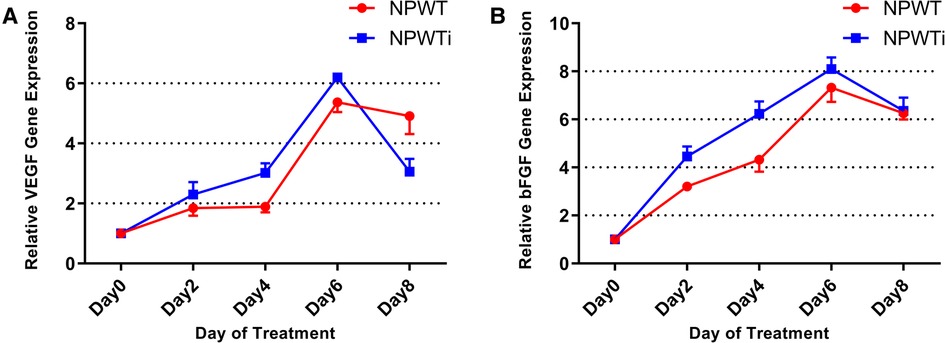
Figure 6. (A) the difference in VEGF expression. (B) The difference of bFGF expression. Expression levels of VEGF and bFGF on postoperative day 2, 4, and 6, were significantly higher in the NPWTi group than in that of the NPWT group (P < 0.05) (fold change).
Measurement of neovascularization, new granulation, and healing
Measurement of the area of neovascularization showed that it was significantly larger in the NPWTi group compared with NPWT on day 4 and day 6 (P < 0.05). No significant difference was observed between the two groups on day 8 (P > 0.05). Similarly, the thickness of new granulation tissue was significantly larger in the NPWTi group compared with NPWT on day 4 and day 6 (P < 0.05). No significant difference was observed between the two groups on day 8 (P > 0.05). No significant difference was observed between the two groups on days 2, 4, 6, and day 8 concerning wound gap (P > 0.05) (Figure 7).
Discussion
Staphylococcus aureus is the most frequent opportunistic pathogen in both acute and chronic wounds. Many studies have shown a decrease in bacterial burden in response to NPWT (22, 23). Compared to NPWT, the effect of NPWTi on infected wounds is less understood. Published animal studies suggested that non-contaminated wounds may benefit from NPWTi as well (24). Up to now, the effects of NPWTi on Staphylococcus aureus infected wounds are still unclear.
There are many types of NPWTi, including NPWT with continuous irrigation, periodic instillation with a soak time, continuous NPWT, and regular instillation. In this experiment, we use the second type of NPWTi, which cycles between 3 discrete phases in order: instillation, soak, and NPWT. If the negative pressure is not stopped while the solution is being delivered, coverage of the wound with the instilled solution may be incomplete (25). And, Bench studies suggest that interruption of negative pressure and the introduction of a soak phase are essential for uniform coverage of the wound with the topical wound treatment solution, especially when complex wound geometries are present.
Bacteria within a wound can range from contamination, colonization, localized infection, spreading infection, and ultimately to systemic infection if not appropriately controlled. Regular instillation of solution can assist with wound cleansing, irrigation, and easier removal of infectious materials. Although the NPWTi can significantly decrease the counts of bacteria and the depths of bacterial invasion, the mechanism remains unclear. That may be what we may need to study in the next step.
Numerous studies have shown that bacterial load levels are closely related to wound healing (26–29). previous studies have reported the effects of NPWT on the bacterial burden, but the results were contradictory. A retrospective study by Weed, T. et al. showed that NPWT increased bacterial colonization significantly in patients with acute or chronic wounds (30). A randomized controlled trial (RCT) by Mouës, C. M. et al. observed that there is no significant statistical difference in the number of bacteria between NPWT and conventional therapy (31). So far, rare studies have compared the bacterial load levels between NPWT and NPWTi groups. A prospective pilot study by Goss, S. G. showed that NPWTi reduced bacterial load levels in patients with chronic wounds over 7 days, while NPWT increased bacterial load levels (32). In this study, we found that both NPWT and NPWTi can decrease the counts of bacteria, and the NPWT with instillation was superior to NPWT alone. On the postoperative day 4, the bacterial count in the NPWTi group was lower than the clinical infection standard, and on day 8, the bacterial count in both groups was lower than the clinical infection standard, but the bacterial count in the NPWTi group had reached below 104 CFUs/wound. The possible reasons for the lower bacterial count in the NPWTi group may be the more efficient removal of exudates from wounds and the reduction of expression levels of Eap, Spa, and Hla genes.
Auto-inducing peptide (AIP), an index of bacterial density, could affect the activation of agr system. On the other hand, agr system could positively regulate the expression of a series of virulence factors, including Eap, Spa, and Hla genes (33). Our results showed that the expression level of agrA in the NPWTi group was significantly lower compared with that in the NPWT group.
Eap is probably a key factor in the pathogenesis of S. aureus working by mediating bacterial adhesion and endowing bacteria with the ability to evade host defense functions (34, 35). Spa protein is an important virulence factor of S.aureus, allowing S.aureus to escape host adaptive immune responses by binding to the Fc segment of immunoglobulin (36). Hla, an important pore-forming toxin (PFTs) of S. aureus, could promote bacterial biofilm development and macrophage dysfunction through the combined action with leukocidin AB (37). We have found that the expression level of Eap peaked on a postoperative day 4 and began to decline thereafter in both two groups. The expression level of Spa and Hla peaked on postoperative day 2 and began to decline thereafter. In general, our results showed that the expression levels of Eap, Spa, and Hla genes in the NPWTi group were significantly lower than that in the NPWT group. A previous study has demonstrated that changes in environmental factors, such as osmotic pressure, oxygen concentration, temperature, and other physical signal stimuli, can affect the virulence regulatory system of bacteria which plays an important role in the pathogenesis of bacteria (38). Negative pressure was a kind of physical signal stimulus and that is why it could affect the expression of these genes.
The depth of bacterial invasion in soft tissue determines the depth of infection. We have observed that the bacterial invasion depth peaked on a postoperative day 2 and began to decline thereafter, which was in accordance with the expression level of Spa and Hla. The bacterial invasion depth in the NPWTi group was significantly lower than that in the NPWT group. The possible reason is that NPWTi recirculates the perfusate through negative pressure suction, which can better reduce the incidence of blockage, reduce the load of wound secretions, and drain local secretions and necrotic tissue, so as to better take away some metabolic wastes and toxins.
VEGF and bFGF are key factors for neovascularization and wound healing. Wounds treated by NPWTi showed relatively more area of neovascularization and new granulation compared with NPWT alone. However, this superiority did not result in an effective conversion in wound healing. The potential reason is that wound healing cannot benefit from the addition of instillation. Besides, saline was used as a solution in this study, and change of solution may result in other outcomes.
There are some limitations in this study. First, only a single bacterium was used as the infection model, and wound infection in clinical practice was mostly a mixed infection of multiple bacteria. Second, wild-type S.aureus was used as the infection strain, which is more sensitive to antibiotics. Infection strains are often drug-resistant in clinical practice, but drug-resistant strains were not explored in this study. Third, a single solution was used in this study, and other antimicrobial solutions such as PHMB, and povidone-iodine were not investigated.
Conclusion
This study demonstrated that NPWTi induced a better decrease in bacterial burden and virulence compared with standard NPWT on the porcine wound model. However, these advantages did not result in better wound healing.
Data availability statement
The original contributions presented in the study are included in the article/Supplementary Material, further inquiries can be directed to the corresponding author/s.
Ethics statement
The animal study was reviewed and approved by All animal experiments were approved by the Medical Ethics Committee of the Chinese PLA General Hospital (Beijing, China).
Author contributions
WG, LD and LZ made contributions to designing the study and providing critical revisions to this article. ST, FX and YT were responsible for completing the experiments and writing the article. Ax, LR and LF made contributions to writing the article. All authors contributed to the article and approved the submitted version.
Funding
This research is supported by Hainan Province Clinical Medical Center and the projects of military logistics research foundation (No. AWS17J004) to ZL.
Conflict of interest
The authors declare that the research was conducted in the absence of any commercial or financial relationships that could be construed as a potential conflict of interest.
Publisher's note
All claims expressed in this article are solely those of the authors and do not necessarily represent those of their affiliated organizations, or those of the publisher, the editors and the reviewers. Any product that may be evaluated in this article, or claim that may be made by its manufacturer, is not guaranteed or endorsed by the publisher.
References
1. Rippon MG, Westgate S, Rogers AA. Implications of endotoxins in wound healing: a narrative review. J Wound Care. (2022) 31(5):380–92. doi: 10.12968/jowc.2022.31.5.380.35579309
2. Laato M, Niinikoski J, Lundberg C, Gerdin B. Inflammatory reaction and blood flow in experimental wounds inoculated with Staphylococcus aureus. Eur Surg Res Europaische Chirurgische Forschung Recherches Chirurgicales Europeennes. (1988) 20(1):33–8. doi: 10.1159/000128738.3165343
3. Mayandi V, Wen Choong AC, Dhand C, Lim FP, Aung TT, Sriram H, et al. Multifunctional antimicrobial nanofiber dressings containing ε-polylysine for the eradication of bacterial bioburden and promotion of wound healing in critically colonized wounds. ACS Appl Mater Interfaces. (2020) 12(14):15989–6005. doi: 10.1021/acsami.9b21683.32172559
4. Neopane P, Nepal HP, Shrestha R, Uehara O, Abiko Y. In vitro biofilm formation by Staphylococcus aureus isolated from wounds of hospital-admitted patients and their association with antimicrobial resistance. Int J Gen Med. (2018) 11:25–32. doi: 10.2147/IJGM.S153268.29403304
5. Putra I, Rabiee B, Anwar KN, Gidfar S, Shen X, Babalooee M, et al. Staphylococcus aureus alpha-hemolysin impairs corneal epithelial wound healing and promotes intracellular bacterial invasion. Exp Eye Res. (2019) 181:263–70. doi: 10.1016/j.exer.2019.02.019.30822400
6. Shettigar K, Murali TS. Virulence factors and clonal diversity of Staphylococcus aureus in colonization and wound infection with emphasis on diabetic foot infection. Eur J Clin Microbiol & Infect Dis. (2020) 39(12):2235–46. doi: 10.1007/s10096-020-03984-8.
7. Novak A, Khan WS, Palmer J. The evidence-based principles of negative pressure wound therapy in trauma & orthopedics. Open Orthop J. (2014) 8:168–77. doi: 10.2174/1874325001408010168.25067971
8. Orgill DP, Manders EK, Sumpio BE, Lee RC, Attinger CE, Gurtner GC, et al. The mechanisms of action of vacuum assisted closure: more to learn. Surgery. (2009) 146(1):40–51. doi: 10.1016/j.surg.2009.02.002.19541009
9. Banwell PE. Topical negative pressure therapy in wound care. J Wound Care. (1999) 8(2):79–84. doi: 10.12968/jowc.1999.8.2.25844.10232203
10. Evans D, Land L. Topical negative pressure for treating chronic wounds: a systematic review. Br J Plast Surg. (2001) 54(3):238–42. doi: 10.1054/bjps.2001.3547.11254418
11. Fleischmann W, Lang E, Russ M. Treatment of infection by vacuum sealing. Unfallchirurg. (1997) 100(4):301–4. doi: 10.1007/s001130050123.9229781
12. Gardner SE, Frantz RA, Doebbeling BN. The validity of the clinical signs and symptoms used to identify localized chronic wound infection. Wound Repair and Regen. (2001) 9(3):178–86. doi: 10.1046/j.1524-475x.2001.00178.x.
13. Li T, Zhang L, Han LI, Wang G, Yin P, Li Z, et al. Early application of negative pressure wound therapy to acute wounds contaminated with Staphylococcus aureus: an effective approach to preventing biofilm formation. Exp Ther Med. (2016) 11(3):769–76. doi: 10.3892/etm.2016.3008.26997991
14. Li T, Wang G, Yin P, Li Z, Zhang L, Liu J, et al. Effect of negative pressure on growth, secretion and biofilm formation of Staphylococcus aureus. Antonie van Leeuwenhoek. (2015) 108(4):907–17. doi: 10.1007/s10482-015-0545-9.26272011
15. Wang GQ, Li TT, Li ZR, Zhang LC, Zhang LH, Han L, et al. Effect of negative pressure on proliferation, virulence factor secretion, biofilm formation, and virulence-regulated gene expression of Pseudomonas aeruginosa in vitro. BioMed Res Int. (2016) 2016:7986234. doi: 10.1155/2016/7986234
16. Gabriel A, Shores J, Heinrich C, Baqai W, Kalina S, Sogioka N, et al. Negative pressure wound therapy with instillation: a pilot study describing a new method for treating infected wounds. Int Wound J. (2008) 5(3):399–413. doi: 10.1111/j.1742-481X.2007.00423.x.18593390
17. Zhen ZJ, Lai ECH, Lee QH, Chen HW, Lau WY, Wang FJ. Conventional wound management versus a closed suction irrigation method for infected laparotomy wound–A comparative study. Int J Surg. (2011) 9(5):378–81. doi: 10.1016/j.ijsu.2011.02.012.21371577
18. Wolvos T. Wound instillation–the next step in negative pressure wound therapy. Lessons learned from initial experiences. Ostomy Wound Manage. (2004) 50(11):56–66.15545698
19. Morykwas MJ, Argenta LC, Shelton-Brown EI, McGuirt W. Vacuum-assisted closure: a new method for wound control and treatment: animal studies and basic foundation. Ann Plast Surg. (1997) 38(6):553–62. doi: 10.1097/00000637-199706000-00001.9188970
20. Liu D, Zhang L, Li T, Wang G, Du H, Hou H, et al. Negative-pressure wound therapy enhances local inflammatory responses in acute infected soft-tissue wound. Cell Biochem Biophys. (2014) 70(1):539–47. doi: 10.1007/s12013-014-9953-0.24748178
21. Liu D, Li Z, Wang G, Li T, Zhang L, Tang P. Virulence analysis of Staphylococcus aureus in a rabbit model of infected full-thickness wound under negative pressure wound therapy. Antonie van Leeuwenhoek. (2018) 111(2):161–70. doi: 10.1007/s10482-017-0938-z.28894985
22. Li T, Wang G, Yin P, Li Z, Zhang L, Tang P. Adaptive expression of biofilm regulators and adhesion factors of Staphylococcus aureus during acute wound infection under the treatment of negative pressure wound therapy in vivo. Exp Ther Med. (2020) 20(1):512–20. doi: 10.3892/etm.2020.8679.32509022
23. Sirisena R, Bellot GL, Puhaindran ME. The role of negative-pressure wound therapy in lower-limb reconstruction. Indian J Plast Surg. (2019) 52(1):73–80. doi: 10.1055/s-0039-1687922.31456615
24. Leung BK, LaBarbera LA, Carroll CA, Allen D, McNulty AK. The effects of normal saline instillation in conjunction with negative pressure wound therapy on wound healing in a porcine model. Wounds-a Compend of Clin Res and Pract. (2010) 22(7):179–87.
25. Rycerz AM, Slack P, McNulty AK. Distribution assessment comparing continuous and periodic wound instillation in conjunction with negative pressure wound therapy using an agar-based model. Int Wound J. (2013) 10(2):214–20. doi: 10.1111/j.1742-481X.2012.00968.x.22487428
26. Xu Z, Hsia HC. The impact of microbial communities on wound healing: a review. Ann Plast Surg. (2018) 81(1):113–23. doi: 10.1097/SAP.0000000000001450.29746280
27. García-Salinas S, Evangelopoulos M, Gámez-Herrera E, Arruebo M, Irusta S, Taraballi F, et al. Electrospun anti-inflammatory patch loaded with essential oils for wound healing. Int J Pharm. (2020) 577:119067. doi: 10.1016/j.ijpharm.2020.119067.
28. Malone-Povolny MJ, Maloney SE, Schoenfisch MH. Nitric oxide therapy for diabetic wound healing. Adv Healthcare Mater. (2019) 8(12):e1801210. doi: 10.1002/adhm.201801210.
29. Edwards R, Harding KG. Bacteria and wound healing. Curr Opin Infect Dis. (2004) 17(2):91–6. doi: 10.1097/00001432-200404000-00004.15021046
30. Weed T, Ratliff C, Drake DB. Quantifying bacterial bioburden during negative pressure wound therapy: does the wound VAC enhance bacterial clearance? Ann Plast Surg. (2004) 52(3):276–9.; discussion 279–280. doi: 10.1097/01.sap.0000111861.75927.4d
31. Mouës CM, van den Bemd GJ, Heule F, Hovius SE. Comparing conventional gauze therapy to vacuum-assisted closure wound therapy: a prospective randomised trial. J Plast Reconstr & Aesthet Surg: JPRAS. (2007) 60(6):672–81. doi: 10.1016/j.bjps.2006.01.041.
32. Goss SG, Schwartz JA, Facchin F, Avdagic E, Gendics C, Lantis JC. Negative pressure wound therapy with instillation (NPWTi) better reduces post-debridement bioburden in chronically infected lower extremity wounds than NPWT alone. J Am Coll Clin Wound Spec. (2012) 4(4):74–80. doi: 10.1016/j.jccw.2014.02.001.26199877
33. Schilcher K, Horswill AR. Staphylococcal biofilm development: structure, regulation, and treatment strategies. Microbiol and Mol Biol Rev: MMBR. (2020) 84(3). doi: 10.1128/MMBR.00026-19.
34. Hammel M, Nemecek D, Keightley JA, Thomas GJ Jr, Geisbrecht BV. The Staphylococcus aureus extracellular adherence protein (eap) adopts an elongated but structured conformation in solution. Protein Sci. (2007) 16(12):2605–17. doi: 10.1110/ps.073170807.18029416
35. Sobke AC, Selimovic D, Orlova V, Hassan M, Chavakis T, Athanasopoulos AN, et al. The extracellular adherence protein from Staphylococcus aureus abrogates angiogenic responses of endothelial cells by blocking ras activation. FASEB journal. (2006) 20(14):2621–3. doi: 10.1096/fj.06-5764fje.17077291
36. Falugi F, Kim HK, Missiakas DM, Schneewind O. Role of protein A in the evasion of host adaptive immune responses by Staphylococcus aureus. mBio. (2013) 4(5):e00575–00513. doi: 10.1128/mBio.00575-13.23982075
37. Scherr TD, Hanke ML, Huang O, James DB, Horswill AR, Bayles KW, et al. Staphylococcus aureus biofilms induce macrophage dysfunction through leukocidin AB and alpha-toxin. mBio. (2015) 6(4). doi: 10.1128/mBio.01021-15.26307164
Keywords: NPWT, instillation, wound healing, staphylococcus aureus, virulence
Citation: Tingting S, Xinyue F, Tiantian Y, xiao A, Rui L, Feng L, Daohong L, Zhirui L and Guoqi W (2023) Comparison of the effects of negative pressure wound therapy and negative pressure wound therapy with instillation on wound healing in a porcine model. Front. Surg. 10:1080838. doi: 10.3389/fsurg.2023.1080838
Received: 26 October 2022; Accepted: 15 March 2023;
Published: 17 April 2023.
Edited by:
Kent Bachus, United States Department of Veterans Affairs, United StatesReviewed by:
Cheng Chen, Chongqing Medical University, ChinaTao Shi, Tianjin First Central Hospital, China
Wang Yunjiao, Army Medical University, China
© 2023 Tingting, Xinyue, Tiantian, xiao, Rui, Feng, Daohong, Zhirui and Guoqi. This is an open-access article distributed under the terms of the Creative Commons Attribution License (CC BY). The use, distribution or reproduction in other forums is permitted, provided the original author(s) and the copyright owner(s) are credited and that the original publication in this journal is cited, in accordance with accepted academic practice. No use, distribution or reproduction is permitted which does not comply with these terms.
*Correspondence: Wang Guoqi guoqi71@126.com Li Zhirui lzr860514@163.com Liu Daohong 13810085005@139.com Lin Feng lyf1011@aliyun.com
†These authors have contributed equally to this work.
Specialty Section: This article was submitted to Reconstructive and Plastic Surgery, a section of the journal Frontiers in Surgery