- 1Department of Orthopedics, Beijing Chaoyang Hospital, Capital Medical University, Beijing, China
- 2Department of Orthopedics, The General Hospital of Taiyuan Iron / Steel (Group) Corporation, Taiyuan, China
Purpose: This study aims to investigate the risk factors for screw loosening after single-level posterior lumbar interbody fusion (PLIF) utilizing cortical bone trajectory (CBT) screw and establish a nomogram for predicting screw loosening.
Methods: A total of 79 patients (316 screws) who underwent single-level PLIF with CBT screw were included in the study. Preoperative, postoperative, and final follow-up demographic data, surgical data, and radiographic parameters were documented and analyzed to identify risk factors, and a predictive nomogram was established for screw loosening. The nomogram was assessed by concordance index (C-index), calibration plot, decision curve analysis (DCA), and internal validation.
Results: The incidence of screw loosening was 26.6% in 79 patients and 11.4% in 316 screws. Multifactorial regression analysis confirmed that fixed to S1 (FS1, OR = 3.82, 95% CI 1.12–12.71, P = 0.029), the coronal angle of the screw (CA, OR = 1.07, 95% CI 1.01–1.14, P = 0.039), and cortical bone contacted layers (CBCLs, OR = 0.17, 95% CI 0.10–0.29, P < 0.001) were risk factors and incorporated in the nomogram for predicting screw loosening after single-level PLIF with a CBT screw. The C-index of the nomogram was 0.877 (95% CI 0.818–0.936), which demonstrated good predictive accuracy. The calibration plot indicated an acceptable calibration of the nomogram that also had a positive benefit in guiding treatment decisions.
Conclusion: FS1, CA, and CBCLs are identified to be significant risk factors for screw loosening after single-level PLIF with the CBT technique. The nomogram we have established can be used to predict screw loosening and contribute to surgical decisions.
Introduction
Cortical bone trajectory (CBT) is an alternative approach first proposed by Santoni et al. as the treatment for a lumbar degenerative disease (1). CBT screw was inserted via the trajectory that could engage the pars, medial, and superior cortices of the pedicle isthmus for spinal fusion, and theoretically, it provided comparable pull-out resistance and stability to a traditional pedicle screw (TPS) (2–4). Likewise, good results have been found in literature works reporting the application of CBT screw in osteoporosis lumbar spine (1, 5, 6). The main role of the screw in lumbar fusion is to reduce the motion of the spine and to conduct the stabilization, whereas screw loosening is observed in quite a few literature works (7–9). As reported, the incidence of screw loosening in TPS was 1%–60% (7, 10, 11), and risk factors were related to osteoporosis, sacrum instrument, excessive load, and local high strains; however, it was not unified. CBT screw conducts a comparable fixation to TPS according to the characteristic, and it may reduce the risk of screw loosening due to the loading resistivity of cortical bone of the pedicles. Nevertheless, the screw loosening rate was still observed to be 62.5% (9, 12–14), and the risk factors were also uncertain. This leads to consideration of the differences in risk factors for screw loosening between CBT screw and TPS.
Screw loosening in both the TPS and CBT screw may require revision surgery due to symptomatic spinal instability and instrument failure (9, 11, 15, 16); thus, comprehension of screw loosening is essential. Previous studies were researched, and we found that few studies have concentrated on CBT screw loosening, or most of them lacked long-term follow-up and sufficient evidence. Hence, clear exploration of screw loosening of CBT screws is demanded with an available analysis of the literature.
The present study aimed to detect the prevalence of screw loosening in single-level PLIF using CBT screw with a minimum of 2 years of follow-up and to establish a nomogram for predicting screw loosening individually in each vertebra.
Methods
This was a retrospective study in the institution. A total of 88 consecutive patients were included in the study from November 2017 to January 2020, and 79 eligible patients were evaluated (Figure 1). Inclusion criteria are listed as follows: (1) patients diagnosed with lumbar degenerative disease (lumbar disc herniation, lumbar spinal stenosis, and lumbar spondylolisthesis) and who underwent single-level PLIF with CBT screws; (2) minimum follow-up time of above 2 years. Excluded criteria are as follows: (1) incomplete radiological data; (2) patients who underwent surgery diagnosed with lumbar infection, lumbar vertebral tumor, or history of lumbar surgery. The study was approved by the institutional review board of the hospital.
Surgical technique
The patient was placed in a prone position. A 5-cm midline skin incision was performed in the lumbar area. Muscular dissection was performed until the vertebral isthmus was exposed. The facet joints were exposed, and adjacent facet joints of the fusion area were avoided. The entry point was selected as an intersection of a vertical line through the center of the inferior facet joint of the adjacent cephalic vertebra and a horizontal line 3–4 mm below the inferior facet joint of the cephalic vertebra (a notch might be identified on the isthmus). The track was drilled with a 2 mm burr into the cortical bone with an approximate 10°–15° angle from medial to lateral and 20°–25° angle from caudal to cranial. Locating pins were placed into the track, and fluoroscopy was performed to check the position. Then, decompression was performed, and a cage (PEEK) filled with autogenous bone was implanted into the intervertebral space after endplate preparation and autogenous bone insertion. After the decompression, pins were removed, and CBT screws (for the S1 vertebra, the screw was 45 mm in length and 6.0 mm in diameter, and for other vertebras, the screw was 35 mm in length and 5.5 mm in diameter) were inserted through the tracks with spinous process preservation. Bended rods were then positioned and tightened bilaterally after compression was performed. Finally, fluoroscopy was performed to recheck the position of the screws and cage before the skin was sutured layer by layer.
Clinical and radiological evaluations
Clinical, demographic, and surgical data including age, gender, body mass index (BMI), operation time, and estimated blood loss (EBL) were collected. Radiological parameters including the coronal angle of the screw (CA), sagittal angle of the screw (SA), fixed to S1 (FS1), Hounsfield unit (HU) measurement of the trabecular bone of screw location, and cortical bone contacted layers (CBCLs) were evaluated (Figures 2, 3). The HU measurement was defined as the average of three points located in the screw track in a preoperative CT scan. Screw loosening was defined as a continuous lucent zone with a size of more than 1 mm and surrounded by a thin sclerotic zone in a CT scan (7, 17, 18). Bone fusion was graded according to Bridwell classification into three grades based on a lumbar CT scan (19): Grade I, complete fusion with the bridging bone bonding with both adjacent vertebral bodies; Grade II, incomplete fusion with the bridging bone bonding with either superior or inferior vertebral bodies; Grade III, failed fusion with incomplete bony bridging. Bone fusion was assessed by CT scan slices selected from the center of the cage or the largest bone grafting (20). The Oswestry Disability Index (ODI) was used to evaluate back pain preoperatively and at a postoperative time point of 6 months and final follow-up.
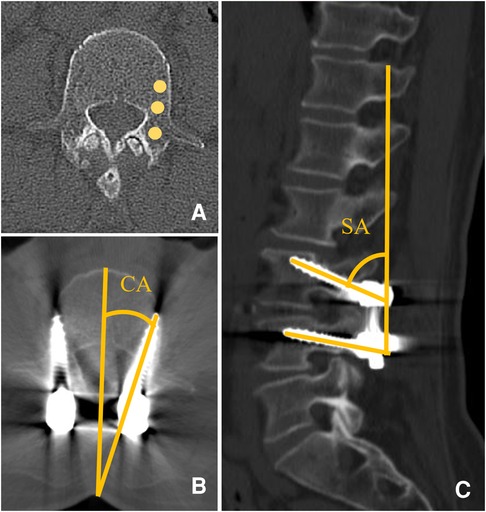
Figure 2. (A) HU measurement of an average of three points identified according to the screw track in the preoperative lumbar CT scan. (B) CA was defined as the angle between the screw and spinous process in the axial plane. (C) SA was defined as the angle between the screw line and vertical line in the sagittal plane.
Statistics analysis
SPSS Statistics Version 23.0 (IBM, Armonk, New York) and R software (version 4.1.2) were used for data analysis. Univariate analysis was performed with an independent t-test and Mann–Whitney U test for continuous data and discontinuous data, respectively, and quantitative data were listed as means ± SD with normal distribution or as medians with interquartile ranges with non-normal distribution. The chi-squared test was used for categorical data analysis.
Multivariate logistic analysis was further performed on variates that had significant differences in univariate analysis (P < 0.05). In this study, CBCLs, CA, and FS1 were put into the logistic model. A multiple logistic regression model was applied to select significant variables with a stepwise forward method, and the odds ratio (OR) and 95% confidence interval (CI) of the variables were recorded. A nomogram was established with R software. The concordance index (C-index) of the nomogram was calculated to evaluate the predictive accuracy of the nomogram utilizing the “rms” package. A calibration plot and the Hosmer–Lemeshow test were used to assess the calibration, and the nomogram was internally validated by the bootstraps of 1,000 resamples. Decision curve analysis (DCA) was calculated by the “rmda” package to evaluate the clinical usefulness of the nomogram. The reliable outcome is considered as C-index >0.75, and P value <0.05 was considered statistically significant for all data.
Results
A total of 88 consecutive patients were identified, and 79 patients (316 screws) were included in the study. The cohort contained 35 (44.3%) male patients with an average age of 65.14 ± 9.74 years, and the average BMI was 26.83 ± 4.49. The mean follow-up time was 25.38 ± 1.77 months (Table 1). The incidence of screw loosening was 26.6% (21) in the cohort and 11.4% (36) in 316 screws; 5 patients presented back pain and received conservative treatment, and the other patients (16) were asymptomatic. The patients were divided into two groups according to the presence of screw loosening: screw loosening group (SL) and no screw loosening group (non-SL). Statistically significant differences were found in CA (P = 0.039), FS1 (P = 0.029), and CBCLs (P < 0.001) between the two groups (Table 2). Multiple logistic regression was performed on these parameters, and the results demonstrated that FS1 (OR = 3.82, 95% CI 1.12–12.71, P = 0.029), CA (OR = 1.07, 95% CI 1.01–1.14, P = 0.039), and CBCLs (OR = 0.17, 95% CI 0.10–0.29, P < 0.001) were risk factors for screw loosening after single-level PLIF with CBT screws (Table 3).
The nomogram was conducted by R software (version 4.1.2) with a 0.877 (95% CI 0.818–0.936) C-index, which demonstrated good discrimination and predictive accuracy (Figure 4). Calibration evaluated by the calibration plot of the nomogram was good (Figure 5), and the Hosmer-Lemeshow test was good (P = 0.755). The internal validation by bootstraps of 1,000 resamples was excellent, with a 0.880 (95% CI 0.815–0.932) C-index. Decision curve analysis was performed, as shown in Figure 6, and when the threshold probabilities ranged from 0% to 60%, the nomogram showed a positive net benefit, which means clinical interventions implemented in those patients guided by the nomogram could obtain more benefit compared with treating all or treating none.
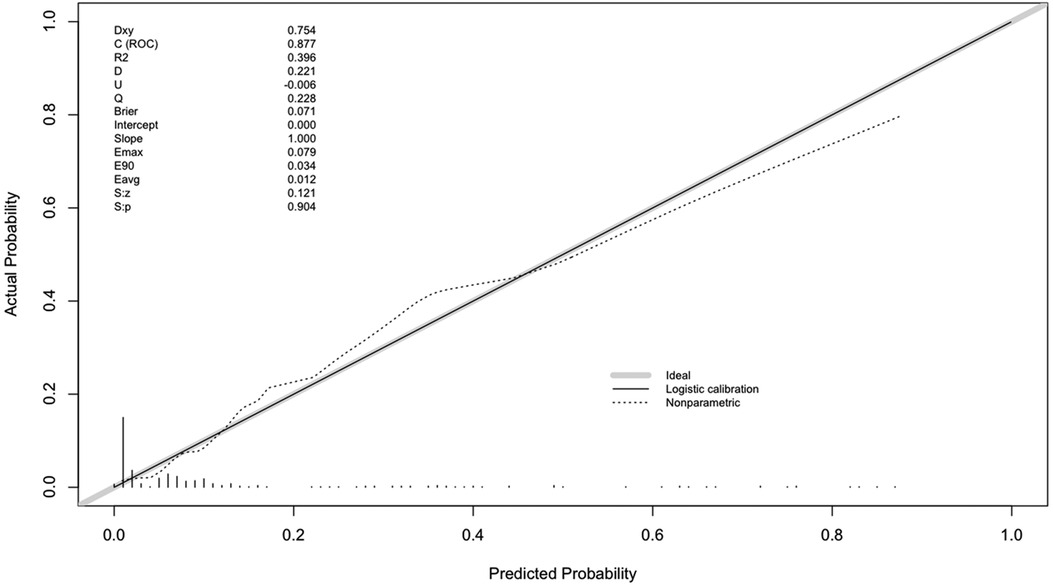
Figure 5. Calibration plot. The calibration of the nomogram was represented by the solid line, and any bias in the nomogram was corrected by the dashed line. The bold gray line indicates the reference line of an ideal nomogram.
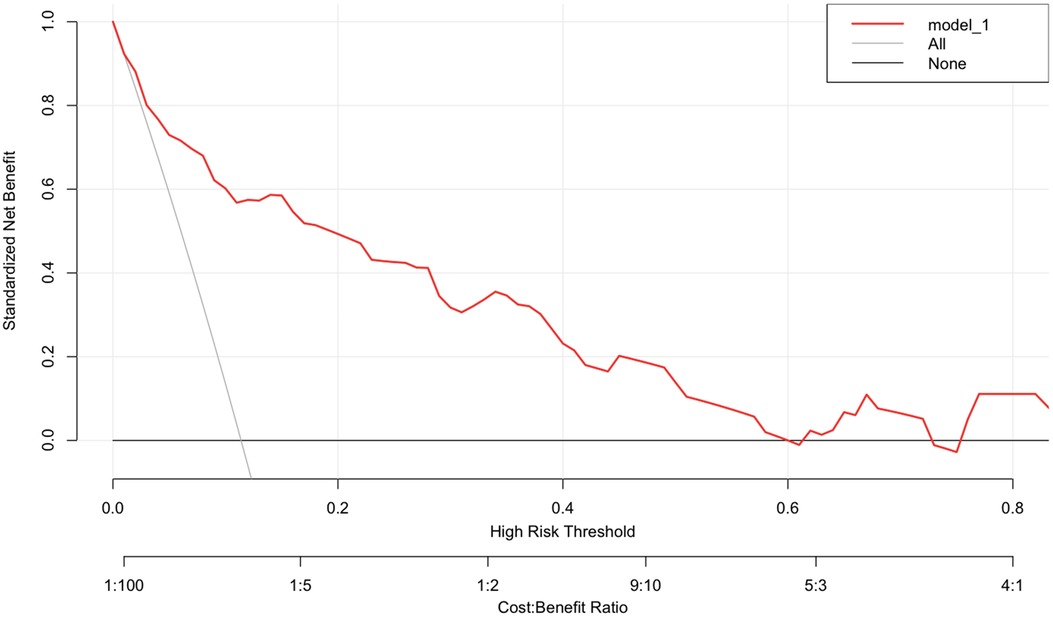
Figure 6. Decision curve analysis of the nomogram. The red line indicates the model. The x-axis and y-axis display the threshold probability and net benefit, respectively. The gray line represents the net benefit of treating all patients. The horizontal black line displays the net benefit of treating no patients.
Application indication of the nomogram is explained in Figure 7: a 62-year-old male patient underwent single-level PLIF with CBT screws at L4/5. A postoperative CT scan showed that the CA of L4L was 5° and of at L4R was 15°. The CAs of L5L and L5R were 16° and 17°, respectively, and the CBCLs of each screw were 3, 3, 2, and 1. Thus, according to the prediction nomogram, the score of each screw was approximately 45, 59, 92, and 126, which indicated that the incidence of screw loosening was <10%, 11%, 42%, and >80%. At the 1-year follow-up, we identified asymptomatic screw loosening at L4L, L5L, and L5R, which verified the accuracy of the nomogram. Also, the patient maintained asymptomatic at the final follow-up.
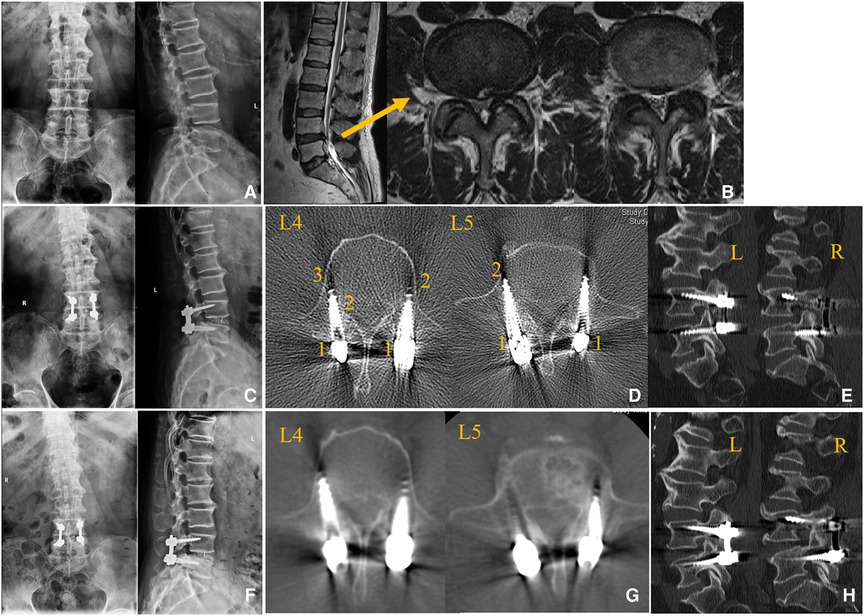
Figure 7. Case of the application of the nomogram. (A,B) Preoperative radiological data. (C–E) Immediate postoperative radiological data. (F–H) Radiological data at 1-year follow-up. (A) Lumbar spine x-ray. (B) Lumbar spine MRI demonstrating lumbar stenosis at L4/5. (C) Postoperative lumbar spine x-ray. (D) Postoperative lumbar spine CT scan indicating that the CBCLs of L4L, L4R, L5L, and L5R were 2, 3, 1, and 2, respectively. (E) Sagittal view of the lumbar spine CT scan. (F) After 1-year follow-up, a lumbar x-ray demonstrated a lucent zone at L4R and L5R. (G) Lumbar spine CT scan showing obvious screw loosening at L4L, L5L, and L5R. (H) Sagittal view of the lumbar spine CT scan.
To indicate whether the screw's location was in S1, three-dimensional surface plots are shown in Figures 8A,B to indicate the impact of CA and CBCLs on the probability of screw loosening.
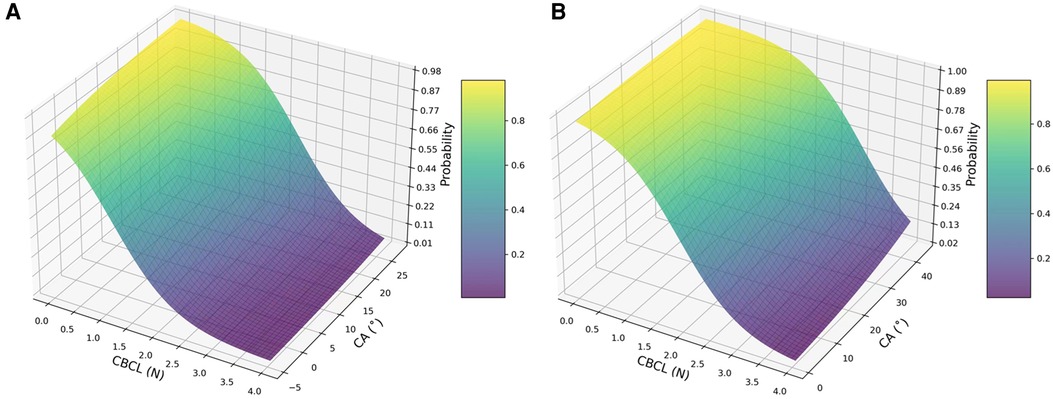
Figure 8. Three-dimensional surface plot demonstrating the impact of CBCL (x-axis) and CA (z-axis) on the probability of screw loosening after lumbar surgery with the CBT technique. (A) Probability of screw loosening when the screw was not instrumented in S1; (B) probability of screw loosening when the screw was instrumented in S1.
Discussion
Screw loosening is common, as reported in PLIF, with an incidence of 1%–60% (7, 10, 13). Risk factors as explored are connected with osteoporosis, incorrect failing loading scenario, insufficient fusion, or screw stress distribution (7); however, most of the research studies have not reached a consensus. CBT screw has comparable pull-out resistance and stability to TPS since it was first proposed in 2009 by Santoni et al. (1). It can provide enhanced screw purchase and preferable interface strength attributed to characteristics of engaging higher density cortical bone even in osteoporosis patients (21–23). Perez-Orribo et al. explored the biomechanics of TPS and CBT and concluded that equivalent stability was found between TPS and CBT fixation (3). Matsukawa et al. found that the screw insertion torque of CBT was 1.71 times higher than that of TPS (24). Thus, theoretically, CBT screw has been proposed to promote pull-out strength and enhance the construct stability. In the present study, we found a 26.6% incidence of screw loosening in 79 samples (11.4% in 316 screws). To investigate the risk factors of screw loosening, we documented and analyzed the mentioned parameters of each screw, which would be more beneficial for surgery, and the results of risk factor analysis showed that three main factors (FS1, CA, and CBCLs) mainly constituted the predict scoring nomogram.
The odds ratio of FS1 was the highest compared to other parameters (OR = 3.82). In our study, there were 22 screws fixed in the S1 vertebra, and 9 of them (40.9%) were found to have an obvious lucent zone in the CT scan. Grigoryan et al. (25) conducted a cadaveric biomechanical study and considered that lumbosacral fixation with CBT screws was stable against loosening, which is contrary compared the results of our study. The reasons of FS1 being concluded as a risk factor of screw loosening were assessed: First, lumbosacral fixation is inherently thought to have a higher risk of screw loosening due to alignment restoration and holding strength (26–28). Second, the learning curve of lumbosacral fixation with CBT is relatively higher. Matsukawa et al. (29) elucidated that the penetrating S1 endplate CBT technique with a mean cephalad angle of 30.7° could provide favorable stability for lumbosacral fixation, while during our work, especially for early cases, it was hard to identify a content position for the instrument in S1 and repeating screw track adjustment might result in instability, and this also occurred in other segments for early cases. Therefore, we considered that experienced surgeons are needed to perform fixation on S1; although the result was not good for FS1, we believe that CBT screw for S1 is an alternative method for fixation due to the reduction of paraspinal dissection and facility for retraction in the sacrum.
With regard to CA and CBCLs played an important role in screw loosening of CBT screw, according to the nomogram. The typical trajectory of the CBT screw contains four parts for the cortical bone to increase the stability of fixation; among these, the lateral par as the starting point is essential. The lateral par is an identifiable structure as an entry point and is less influenced by a degenerative change to provide a good bony reference in the surgery (30, 31). The starting point could also have an influence on CA. Literature works recommended an approximate 10°–14° angle to medial (32, 33), and in our study, the mean CA was 10.36° in the non-SL group and 13.94° in the SL group, which concluded similarly to the previous studies. Matsukawa (4) stated that CA was more variable than SA, and CA might have been derived from differences in the location of the starting point. We believe biochemical studies will be performed to clarify the mechanism in the future.
In the present study, we have documented and provided a reference for the measurement of SA as an angle between the screw line and vertical line because we think that this might reduce the error for measurement of wedge-shaped vertebra in some cases, while some authors recommended a method of the measurement of the angle between the screw and vertebral endplate (33, 34). However, the results showed no statistically significant difference between the two groups, but there was no denial that SA was an important parameter. Zhang et al. (35) conducted a study to compare the fixation failure between PS and CBT and concluded that different failure mechanisms underlay PS and CBT under large vertical displacement, and this may emphasize the characteristic of CBT screws in a sagittal view.
Lower BMD evaluated by dual x-ray absorptiometry (DXA) was significantly associated with screw loosening by influencing the pull-out strength (1, 36, 37); nevertheless, DXA assessed the average value of BMD. In addition to DXA, the use of HU based on a CT scan has been applied and clarified to be a reliable method for BMD evaluation (38–40), which can be used to assess the region involved by each screw. However, literature works revealed that there was no consensus on the HU value to evaluate a low BMD as a risk for osteoporosis. In the current study, BMD around the screw was assessed by the HU value to explore whether BMD would be a risk factor for screw loosening, and the result was negative. This demonstrated that the BMD of the region where screw threaded could not make much difference. Lee et al. (33) reported the HU measurement of cortical bone; however, we have attempted to make the repetition and the results showed poor inter-rater reproducibility due to the thin wall of cortical bone, and we did not adopt the method to replace CBCLs.
The study had some limitations, mainly due to retrospective analysis with small sample size. The surgery with the CBT technique was performed during the learning curve of the early period, and this might contribute to the loosening of the S1 screw. Our study focused on the local view of screws to explore the potential factors of screw loosening; however, we did not include parameters related to fusion because it was hard to judge whether the fusion failure was caused by screw loosening or bone graft. Further studies with experienced surgical techniques will be performed to validate the present study.
Conclusion
The CBT technique offers an alternative method for lumbar surgery with TPS. Although CBT screws provide good stability for fixation, we have identified significant risk factors for screw loosening. A perioperative evaluation with the nomogram can provide a reliable prediction of screw loosening with CBT screws and contribute to surgical decisions to avoid complications.
Data availability statement
The datasets used and/or analyzed during the current study are not publicly available due to the data being confidential; however, they are available from the corresponding author on a reasonable request.
Ethics statement
The studies involving human participants were reviewed and approved by the Institutional Review Board of Beijing Chao-yang Hospital. The patients/participants provided their written informed consent to participate in this study.
Author contributions
YZ writing—review and editing, formal analysis, collection and analysis of the data, and study design. YL formal analysis, collection and analysis of the data, and study design. YH performed the surgeries and designed and supervised the study. LG performed the surgeries. XZ collected and analyzed the data and methodology. AP validation, methodology, and investigation. HL collected and analyzed the data and validation. BW collected and analyzed the data. YuzL performed the surgeries and designed and supervised the study. All authors contributed to the article and approved the submitted version.
Conflict of interest
The authors declare that the research was conducted in the absence of any commercial or financial relationships that could be construed as a potential conflict of interest.
Publisher's note
All claims expressed in this article are solely those of the authors and do not necessarily represent those of their affiliated organizations, or those of the publisher, the editors and the reviewers. Any product that may be evaluated in this article, or claim that may be made by its manufacturer, is not guaranteed or endorsed by the publisher.
References
1. Santoni BG, Hynes RA, McGilvray KC, Rodriguez-Canessa G, Lyons AS, Henson MA, et al. Cortical bone trajectory for lumbar pedicle screws. Spine J. (2009) 9(5):366–73. doi: 10.1016/j.spinee.2008.07.008
2. Akpolat YT, İnceoğlu S, Kinne N, Hunt D, Cheng WK. Fatigue performance of cortical bone trajectory screw compared with standard trajectory pedicle screw. Spine (Phila Pa 1976). (2016) 41(6):E335–41. doi: 10.1097/BRS.0000000000001233
3. Perez-Orribo L, Kalb S, Reyes PM, Chang SW, Crawford NR. Biomechanics of lumbar cortical screw-rod fixation versus pedicle screw-rod fixation with and without interbody support. Spine (Phila Pa 1976). (2013) 38(8):635–41. doi: 10.1097/BRS.0b013e318279a95e
4. Matsukawa K, Yato Y. Lumbar pedicle screw fixation with cortical bone trajectory: a review from anatomical and biomechanical standpoints. Spine Surg Relat Res. (2017) 1(4):164–73. doi: 10.22603/ssrr.1.2017-0006
5. Shi W, Aierken G, Wang S, Abuduwali N, Xia Y, Rezhake R, et al. Application study of three-dimensional printed navigation template between traditional and novel cortical bone trajectory on osteoporosis lumbar spine. J Clin Neurosci. (2021) 85:41–8. doi: 10.1016/j.jocn.2020.11.038
6. Rexiti P, Aierken G, Wang S, Abudurexiti T, Abuduwali N, Deng Q, et al. Anatomical research on strength of screw track fixation in novel cortical bone trajectory for osteoporosis lumbar spine. Am J Transl Res. (2019) 11(11):6850–9.31814892
7. Galbusera F, Volkheimer D, Reitmaier S, Berger-Roscher N, Kienle A, Wilke HJ. Pedicle screw loosening: a clinically relevant complication? Eur Spine J. (2015) 24(5):1005–16. doi: 10.1007/s00586-015-3768-6
8. Huang Y, Wang J. Mechanism of and factors associated with the loosening of the implant abutment screw: a review. J Esthet Restor Dent. (2019) 31(4):338–45. doi: 10.1111/jerd.12494
9. Chen CH, Chen DC, Huang HM, Chuang HY, Hsu WL, Cho DY, et al. Level-based analysis of screw loosening with cortical bone trajectory screws in patients with lumbar degenerative disease. Medicine (Baltimore). (2020) 99(40):e22186. doi: 10.1097/MD.0000000000022186
10. Marie-Hardy L, Pascal-Moussellard H, Barnaba A, Bonaccorsi R, Scemama C. Screw loosening in posterior spine fusion: prevalence and risk factors. Global Spine J. (2020) 10(5):598–602. doi: 10.1177/2192568219864341
11. Yuan L, Zhang X, Zeng Y, Chen Z, Li W. Incidence, risk, and outcome of pedicle screw loosening in degenerative lumbar scoliosis patients undergoing long-segment fusion. Global Spine J. (2021) 5:21925682211017477. doi: 10.1177/21925682211017477
12. Hoffman H, Verhave B, Jalal MS, Beutler T, Galgano MA, Chin LS. Comparison of cortical bone trajectory screw placement using the midline lumbar fusion technique to traditional pedicle screws: a case-control study. Int J Spine Surg. (2019) 13(1):33–8. doi: 10.14444/6005
13. Chen CH, Tu CH, Chen DC, Huang HM, Chuang HY, Cho DY, et al. Incidence of screw loosening in cortical bone trajectory fixation technique between single- and dual-threaded screws. Medicines (Basel, Switzerland). (2021) 8(9):1–8. doi: 10.3390/medicines8090050
14. Liu L, Zhang S, Liu G, Yang B, Wu X. Early clinical outcome of lumbar spinal fixation with cortical bone trajectory pedicle screws in patients with osteoporosis with degenerative disease. Orthopedics. (2019) 42(5):e465–e71. doi: 10.3928/01477447-20190604-01
15. Wu JC, Huang WC, Tsai HW, Ko CC, Wu CL, Tu TH, et al. Pedicle screw loosening in dynamic stabilization: incidence, risk, and outcome in 126 patients. Neurosurg Focus. (2011) 31(4):E9. doi: 10.3171/2011.7.FOCUS11125
16. McAfee PC, Weiland DJ, Carlow JJ. Survivorship analysis of pedicle spinal instrumentation. Spine (Phila Pa 1976). (1991) 16(8 Suppl):S422–7.1785099
17. Pihlajämaki H, Myllynen P, Böstman O. Complications of transpedicular lumbosacral fixation for non-traumatic disorders. J Bone Joint Surg Br Vol. (1997) 79(2):183–9. doi: 10.1302/0301-620X.79B2.0790183
18. Ohlin A, Karlsson M, Düppe H, Hasserius R, Redlund-Johnell I. Complications after transpedicular stabilization of the spine. A survivorship analysis of 163 cases. Spine (Phila Pa 1976). (1994) 19(24):2774–9. doi: 10.1097/00007632-199412150-00007
19. Bridwell KH, Lenke LG, McEnery KW, Baldus C, Blanke K. Anterior fresh frozen structural allografts in the thoracic and lumbar spine. Do they work if combined with posterior fusion and instrumentation in adult patients with kyphosis or anterior column defects? Spine (Phila Pa 1976). (1995) 20(12):1410–8. doi: 10.1097/00007632-199506020-00014
20. Hasegawa T, Ushirozako H, Shigeto E, Ohba T, Oba H, Mukaiyama K, et al. The titanium-coated PEEK cage maintains better bone fusion with the endplate than the PEEK cage 6 months after PLIF surgery: a multicenter, prospective, randomized study. Spine (Phila Pa 1976). (2020) 45(15):E892–e902. doi: 10.1097/BRS.0000000000003464
21. Li B, Jiang B, Fu Z, Zhang D, Wang T. Accurate determination of isthmus of lumbar pedicle: a morphometric study using reformatted computed tomographic images. Spine (Phila Pa 1976). (2004) 29(21):2438–44. doi: 10.1097/01.brs.0000144355.50660.65
22. Mai HT, Mitchell SM, Hashmi SZ, Jenkins TJ, Patel AA, Hsu WK. Differences in bone mineral density of fixation points between lumbar cortical and traditional pedicle screws. Spine J. (2016) 16(7):835–41. doi: 10.1016/j.spinee.2015.11.034
23. Li HM, Zhang RJ, Gao H, Jia CY, Xing T, Zhang JX, et al. Biomechanical fixation properties of the cortical bone trajectory in the osteoporotic lumbar spine. World Neurosurg. (2018) 119:e717–e27. doi: 10.1016/j.wneu.2018.07.253
24. Matsukawa K, Yato Y, Kato T, Imabayashi H, Asazuma T, Nemoto K. In vivo analysis of insertional torque during pedicle screwing using cortical bone trajectory technique. Spine (Phila Pa 1976). (2014) 39(4):E240–5. doi: 10.1097/BRS.0000000000000116
25. Grigoryan G, Inceoglu S, Danisa OA, Cheng W. Sacral endplate penetrating screw for lumbosacral fixation: a cadaveric biomechanical study. Oper Neurosurg (Hagerstown). (2019) 17(4):396–402. doi: 10.1093/ons/opy388
26. Nakashima H, Kanemura T, Satake K, Ito K, Ishikawa Y, Ouchida J, et al. The prevalence and risk factors for S2 alar-iliac screw loosening with a minimum 2-year follow-up. Asian Spine J. (2020) 14(2):177–84. doi: 10.31616/asj.2019.0127
27. Guo HZ, Tang YC, Guo DQ, Ma YH, Yuan K, Li YX, et al. Pedicle screw fixation in single-level, double-level, or multilevel posterior lumbar fusion for osteoporotic spine: a retrospective study with a minimum 2-year follow-up. World Neurosurg. (2020) 140:e121–e8. doi: 10.1016/j.wneu.2020.04.198
28. Banno T, Hasegawa T, Yamato Y, Kobayashi S, Togawa D, Oe S, et al. Prevalence and risk factors of iliac screw loosening after adult spinal deformity surgery. Spine (Phila Pa 1976). (2017) 42(17):E1024–e30. doi: 10.1097/BRS.0000000000002047
29. Matsukawa K, Yato Y, Kato T, Imabayashi H, Asazuma T, Nemoto K. Cortical bone trajectory for lumbosacral fixation: penetrating S-1 endplate screw technique: technical note. J Neurosurg Spine. (2014) 21(2):203–9. doi: 10.3171/2014.3.SPINE13665
30. Su BW, Kim PD, Cha TD, Lee J, April EW, Weidenbaum M, et al. An anatomical study of the mid-lateral pars relative to the pedicle footprint in the lower lumbar spine. Spine (Phila Pa 1976). (2009) 34(13):1355–62. doi: 10.1097/BRS.0b013e3181a4f3a9
31. Iwatsuki K, Yoshimine T, Ohnishi Y, Ninomiya K, Ohkawa T. Isthmus-guided cortical bone trajectory for pedicle screw insertion. Orthop Surg. (2014) 6(3):244–8. doi: 10.1111/os.12122
32. Kaye ID, Prasad SK, Vaccaro AR, Hilibrand AS. The cortical bone trajectory for pedicle screw insertion. JBJS Rev. (2017) 5(8):e13. doi: 10.2106/JBJS.RVW.16.00120
33. Lee CK, Kim D, An SB, Shin DA, Ha Y, Kim KN, et al. An optimal cortical bone trajectory technique to prevent early surgical complications. Br J Neurosurg. (2020) 9:1–7. doi: 10.1080/02688697.2020.1821172
34. Matsukawa K, Yato Y, Nemoto O, Imabayashi H, Asazuma T, Nemoto K. Morphometric measurement of cortical bone trajectory for lumbar pedicle screw insertion using computed tomography. J Spinal Disord Tech. (2013) 26(6):E248–53. doi: 10.1097/BSD.0b013e318288ac39
35. Zhang T, Ren X, Feng X, Diwan A, Luk KDK, Lu WW, et al. Failure mechanisms of pedicle screws and cortical screws fixation under large displacement: a biomechanical and microstructural study based on a clinical case scenario. J Mech Behav Biomed Mater. (2020) 104:103646. doi: 10.1016/j.jmbbm.2020.103646
36. Matsukawa K, Taguchi E, Yato Y, Imabayashi H, Hosogane N, Asazuma T, et al. Evaluation of the fixation strength of pedicle screws using cortical bone trajectory: what is the ideal trajectory for optimal fixation? Spine (Phila Pa 1976). (2015) 40(15):E873–8. doi: 10.1097/BRS.0000000000000983
37. Löffler MT, Sollmann N, Burian E, Bayat A, Aftahy K, Baum T, et al. Opportunistic osteoporosis screening reveals low bone density in patients with screw loosening after lumbar semi-rigid instrumentation: a case-control study. Front Endocrinol (Lausanne). (2020) 11:552719. doi: 10.3389/fendo.2020.552719
38. Pickhardt PJ, Pooler BD, Lauder T, del Rio AM, Bruce RJ, Binkley N. Opportunistic screening for osteoporosis using abdominal computed tomography scans obtained for other indications. Ann Intern Med. (2013) 158(8):588–95. doi: 10.7326/0003-4819-158-8-201304160-00003
39. Zaidi Q, Danisa OA, Cheng W. Measurement techniques and utility of hounsfield unit values for assessment of bone quality prior to spinal instrumentation: a review of current literature. Spine (Phila Pa 1976). (2019) 44(4):E239–e44. doi: 10.1097/BRS.0000000000002813
Keywords: cortical bone trajectory, screw loosening, lumbar spine, nomogram, spine
Citation: Zhang Y, Li Y, Hai Y, Guan L, Zhang X, Pan A, Lu H, Wu B and Liu Y (2022) A nomogram for predicting screw loosening after single-level posterior lumbar interbody fusion utilizing cortical bone trajectory screw: A minimum 2-year follow-up study. Front. Surg. 9:950129. doi: 10.3389/fsurg.2022.950129
Received: 22 May 2022; Accepted: 23 August 2022;
Published: 13 September 2022.
Edited by:
Vadim Byvaltsev, Irkutsk State Medical University, RussiaReviewed by:
Xiaolong Chen, University of New South Wales, AustraliaTeng Zhang, The University of Hong Kong, Hong Kong, SAR China
© 2022 Zhang, Li, Hai, Guan, Zhang, Pan, Lu, Wu and Liu. This is an open-access article distributed under the terms of the Creative Commons Attribution License (CC BY). The use, distribution or reproduction in other forums is permitted, provided the original author(s) and the copyright owner(s) are credited and that the original publication in this journal is cited, in accordance with accepted academic practice. No use, distribution or reproduction is permitted which does not comply with these terms.
*Correspondence: Yuzeng Liu beijingspine2010@163.com Yong Hai yong.hai@ccmu.edu.com
†ORCID Yiqi Zhang orcid.org/0000-0001-5812-7803 Yue Li orcid.org/0000-0001-6924-8969 Yuzeng Liu orcid.org/0000-0003-2128-4594 Yong Hai orcid.org/0000-0002-7206-325X
‡These authors have contributed equally to this work
Specialty Section: This article was submitted to Neurosurgery, a section of the journal Frontiers in Surgery