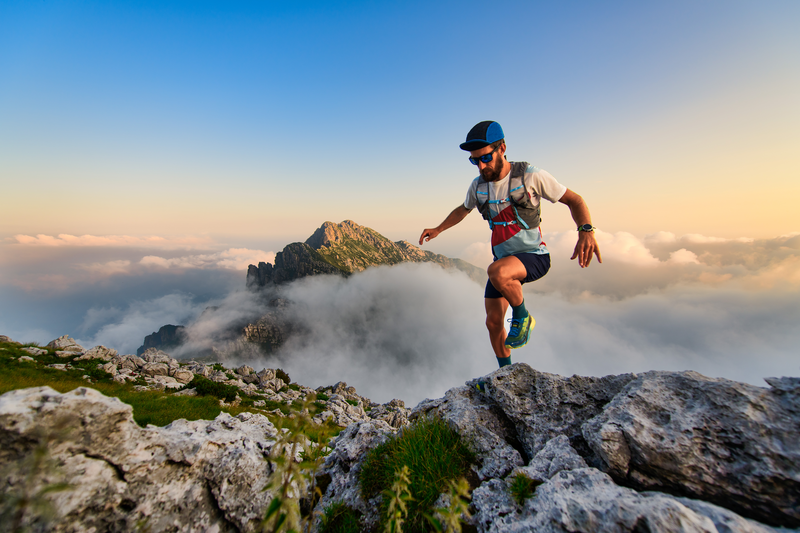
94% of researchers rate our articles as excellent or good
Learn more about the work of our research integrity team to safeguard the quality of each article we publish.
Find out more
CASE REPORT article
Front. Surg. , 27 July 2022
Sec. Neurosurgery
Volume 9 - 2022 | https://doi.org/10.3389/fsurg.2022.936259
Epidural electrical stimulation (EES) has been used to improve motor function in patients with chronic spinal cord injury (SCI). The effect of EES on paravertebral muscles in patients with SCI has been unnoticed. We reported a case of paravertebral muscles hypertrophy after the electrode shifted in a patient with spinal cord injury. We also discussed possible mechanistic accounts for this occurs.
Spinal cord injury (SCI) can cause certain physiological and pathological changes in muscle fibers, among which muscle atrophy is the most critical (1, 2). Epidural electrical stimulation (EES) can activate the lumbar central pattern generator and allow patients with SCI to produce rhythmic movement, thus helping them achieve independent standing and physical stability (3–8). The paravertebral muscles are important for maintaining standing posture and balance. At present, no study has reported the effect of EES on the paravertebral muscles in patients with SCI. We reported a case of paravertebral muscles hypertrophy after the electrode shifted in a patient with spinal cord injury.
A 45-year-old man with T3-T4 level SCI due to a traffic accident 2 years ago. A surgical electrode with 16 contacts was implanted in the patient (Figures 1A,B). The parameter of stimulation was 4.4 V, 210 µm, and 60 Hz. However, the electrode shifted to the left after the surgery (Figure 1C), and the size of paravertebral muscles on this side significantly increased (Figure 2). Finally, we performed revision surgery 6 months after the initial surgery and readjusted the electrode position, and obtained bilateral paravertebral muscles tissue for biopsy.
Figure 1. (A) Surgical electrode with 16 contacts; (B) Surgical electrode was implanted in the spinal canal; (C) The position of the electrode 6 months after the initial surgery.
Figure 2. The images of paravertebral muscles on PET-CT at 3 and 6 months after surgery. (A) The image of paravertebral muscles on PET-CT at 3 months (white arrows indicates hypertrophic paravertebral muscles); (B) The image of paravertebral muscles on PET-CT at 6 months.
We reviewed positron emission tomography-computed tomography (PET-CT) on this patient at 3 and 6 months postoperatively and obtained the cross-sectional areas (CSAs) of the bilateral paravertebral muscles. The fused PET-CT images showed that the CSAs of the bilateral paravertebral muscles in this patient at 6 months after surgery was significantly greater than that in corresponding slices at 3 months (Table 1), especially on the left side (Figure 2).
In addition, we further measured the mean standard uptake value (SUVmean) of 18F-fluorodeoxyglucose (18F-FDG) on the corresponding muscles. The SUVmean on the left paravertebral muscles below the electrode was significantly higher than that on the right at 6 months after surgery (Right: 0.229 ± 0.028, Left: 0.558 ± 0.181).
The muscle biopsy results showed that the muscle fibers on both sides showed compensatory hypertrophy. Type II fibers were the main type of muscle fiber in the bilateral paravertebral muscles. The number of nuclei in some muscle fibers increased, moved inward to the sarcoplasmic cells, and were distributed in bundles, especially on the left side. PAS staining showed increased glycogen in the muscle fibers of the bilateral paravertebral muscles (Figure 3).
Figure 3. Biopsy of paravertebral muscles. (A) Hematoxylin-Eosin (HE) staining of the left paravertebral muscle, 20× ; (B) HE staining of the right paravertebral muscle, 20×; (C) Nicotinamide adenine dinucleotide tetrazolium oxidoreductase (NADH-TR) staining, 10×; (D) Periodic acid-schiff (PAS) staining, 20×.
In SCI, joint activities of muscles are impaired due to denervated atrophy (9). Skeletal muscles are the foundation for maintaining posture and balance. EES promotes gait-related muscle recruitment by activating the lumbar central pattern generator, enabling patients with SCI to stand independently and achieve physical stability (3, 7, 10–12). EES can not only be used to treat traumatic SCI, but also has attractive prospects in the management of other forms of chronic SCI such as myelopathic changes induced by cervical spondylotic myelopathy or central cord syndrome.
This patient with SCI underwent EES and showed abnormal hypertrophy of the paravertebral muscle on one side due to electrode shift after surgery. The CSAs of the paravertebral muscles on both sides significantly increased after EES. Due to the asymmetric hypertrophy of the bilateral paravertebral muscles, we speculate that the influence of electrode movement on the bilateral muscles may be different. The SUVmean values of 18F-FDG on the left paravertebral muscles below the electrode were significantly higher than those on the right. Enhancing metabolism by EES might be a factor in promoting muscle hypertrophy.
Hypertrophy of the skeletal muscle occurs by promoting satellite cell activation and maintaining protein balance (13–16). Khodabukus et al. (14) found that the number of nuclei in the cross-section of the muscle bundle after electrical stimulation increased by 1.5 times compared with that in the non-stimulated group. In addition, the CSA of the muscle bundles in the electrically stimulated group increased by 1.8 times compared with that in the non-stimulated group. Electrical stimulation promotes human muscle hypertrophy, myotube maturation, and increased metabolism.
Some studies have shown that the mammalian target of rapamycin-1 can be activated by 4E-BP1 and S6K, thereby increasing protein synthesis (17–21). Phosphorylation of these proteins significantly increased with electrical stimulation at 10 and 1 Hz, which suggested that frequency-dependent hypertrophy of muscle fibers is the result of increased anabolic signals (14).
In addition, skeletal muscles have high plasticity, which is mainly reflected in the mutual transformation between different types of muscle fibers. These muscles can transform from fast twitch to slow twitch, which can be influenced by internal (genetics and nutrition) and external factors (22). At the waist, type I fibers account for 57% of superficial muscles and 63% of deep muscles (23). Hypertrophy of muscle fibers caused by electrical stimulation may be more obvious in type I muscle fibers (24). Muscle biopsy in this patient showed that the fiber types on both sides were almost dominated by type II muscle fibers. The electric pulse emitted by EES may induce transformation from type I to type II fibers.
In addition, the surgical electrode shift resulted not only in hypertrophy of paravertebral muscles, but also in an imbalance in the electrical impulses received by both sides. In order to prevent electrode shifted, the surgical electrode should be secured with a fixed anchor. The fixed anchor should be as close to the surgical electrode as possible. In addition, the position of surgical electrode after fixation was determined by intraoperative localization. If the electrode has shifted, revision surgery should be performed timely.
In this case, the paravertebral muscles on the side of the electrode shifted were abnormally hypertrophy. We surmise that EES may affect the metabolism of these muscles and lead to skeletal muscle hyperplasia. In addition, EES may be involved in regulating transformation between different muscle fiber types. However, since only one case was reported, this conjecture requires further experimental confirmation.
The raw data supporting the conclusions of this article will be made available by the authors, without undue reservation.
The studies involving human participants were reviewed and approved by The Ethics Committee of Tianjin Medical University General Hospital (NO. ZYY-IRB-SOP-016(F)-002-04). The patients/participants provided their written informed consent to participate in this study.
Written informed consent was obtained from the individual for the publication of any potentially identifiable images or data included in this article.
SL and HR were contributed equally to this article and mainly responsible for article writing. TZ and HR were mainly responsible for surgical design, and implementation. SL, ZH, RT and HL were mainly responsible for image acquisition and data collection. TZ was mainly responsible for article revising and improving. All authors contributed to the article and approved the submitted version.
This work was supported by Scientific Research Program of Tianjin Municipal Science and Technology Bureau (NO. 19ZXDBSY00040).
The authors declare that the research was conducted in the absence of any commercial or financial relationships that could be construed as a potential conflict of interest.
All claims expressed in this article are solely those of the authors and do not necessarily represent those of their affiliated organizations, or those of the publisher, the editors and the reviewers. Any product that may be evaluated in this article, or claim that may be made by its manufacturer, is not guaranteed or endorsed by the publisher.
1. Castro MJ, Apple DF Jr, Hillegass EA, Dudley GA. Influence of complete spinal cord injury on skeletal muscle cross-sectional area within the first 6 months of injury. Eur J Appl Physiol Occup Physiol. (1999) 80:373–8. doi: 10.1007/s004210050606
2. Chandrasekaran S, Davis J, Bersch I, Goldberg G, Gorgey AS. Electrical stimulation and denervated muscles after spinal cord injury. Neural Regen Res. (2020) 15:1397–407. doi: 10.4103/1673-5374.274326
3. Angeli CA, Boakye M, Morton RA, Vogt J, Benton K, Chen Y, et al. Recovery of over-ground walking after chronic motor complete spinal cord injury. N Engl J Med. (2018) 379:1244–50. doi: 10.1056/NEJMoa1803588
4. Bussel B, Roby-Brami A, Azouvi P, Biraben A, Yakovleff A, Held JP. Myoclonus in a patient with spinal cord transection. Possible involvement of the spinal stepping generator. Brain. (1988) 111(Pt 5):1235–45. doi: 10.1093/brain/111.5.1235
5. Calvert JS, Grahn PJ, Zhao KD, Lee KH. Emergence of epidural electrical stimulation to facilitate sensorimotor network functionality after spinal cord injury. Neuromodulation. (2019) 22:244–52. doi: 10.1111/ner.12938
6. Danner SM, Hofstoetter US, Freundl B, Binder H, Mayr W, Rattay F, et al. Human spinal locomotor control is based on flexibly organized burst generators. Brain. (2015) 138:577–88. doi: 10.1093/brain/awu372
7. Harkema S, Gerasimenko Y, Hodes J, Burdick J, Angeli C, Chen Y, et al. Effect of epidural stimulation of the lumbosacral spinal cord on voluntary movement, standing, and assisted stepping after motor complete paraplegia: a case study. Lancet. (2011) 377:1938–47. doi: 10.1016/S0140-6736(11)60547-3
8. Minassian K, Jilge B, Rattay F, Pinter MM, Binder H, Gerstenbrand F, et al. Stepping-like movements in humans with complete spinal cord injury induced by epidural stimulation of the lumbar cord: electromyographic study of compound muscle action potentials. Spinal Cord. (2004) 42:401–16. doi: 10.1038/sj.sc.3101615
9. Doucet BM, Lam A, Griffin L. Neuromuscular electrical stimulation for skeletal muscle function. Yale J Biol Med. (2012) 85:201–15.22737049
10. Huang H, He J, Herman R, Carhart MR. Modulation effects of epidural spinal cord stimulation on muscle activities during walking. IEEE Trans Neural Syst Rehabil Eng. (2006) 14:14–23. doi: 10.1109/TNSRE.2005.862694
11. Wagner FB, Mignardot JB, Le Goff-Mignardot CG, Demesmaeker R, Komi S, Capogrosso M, et al. Targeted neurotechnology restores walking in humans with spinal cord injury. Nature. (2018) 563:65–71. doi: 10.1038/s41586-018-0649-2
12. Young W. Electrical stimulation and motor recovery. Cell Transplant. (2015) 24:429–46. doi: 10.3727/096368915X686904
13. Handschin C, Mortezavi A, Plock J, Eberli D. External physical and biochemical stimulation to enhance skeletal muscle bioengineering. Adv Drug Deliv Rev. (2015):82-83:168-75. doi: 10.1016/j.addr.2014.10.021
14. Khodabukus A, Madden L, Prabhu NK, Koves TR, Jackman CP, Muoio DM, et al. Electrical stimulation increases hypertrophy and metabolic flux in tissue-engineered human skeletal muscle. Biomaterials. (2019) 198:259–69. doi: 10.1016/j.biomaterials.2018.08.058
15. Schiaffino S, Dyar KA, Ciciliot S, Blaauw B, Sandri M. Mechanisms regulating skeletal muscle growth and atrophy. FEBS J. (2013) 280:4294–314. doi: 10.1111/febs.12253
16. Shadrin IY, Khodabukus A, Bursac N. Striated muscle function, regeneration, and repair. Cell Mol Life Sci. (2016) 73:4175–202. doi: 10.1007/s00018-016-2285-z.27271751
17. Baar K, Esser K. Phosphorylation of p70(S6k) correlates with increased skeletal muscle mass following resistance exercise. Am J Physiol. (1999) 276:C120–7. doi: 10.1152/ajpcell.1999.276.1.C120.9886927
18. Bodine SC, Stitt TN, Gonzalez M, Kline WO, Stover GL, Bauerlein R, et al. Akt/mTOR pathway is a crucial regulator of skeletal muscle hypertrophy and can prevent muscle atrophy in vivo. Nat Cell Biol. (2001) 3:1014–9. doi: 10.1038/ncb1101-1014
19. Terzis G, Georgiadis G, Stratakos G, Vogiatzis I, Kavouras S, Manta P, et al. Resistance exercise-induced increase in muscle mass correlates with p70S6 kinase phosphorylation in human subjects. Eur J Appl Physiol. (2008) 102:145–52. doi: 10.1007/s00421-007-0564-y
20. Ma XM, Blenis J. Molecular mechanisms of mTOR-mediated translational control. Nat Rev Mol Cell Biol. (2009) 10:307–18. doi: 10.1038/nrm2672
21. Marabita M, Baraldo M, Solagna F, Ceelen J, Sartori R, Nolte H, et al. S6k1 is required for increasing skeletal muscle force during hypertrophy. Cell Rep. (2016) 17:501–13. doi: 10.1016/j.celrep.2016.09.020
22. Xu M, Chen X, Huang Z, Chen D, Yu B, Chen H, et al. Grape seed proanthocyanidin extract promotes skeletal muscle fiber type transformation via AMPK signaling pathway. J Nutr Biochem. (2020) 84:108462. doi: 10.1016/j.jnutbio.2020.108462
23. Sirca A, Kostevc V. The fibre type composition of thoracic and lumbar paravertebral muscles in man. J Anat. (1985) 141:131–7.2934358
Keywords: epidural electrical stimulation, paravertebral muscles, 18F-fluorodeoxyglucose, spinal cord injury, case report
Citation: Li S, Rong H, Hao Z, Tan R, Li H and Zhu T (2022) Hypertrophy of paravertebral muscles after epidural electrical stimulation shifted: A case report. Front. Surg. 9:936259. doi: 10.3389/fsurg.2022.936259
Received: 5 May 2022; Accepted: 13 July 2022;
Published: 27 July 2022.
Edited by:
Mario Ganau, Oxford University Hospitals NHS Trust, United KingdomReviewed by:
S. Ottavio Tomasi, Paracelsus Medical University, Austria© 2022 Li, Rong, Hao, Tan, Li and Zhu. This is an open-access article distributed under the terms of the Creative Commons Attribution License (CC BY). The use, distribution or reproduction in other forums is permitted, provided the original author(s) and the copyright owner(s) are credited and that the original publication in this journal is cited, in accordance with accepted academic practice. No use, distribution or reproduction is permitted which does not comply with these terms.
*Correspondence: Tao Zhu emh1dGFvNUAxMjYuY29t
Specialty Section: This article was submitted to Neurosurgery, A section of the journal Frontiers in Surgery
Disclaimer: All claims expressed in this article are solely those of the authors and do not necessarily represent those of their affiliated organizations, or those of the publisher, the editors and the reviewers. Any product that may be evaluated in this article or claim that may be made by its manufacturer is not guaranteed or endorsed by the publisher.
Research integrity at Frontiers
Learn more about the work of our research integrity team to safeguard the quality of each article we publish.