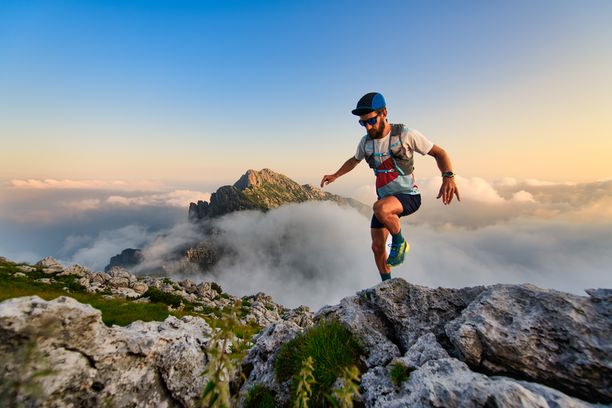
95% of researchers rate our articles as excellent or good
Learn more about the work of our research integrity team to safeguard the quality of each article we publish.
Find out more
REVIEW article
Front. Surg., 12 August 2022
Sec. Reconstructive and Plastic Surgery
Volume 9 - 2022 | https://doi.org/10.3389/fsurg.2022.933781
This article is part of the Research TopicApplication of Stem Cell Therapy and Bioinformatics in Wound Repair and Skin DiseasesView all 13 articles
Wound healing is a complex and integrated process of the interaction of various components within the injured tissue. Accumulating evidence suggested that stem cell-derived exosomal transcriptomes could serve as key regulatory molecules in wound healing in stem cell therapy. Stem cell-derived exosomal transcriptomes mainly consist of long noncoding RNAs (lncRNAs), microRNAs (miRNAs), circular RNAs (circRNAs) and messenger RNAs (mRNAs). In this article we presented a brief introduction on the wound repair process and exosomal transcriptomes. Meanwhile, we summarized our current knowledge of the involvement of exosomal transcriptomes in physiological and pathological wound repair process including inflammation, angiogenesis, and scar formation.
Skin wounds are classified primarily into acute and chronic wounds. Acute wounds included cuts, scrapes, burns, trauma, needle punctures, and surgical incisions. While chronic wounds refer to wounds like diabetic foot ulcers, pressure ulcers and infected wounds (1). The healing of wound is the process of tissue regeneration involving the synergistic and integrated actions of cells and intercellular factors, cells and cells, cells and the microenvironment. The normal wound healing involves four successive but overlapping phases, including the hemostasis phase, the inflammatory phase, the proliferative phase, and the remodeling phase (2). Many factors affect and interfere with normal wound healing, such as infection, medication, complex comorbidities, age, obesity, smoking and nutrition (3, 4). Any disturbance in these four phases affects wound healing or even proper functioning of the skin, results in the formation of chronic non-healing wounds or repaired with hypertrophic scar tissue or keloids (5).
Currently, therapeutic research on wound healing is focused on the use of small molecules, including growth factors, insulin, stromal cell-derived factor-1, antimicrobial peptides, platelet rich plasma, and so on (6). In addition, silk biomaterials, artificial skin grafts, honey are also potential therapeutic means for wound healing (7–9). In recent decades, stem cell therapy has become a promising approach in wound healing due to its distinguished functions on tissue regeneration, immunoregulation and angiogenesis (10). Stem cells participate in the regulation of inflammatory, proliferative and remodeling phases via paracrine of cytokines, chemokines and growth factors. Stem cells ranging from immature pluripotent stem cells to more restricted multipotent progenitor cells have been investigated for their abilities in facilitating wound healing in several animal models and clinical trials (11). However, because stem cells do not readily survive in the wound microenvironment in a large portion of cases, their effects in wound healing may be difficult to be accomplished (12). One fundamental hurdle is that reactive oxygen species can weaken stem cell function and therefore impair wound healing (13). In addition, small molecules are easily degraded in wound fluids and carry risks of drug resistance, new therapies are still being explored.
Stem cell-derived exosomes have demonstrated great potentials to provide therapeutical benefits in nearly all stages of wound healing process, including alleviating inflammation (14), promoting vascularization (15), promoting proliferation and migration of epithelial cells and fibroblasts (16), while reducing scar formation (17). However, the exact mechanism of action remains to be explored. Growing evidences have shown that exosome-derived transcriptomes (RNAs) participate in comprehensive biological and functional aspects of physiological conditions (18–20). Therefore, stem cell derived exosomal transcriptomes attracted great research interests (21–24). In this review, we summarized the process of wound healing and several key RNAs of exosome transcriptomes involved, such as messenger RNAs (mRNAs), microRNAs (miRNAs), long non-coding RNAs (lncRNAs) and circular RNAs (circRNAs), with the focus on the effects of several transcriptional molecules of stem cell derived exosomes on wound healing.
Wound healing is a complex and dynamic process to skin injury. Wound healing is classically divided into four overlapping phases: hemostasis, inflammation, proliferation and remodeling. During the hemostatic phase, platelets and circulating coagulant factors accumulate and interact at the site of tissue injury, followed by activation of endothelial cells. Inflammation state involves complex mechanisms among resident immune cells, circulating leucocytes and their pro-inflammatory cytokines and chemokines. Uncontrolled and excessive inflammation promotes tissue injury and delays healing, thereby causing chronic wounds or scars. The proliferative phase of healing is characterized by extensive activation of keratinocytes, epidermal stem cells, fibroblasts, macrophages and endothelial cells to orchestrate wound closure and angiogenesis. Extracellular matrix (ECM) remodeling spans the entire wound healing process. Fibroblasts, myofibroblasts, collagen, transforming growth factor (TGF)-β, proteoglycans and matrix metalloproteinases are major players involved in ECM remodeling, balancing collagen synthesis and degradation (25, 26).
Disturbance of any of the above stages of the normal wound healing process can lead to pathological wound healing, which mainly includes two types: excessive scarring and chronic wound healing. Persistent activation of TGF-β pathway provided a deviant signal to myofibroblasts, leading to continuous production of ECM triggering pathological scarring (27). Hyper-proliferation of fibroblasts and excessive collagen deposition may lead to hypertrophic scar formation as well (28). Chronic wounds are typically caused by a temporal arrest in the inflammation phase with no further progress to the stages of healing. Chronic wounds are characterized by massive immune cell infiltration, excessive levels of proinflammatory cytokines, persistent infection, the formation of biofilms of drug-resistant microorganisms, and appearance of senescent cells which are unresponsive to restorative stimuli (29, 30).
Exosomes are bioactive membranous vesicles with a diameter of 40–100 nm secreted by many type of cells. They were discovered for the first time in sheep reticulocytes in 1983 (31). Exosome formation begins with endocytosis on the surface of the cell membrane with the formation of early endosomes via inward budding (32). Recently, various nucleic acids have been identified within exosomes, including mRNAs and non-coding RNAs such as miRNAs, lncRNAs, circRNAs, ribosomal RNAs (rRNAs), transfer RNAs (tRNAs), small nucleolar RNAs (snoRNAs), small nuclear RNAs (snRNAs) and piwi-interacting RNAs (piRNAs). These RNAs are transferred from parent cells to recipient cells through exosomes to exert specific functions (33, 34). Secreted exosomes can be transferred to recipient cells via endocytosis, direct membrane fusion, and receptor ligand interactions. Exosomes are associated with a wide spectrum of physiological and pathological processes including but not limited to immune responses, viral pathogenicity, pregnancy, cardiovascular diseases, central nervous system-related diseases, cancer progression and so on. In addition, exosomes have potential as diagnostic and therapeutic tools for a variety of diseases, including neurodegenerative diseases, cardiovascular dysfunction, and cancer (35).
Exosomal transcriptomes mainly contain mRNAs, lncRNAs, miRNAs and circRNAs, which have been intensively studied in a number of studies. mRNAs, macromolecules carry codes from the DNA in the nucleus to the cytoplasm (the ribosomes), where protein synthesis occurs, were first found highly enriched in exosomes released by mast cells (36, 37). mRNAs in exosomes differ significantly from those in the cytoplasm of the donor cells (37). Exosomal mRNAs could be transferred to other cells and be translated into proteins in the target cells (37, 38). Exosomes derived from cells growing under oxidative stress may induce tolerance of the recipient cells to external stress by mRNAs transported by exosomes (39). In addition, exosomal mRNAs may potentially play a role in epigenetic inheritance in mammals (40).
Although 70% of the human genome is transcribed into RNAs, only 2% of these transcripts are translated into proteins. The remaining transcripts are defined as noncoding RNAs, including lncRNAs, miRNAs and circRNAs, which participate in the regulation of mRNA and protein function, in the binding with DNA to modulate gene transcription, or in acting as the competing endogenous RNA (ceRNA) or miRNA sponges to regulate gene expression (Figure 1). LncRNAs are a category of cellular RNAs which are longer than 200 nucleotides in length but in lack of the open reading frames. This class comprises, among others, long intergenic noncoding RNAs (lincRNAs), antisense RNAs, and sense overlapping lncRNAs. LncRNAs may serve as biomarkers of cancer, such as nuclear enriched abundant transcript 1 (NEAT1), HOX Transcript Antisense RNA (HOTAIR), metastasis-associated lung adenocarcinoma transcript 1 (MALAT-1), H19. Moreover, lncRNAs participated in cancer development, progression, metastasis and prognosis (41), and other diseases like cerebrovascular diseases, cardiac diseases, inflammatory bowel diseases, metabolic syndrome related disorders as well (42–45).The abundance of exosomal lncRNAs correlates with their expression levels in the cell of origin (46).
Figure 1. Intercellular transcriptomes delivery by exosomes. Exosomal noncoding RNAs, mainly microRNAs (miRNAs), long noncoding RNAs (lncRNAs), and circular RNAs (circRNAs) from donor cells act as competitive endogenous RNAs (ceRNAs) for binding to common miRNA response elements (MREs) from recipient cells altering their activity.
Among the RNAs in exosomes, miRNAs have attracted the most attention due to their roles in gene expression regulation. MiRNAs are a class of 17–24 nt, small, noncoding and evolutionarily conserved RNAs, They can mediate post-transcriptional gene silencing by targeting the 3′-UTR of target mRNAs under the control of the RNA-induced silencing complex (47–49). MiRNAs can regulate cell proliferation and differentiation (50), and thus participate in development (51, 52). They can also serve as therapeutic targets and prognostic markers for diseases and cancers (53, 54).
CircRNAs are a new category of single-stranded RNAs characterized by their covalently closed loop structures lacking of 5′ caps or a 3′ poly(A) tails in all eukaryotic cells. They are generated through a particular mechanism of alternative splicing called “back-splicing”. In 2015, Li et al. reported for the first time that exosomes contained abundant circRNAs. Genome-wide RNA-seq analysis found that circRNAs were enriched in the exosomes relative to the parental cells. It has been reported that the sorting of circRNAs to exosomes can be controlled by modulating the levels of relevant miRNAs in parental cells and can transfer bioactive substance to target cells (55). It has been confirmed that circRNAs could exert biological functions in multiple aspects, including participating in neuronal development (56) and the pathogenesis of cancer, cardiovascular, neurological, and autoimmune diseases (57–60). In addition, circRNAs serve as potential biomarkers for many diseases (61), especially cancer (62).
Harsh wound microenvironments, such as deficiency of nutritional factors, enhanced inflammatory response, increased reactive oxygen species, and impaired vasculature, all resulted in poor survival of the transplanted stem cells (63).
Stem cell derived exosomes carrying bioactive contents of parental stem cells, transmit signals and participate in the remodeling of extracellular matrix through paracrine mechanism. Exosomes are less immunogenic, more stable and easier to store relative to their donor stem cells, and therefore can be used as an alternative therapy for stem cells in wound healing regulation. There have been clinical trials on stem cell derived exosomes for the treatment of diabetic wounds registered on the Clinicaltrial.gov website [NCT05243368]. Besides, patents on stem cell derived exosomes as wound dressings have been published [CN114376989A&CN114377194A]. However, the low yields of stem cell derived exosomes require a large number of stem cells to be consumed. Exosome components are complex and can hardly be purified due to the limitation of extraction techniques. The transcriptomes of exsomes, however, as intercellular transport cargos of exosomes, is relatively well-defined in composition and more suitable for research. Moreover, their functions in wound healing can be defined by loss- or gain-of-function experiments after overexpression or knockdown their expressions in the parent cells. Unlike the great risks such as mutagenesis, toxicity, and limited capacity for genetic cargo posed by viral vectors, exosomal RNAs transferring presented good safety and biocompatibility (64).
At present, research on stem cell derived exosomes mainly focuses on those derived from mesenchymal stem cells (65–67). However, there are still some studies on exosomes from other sources of stem cells, such as pluripotent stem cells (68, 69) or embryonic stem cells (70). Consistent with the major functions of the parental cells, exosomes derived from MSCs embrace immunomodulatory as well as regenerative effects on the recipient cells (21, 71).
The stem cell derived exosomal transcriptomes have been shown to promote wound healing in terms of anti-inflammation, cell proliferation and migration, blood vessel formation, and inhibition of scar formation, and the effects on wound healing of four transcriptomes, including miRNAs, lncRNAs, circRNAs, as well as mRNAs, will be illustrated below (see Figure 2).
Figure 2. Schematic demonstration of the exosomal RNAs derived from stem cells promote wound healing by enhancing angiogenesis, cell proliferation and migration, reducing inflammation, and anti-scarring.
A number of studies have demonstrated that miRNAs were the active molecules of stem cell derived exosomes to regulate the functions of the recipient cells (72, 73). In wound healing for examples, miRNAs in the stem cell derived-exosomes have shown potent effects on regeneration, anti-inflammatory and anti-scarring to the wounds (see Table 1).
During wound healing process, regeneration is mainly manifested by promoting fibroblasts proliferation, boosting vascularization, formation of granulation tissue, and enhancing re-epithelialization, among other phathophyioloigcal events. Four miRNAs (miR-126-3p, miR-21-3p, miR-146a-3p and miR-21-5p) were reported to have the potentials to enhance the proliferation and migration of the fibroblasts and endothelial cells, and to promote angiogenesis of human endothelial cells through phosphatase and tension homologs (PTEN), PI3K/AKT and ERK signaling pathways, respectively (74–77). Local application of miRNA-21-3p-enriched umbilical cord blood-exosomes into mouse skin wounds accelerated re-epithelialization, reduced scar width, and enhanced angiogenesis. Inhibition of PTEN and sprouty RTK signaling antagonist 1 may be the key function of miR-21-3p (75). MiR-146a-3p, derived from ADSC-exosomes could promote the proliferation and migration of fibroblasts by inducing the expressions of serpin family H member 1 and p-ERK2 to rapidly reduce the wound area and foster the formation of new blood vessels in rats with wounds (74). An in vitro study by Tao et al. found that exosomes over-expressing miR-126-3p derived from synovium mesenchymal stem cells stimulated the proliferation of human dermal fibroblasts and human dermal microvascular endothelial cells (HMEC-1) in a dose-dependent manner, and enhanced HMEC-1 migration and tube formation as well. In addition, exosomes overloaded with miR-126-3p accelerated re-epithelialization, activated angiogenesis, and promoted collagen maturation in diabetic wound healing (76). Gao et al. suggested that exosomes enriced in miR-135a from human amniotic mesenchymal stem cells have shown efficacy in enhacing epidermal cell migration to promote wound healing in SD rats. Furthermore, knockdown of miR-135a in ADSC-exosomes validly attenuated the effect of exosomes on BJ fibroblasts migration. The study suggested that the abovementioned function of miR-135a may be realized through inhibiting the expression of large tumor suppressor kinase 2 (78).
MiRNAs from other stem cell derived exosomes have been reported to enhance cell proliferation and migration to improve wound healing. MiR-200a-enriched embryonic stem cell derived exosomes were capble of rejuvenating vascular endothelial cell senescence and restore impaired proliferation, migration, and tube formation by downregulating Keap1 to activated nuclear factor erythroid2-related factor 2 (79).
Although most stem cell-exosomes derived miRNAs can promote cell proliferation, migration, and angiogenesis, a small proportion of miRNAs play a part in inhibiting wound healing. Exosomes enriched with miR-100-5p and miR-1246 from human deciduous tooth exfoliated dental stem cells were found to decrease cell proliferation and migration of HUVECs, induce cell apoptosis, and suppress tube like structure formation of HUVECs by downregulating several angiogenesis related factors, including VEGFA, MMP-9 and angiopoietin 1 (80), suggesting that miR-100-5p and miR-1246 play an anti-angiogenic role in wound healing.
The inflammatory response performs multiple tasks at the wound site by improving wound debridement and producing chemokines, metabolites, and growth factors. If this orchestrated response becomes dysregulated, wounds can become chronic or progressive fibrosis, both of which can impair tissue function and ultimately lead to organ failure and even death. Investigations have shown that miR-181c in UCMSC exosomes played a critical role in regulating burn induced inflammation. Exosomes overexpressing miR-181c potently inhibited toll-like receptor 4 (TLR4) signaling and attenuated inflammation in skin-burned rats, and significantly reduced lipopolysaccharide (LPS) induced TLR4 expression and inflammatory responses in macrophages (81). Besides, macrophages have been shown to play a central role in the inflammatory phase of tissue repair, such as the removal of dead cells, debris and pathogens. The dynamic plasticity allows them to mediate tissue damage and repair functions. For instance, LPS-pretreated UCMSC-derived exosomes can uniquely express miR let-7b, and upregulate of the expression of anti-inflammatory cytokines and promote M2 macrophage activation. Let-7b regulated macrophage polarization by downregulating TLR4/NF-κB/STAT3/AKT signaling pathway (82). In another study, miR-223 derived from BMSC-exosomes regulated macrophage polarization by targeting pknox1, suggesting that macrophage M2 polarization may accelerate wound healing via miR-223 (83). MiR-19b, derived from ADSC exosomes, were significantly increased in recipient cells and were able to decrease inflammatory CCL1 levels acting through TGF-β pathway in H2O2 pretreated HaCaT cells (84).
Skin fibrosis results from poor wound healing following severe tissue damage such as severe burns, trauma, and major surgery. Pathological skin fibrosis tends to cause scarring. Dysregulation of each stage of wound healing, including the inflammatory, proliferative and remodeling stages, can lead to skin fibrosis (85). In vivo, ADSC derived exosomes over-expressing miR-29a inhibited the proliferation, migration, fibrosis and scar hyperplasia of human hypertrophic scar fibroblasts after scald wound in mice by targeting TGF-β2/Smad3 signaling pathway (86). Besides, ADSC exosomal miR-192-5p reduced pro-fibrotic protein levels and ameliorated hypertrophic scar fibrosis in mice through the IL-17RA /Smad axis (87).
Myofibroblasts aggregation also results in excessive scarring. A study examined UCMSC exosomal miRNAs by high-throughput sequencing and found that a group of specific miRNAs (miR-21, miR-23a, miR-125b and miR-145) played a key role during myofibroblast formation. In vivo and in vitro studies have validated that these miRNAs inhibited α-smooth muscle actin formation and collagen deposition through suppressing TGFβ/Smad2 axis (88).UCB exosomes were identified to contain abundant miRNAs. MiR-21-5p and miR-125b-5p from UCB exosomes were found essential for TGF-β1-induced anti-myofibroblast differentiation in human dermal fibroblasts by repressing receptor type II (TGFBR2) and TGF-β receptor type I (TGFBR1) respectively (17).
Stem cell-derived exosomal lncRNAs promotes wound healing in cell proliferation, migration, angiogenesis, anti-inflammatory and anti-fibrosis (see Table 2).
The lncRNA MALAT 1, a lncRNA abundant in exosomes from ADSCs, was validated to have the function in promoting human dermal fibroblasts migration and ischemic wound healing (89). Another study demonstrated that MALAT1-enriched exosomes derived from ADSCs promoted cell proliferation, migration and apoptosis in H2O2 induced HaCaT and human dermal fibroblasts by targeting miR-124 and activating the Wnt/β-catenin pathway (90).
LncRNA H19 (H19) was one of the first discovered lncRNAs and was found enriched in MSC-exosomes. ADSC exosomes inhibited the expression of miR-19b via lncRNA H19, thereby upregulating SOX9 to activate the Wnt/β-catenin pathway, resulting in accelerating the proliferation, migration and invasion of human skin fibroblasts. In vivo experiments also confirmed that exosomes from ADSCs promoted mouse skin wound healing through H19 (91). In a streptozotocin-induced diabetic foot mouse model, BMSCs-derived lncRNA H19 transferred to fibroblasts via exosomes, prevented fibroblasts apoptosis and inflammation by attenuating miR-152-3p-mediated PTEN repression to improve diabetic wound healing (92).
Another lncRNA KLF3-AS1 from BMSC exosomes induced angiogenesis to promote wound healing in diabetic skin. KLF3-AS1 accelerated the proliferation, migration and tube formation of HUVECs, while inhibited the apoptosis of HUVECs challenged by high glucose. In vivo, exosomes from BMSCs over-expressing KLF3-AS1 also promoted skin wound healing in diabetic mice through increasing angiogenesis, and decreasing inflammation and miR-383 expression (93). Besides, LncRNA GAS 5, which is highly enriched in exosomes from ADSCs, was found to attenuate LPS induced inflammation in human skin fibroblasts, indicating it may have a role in promoting healing of chronic recalcitrant wounds (94). HOTAIR, a long noncoding RNA from extracellular vesicles of BMSCs, was confirmed to participate in promoting angiogenesis and wound healing in diabetic db/db mouse (95).
The roles of circRNAs in wound healing have also received increasing attention because studies have shown that circRNAs involved in the physiological process of wound healing (see Table 2). CircRNAs are crucial in regulating different disease microenvironments. ADSC-exosomes containing high concentrations of mmu_circ_0000250 facilitated the recovery of endothelial progenitor cells (EPCs) function by enhancing autophagy, and reducing apoptosis of EPCs under high glucose conditions. In diabetic mouse wounds, mmu_circ_0000250-enriched exosomes facilitated skin angiogenesis and inhibited apoptosis through autophagy activation via inhibiting miR-128-3p and upregulating SIRT1 (96). Circ-Gcap14 from hypoxic preconditioned ADSCs increased the expression of angiogenic growth factors and accelerated diabetic wound closure by inhibiting downstream miR-18a-5p and promoting the expression of HIF-1α in a mouse wound model (97).
Current studies have confirmed that mRNAs are enriched in exosomes, can be transferred to recipient cells and perform coding functions. Few studies have focused on the contribution of exosomal mRNAs in wound healing. A study revealed that microvesicles derived from UCMSCs improved the dedifferentiation and proliferation of damaged tubular cells by transferring hepatocyte growth factor (HGF) mRNAs and enhancing HGF synthesis, thereby accelerating renal regeneration and delaying fibrosis (98). Deregibus et al. first reported that endothelial progenitor cell-derived microvesicles improved angiogenesis through transfered mRNAs associated with PI3K/AKT and endothelial NOS signaling pathways (99). Future research may focus on how to transport functional mRNAs to encode active molecules for wound healing.
In recent years, there have been a large number of studies confirming that exosomes derived from different stem cell types are effective for wound healing (15, 79, 81, 100, 101). Since the targets of exosomes on recipient cells are not well-defined, the investigation of their molecular mechanisms is necessary. The skin wound healing process is a precisely regulated, therefore, the functions of the transcriptomes of exosomes on skin wound healing are complex. At present, the majority of the research work concentrated on how miRNAs from the stem cell derived exosomal transcriptomes act on fibroblast proliferation and migration in wound healing, and yet a few studies aimed at lncRNAs, circRNAs and mRNAs. This article reviews the major effects of mRNAs, miRNAs, lnc RNAs, circRNAs, and other stem cell derived exosomal transcriptomes on wound healing. Most of these exosomal RNAs were derived from mesenchymal stem cells, especially ADSCs and BMSCs. Although these RNAs are from different sources, their functions are nearly identical.
Exosomes are abundant in RNAs, including coding or non coding RNAs (ncRNAs). It is worth exploring whether RNAs interact with each other. The prevailing hypothesis is competitive endogenous RNA (ceRNA) hypothesis regarding the interplay between RNAs currently. The current research on stem cell derived exosomes and ceRNAs is still lacking. Han et al. investigated the interaction between differentially expressed lncRNAs, miRNAs and mRNAs during wound healing in normal individuals. After analyzing the ceRNA network, four up-regulated lncRNAs (MEG8, MEG3, MIR181A1HG, MIR4435-2HG) were found express during wound healing. MEG8/MEG3 may regulate fibroblast proliferation, differentiation and apoptosis via hsa-miR-296-3p/miR-6763-5p (102).With this lead, more studies regarding the interaction between ceRNA and other RNAs in wound healing are warranted.
To date, there have been a number of studies on the clinical application of ncRNAs which served as biomarkers for judging disease progression and prognosis of patients (103–105). However, the application of stem cell-derived exosomal transcriptomes remains at the basic research stage, and some urgent concerns need to be solved before clinical translation. For example, which source of exosomal transcriptomes is with the most potent efficacy? Which ncRNA plays the major regulatory role? Do lncRNAs alone or co-working with other exosomal components such as lipids and proteins participate in wound healing? How to improve the yields of specific RNAs from exosomes? Although the above challenges exist, with the rise of gene editing technology and the continuous development of RNA delivery techniques, it is foreseeable that stem cell derived exosomal transcriptomes can be widely used in the field of regenerative medicine in the near future.
WZ designed the overall review. GC wrote the manuscript and drew the figures. HC polished the manuscript. WZ and XZ gave final approval of the manuscript. All authors contributed to the article and approved the submitted version.
This work was supported by the special foundation of Guangzhou Key Laboratory (No. 542 202002010004), Guangzhou Science and Technology Plan Project (No. 202201020296), Guangdong Provincial Hospital of Chinese Medicine Science and Technology Research Program (No.YN2019MJ11), the Startup Grant of Guangdong Provincial Hospital of Chinese Medicine to Xiang Zeng (2022KT1032), and the scientific research project of Guangdong Administration of Traditional Chinese Medicine (No. 20211315&20222082).
Author HC was employed by Guangzhou Qinglan Biotechnology Company Limited. The remaining authors declare that the research was conducted in the absence of any commercial or financial relationships that could be construed as a potential conflict of interest
All claims expressed in this article are solely those of the authors and do not necessarily represent those of their affiliated organizations, or those of the publisher, the editors and the reviewers. Any product that may be evaluated in this article, or claim that may be made by its manufacturer, is not guaranteed or endorsed by the publisher.
1. Clark M, Adcock L. Cadth Rapid Response Reports. Honey for Wound Management: A Review of Clinical Effectiveness and Guidelines. Ottawa (ON): Canadian Agency for Drugs and Technologies in Health. Copyright © 2018 Canadian Agency for Drugs and Technologies in Health. (2018).
2. Wang PH, Huang BS, Horng HC, Yeh CC, Chen YJ. Wound healing. J Chin Med Assoc. (2018) 81(2):94–101. doi: 10.1016/j.jcma.2017.11.002
3. Bonifant H, Holloway S. A review of the effects of ageing on skin integrity and wound healing. Br J Community Nurs. (2019) 24(Sup3):S28–S33. doi: 10.12968/bjcn.2019.24.Sup3.S28
5. Thapa RK, Diep DB, Tønnesen HH. Topical antimicrobial peptide formulations for wound healing: current developments and future prospects. Acta Biomater. (2020) 103:52–67. doi: 10.1016/j.actbio.2019.12.025
6. Veith AP, Henderson K, Spencer A, Sligar AD, Baker AB. Therapeutic strategies for enhancing angiogenesis in wound healing. Adv Drug Delivery Rev. (2019) 146:97–125. doi: 10.1016/j.addr.2018.09.010
7. Chouhan D, Mandal BB. Silk biomaterials in wound healing and skin regeneration therapeutics: from bench to bedside. Acta Biomater. (2020) 103:24–51. doi: 10.1016/j.actbio.2019.11.050
8. Kim HS, Sun X, Lee JH, Kim HW, Fu X, Leong KW. Advanced drug delivery systems and artificial skin grafts for skin wound healing. Adv Drug Delivery Rev. (2019) 146:209–39. doi: 10.1016/j.addr.2018.12.014
9. Saikaly SK, Khachemoune A. Honey and wound healing: an update. Am J Clin Dermatol. (2017) 18(2):237–51. doi: 10.1007/s40257-016-0247-8
10. Mazini L, Rochette L, Admou B. Hopes and limits of adipose-derived stem cells (adscs) and mesenchymal stem cells (mscs) in wound healing. Int J Mol Sci. (2020) 21(4):1–19. doi: 10.3390/ijms21041306
11. Duscher D, Barrera J, Wong VW, Maan ZN, Whittam AJ, Januszyk M, et al. Stem cells in wound healing: the future of regenerative medicine? A mini-review. Gerontology. (2016) 62(2):216–25. doi: 10.1159/000381877
12. King A, Balaji S, Keswani SG, Crombleholme TM. The role of stem cells in wound angiogenesis. Adv Wound Care. (2014) 3(10):614–25. doi: 10.1089/wound.2013.0497
13. Gonçalves RV, Costa AMA. Oxidative stress and tissue repair: mechanism, biomarkers, and therapeutics. Oxid Med Cell Longevity. (2021) 2021:6204096. doi: 10.1155/2021/6204096
14. Liu W, Yu M, Xie D, Wang L, Ye C, Zhu Q, et al. Melatonin-Stimulated Msc-derived exosomes improve diabetic wound healing through regulating macrophage M1 and M2 polarization by targeting the Pten/Akt pathway. Stem Cell Res Ther. (2020) 11(1):259. doi: 10.1186/s13287-020-01756-x
15. Zhang J, Guan J, Niu X, Hu G, Guo S, Li Q, et al. Exosomes released from human induced pluripotent stem cells-derived Mscs facilitate cutaneous wound healing by promoting collagen synthesis and angiogenesis. J Transl Med. (2015) 13:49. doi: 10.1186/s12967-015-0417-0
16. Ma T, Fu B, Yang X, Xiao Y, Pan M. Adipose mesenchymal stem cell-derived exosomes promote cell proliferation, migration, and inhibit cell apoptosis via Wnt/Β-catenin signaling in cutaneous wound healing. J Cell Biochem. (2019) 120(6):10847–54. doi: 10.1002/jcb.28376
17. Zhang Y, Pan Y, Liu Y, Li X, Tang L, Duan M, et al. Exosomes derived from human umbilical cord blood mesenchymal stem cells stimulate regenerative wound healing via transforming growth factor-Β receptor inhibition. Stem Cell Res Ther. (2021) 12(1):434. doi: 10.1186/s13287-021-02517-0
18. Yue B, Yang H, Wang J, Ru W. Exosome biogenesis, secretion and function of exosomal mirnas in skeletal muscle myogenesis. Cell Prolif. (2020) 53(7):e12857. doi: 10.1111/cpr.12857
19. Melnik BC, Stremmel W, Weiskirchen R. Exosome-Derived micrornas of human milk and their effects on infant health and development. Biomolecules. (2021) 11(6):1–47. doi: 10.3390/biom11060851
20. Chang W, Wang J. Exosomes and their noncoding Rna cargo are emerging as new modulators for diabetes Mellitus. Cells. (2019) 8(8):1–15. doi: 10.3390/cells8080853
21. Harrell CR, Jovicic N. Mesenchymal stem cell-derived exosomes and other extracellular vesicles as new remedies in the therapy of inflammatory diseases. Cells. (2019) 8(12):1–22. doi: 10.3390/cells8121605
22. Mao Q, Liang XL, Zhang CL, Pang YH, Lu YX. Lncrna Klf3-As1 in human mesenchymal stem cell-derived exosomes ameliorates pyroptosis of cardiomyocytes and myocardial infarction through Mir-138-5p/Sirt1 axis. Stem Cell Res Ther. (2019) 10(1):393. doi: 10.1186/s13287-019-1522-4
23. Zhang X, Sai B, Wang F, Wang L, Wang Y, Zheng L, et al. Hypoxic bmsc-derived exosomal mirnas promote metastasis of lung cancer cells via Stat3-induced Emt. Mol Cancer. (2019) 18(1):40. doi: 10.1186/s12943-019-0959-5
24. Xing X, Li Z, Yang X, Li M, Liu C, Pang Y, et al. Adipose-Derived mesenchymal stem cells-derived exosome-mediated microrna-342-5p protects endothelial cells against atherosclerosis. Aging. (2020) 12(4):3880–98. doi: 10.18632/aging.102857
25. Darby IA, Laverdet B, Bonté F, Desmoulière A. Fibroblasts and myofibroblasts in wound healing. Clin Cosmet Investig Dermatol. (2014) 7:301–11. doi: 10.2147/ccid.s50046
26. Xue M, Jackson CJ. Extracellular matrix reorganization during wound healing and its impact on abnormal scarring. Adv Wound Care. (2015) 4(3):119–36. doi: 10.1089/wound.2013.0485
27. Sarrazy V, Billet F, Micallef L, Coulomb B, Desmoulière A. Mechanisms of pathological scarring: role of myofibroblasts and current developments. Wound Repair Regen. (2011) 19(Suppl 1):S10–5. doi: 10.1111/j.1524-475X.2011.00708.x
28. Zeng G, Zhong F, Li J, Luo S, Zhang P. Resveratrol-mediated reduction of collagen by inhibiting proliferation and producing apoptosis in human hypertrophic scar fibroblasts. Biosci Biotechnol Biochem. (2013) 77(12):2389–96. doi: 10.1271/bbb.130502
29. Bowers S, Franco E. Chronic wounds: evaluation and management. Am Fam Physician. (2020) 101(3):159–66.32003952
30. Wilkinson HN, Hardman MJ. Wound healing: cellular mechanisms and pathological outcomes. Open Biol. (2020) 10(9):200223. doi: 10.1098/rsob.200223
31. Pan BT, Johnstone RM. Fate of the transferrin receptor during maturation of sheep reticulocytes in vitro: selective externalization of the receptor. Cell. (1983) 33(3):967–78. doi: 10.1016/0092-8674(83)90040-5
32. Liu J, Ren L, Li S, Li W, Zheng X, Yang Y, et al. The biology, function, and applications of exosomes in cancer. Acta Pharm Sin B. (2021) 11(9):2783–97. doi: 10.1016/j.apsb.2021.01.001
33. Van den Boorn JG, Dassler J, Coch C, Schlee M, Hartmann G. Exosomes as nucleic acid nanocarriers. Adv Drug Delivery Rev. (2013) 65(3):331–5. doi: 10.1016/j.addr.2012.06.011
34. Xie Y, Dang W, Zhang S, Yue W, Yang L, Zhai X, et al. The role of exosomal noncoding Rnas in cancer. Mol Cancer. (2019) 18(1):37. doi: 10.1186/s12943-019-0984-4
35. Kalluri R, LeBleu VS. The biology, function, and biomedical applications of exosomes. Science. (2020) 367(6478):1–40. doi: 10.1126/science.aau6977
36. Chen M, Xu R, Ji H, Greening DW, Rai A, Izumikawa K, et al. Transcriptome and long noncoding RNA sequencing of three extracellular vesicle subtypes released from the human colon cancer Lim1863 cell line. Sci Rep. (2016) 6:38397. doi: 10.1038/srep38397
37. Valadi H, Ekström K, Bossios A, Sjöstrand M, Lee JJ, Lötvall JO. Exosome-Mediated transfer of MRNAs and MicroRNAs is a novel mechanism of genetic exchange between cells. Nat Cell Biol. (2007) 9(6):654–9. doi: 10.1038/ncb1596
38. Jiang H, Li Z, Li X, Xia J. Intercellular transfer of messenger RNAs in multiorgan tumorigenesis by tumor cell-derived exosomes. Mol Med Rep. (2015) 11(6):4657–63. doi: 10.3892/mmr.2015.3312
39. Eldh M, Ekström K, Valadi H, Sjöstrand M, Olsson B, Jernås M, et al. Exosomes communicate protective messages during oxidative stress; possible role of exosomal shuttle RNA. PLoS One. (2010) 5(12):e15353. doi: 10.1371/journal.pone.0015353
40. Sharma A. Bioinformatic analysis revealing association of exosomal mrnas and proteins in epigenetic inheritance. J Theor Biol. (2014) 357:143–9. doi: 10.1016/j.jtbi.2014.05.019
41. Lorenzi L, Avila Cobos F. Long noncoding RNA expression profiling in cancer: challenges and opportunities. Genes Chromosom Cancer. (2019) 58(4):191–9. doi: 10.1002/gcc.22709
42. Zhang H, Liu B, Shi X, Sun X. Long noncoding rnas: potential therapeutic targets in cardiocerebrovascular diseases. Pharmacol Ther. (2021) 221:107744. doi: 10.1016/j.pharmthera.2020.107744
43. Greco S, Salgado Somoza A, Devaux Y, Martelli F. Long noncoding RNAs and cardiac disease. Antioxid Redox Signal. (2018) 29(9):880–901. doi: 10.1089/ars.2017.7126
44. Lin L, Zhou G, Chen P, Wang Y, Han J, Chen M, et al. Which long noncoding RNAs and circular RNAs contribute to inflammatory bowel disease? Cell Death Dis. (2020) 11(6):456. doi: 10.1038/s41419-020-2657-z
45. Losko M, Kotlinowski J. Long noncoding rnas in metabolic syndrome related disorders. Mediat Inflamm. (2016) 2016:5365209. doi: 10.1155/2016/5365209
46. Naderi-Meshkin H, Lai X, Amirkhah R, Vera J, Rasko JEJ, Schmitz U. Exosomal LncRNAs and cancer: connecting the missing links. Bioinformatics. (2019) 35(2):352–60. doi: 10.1093/bioinformatics/bty527
47. Bartel DP. MicroRNAs: genomics, biogenesis, mechanism, and function. Cell. (2004) 116(2):281–97. doi: 10.1016/s0092-8674(04)00045-5
48. Sonenberg N, Hinnebusch AG. Regulation of translation initiation in eukaryotes: mechanisms and biological targets. Cell. (2009) 136(4):731–45. doi: 10.1016/j.cell.2009.01.042
49. Tafrihi M, Hasheminasab E. Mirnas: biology, biogenesis, their web-based tools, and databases. MicroRNA. (2019) 8(1):4–27. doi: 10.2174/2211536607666180827111633
50. Kabekkodu SP, Shukla V, Varghese VK, D’Souza J, Chakrabarty S, Satyamoorthy K. Clustered mirnas and their role in biological functions and diseases. Biol Rev Camb Philos Soc. (2018) 93(4):1955–86. doi: 10.1111/brv.12428
51. Kosik KS, Nowakowski T. Evolution of new mirnas and cerebro-cortical development. Annu Rev Neurosci. (2018) 41:119–37. doi: 10.1146/annurev-neuro-080317-061822
52. Shvedova M, Kobayashi T. Micrornas in cartilage development and dysplasia. Bone. (2020) 140:115564. doi: 10.1016/j.bone.2020.115564
53. Lu Q, Wu R, Zhao M, Garcia-Gomez A, Ballestar E. Mirnas as therapeutic targets in inflammatory disease. Trends Pharmacol Sci. (2019) 40(11):853–65. doi: 10.1016/j.tips.2019.09.007
54. Shah V, Shah J. Recent trends in targeting mirnas for cancer therapy. J Pharm Pharmacol. (2020) 72(12):1732–49. doi: 10.1111/jphp.13351
55. Li Y, Zheng Q, Bao C, Li S, Guo W, Zhao J, et al. Circular Rna is enriched and stable in exosomes: a promising biomarker for cancer diagnosis. Cell Res. (2015) 25(8):981–4. doi: 10.1038/cr.2015.82
56. Mehta SL, Dempsey RJ, Vemuganti R. Role of circular rnas in brain development and Cns diseases. Prog Neurobiol. (2020) 186:101746. doi: 10.1016/j.pneurobio.2020.101746
57. Cao YZ, Sun JY, Chen YX, Wen CC, Wei L. The roles of circrnas in cancers: perspectives from molecular functions. Gene. (2021) 767:145182. doi: 10.1016/j.gene.2020.145182
58. Li J, Sun C, Cui H, Sun J, Zhou P. Role of circrnas in neurodevelopment and neurodegenerative diseases. J Mol Neurosci. (2021) 71(9):1743–51. doi: 10.1007/s12031-021-01882-y
59. Xia X, Tang X, Wang S. Roles of circrnas in autoimmune diseases. Front Immunol. (2019) 10:639. doi: 10.3389/fimmu.2019.00639
60. Yu Z, Huang Q, Zhang Q, Wu H, Zhong Z. Circrnas open a new era in the study of cardiovascular disease (review). Int J Mol Med. (2021) 47(1):49–64. doi: 10.3892/ijmm.2020.4792
61. Ma Y, Liu Y, Jiang Z. Circrnas: a new perspective of biomarkers in the nervous system. Biomed Pharmacother. (2020) 128:110251. doi: 10.1016/j.biopha.2020.110251
62. Solé C, Mentxaka G, Lawrie CH. The use of circrnas as biomarkers of cancer. Methods Mol Biol. (2021) 2348:307–41. doi: 10.1007/978-1-0716-1581-2_21
63. Sylakowski K, Bradshaw A, Wells A. Mesenchymal stem cell/multipotent stromal cell augmentation of wound healing: lessons from the physiology of matrix and hypoxia support. Am J Pathol. (2020) 190(7):1370–81. doi: 10.1016/j.ajpath.2020.03.017
64. Meng Z, Zhou D, Gao Y, Zeng M, Wang W. Mirna delivery for skin wound healing. Adv Drug Delivery Rev. (2018) 129:308–18. doi: 10.1016/j.addr.2017.12.011
65. Huang Y, He B, Wang L, Yuan B, Shu H, Zhang F, et al. Bone marrow mesenchymal stem cell-derived exosomes promote rotator cuff tendon-bone healing by promoting angiogenesis and regulating M1 macrophages in rats. Stem Cell Res Ther. (2020) 11(1):496. doi: 10.1186/s13287-020-02005-x
66. Liu W, Rong Y, Wang J, Zhou Z, Ge X, Ji C, et al. Exosome-Shuttled mir-216a-5p from hypoxic preconditioned mesenchymal stem cells repair traumatic spinal cord injury by shifting microglial M1/M2 polarization. J Neuroinflammation. (2020) 17(1):47. doi: 10.1186/s12974-020-1726-7
67. Wen Z, Mai Z, Zhu X, Wu T, Chen Y, Geng D. Mesenchymal stem cell-derived exosomes ameliorate cardiomyocyte apoptosis in hypoxic conditions through Microrna144 by targeting the Pten/Akt pathway. Stem Cell Res Ther. (2020) 11(1):36. doi: 10.1186/s13287-020-1563-8
68. Sahoo S, Klychko E, Thorne T, Misener S, Schultz KM, Millay M, et al. Exosomes from human Cd34(+) stem cells mediate their proangiogenic paracrine activity. Circ Res. (2011) 109(7):724–8. doi: 10.1161/circresaha.111.253286
69. Wang Y, Zhang L, Li Y, Chen L, Wang X, Guo W, et al. Exosomes/microvesicles from induced pluripotent stem cells deliver cardioprotective mirnas and prevent cardiomyocyte apoptosis in the ischemic myocardium. Int J Cardiol. (2015) 192:61–9. doi: 10.1016/j.ijcard.2015.05.020
70. Khan M, Nickoloff E, Abramova T, Johnson J, Verma SK, Krishnamurthy P, et al. Embryonic stem cell-derived exosomes promote endogenous repair mechanisms and enhance cardiac function following myocardial infarction. Circ Res. (2015) 117(1):52–64. doi: 10.1161/circresaha.117.305990
71. Ha DH, Kim HK, Lee J, Kwon HH, Park GH, Yang SH, et al. Mesenchymal stem/stromal cell-derived exosomes for immunomodulatory therapeutics and skin regeneration. Cells. (2020) 9(5):1–45. doi: 10.3390/cells9051157
72. Nakano M, Fujimiya M. Potential effects of mesenchymal stem cell derived extracellular vesicles and exosomal mirnas in neurological disorders. Neural Regen Res. (2021) 16(12):2359–66. doi: 10.4103/1673-5374.313026
73. Zhang Y, Kim MS, Jia B, Yan J, Zuniga-Hertz JP, Han C, et al. Hypothalamic stem cells control ageing speed partly through exosomal mirnas. Nature. (2017) 548(7665):52–7. doi: 10.1038/nature23282
74. Md G C, Md Y W, Md L Z, Md Y Z. Effect of microrna-146a modified adipose-derived stem cell exosomes on rat back wound healing. Int J Low Extrem Wounds. (2021):15347346211038092. doi: 10.1177/15347346211038092 [Epub ahead of print].34459668
75. Hu Y, Rao SS, Wang ZX, Cao J, Tan YJ, Luo J, et al. Exosomes from human umbilical cord blood accelerate cutaneous wound healing through Mir-21-3p-mediated promotion of angiogenesis and fibroblast function. Theranostics. (2018) 8(1):169–84. doi: 10.7150/thno.21234
76. Tao SC, Guo SC, Li M, Ke QF, Guo YP, Zhang CQ. Chitosan wound dressings incorporating exosomes derived from microrna-126-overexpressing synovium mesenchymal stem cells provide sustained release of exosomes and heal full-thickness skin defects in a diabetic rat model. Stem Cells Transl Med. (2017) 6(3):736–47. doi: 10.5966/sctm.2016-0275
77. Wu D, Kang L, Tian J, Wu Y, Liu J, Li Z, et al. Exosomes derived from bone mesenchymal stem cells with the stimulation of Fe(3)O(4) nanoparticles and static magnetic field enhance wound healing through upregulated Mir-21-5p. Int J Nanomed. (2020) 15:7979–93. doi: 10.2147/ijn.s275650
78. Gao S, Chen T, Hao Y, Zhang F, Tang X, Wang D, et al. Exosomal Mir-135a derived from human amnion mesenchymal stem cells promotes cutaneous wound healing in rats and fibroblast migration by directly inhibiting Lats2 expression. Stem Cell Res Ther. (2020) 11(1):56. doi: 10.1186/s13287-020-1570-9
79. Chen B, Sun Y, Zhang J, Zhu Q, Yang Y, Niu X, et al. Human embryonic stem cell-derived exosomes promote pressure ulcer healing in aged mice by rejuvenating senescent endothelial cells. Stem Cell Res Ther. (2019) 10(1):142. doi: 10.1186/s13287-019-1253-6
80. Liu P, Zhang Q, Mi J, Wang S, Xu Q, Zhuang D, et al. Exosomes derived from stem cells of human deciduous exfoliated teeth inhibit angiogenesis in vivo and in vitro via the transfer of Mir-100-5p and Mir-1246. Stem Cell Res Ther. (2022) 13(1):89. doi: 10.1186/s13287-022-02764-9
81. Li X, Liu L, Yang J, Yu Y, Chai J, Wang L, et al. Exosome derived from human umbilical cord mesenchymal stem cell mediates Mir-181c attenuating burn-induced excessive inflammation. EBioMedicine. (2016) 8:72–82. doi: 10.1016/j.ebiom.2016.04.030
82. Ti D, Hao H, Tong C, Liu J, Dong L, Zheng J, et al. Lps-Preconditioned mesenchymal stromal cells modify macrophage polarization for resolution of chronic inflammation via exosome-shuttled Let-7b. J Transl Med. (2015) 13:308. doi: 10.1186/s12967-015-0642-6
83. He X, Dong Z, Cao Y, Wang H, Liu S, Liao L, et al. Msc-derived exosome promotes M2 polarization and enhances cutaneous wound healing. Stem Cells Int. (2019) 2019:7132708. doi: 10.1155/2019/7132708
84. Cao G, Chen B, Zhang X, Chen H. Human adipose-derived mesenchymal stem cells-derived exosomal microrna-19b promotes the healing of skin wounds through modulation of the Ccl1/Tgf-Β signaling axis. Clin Cosmet Investig Dermatol. (2020) 13:957–71. doi: 10.2147/ccid.s274370
85. Ayadi A E, Jay JW, Prasai A. Current approaches targeting the wound healing phases to attenuate fibrosis and scarring. Int J Mol Sci. (2020) 21(3):1–28. doi: 10.3390/ijms21031105
86. Yuan R, Dai X, Li Y, Li C, Liu L. Exosomes from Mir-29a-modified adipose-derived mesenchymal stem cells reduce excessive scar formation by inhibiting Tgf-Β2/Smad3 signaling. Mol Med Rep. (2021) 24(5):1–12. doi: 10.3892/mmr.2021.12398
87. Li Y, Zhang J, Shi J, Liu K, Wang X, Jia Y, et al. Correction to: exosomes derived from human adipose mesenchymal stem cells attenuate hypertrophic scar fibrosis by Mir-192-5p/Il-17ra/Smad axis. Stem Cell Res Ther. (2021) 12(1):490. doi: 10.1186/s13287-021-02568-3
88. Fang S, Xu C, Zhang Y, Xue C, Yang C, Bi H, et al. Umbilical cord-derived mesenchymal stem cell-derived exosomal micrornas suppress myofibroblast differentiation by inhibiting the transforming growth factor-Β/Smad2 pathway during wound healing. Stem Cells Transl Med. (2016) 5(10):1425–39. doi: 10.5966/sctm.2015-0367
89. Cooper DR, Wang C, Patel R, Trujillo A, Patel NA, Prather J, et al. Human adipose-derived stem cell conditioned media and exosomes containing Malat1 promote human dermal fibroblast migration and ischemic wound healing. Adv Wound Care. (2018) 7(9):299–308. doi: 10.1089/wound.2017.0775
90. He L, Zhu C, Jia J, Hao XY, Yu XY, Liu XY, et al. Adsc-Exos containing Malat1 promotes wound healing by targeting Mir-124 through activating Wnt/Β-catenin pathway. Biosci Rep. (2020) 40(5):1–13. doi: 10.1042/bsr20192549
91. Qian L, Pi L, Fang BR, Meng XX. Adipose mesenchymal stem cell-derived exosomes accelerate skin wound healing via the Lncrna H19/Mir-19b/Sox9 axis. Lab Invest. (2021) 101(9):1254–66. doi: 10.1038/s41374-021-00611-8
92. Li B, Luan S, Chen J, Zhou Y, Wang T, Li Z, et al. The Msc-derived exosomal Lncrna H19 promotes wound healing in diabetic foot ulcers by upregulating pten via microrna-152-3p. Mol Ther Nucleic. (2020) 19:814–26. doi: 10.1016/j.omtn.2019.11.034
93. Han ZF, Cao JH, Liu ZY, Yang Z, Qi RX, Xu HL. Exosomal Lncrna Klf3-As1 derived from bone marrow mesenchymal stem cells stimulates angiogenesis to promote diabetic cutaneous wound healing. Diabetes Res Clin Pract. (2022) 183:109126. doi: 10.1016/j.diabres.2021.109126
94. Patel RS, Impreso S, Lui A. Long noncoding Rna Gas5 contained in exosomes derived from human adipose stem cells promotes repair and modulates inflammation in a chronic dermal wound healing model. Biology. (2022) 11(3):1–19. doi: 10.3390/biology11030426
95. Born LJ, Chang KH, Shoureshi P, Lay F, Bengali S, Hsu ATW, et al. Hotair-loaded mesenchymal stem/stromal cell extracellular vesicles enhance angiogenesis and wound healing. Adv Healthcare Mater. (2022) 11(5):e2002070. doi: 10.1002/adhm.202002070
96. Shi R, Jin Y, Hu W, Lian W, Cao C, Han S, et al. Exosomes derived from Mmu_Circ_0000250-modified adipose-derived mesenchymal stem cells promote wound healing in diabetic mice by inducing Mir-128-3p/Sirt1-mediated autophagy. Am J Physiol Cell Physiol. (2020) 318(5):C848–C56. doi: 10.1152/ajpcell.00041.2020
97. Wang Z, Feng C, Liu H, Meng T, Huang W, Long X, et al. Hypoxic pretreatment of adipose-derived stem cells accelerates diabetic wound healing via Circ-Gcap14 and Hif-1α/Vegf mediated angiopoiesis. Int J Stem Cells. (2021) 14(4):447–54. doi: 10.15283/ijsc21050
98. Ju GQ, Cheng J, Zhong L, Wu S, Zou XY, Zhang GY, et al. Microvesicles derived from human umbilical cord mesenchymal stem cells facilitate tubular epithelial cell dedifferentiation and growth via hepatocyte growth factor induction. PLoS One. (2015) 10(3):e0121534. doi: 10.1371/journal.pone.0121534
99. Deregibus MC, Cantaluppi V, Calogero R, Lo Iacono M, Tetta C, Biancone L, et al. Endothelial progenitor cell derived microvesicles activate an angiogenic program in endothelial cells by a horizontal transfer of mrna. Blood. (2007) 110(7):2440–8. doi: 10.1182/blood-2007-03-078709
100. Liu J, Yan Z, Yang F, Huang Y, Yu Y, Zhou L, et al. Exosomes derived from human umbilical cord mesenchymal stem cells accelerate cutaneous wound healing by enhancing angiogenesis through delivering angiopoietin-2. Stem Cell Rev Rep. (2021) 17(2):305–17. doi: 10.1007/s12015-020-09992-7
101. Shabbir A, Cox A, Rodriguez-Menocal L, Salgado M, Van Badiavas E. Mesenchymal stem cell exosomes induce proliferation and migration of normal and chronic wound fibroblasts, and enhance angiogenesis in vitro. Stem Cells Dev. (2015) 24(14):1635–47. doi: 10.1089/scd.2014.0316
102. Han B, Xu S, Liu X, Shi J, Liu Z, Zhang Y, et al. Competing endogenous Rna network in non-keloid-prone individuals during wound healing. J Craniofac Surg. (2022) 33(1):29–34. doi: 10.1097/scs.0000000000007824
103. Huang M, He YR, Liang LC, Huang Q, Zhu ZQ. Circular Rna Hsa_Circ_0000745 may serve as a diagnostic marker for gastric cancer. World J Gastroenterol. (2017) 23(34):6330–8. doi: 10.3748/wjg.v23.i34.6330
104. Pichu S, Vimalraj S, Viswanathan V. Impact of microrna-210 on wound healing among the patients with diabetic foot ulcer. PLoS One. (2021) 16(7):e0254921. doi: 10.1371/journal.pone.0254921
105. Yu Y, Zhang W, Li A, Chen Y, Ou Q, He Z, et al. Association of long noncoding Rna biomarkers with clinical immune subtype and prediction of immunotherapy response in patients with cancer. JAMA Netw. Open. (2020) 3(4):e202149. doi: 10.1001/jamanetworkopen.2020.2149
106. Pi L, Yang L, Fang BR, Meng XX, Qian L. Exosomal microrna-125a-3p from human adipose-derived mesenchymal stem cells promotes angiogenesis of wound healing through inhibiting pten. Mol Cell Biochem. (2022) 477(1):115–27. doi: 10.1007/s11010-021-04251-w
107. Yu M, Liu W, Li J, Lu J, Lu H, Jia W, et al. Exosomes derived from atorvastatin-pretreated Msc accelerate diabetic wound repair by enhancing angiogenesis via Akt/Enos pathway. Stem Cell Res Ther. (2020) 11(1):350. doi: 10.1186/s13287-020-01824-2
108. Zhang L, Ouyang P, He G, Wang X, Song D, Yang Y, et al. Exosomes from microrna-126 overexpressing mesenchymal stem cells promote angiogenesis by targeting the Pik3r2-mediated Pi3k/Akt signalling pathway. J Cell Mol Med. (2021) 25(4):2148–62. doi: 10.1111/jcmm.16192
109. Wang W, Zhao Y, Li H, Zhang Y, Jia X, Wang C, et al. Exosomes secreted from mesenchymal stem cells mediate the regeneration of endothelial cells treated with rapamycin by delivering pro-angiogenic micrornas. Exp Cell Res. (2021) 399(1):112449. doi: 10.1016/j.yexcr.2020.112449
110. Liang X, Zhang L, Wang S, Han Q, Zhao RC. Exosomes secreted by mesenchymal stem cells promote endothelial cell angiogenesis by transferring Mir-125a. J Cell Sci. (2016) 129(11):2182–9. doi: 10.1242/jcs.170373
111. Yang C, Luo L, Bai X, Shen K, Liu K, Wang J, et al. Highly-expressed micorna-21 in adipose derived stem cell exosomes can enhance the migration and proliferation of the Hacat cells by increasing the Mmp-9 expression through the Pi3k/Akt pathway. Arch Biochem Biophys. (2020) 681:108259. doi: 10.1016/j.abb.2020.108259
112. Zheng T, Shao W, Tian J. Exosomes derived from adscs containing Mir-378 promotes wound healing by targeting caspase-3. J Biochem Mol Toxicol. (2021) 35(10):e22881. doi: 10.1002/jbt.22881
Keywords: wound healing, stem cell, exosome, microRNA, long noncoding RNA, circular RNA, messenger RNA
Citation: Chen G, Chen H, Zeng X and Zhu W (2022) Stem cell-derived exosomal transcriptomes for wound healing. Front. Surg. 9:933781. doi: 10.3389/fsurg.2022.933781
Received: 1 May 2022; Accepted: 1 August 2022;
Published: 12 August 2022.
Edited by:
Xie Julin, Sun Yat-sen University, ChinaReviewed by:
Rongbiao Pi, Sun Yat-sen University, China© 2022 Chen, Chen, Zeng and Zhu. This is an open-access article distributed under the terms of the Creative Commons Attribution License (CC BY). The use, distribution or reproduction in other forums is permitted, provided the original author(s) and the copyright owner(s) are credited and that the original publication in this journal is cited, in accordance with accepted academic practice. No use, distribution or reproduction is permitted which does not comply with these terms.
*Correspondence: Xiang Zeng emVuZ3hpYW5nQHN0ZW1jZWxsLXgub3Jn Wei Zhu emh1d2VpMDkyMUBnenVjbS5lZHUuY24=
Specialty Section: This article was submitted to Reconstructive and Plastic Surgery, a section of the journal Frontiers in Surgery
Disclaimer: All claims expressed in this article are solely those of the authors and do not necessarily represent those of their affiliated organizations, or those of the publisher, the editors and the reviewers. Any product that may be evaluated in this article or claim that may be made by its manufacturer is not guaranteed or endorsed by the publisher.
Research integrity at Frontiers
Learn more about the work of our research integrity team to safeguard the quality of each article we publish.