- Department of Anesthesia and Pain Medicine, Seoul St. Mary's Hospital, College of Medicine, The Catholic University of Korea, Seoul, Republic of Korea
While surgical resection is the gold standard treatment for solid tumors, cancer recurrence after surgery is common. Immunosurveillance of remnant tumor cells is an important protective mechanism. Therefore, maintenance of anti-tumor cell activity and proper levels of inflammatory mediators is crucial. An increasing body of evidence suggests that surgery itself and perioperative interventions could affect these pathophysiological responses. Various factors, such as the extent of tissue injury, perioperative medications such as anesthetics and analgesics, and perioperative management including transfusions and methods of mechanical ventilation, modulate the inflammatory response in lung cancer surgery. This narrative review summarizes the pathophysiological mechanisms involved in cancer recurrence after surgery and perioperative management related to cancer recurrence after lung cancer surgery.
Introduction
Lung cancer is the second most common type of cancer in the world and the leading cause of cancer-related deaths (1). Non-small-cell lung cancer (NSCLC) accounts for up to 85% of all lung cancers, and like most other solid cancers, is primarily treated with surgical resection for curative intent. Despite curative resection, 30%–55% of NSCLC patients experience recurrence and metastasis; the median survival time of patients with NSCLC recurrence is only about 21 months (2, 3). Even after complete surgical resection, microscopic tumor cells may remain, which increases the risk for local recurrence and distant metastasis through circulating tumor cells (CTCs) that disseminate in the bloodstream or the lymphatic system (4).
Surgery elicits a stress response that results in a catecholamine surge and activation of physiologic responses that promote wound healing and organ recovery. However, these physiologic responses, including the inflammatory response and angiogenesis, may contribute to cancer recurrence and metastasis (5). For example, perioperative inflammation and subsequent immunosuppression inhibit natural killer (NK) cell and T lymphocyte activity, which is critical for CTC detection and clearance (6). In this regard, evidence is accumulating that operative and anesthetic techniques may influence the effect of surgery on inflammatory response and cancer recurrence. This review summarizes the pathophysiological mechanisms involved in cancer recurrence after surgery and perioperative management related to cancer recurrence after lung cancer surgery.
Material and Methods
We extracted the most recent evidence from various databases, including PubMed, EMBASE, Web of Science, Google Scholar, and Cochrane Library databases. A literature search was performed using the following keywords: anesthesia, anesthetics, lung cancer, cancer recurrence, metastasis, and inflammation. All retrieved articles and relevant reviews were manually searched to find other potentially eligible studies. There was no restriction for the article type. Appropriateness for inclusion was determined by the authors to include a wide and unbiased range of relevant and recent studies.
Links Between Inflammatory Response and Cancer Progression
Inflammation and Cancer Recurrence
After surgical resection, tumor cells can disseminate in the peripheral blood as CTCs or propagate to the bone marrow or lymph nodes as disseminated tumor cells (DTCs) (5–7). The presence of CTCs does not entirely represent metastasis or recurrence. The host immune system generally detects and eliminates them. However, surgical stress can induce remnant cancer cells with complex involvements of sympathetic, inflammatory, and immune systems. In lung cancer surgery, CTC numbers are increased following surgery (8, 9), and are associated with cancer recurrence (10).
Cancer cells, inflammatory cells, immune cells, and stromal elements within the tissue interact with each other in a complex and dynamic way (5–7). This is called a “tumor microenviroment (TME)” that determines the potential for tumor metastasis. In normal conditions, the lack of extracellular matrix support, damage by shear stress, and immune surveillance hampers CTC survival and colonization. However, surgical intervention and tissue trauma easily disrupt TME and promote spread of residual cancer cells. Cancer recurrence occurs from remnant tumor cells at surgical sites in four stages (5, 6). First, remnant tumor cells acquire fibroblast-like properties such as motility, invasiveness, and exudation. This is called the “epithelial-mesenchymal transition”. Second, tumor cells invade the basement membrane, lymphatics, and blood vessels. Third, CTCs mitigate or survive in the circulatory system and metastasize to distant sites. Finally, single progenitor cells interact with stromal and inflammatory cells to proliferate. The inflammatory microenvironment plays a crucial role in the modulation of these steps. For example, NK and cytotoxic T (Tc) cell activity decrease after surgery, resulting in remnant tumor cells. Excessive secretion of growth factor and enzymes is harmful because they increase invasiveness and permeability of the tumor cells.
Surgery-induced stress response can promote tumor cell shedding by sympathetic activation, inflammatory imbalance, and immunosuppression (Figure 1).
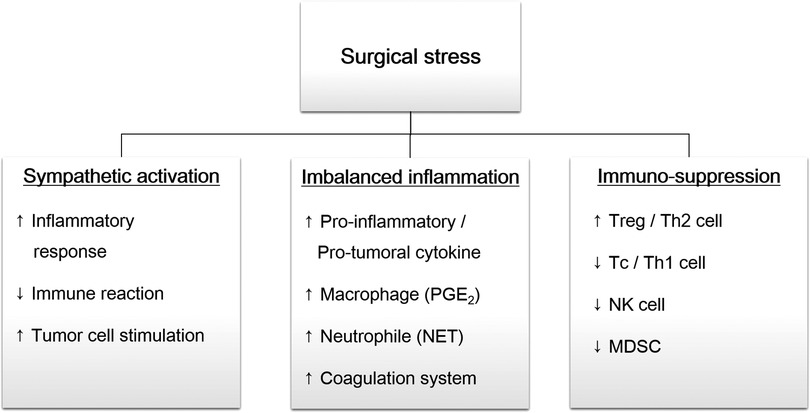
Figure 1. Overview of surgical stress response. Surgical trauma induce sympathetic activation, followed by imbalanced inflammation and immunosuppression. These changes promote remnant tumor cells survival and distant metastasis. PGE2, prostaglandin E2; NET, neutrophil extracellular traps; Treg, regulatory T cell, Th, helper T cell; Tc, cytotoxic T cell; NK cell, natural killer cell; MDSC, myeloid-derived suppressor cell.
Sympathetic Activation
Sympathetic activation triggers inflammatory response and promotes tumor cell growth. Tumor cell excision stimulates the hypothalamic-pituitary-adrenal axis and the sympathetic nervous system to release cortisol and catecholamines (11, 12). These factors increase pro-inflammatory cytokines (e.g., interleukin [IL]-6 and IL-8) and immunosuppressive cytokines (e.g., IL-4, IL-10, TGF- β, VEGF). Activated sympathetic system suppress NK and Tc cell activity, both of which are the main cells of immunosurveillance. They also stimulate helper T (Th)2 and regulatory T (Treg) cell proliferation. In addition, catecholamines directly act on tumor cells via the β-receptors. Activated β-receptors increase pro-inflammatory cytokines, vascular endothelial growth factor (VEGF), and matrix metalloprotease (MMP), which promotes tumor cell mobility and invasion (13).
Imbalanced Inflammatory Response
Initial tissue injury involves the release of humoral factors, resulting in the recruitment and activation neutrophils, macrophages, monocytes, and fibroblasts (5, 6, 14). Recruited inflammatory cells release more pro-inflammatory cytokines, including IL-1, IL-6, tumor necrosis factor (TNF)-α, and neutrophil extracellular traps (NET). These mediators increase the Th1/Th2 ratio and the secretion of interferon (IFN)-γ and IL-2 with anti-inflammatory and anti-tumoral effects.
Unfortunately, an imbalance between the pro- and anti- inflammatory responses can lead to the dysregulation of cellular immunity and subsequent immunosuppression. Macrophages and neutrophils, the main cells of the inflammatory response, continuously secrete IL-1, TNF-α, VEGF, and MMP, which contribute to tumor progression (15). Neutrophils can form a CTC-white blood cell cluster to expand the metastatic potential of CTCs, which promotes cell cycle progression (16). Extravasated neutrophil in the tissues after surgery has been shown to promote tumor capture and growth (17). Circulating neutrophile increase CTCs adhesion to the microvascular endothelium to induce epithelial-mesenchymal transition (18).
Excessive IL-6 stimulates macrophages to release prostaglandin E2 (PGE2). It increases the growth and motility of cancer cells and can induce angiogenic conversion in various cancers (19). In cases of lung cancer, PGE2 levels increase after surgery, which promotes metastasis by upregulation of MMP9 and downregulation of E-cadherin (20). PGE2 triggers an immunosuppressive state by increasing cancer-promoting Treg cells, decreasing activated Tc cells, and decreasing the Th1/Th2 ratio toward pro-inflammatory and pro-tumoral effects (21).
NETs released during the inflammatory response also promote cancer recurrence. NETs sequester CTCs and promote metastasis (22). Moreover, sequestered CTCs can trigger NET generation, resulting in tumor-host interaction (23). After surgical stress, NETs promote tumor cell proliferation, adhesion, migration, and invasion by inducing high-mobility group box (HMGB)-1 release, which interacts with platelets through toll-like receptor (TLR)-4 and activates TLR9-dependent pathways (24). In an animal study, NETs have a potential to activate dormant cancer cells following inflammation (25).
Recent trials have demonstrated that the complement system and fibrinogen also play a role in cancer recurrence. The complement proteins C7 and CFH are required for the maintenance of stemness in cancer cells (26). C5a anaphylatoxin promote angiogenesis in the tumor microenvironment (27), while complement system activation inhibits T-cell mediated anti-tumor immunity in lung cancer (28). Activated platelets and fibrin coat the CTCs and protect them from detection and removal by NK cells (29). In addition, platelet-fibrin complex mediate tumor cell adherence to endothelial cells and enhance vascular permeability, releasing mitogenentic and proangiogenetic factor.
Immunosuppression
The inflammatory response after surgery may promote systemic immunosuppression. Immunosuppressive microenvironments promote tumor progression and metastasis (30). The postoperative immunosuppression may last for about 2 weeks (31), and peaks at 3 days after surgery (32).
Each T cell population contributes differently to tumor cell survival (33, 34). Tc cells are involved in killing the tumor cells, and Th1 cells regulate the cytotoxic immunity to inhibit tumor progression. Meanwhile, Treg cells inhibit anti-tumor immune responses to create a pro-tumorigenic environment, and Th2 cells stimulate MMP expression, invasive potential, and metastasis. After surgery, Treg and Th2 cells are markedly increased, while Tc and Th1 cells are decreased, which contributes to tumor cell survival to varying degrees (31, 35, 36). In lung cancer surgery, an increase in Treg cells is observed in partial tumor resections, preventing the recruitment of Tc cells to the tumors and promoting recurrence rate (30).
NK cells, a type of cytotoxic lymphocyte, play an important role in tumor immunosurveillance as a major source of IFN-γ (37). Both NK cell toxicity and the secretion of IFN-γ by NK cells are profoundly suppressed perioperatively (38). A significant decline in IFN-γ has been observed after partial lung tumor resections (30). Intra-tumoral NK cell density is related to non-small cell lung cancer prognosis and recurrence (39).
Another mechanism leading to immunosuppression after surgery is the downregulation of chemokine (C-X-C motif) ligand 4 (CXCL4) and the recruitment of myeloid-derived suppressor cells (MDSC) (40). MDSCs trigger tumor progression by modulating the formation of premetastatic niches, and by inducing angiogenesis and tumor cell invasion (41). The number of MDSCs after surgery is related to cancer recurrence and prognosis (42). In lung cancer surgery, human MDSCs expressing CD11b(+), CD33(+), and HLA-DR(−) significantly increase after thoracotomy, and promote angiogenesis and tumor growth (43).
Postoperative Inflammatory Biomarkers as Prognostic Parameters
Objective evaluation of the inflammatory state after surgery may be useful for early detection of patients with a systemic inflammatory response (44). In addition, considering the association between inflammation and cancer recurrence, an evaluation of the inflammatory state after surgery may identify patients at risk for recurrence (45, 46). Predictive biomarkers commonly used to evaluate the inflammatory state after lung cancer surgery, including acute-phase proteins, complete blood count (CBC)-derived values and cytokines, are summarized in Table 1.
In response to inflammatory cytokines produced by local inflammatory cells, the liver produces acute-phase reactants, while the production of other proteins decreases (60). The most commonly used acute-phase proteins are C-reactive protein (CRP), fibrinogen, and albumin (Table 1). Postoperative serum CRP (47–51), fibrinogen (52), and albumin (53, 54) levels are associated with overall survival (OS) and disease-free survival (DFS). In a retrospective study of patients who underwent complete resections of pathological stage I and II NSCLCs, the Cox proportional hazard model revealed perioperative CRP grade as an independent poor prognostic factor for OS (grade 3 vs. grade 0 hazard ratio; HR: 5.05, 95% confidence interval; CI, 1.59, 19.60; p = 0.005), and DFS (HR: 3.62, 95% CI, 1.50, 9.33; p = 0.004) (47). On the other hand, a retrospective study of patients with resected NSCLC demonstrated that high serum CRP levels, measured on postoperative days 3, were associated with a favorable prognosis (HR: 0.36, 95%: CI, 0.20, 0.65; p < 0.001) (51). In a prospective study of patients with stage I–IIIA NSCLCs, serum plasma fibrinogen after surgery was an independent predictor for unfavorable DFS (HR: 3.77, 95% CI, 1.24, 9.87; p = 0.009) (52). A retrospective study of stage I NSCLC patients identified postoperative hypoalbuminemia as an independent negative prognostic factor for recurrence (HR: 0.221, 95% CI, 0.077, 0.634; p = 0.005) (53).
CBC analysis provides the crude numbers of neutrophils, granulocytes, lymphocytes, and platelets (45, 46). CBC-derived values, such as neutrophil-to-lymphocyte ratio (NLR) and platelet-to- lymphocyte ratio (PLR), have been widely studied (Table 1). A retrospective study of stage I NSCLC patients demonstrated that postoperative NLR was an independent predictor of DFS (HR: 2.435, 95% CI, 1.526, 4.322; p = 0.001) and OS (HR: 2.747, 95% CI, 1.668, 4.408; p = 0.001) (55). A retrospective study of patients with resectable lung cancer demonstrated that increased postoperative/preoperative PLR was independently associated with poor survival (HR: 1.890, 95% CI, 1.238, 2.887; p = 0.003) (56).
Cytokines are a broad category of cell signaling proteins that act as immunomodulators (61). IL-1, IL-8, IL-12, IL-18, TNF-α, IFN-γ, and granulocyte-macrophage colony-stimulating factors (GM-CSFs) are recognized as pro-inflammatory cytokines, whereas IL-4, IL-10, IL-13, IFN-α, and transforming growth factor (TGF)-β are anti-inflammatory cytokines. Interestingly, IL-6 exhibits both pro- and anti-inflammatory properties. Inflammatory cytokines related to lung cancer include IL-1β, IL-4, IL-6, IL-11, IL-12, TNF-α, monocyte chemotactic protein (MCP)-1, and TGF-β (62). Studies that have investigated cytokines as prognostic markers for OS and DFS after cancer surgery are scarce because measuring cytokines requires a complex procedure such as flow cytometry, which is expensive and not routinely performed (46). Postoperative IL-6, IL-4, and IFN-γ changes have been suggested as prognostic markers in lung cancer surgery (Table 1). In a prospective study of patients who underwent curative pulmonary resections for NSCLCs, serum IL-6 levels on postoperative day 1 were significant independent predictors of early postoperative recurrences (Odds ratio; OR: 1.008, 95% CI, 1.003, 1.013; p = 0.003) (57). A prospective study of patients who underwent radical surgery for NSCLC demonstrated that patients with postoperative IL-4 abnormalities had significantly greater one- and three-year cumulative relapse frequencies compared to patients with normal IL-4 levels (1-year: 40.00% vs. 15.15%; 3-year: 72.00% vs. 33.33%; p = 0.001) (58).
In summary, various inflammatory biomarkers have been investigated, but most have been retrospective, and more evidence is needed to identify simple and cost-effective inflammatory biomarkers that can link inflammatory responses after lung cancer surgery to cancer recurrence.
Perioperative Modulation of Inflammatory Response and Oncologic Outcome
Several perioperative factors influence the inflammatory response after surgery. Because the inflammatory status of the patient is critical for cancer recurrence, it is important for surgeons and anesthesiologists to understand these factors and apply them to perioperative management. Various perioperative management processes that modulate the inflammatory response have been studied in clinical trials, and have shown advantages in postoperative complications, but few have translated into oncological outcomes. The following section focuses on perioperative strategies that are beneficial or harmful in terms of oncological outcomes (Table 2).
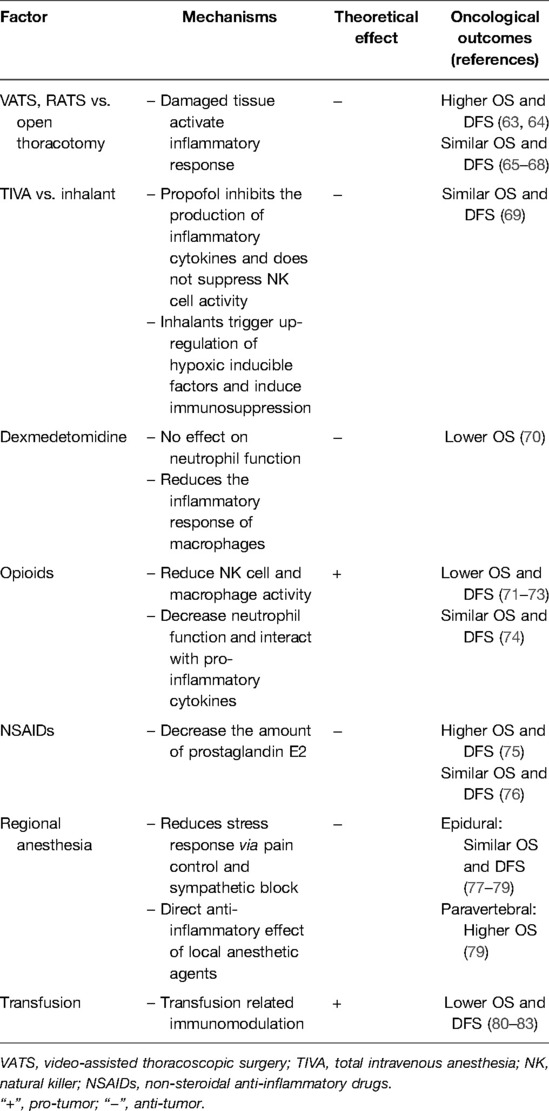
Table 2. Perioperative factors that influence the inflammatory response after surgery and their impact on oncological outcomes.
Extent of Tissue Injury
The extent of tissue injury is an important factor in the inflammatory response after surgery. Tissue and organ damage that occurs during surgical manipulation results in the release of inflammatory mediators (44). For example, macrophages in the damaged skin release chemokines such as keratinocyte chemoattractant and macrophage inflammatory protein-2, causing neutrophil infiltration. Adipocytes can secrete TNF-α, suggesting that adipose tissue damage can lead to the release of inflammatory mediators.
Clinical trials have shown that minimally invasive video-assisted thoracoscopic surgery (VATS) attenuates the inflammatory response and maintains immune cell function compared to open thoracotomy. In studies that compared VATS and thoracotomy in patients undergoing lobectomy, lower serum IL-6 and CRP levels were reported in patients who underwent VATS (84–86). A study that evaluated IL-6 levels in the pleural fluid following lobectomy also demonstrated that the increase in pleural IL-6 levels 3 h after surgery was significantly lower after VATS compared to open lobectomy (87). Similar results were reported by a study of bronchoalveolar lavage (BAL). In a study that evaluated cytokines from BAL in the contralateral lung, IL-6, IL-8 and IL-10 levels were lower in VATS patients (88). It has also been reported that VATS results in a lesser decrease in circulating T and NK cells, lesser suppression of lymphocyte oxidation, and decreased phagocyte reaction oxygen species (ROS) generation compared to open thoracotomy (85, 89–91).
VATS shows either superior or non-inferior oncological outcomes compared to conventional open thoracotomy. A retrospective study of stage IA NSCLC patients who underwent lobectomy demonstrated that VATS was associated with increased 5-year OS compared to open thoracotomy (100% vs. 87%, p = 0.01) and DFS (100% vs. 86%, p = 0.03) (63). In tumors greater than 5 cm, VATS was associated with greater OS and DFS (p = 0.056 and 0.031, respectively) compared to open thoracotomy (64), and in clinical N2 lung cancer, VATS showed similar 5-year OS (50.5% vs. 48.4%, p = 0.127) and DFS (60.5% vs. 44.6%, p = 0.069) (65). A study of oncological outcomes in patients who underwent surgical resection for early-stage lung cancer found no differences in 5-year OS (71.6% vs. 65.9%, p = 0.36) and DFS (75.2% vs. 69.2%, p = 0.55) between VATS and open lobectomy (66). In two National Cancer Database analyses, VATS was not found to be inferior to the open approach in terms of 5-year OS in stage I (66.3% vs. 65.8%, p = 0.92) and II (49.0% vs. 51.2%, p value not provided) NSCLC patients (67, 68). Some controversy exists because the restricted instrument handling in VATS can make complete oncologic resection difficult, despite the benefits of VATS, such as less tissue injury. Previous studies have mainly focused on comparing different surgical methods without considering the impact of perioperative management. Therefore, single-center studies with relatively consistent perioperative management might show superior oncological outcomes, while multi-center studies with various perioperative management might show noninferior oncological outcomes.
Perioperative Medicine
Anesthesia Techniques
Anesthetic Agents
Inhaled anesthetics may promote growth of residual cancer cells by two mechanisms: upregulation of hypoxia-inducible factor (HIF) and immunosuppression. Inhaled provide organ protection in different models of organ damage, particularly in ischemia-reperfusion injury. Their protective properties are due to HIF-1α upregulation, which has been linked to more aggressive cancer phenotypes and poorer clinical prognosis (92). It has been proposed that the cytoprotective properties of HIFs in organs may also provide protection to residual cancer cells. In an in vitro study, isoflurane upregulated HIF-1α and HIF-2α levels and intensified VEGF expression (93). Immunosuppressive properties of inhaled anesthetics have been demonstrated in in vitro studies, where isoflurane and sevoflurane inhibited T lymphocytes (94), and induced apoptosis of T and B lymphocytes (95). In a study of the human NSCLC cell-line, sevoflurane suppressed NK cell cytotoxicity and increased immunosurveillance mediators (96).
Propofol, the most commonly used intravenous anesthetic agent, has anti-inflammatory effects, which may protect against perioperative immunosuppression. In lipopolysaccharide (LPS)-activated macrophages, exposure to a therapeutic concentration of propofol significantly reduces the levels of LPS-enhanced IL-1β, IL-6 and TNF-α proteins (97). Propofol can reduce inflammatory responses in LPS-induced alveolar epithelial type-III cell injury through downregulation of CD-14 and TLR-14 expressions (98, 99). In a mouse model with d-galactosamine/LPS induced acute liver injury, propofol inhibited the production of inflammatory cytokines and oxidative stress-related factors. In a study of the effects of anesthetics on NK cell activity and metastasis in a breast cancer mouse model, propofol was reported as the only agent that did not suppress NK cell activity or increase metastasis (100). Other intravenous agents such as thiopental, etomidate and ketamine, also have anti-inflammatory properties (101), and thiopental and ketamine were associated with suppressed NK cell activity (100).
Comparison of anesthetic agents have focused on the two most commonly used anesthetic agents; inhalants and propofol. Inhalants seem to attenuate the local pulmonary inflammatory response more than propofol, while propofol provides greater protection against the systemic inflammatory response. A meta-analysis of 8 studies involving 488 patients undergoing lung resection with one-lung ventilation (OLV), found no significant differences in the concentrations of systemic IL-6, IL-10 and TNF-α between sevoflurane and propofol (102). However, in the same meta-analysis, IL-6 levels in the BAL fluid in both the dependent and independent lung were decreased with sevoflurane compared to propofol (102). A prospective study reported decreased number of T lymphocytes and NK cells after surgery in NSCLC patients, but the decrease was lesser in patients who received combined sevoflurane-epidural anesthesia compared to those who received total intravenous anesthesia (103). Whether these effects on cellular immunity were due to differences between sevoflurane and propofol, or due to epidural anesthesia is unknown. Despite the different effects on cellular immunity, the 3-year DFS was similar between the two groups. Similarly, another prospective study demonstrated that postoperative serum VEGF and TGF-β levels were significantly lower in patients who received propofol and paravertebral blocks than those who received sevoflurane (104). Although inhalants and propofol seem to affect the inflammatory and immune responses differently, this has not translated into oncological outcomes. In a retrospective study of NSCLC patients who underwent curative resections, there were no significant differences in the hazard ratios for recurrence (HR: 1.310, 95% CI, 0.841, 2.041; p = 0.233) and death (HR: 0.902, 95% CI, 0.643, 1.265; p = 0.551) between sevoflurane and propofol (69).
Local Anesthetic Agents and Regional Anesthesia
Local anesthetic agents have beneficial effects on the inflammatory response and cancer recurrence by attenuation of the stress response and immunosuppression while reducing perioperative doses of opioids and other anesthetic agents (44). Local anesthetic agents are capable of inhibiting adhesion, chemotaxis, phagocytosis, and the production of superoxide anion and hydrogen peroxide by neutrophils and macrophages (105). Studies have shown that lidocaine can enhance NK cell activity (106). An in vitro study that used clinically relevant concentrations of lidocaine and ropivacaine demonstrated that local anesthetic agents inhibit TNF-α-induced invasion of lung adenocarcinoma by blocking the activation of Akt and focal adhesion kinase (107). Another in vitro study that used lidocaine, ropivacaine, and chloroprocaine demonstrated that amide-linked local anesthetic agents inhibited TNF-α-induced Src-activation and ICAM-1 phosphorylation, which are important in the migration of lung adenocarcinoma cells (108). In a study of pigs undergoing lung resection surgery, continuous intravenous lidocaine infusion resulted in decreased TNF-α levels in BAL, plasma, and lung samples (109). In a study of NSCLC patients undergoing VATS, patients who received intravenous lidocaine had lower serum IL-17 and cortisol compared to patients without lidocaine administration (110). Although intravenous administration of local anesthetic agents has been shown to attenuate the inflammatory response, this effect has not translated into oncological outcomes. However, the effects of local anesthetic agents through regional anesthesia have been extensively studied.
Regional anesthesia with thoracic epidural, thoracic paravertebral, intercostal nerve, or fascial plane blocks is considered an essential component of pain management in lung cancer surgery (111). It can reduce the inflammatory response to surgery and thus, cancer recurrence through several mechanisms. Regional anesthesia attenuates the stress response to surgery via pain control or sympathetic blocks, reduces the need for anesthetic agents, and exhibits direct effects by absorption of local anesthetic agents, as described above (112, 113). In a prospective study, patients who underwent lung cancer surgery with thoracic epidural anesthesia had significantly lower IL-6 levels in serum and lung epithelial lining fluid (114). Another prospective study of patients undergoing radical resections for lung cancer demonstrated that T lymphocytes levels were better preserved in patients who received intravenous anesthesia with epidural anesthesia compared to those who received only intravenous anesthesia (115). However, these effects did not correlate with oncological outcomes. A prospective study in patients who underwent VATS lung cancer resection demonstrated that epidural anesthesia-analgesia did not improve OS (HR: 1.12, 95% CI, 0.64, 1.96; p = 0.697) and DFS (HR: 0.90, 95% CI, 0.60, 1.35; p = 0.608) compared to general anesthesia (77). A retrospective study that compared epidural and intravenous analgesia in stage I NSCLC patients undergoing lung resections found no statistically significant differences in five-year OS (HR: 0.91, 95% CI, 0.58, 1.41; p = 0.663) and DFS (HR: 1.11, 95% CI, 0.12, 10.11; p = 0.925) (78). Another retrospective study that compared epidural, paravertebral, and intravenous patient-controlled analgesia (PCA) in patients undergoing open thoracotomy for curative resections of primary lung cancer demonstrated that pain-control methods were not related to cancer recurrence, but that paravertebral PCA may have a beneficial effect on OS (HR against epidural: 0.58, 95% CI, 0.39, 0.87; HR against PCA: 0.60, 95% CI, 0.45, 0.79; p = 0.002) (79).
Non-Intubated Thoracic Anesthesia
Mechanical ventilation is commonly used with general anesthesia in many surgeries, and OLV is used in almost all lung cancer surgeries to facilitate surgical exposure. However, mechanical ventilation and OLV are associated with a profound systemic and local inflammatory response in both ventilated and collapsed lungs (116).
Non-intubated (NI) thoracic anesthesia is a novel technique where acute lung injury and accompanying inflammatory response can be attenuated by avoiding of general anesthesia and mechanical ventilation. Conventional thoracic anesthesia is performed under general anesthesia with mechanical ventilation and OLV, whereas NI thoracic anesthesia is performed under sedation and regional anesthesia with spontaneous ventilation (117). NIVATS is a safe and feasible technique for lung cancer resection surgery (118–120). In a study of patients who underwent VATS, NIVATS resulted in an attenuated stress response compared to conventional intubated VATS (121). A study that compared NIVATS with epidural anesthesia demonstrated that NIVATS resulted in a lesser decrease in postoperative serum NK cells and total lymphocyte count compared to general anesthesia with OLV (122). In a study of patients undergoing VATS metastasectomy, NIVATS was associated with a lesser reduction of serum NK cells at 7 days after the procedure, and lesser spillage of IL-6 at 1, 7, and 14 days compared to intubated VATS (123). In a study of stage I NSCLC patients undergoing surgical resections, postoperative serum IL-6, TNF-α, and IL-6/IL-10 ratio were significantly lower in NIVATS patients compared to intubated patients (117). Although NI thoracic anesthesia has been shown to attenuate the inflammatory response, there have been no clinical studies to investigate the oncological outcomes in lung cancer surgery.
Opioids
Opioids are generally considered as immunosuppressive and reduce NK cell cytotoxicity and macrophage and neutrophil phagocytosis (124). They also decrease the neutrophil production of ROS, impair neutrophil chemotaxis, and decrease cytokine production (125). Interestingly, opioids can interact with inflammatory cytokines such as IL-1, IL-4, IL-6 and TNF-α, which regulate gene expression at the mu-opioid receptor to cause immunosuppression (126). In a retrospective study of stage I–III lung adenocarcinoma patients, higher intraoperative morphine administration was associated with worse OS (HR: 1.09, 95% CI, 1.02, 1.17; p = 0.010), whereas ketamine was associated with improved DFS (HR: 0.44, 95% CI, 0.24, 0.80; p = 0.007) (71). This was also demonstrated in a retrospective study of NSCLC patients undergoing VATS lobectomy, where increased doses of opioids during the initial 96 h postoperatively was associated with a higher 5-year recurrence rate (OR: 1.003, 95% CI, 1.000, 1.006; p = 0.04) (72). A retrospective study of NSCLC patients undergoing surgery reported that opioids were a risk factor for OS in stage I patients (HR: 1.15, 95% CI, 1.01, 1.32; p = 0.036), but not for stage II (HR: 0.94, 95% CI, 0.76, 1.16, p = 0.586) and stage III patients (HR: 0.98, 95% CI, 0.83, 1.15, p = 0.862) (73). However, another retrospective study of NSCLC patients undergoing curative resection reported that the amount of opioid usage did not affect the risk for recurrence (p = 0.521) and death (p = 0.660) (74).
Adjuvant Agents
Dexmedetomidine is known for its anti-inflammatory properties. It has no effects on neutrophil chemotaxis, phagocytosis, or superoxide production at clinically relevant doses, and it reduces the inflammatory response of macrophages (127). It can reduce the extent of lung injury by inhibiting IL-6 and TNF-α expression in lung tissues (128). A study of lung cancer patients undergoing radical resections demonstrated that dexmedetomidine reduced the inflammatory response and oxidative stress response, evidenced by lower IL-6, IL-8, and malondialdehyde levels, and higher superoxide dismutase levels compared to controls (129). In a prospective study of patients undergoing thoracoscopic surgery, intraoperative dexmedetomidine administration reduced serum HMGB-1, monocyte chemoattractant protein 1, neutrophil elastase, and IL-6 levels compared to saline infusion (130). On the other hand, dexmedetomidine has been shown to promote tumor metastases by inducing myeloid-derived suppressor cells that have immunosuppressive and pro-angiogenic properties (131). Consequently, the anti-inflammatory properties of dexmedetomidine failed to show benefits in cancer recurrence. An animal study demonstrated that dexmedetomidine increases tumor-cell retention and growth of metastases in breast, colon, and lung cancers (132). In a study of stage I–IIIa NSCLC patients undergoing surgery, intraoperative dexmedetomidine administration had no significant impact on DFS (HR: 1.18, 95% CI, 0.91, 1.53; p = 0.199), but was associated with worse OS (HR: 1.28, 95% CI, 1.03, 1.59; p = 0.024) (70).
Nonsteroidal anti-inflammatory drugs (NSAIDs) are commonly used perioperatively for their analgesic and opioid-sparing properties (101). They have well-recognized anti-inflammatory and anti-thrombotic properties. NSAIDs inhibit COX-1 and COX-2 expression, which decreases the amount of available PGE2. PGE2 upregulates the immunosuppressive IL-10, downregulates the antiangiogenic IL-12, and has a role in tumor invasion, apoptosis resistance, and dendritic cell differentiation and migration (133, 134). COX-2 can also trigger various cellular inhabitants favoring the tumor microenvironment, such as IL-1b, TGF-β, and VEGF (134). Not only do NSAIDs inhibit COX enzymes, but their opioid-sparing and anti-thrombotic properties provide a defense against cancer recurrence (135). In a retrospective study of NSCLC patients undergoing surgery, postoperative NSAID administration was related to longer OS (HR: 0.528, 95% CI, 0.278, 0.884; p = 0.006) and DFS (HR: 0.557, 95% CI, 0.317, 0.841; p = 002) (75). Meanwhile, another retrospective study of NSCLC patients undergoing surgical resections reported that postoperative NSAID administration was not an independent predictor of OS (p = 0.18) and DFS (p = 0.66) (76).
Transfusion
Transfusion of blood products may result in a transient depression of the immune system referred to as transfusion-related immunomodulation (TRIM) (33). TRIM may develop due to suppression of cytotoxic cell and monocyte activity, release of immunosuppressive prostaglandins, inhibition of IL-2 production, and increase in suppressor T-cell activity. Although the leukocyte reduction technique has been used to eliminate white cells implicated in TRIM, a few remaining leukocytes may still modulate the immune response in the recipient.
The concentration of cytokines is increased in stored packed red blood cell (PRC) units (136). PRC units contain pro-inflammatory lysophosphatidylcholines (lyso-PCs), which modulate NK and T cell activity, and induce pro-inflammatory cytokine production in macrophages (137). Ecosanoids, such as prostaglandins and thromboxane, can also accumulate in PRCs (138). These mechanisms all contribute to the immunomodulatory effects of PRCs, leading to a pro-inflammatory and immunosuppressive state.
Many clinical studies have investigated the role of blood transfusions in lung cancer recurrence. In a retrospective study of stage I–III NSCLC patients who were transfused for hemoglobin levels < 8.0 g/dL within 7 days after surgical resection, patients who received transfusions were at greater risk for early recurrence (HR: 1.81, 95% CI, 1.59, 2.06; p < 0.001) and all-cause mortality (HR: 2.38, 95% CI, 1.97, 2.87; p < 0.001) (80). A meta-analysis of 23 studies with 6473 patients showed that allogeneic blood transfusions were significantly associated with earlier recurrence and worse OS in patients with surgically resected lung cancers (81). In another meta-analysis of 18 studies with 5915 patients, perioperative blood transfusion was associated with worse OS (HR: 1.42, 95% CI, 1.20, 1.69; p < 0.001) and DFS (HR: 1.49, 95% CI, 1.29, 1.65; p < 0.001) in patients with resected lung cancers (82). A retrospective study of NSCLC patients who underwent pulmonary resections demonstrated that, although a single-unit blood transfusion did not affect survival, greater units of blood transfusions were associated with significantly decreased OS (2 units HR: 1.55, 95% CI, 1.262, 1.91; p < 0.001; 3–7 units HR: 2.02, 95% CI, 1.61, 2.53; p < 0.001; and ≥8 units HR: 4.29, 95% CI, 2.91, 6.33; p < 0.001) and DFS (2 units HR: 1.44, 95% CI, 1.19, 1.76; p < 0.001; 3–7 units HR: 1.85, 95% CI, 1.49, 2.30; p < 0.001; and ≥8 units HR: 3.57, 95% CI, 2.45, 5.21; p < 0.001) in a dose-dependent manner (83).
Conclusions
The inflammatory response during cancer resection surgery is closely linked to postoperative oncological outcomes. Many factors, such as tissue injury, perioperative medications, and perioperative management (transfusion, methods of mechanical ventilation, and so forth), modulate the inflammatory response in lung cancer surgery. However, only a few high-quality clinical trials have investigated the impact of perioperative strategies on lung cancer recurrence compared to other types of cancers. Most published studies are retrospective, or prospective but designed for outcomes other than cancer recurrence. Fortunately, high-quality randomized trials are recently starting to get published (77, 139), and are in progress; “Volatile Anaesthesia and Perioperative Outcomes Related to Cancer: The VAPOR-C Trial” (NCT04316013) is evaluating propofol versus sevoflurane in colorectal or lung cancer patients (140), “General Anesthetics in CAncer REsection Surgery (GA-CARES) Trial” (NCT03034096) is evaluating propofol versus volatile agents in various types of cancer including lung cancer, and “The Effect of Combined General/Regional Anesthesia on Cancer Recurrence in Patients Having Lung Cancer Resections” (NCT02840227) is in progress.
Although results on the inflammatory response and other postoperative outcomes seem promising, current evidence does not support a change in anesthetic practice, or the use of specific agents or techniques for the purpose of reducing the risk of cancer recurrence in lung cancer surgery. Considering the enormous impact of lung cancer in the field of medicine, understanding the mechanisms of inflammation and cancer recurrence, and influencing the perioperative factors is of paramount importance. Certain anesthetic and adjuvant agents, regional anesthesia, transfusions, and NI thoracic anesthesia appear promising. Mechanical ventilation and OLV result in profound systemic and local inflammatory response in both ventilated and collapsed lung (116). Lung-protective ventilation may be a useful strategy to mitigate acute lung injury (111). As demonstrated by a study of patients undergoing open thoracic surgery, where reduction of tidal volume during OLV reduced alveolar concentrations of TNF-α and ICAM-1 (141), lung-protective ventilation might have a role in preventing cancer recurrence after lung cancer surgery. The effect of neoadjuvant therapy on perioperative inflammation during lung cancer surgery also deserves attention. A preoperative inflammatory state is associated with OS and DFS in patients undergoing neoadjuvant chemoradiotherapy followed by surgical resection (142). Preoperative chemotherapy in lung cancer patients can exacerbate the perioperative overproduction of inflammatory cytokines (143). More recently, neoadjuvant immunotherapy, using immune checkpoint inhibitors, is gaining attention for the treatment of advanced NSCLC (144). However, there is lack of data on the effects of neoadjuvant therapy on perioperative inflammation, and the association between the perioperative inflammatory state after neoadjuvant therapy and oncological outcomes. Well-designed prospective studies are needed to determine whether these perioperative management processes could contribute to better oncological outcomes. Meanwhile, the recently published guidelines for an enhanced recovery program after thoracic surgery include components that reduce surgical stress and the resultant inflammatory response (111).
Author Contributions
Conceptualization, Structure, Outline, Supervision - W.H. Literature review - C.H., W.H. Current evidence synthesis - C.H. Writing – original draft - C.H. Writing – reviewing and editing -W.H. All authors contributed to the article and approved the submitted version.
Funding
This work was supported by the National Research Foundation of Korea (NRF) grant funded by the Korea government (MSIT; Ministry of Science and ICT) (No. 2021R1G1A1014702).
Conflict of Interest
The authors declare that the research was conducted in the absence of any commercial or financial relationships that could be construed as a potential conflict of interest.
Publisher's Note
All claims expressed in this article are solely those of the authors and do not necessarily represent those of their affiliated organizations, or those of the publisher, the editors and the reviewers. Any product that may be evaluated in this article, or claim that may be made by its manufacturer, is not guaranteed or endorsed by the publisher.
References
1. World Health Organization International Agency for Research on Cancer. Globocan: Estimated Age-Standardized Incidence and Mortality Rates (World) in 2020, Worldwide, Both Sexes, All Ages [cited 2022 January 13]. Available from: http://gco.iarc.fr/today/fact-sheets-cancers
2. Uramoto H, Tanaka F. Recurrence after surgery in patients with nsclc. Transl Lung Cancer Res. (2014) 3(4):242–9. doi: 10.3978/j.issn.2218-6751.2013.12.05
3. Sasaki H, Suzuki A, Tatematsu T, Shitara M, Hikosaka Y, Okuda K, et al. Prognosis of recurrent non-small cell lung cancer following complete resection. Oncol Lett. (2014) 7(4):1300–4. doi: 10.3892/ol.2014.1861
4. Popper HH. Progression and metastasis of lung cancer. Cancer Metastasis Rev. (2016) 35(1):75–91. doi: 10.1007/s10555-016-9618-0
5. Kinoshita T, Goto T. Links between inflammation and postoperative cancer recurrence. J Clin Med. (2021) 10(2):228. doi: 10.3390/jcm10020228
6. Onuma AE, Zhang H, Gil L, Huang H, Tsung A. Surgical stress promotes tumor progression: a focus on the impact of the immune response. J Clin Med. (2020) 9(12):4096. doi: 10.3390/jcm9124096
7. Chen Z, Zhang P, Xu Y, Yan J, Liu Z, Lau WB, et al. Surgical stress and cancer progression: the twisted Tango. Mol Cancer. (2019) 18(1):132. doi: 10.1186/s12943-019-1058-3
8. Matsutani N, Sawabata N, Yamaguchi M, Woo T, Kudo Y, Kawase A, et al. Does lung cancer surgery cause circulating tumor cells? A multicenter, prospective study. J Thorac Dis. (2017) 9(8):2419–26. doi: 10.21037/jtd.2017.07.33
9. Duan X, Zhu Y, Cui Y, Yang Z, Zhou S, Han Y, et al. Circulating tumor cells in the pulmonary vein increase significantly after lobectomy: a prospective observational study. Thorac Cancer. (2019) 10(2):163–9. doi: 10.1111/1759-7714.12925
10. Sawabata N, Nakamura T, Kawaguchi T, Watanabe T, Ouji NS, Ito T, et al. Circulating tumor cells detected only after surgery for non-small cell lung cancer: is it a predictor of recurrence? J Thorac Dis. (2020) 12(9):4623–32. doi: 10.21037/jtd-20-1636
11. Hiller JG, Perry NJ, Poulogiannis G, Riedel B, Sloan EK. Perioperative events influence cancer recurrence risk after surgery. Nat Rev Clin Oncol. (2018) 15(4):205–18. doi: 10.1038/nrclinonc.2017.194
12. Neeman E, Ben-Eliyahu S. Surgery and stress promote cancer metastasis: new outlooks on perioperative mediating mechanisms and immune involvement. Brain Behav Immun. (2013) 30(Suppl):S32–40. doi: 10.1016/j.bbi.2012.03.006
13. Zhang H, Kong Q, Wang J, Jiang Y, Hua H. Complex roles of camp-pka-creb signaling in cancer. Exp Hematol Oncol. (2020) 9(1):32. doi: 10.1186/s40164-020-00191-1
14. Wall T, Sherwin A, Ma D, Buggy DJ. Influence of perioperative anaesthetic and analgesic interventions on oncological outcomes: a narrative review. Br J Anaesth. (2019) 123(2):135–50. doi: 10.1016/j.bja.2019.04.062
15. Murdoch C, Muthana M, Coffelt SB, Lewis CE. The role of myeloid cells in the promotion of tumour angiogenesis. Nat Rev Cancer. (2008) 8(8):618–31. doi: 10.1038/nrc2444
16. Szczerba BM, Castro-Giner F, Vetter M, Krol I, Gkountela S, Landin J, et al. Neutrophils escort circulating tumour cells to enable cell cycle progression. Nature. (2019) 566(7745):553–7. doi: 10.1038/s41586-019-0915-y
17. Tohme S, Simmons RL, Tsung A. Surgery for cancer: a trigger for metastases. Cancer Res. (2017) 77(7):1548–52. doi: 10.1158/0008-5472.Can-16-1536
18. Ten Kate M, Aalbers AG, Sluiter W, Hofland LJ, Sonneveld P, Jeekel J, et al. Polymorphonuclear leukocytes increase the adhesion of circulating tumor cells to microvascular endothelium. Anticancer Res. (2007) 27(1a):17–22.
19. Chang SH, Liu CH, Conway R, Han DK, Nithipatikom K, Trifan OC, et al. Role of prostaglandin E2-dependent angiogenic switch in cyclooxygenase 2-induced breast cancer progression. Proc Natl Acad Sci USA. (2004) 101(2):591–6. doi: 10.1073/pnas.2535911100
20. Zhang S, Da L, Yang X, Feng D, Yin R, Li M, et al. Celecoxib potentially inhibits metastasis of lung cancer promoted by surgery in mice, via suppression of the Pge2-modulated Β-catenin pathway. Toxicol Lett. (2014) 225(2):201–7. doi: 10.1016/j.toxlet.2013.12.014
21. Wang D, Dubois RN. Eicosanoids and cancer. Nat Rev Cancer. (2010) 10(3):181–93. doi: 10.1038/nrc2809
22. Cools-Lartigue J, Spicer J, McDonald B, Gowing S, Chow S, Giannias B, et al. Neutrophil extracellular traps sequester circulating tumor cells and promote metastasis. J Clin Invest. (2013) 123(8):3446–58. doi: 10.1172/jci67484
23. Park J, Wysocki RW, Amoozgar Z, Maiorino L, Fein MR, Jorns J, et al. Cancer cells induce metastasis-supporting neutrophil extracellular DNA traps. Sci Transl Med. (2016) 8(361):361ra138. doi: 10.1126/scitranslmed.aag1711
24. Yu LX, Yan L, Yang W, Wu FQ, Ling Y, Chen SZ, et al. Platelets promote tumour metastasis via interaction between Tlr4 and tumour cell-released high-mobility group box1 protein. Nat Commun. (2014) 5:5256. doi: 10.1038/ncomms6256
25. Albrengues J, Shields MA, Ng D, Park CG, Ambrico A, Poindexter ME, et al. Neutrophil extracellular traps produced during inflammation awaken dormant cancer cells in mice. Science. (2018) 361(6409):eaao4227. doi: 10.1126/science.aao4227
26. Seol HS, Lee SE, Song JS, Rhee JK, Singh SR, Chang S, et al. Complement proteins C7 and cfh control the stemness of liver cancer cells via lsf-1. Cancer Lett. (2016) 372(1):24–35. doi: 10.1016/j.canlet.2015.12.005
27. Corrales L, Ajona D, Rafail S, Lasarte JJ, Riezu-Boj JI, Lambris JD, et al. Anaphylatoxin C5a creates a favorable microenvironment for lung cancer progression. J Immunol. (2012) 189(9):4674–83. doi: 10.4049/jimmunol.1201654
28. Kwak JW, Laskowski J, Li HY, McSharry MV, Sippel TR, Bullock BL, et al. Complement activation via a C3a receptor pathway alters Cd4(+) T lymphocytes and mediates lung cancer progression. Cancer Res. (2018) 78(1):143–56. doi: 10.1158/0008-5472.Can-17-0240
29. Seth R, Tai LH, Falls T, de Souza CT, Bell JC, Carrier M, et al. Surgical stress promotes the development of cancer metastases by a coagulation-dependent mechanism involving natural killer cells in a murine model. Ann Surg. (2013) 258(1):158–68. doi: 10.1097/SLA.0b013e31826fcbdb
30. Predina J, Eruslanov E, Judy B, Kapoor V, Cheng G, Wang LC, et al. Changes in the local tumor microenvironment in recurrent cancers may explain the failure of vaccines after surgery. Proc Natl Acad Sci USA. (2013) 110(5):E415–24. doi: 10.1073/pnas.1211850110
31. Shakhar G, Ben-Eliyahu S. Potential prophylactic measures against postoperative immunosuppression: could they reduce recurrence rates in oncological patients? Ann Surg Oncol. (2003) 10(8):972–92. doi: 10.1245/aso.2003.02.007
32. Coffey JC, Wang JH, Smith MJ, Bouchier-Hayes D, Cotter TG, Redmond HP. Excisional surgery for cancer cure: therapy at a cost. Lancet Oncol. (2003) 4(12):760–8. doi: 10.1016/s1470-2045(03)01282-8
33. Cata JP, Wang H, Gottumukkala V, Reuben J, Sessler DI. Inflammatory response, immunosuppression, and cancer recurrence after perioperative blood transfusions. Br J Anaesth. (2013) 110(5):690–701. doi: 10.1093/bja/aet068
34. Dunn GP, Old LJ, Schreiber RD. The immunobiology of cancer immunosurveillance and immunoediting. Immunity. (2004) 21(2):137–48. doi: 10.1016/j.immuni.2004.07.017
35. Albertsmeier M, Quaiser D, von Dossow-Hanfstingl V, Winter H, Faist E, Angele MK. Major surgical trauma differentially affects T-cells and apc. Innate Immun. (2015) 21(1):55–64. doi: 10.1177/1753425913516659
36. Bartal I, Melamed R, Greenfeld K, Atzil S, Glasner A, Domankevich V, et al. Immune perturbations in patients along the perioperative period: alterations in cell surface markers and leukocyte subtypes before and after surgery. Brain Behav Immun. (2010) 24(3):376–86. doi: 10.1016/j.bbi.2009.02.010
37. Bremnes RM, Busund LT, Kilvær TL, Andersen S, Richardsen E, Paulsen EE, et al. The role of tumor-infiltrating lymphocytes in development, progression, and prognosis of non-small cell lung cancer. J Thorac Oncol. (2016) 11(6):789–800. doi: 10.1016/j.jtho.2016.01.015
38. Angka L, Martel AB, Kilgour M, Jeong A, Sadiq M, de Souza CT, et al. Natural killer cell Ifnγ secretion is profoundly suppressed following colorectal cancer surgery. Ann Surg Oncol. (2018) 25(12):3747–54. doi: 10.1245/s10434-018-6691-3
39. Aktaş ON, Öztürk AB, Erman B, Erus S, Tanju S, Dilege Ş. Role of natural killer cells in lung cancer. J Cancer Res Clin Oncol. (2018) 144(6):997–1003. doi: 10.1007/s00432-018-2635-3
40. Xu P, He H, Gu Y, Wang Y, Sun Z, Yang L, et al. Surgical trauma contributes to progression of colon cancer by downregulating CXCL4 and recruiting MDSCs. Exp Cell Res. (2018) 370(2):692–8. doi: 10.1016/j.yexcr.2018.07.035
41. Condamine T, Ramachandran I, Youn JI, Gabrilovich DI. Regulation of tumor metastasis by myeloid-derived suppressor cells. Annu Rev Med. (2015) 66:97–110. doi: 10.1146/annurev-med-051013-052304
42. Tang F, Tie Y, Hong W, Wei Y, Tu C, Wei X. Targeting myeloid-derived suppressor cells for premetastatic niche disruption after tumor resection. Ann Surg Oncol. (2021) 28(7):4030–48. doi: 10.1245/s10434-020-09371-z
43. Wang J, Su X, Yang L, Qiao F, Fang Y, Yu L, et al. The influence of myeloid-derived suppressor cells on angiogenesis and tumor growth after cancer surgery. Int J Cancer. (2016) 138(11):2688–99. doi: 10.1002/ijc.29998
44. Margraf A, Ludwig N, Zarbock A, Rossaint J. Systemic inflammatory response syndrome after surgery: mechanisms and protection. Anesth Analg. (2020) 131(6):1693–707. doi: 10.1213/ane.0000000000005175
45. Guner A, Kim HI. Biomarkers for evaluating the inflammation status in patients with cancer. J Gastric Cancer. (2019) 19(3):254–77. doi: 10.5230/jgc.2019.19.e29
46. Paruk F, Chausse JM. Monitoring the post surgery inflammatory host response. J Emerg Crit Care Med. (2019) 3:47.doi: 10.21037/jeccm.2019.08.06
47. Okada S, Shimomura M, Tsunezuka H, Teramukai S, Ishihara S, Shimada J, et al. Prognostic significance of perioperative C-reactive protein in resected non-small cell lung cancer. Semin Thorac Cardiovasc Surg. (2020) 32(4):1046–55. doi: 10.1053/j.semtcvs.2020.03.019
48. Shinohara S, Otsuki R, Onitsuka T, Machida K, Matsuo M, Nakagawa M, et al. Postoperative C-reactive protein is a predictive biomarker for survival after non-small cell lung cancer resection. Anticancer Res. (2019) 39(4):2193–8. doi: 10.21873/anticanres.13334
49. Pastorino U, Morelli D, Leuzzi G, Gisabella M, Suatoni P, Taverna F, et al. Baseline and postoperative C-reactive protein levels predict mortality in operable lung cancer. Eur J Cancer. (2017) 79:90–7. doi: 10.1016/j.ejca.2017.03.020
50. Hara M, Yonei A, Ayabe T, Tomita M, Nakamura K, Onitsuka T. Postoperative serum C-reactive protein levels in non-small cell lung cancer patients. Ann Thorac Cardiovasc Surg. (2010) 16(2):85–90.
51. Shinohara S, Sugaya M, Onitsuka T, Machida K, Matsuo M, Tanaka F. Prognostic impact of postoperative C-reactive protein for non-small cell lung cancer following lobectomy. Anticancer Res. (2018) 38(5):3193–8. doi: 10.21873/anticanres.12584
52. Jiang HG, Li J, Shi SB, Chen P, Ge LP, Jiang Q, et al. Value of fibrinogen and D-dimer in predicting recurrence and metastasis after radical surgery for non-small cell lung cancer. Med Oncol. (2014) 31(7):22. doi: 10.1007/s12032-014-0022-8
53. Jin Y, Zhao L, Peng F. Prognostic impact of Serum albumin levels on the recurrence of stage I non-small cell lung cancer. Clinics (Sao Paulo). (2013) 68(5):686–93. doi: 10.6061/clinics/2013(05)17
54. Hayasaka K, Shiono S, Suzuki K, Endoh M, Okada Y. Postoperative prognostic nutritional index as a prognostic factor after non-small cell lung cancer surgery. Gen Thorac Cardiovasc Surg. (2020) 68(10):1163–71. doi: 10.1007/s11748-020-01366-7
55. Jin F, Han A, Shi F, Kong L, Yu J. The postoperative neutrophil-to-lymphocyte ratio and changes in this ratio predict survival after the complete resection of stage I non-small cell lung cancer. Onco Targets Ther. (2016) 9:6529–37. doi: 10.2147/ott.S117290
56. Zhu J, Lian L, Qin H, Wang WJ, Ren R, Xu MD, et al. Prognostic evaluation of patients with resectable lung cancer using systemic inflammatory response parameters. Oncol Lett. (2019) 17(2):2244–56. doi: 10.3892/ol.2018.9858
57. Kita H, Shiraishi Y, Watanabe K, Suda K, Ohtsuka K, Koshiishi Y, et al. Does postoperative serum interleukin-6 influence early recurrence after curative pulmonary resection of lung cancer? Ann Thorac Cardiovasc Surg. (2011) 17(5):454–60. doi: 10.5761/atcs.oa.10.01627
58. Li J, Wang Z, Mao K, Guo X. Clinical significance of serum T helper 1/T helper 2 cytokine shift in patients with non-small cell lung cancer. Oncol Lett. (2014) 8(4):1682–6. doi: 10.3892/ol.2014.2391
59. Zhang N, Tan Q, Tao D, Song Y, Song W, Wang J, et al. Cytokines screening identifies mig (Cxcl9) for postoperative recurrence prediction in operated non-small cell lung cancer patients. Cytokine. (2022) 149:155759. doi: 10.1016/j.cyto.2021.155759
60. Jain S, Gautam V, Naseem S. Acute-phase proteins: as diagnostic tool. J Pharm Bioallied Sci. (2011) 3(1):118–27. doi: 10.4103/0975-7406.76489
61. Lin E, Calvano SE, Lowry SF. Inflammatory cytokines and cell response in surgery. Surgery. (2000) 127(2):117–26. doi: 10.1067/msy.2000.101584
62. Tan Z, Xue H, Sun Y, Zhang C, Song Y, Qi Y. The role of tumor inflammatory microenvironment in lung cancer. Front Pharmacol. (2021) 12:688625. doi: 10.3389/fphar.2021.688625
63. Oda R, Okuda K, Osaga S, Watanabe T, Sakane T, Tatematsu T, et al. Long-term outcomes of video-assisted thoracoscopic surgery lobectomy vs. thoracotomy lobectomy for stage ia non-small cell lung cancer. Surg Today. (2019) 49(5):369–77. doi: 10.1007/s00595-018-1746-4
64. Batihan G, Ceylan KC, Usluer O, Kaya ŞÖ. Video-assisted thoracoscopic surgery vs thoracotomy for non-small cell lung cancer greater than 5 cm: is vats a feasible approach for large tumors? J Cardiothorac Surg. (2020) 15(1):261. doi: 10.1186/s13019-020-01305-w
65. Yun JK, Lee GD, Choi S, Kim HR, Kim Y-H, Park S-I, et al. Video-assisted thoracoscopic lobectomy is feasible for selected patients with clinical N2 non-small cell lung cancer. Sci Rep. (2020) 10(1):15217. doi: 10.1038/s41598-020-72272-4
66. Su S, Scott WJ, Allen MS, Darling GE, Decker PA, McKenna RJ, et al. Patterns of survival and recurrence after surgical treatment of early stage non–small cell lung carcinoma in the acosog Z0030 (alliance) trial. J Thorac Cardiovasc Surg. (2014) 147(2):747–53. doi: 10.1016/j.jtcvs.2013.10.001
67. Yang CJ, Kumar A, Deng JZ, Raman V, Lui NS, D’Amico TA, et al. A national analysis of short-term outcomes and long-term survival following thoracoscopic versus open lobectomy for clinical stage ii non-small-cell lung cancer. Ann Surg. (2021) 273(3):595–605. doi: 10.1097/sla.0000000000003231
68. Yang CJ, Kumar A, Klapper JA, Hartwig MG, Tong BC, Harpole DH Jr., et al. A national analysis of long-term survival following thoracoscopic versus open lobectomy for stage I non-small-cell lung cancer. Ann Surg. (2019) 269(1):163–71. doi: 10.1097/sla.0000000000002342
69. Oh TK, Kim K, Jheon S, Lee J, Do S-H, Hwang J-W, et al. Long-term oncologic outcomes for patients undergoing volatile versus intravenous anesthesia for non-small cell lung cancer surgery. Cancer Control. (2018) 25(1):107327481877536. doi: 10.1177/1073274818775360
70. Cata JP, Singh V, Lee BM, Villarreal J, Mehran JR, Yu J, et al. Intraoperative use of dexmedetomidine is associated with decreased overall survival after lung cancer surgery. J Anaesthesiol Clin Pharmacol. (2017) 33(3):317–23. doi: 10.4103/joacp.JOACP_299_16
71. Connolly JG, Tan KS, Mastrogiacomo B, Dycoco J, Caso R, Jones GD, et al. Intraoperative opioid exposure, tumour genomic alterations, and survival differences in people with lung adenocarcinoma. Br J Anaesth. (2021) 127(1):75–84. doi: 10.1016/j.bja.2021.03.030
72. Maher DP, Wong W, White PF, McKenna R, Rosner H, Shamloo B, et al. Association of increased postoperative opioid administration with non-small-cell lung cancer recurrence: a retrospective analysis. Br J Anaesth. (2014) 113(suppl 1):i88–94. doi: 10.1093/bja/aeu192
73. Cata JP, Keerty V, Keerty D, Feng L, Norman PH, Gottumukkala V, et al. A retrospective analysis of the effect of intraoperative opioid dose on cancer recurrence after non-small cell lung cancer resection. Cancer Med. (2014) 3(4):900–8. doi: 10.1002/cam4.236
74. Oh TK, Jeon JH, Lee JM, Kim MS, Kim JH, Cho H, et al. Investigation of opioid use and long-term oncologic outcomes for non-small cell lung cancer patients treated with surgery. PLoS ONE. (2017) 12(7):e0181672. doi: 10.1371/journal.pone.0181672
75. Jiang W, Wang L, Zhang J, Shen H, Dong W, Zhang T, et al. Effects of postoperative non-steroidal anti-inflammatory drugs on long-term survival and recurrence of patients with non-small cell lung cancer. Medicine (Baltimore). (2018) 97(39):e12442. doi: 10.1097/md.0000000000012442
76. Choi JE, Villarreal J, Lasala J, Gottumukkala V, Mehran RJ, Rice D, et al. Perioperative neutrophil:lymphocyte ratio and postoperative nsaid use as predictors of survival after lung cancer surgery: a retrospective study. Cancer Med. (2015) 4(6):825–33. doi: 10.1002/cam4.428
77. Xu Z-Z, Li H-J, Li M-H, Huang S-M, Li X, Liu Q-H, et al. Epidural anesthesia–analgesia and recurrence-free survival after lung cancer surgery: a randomized trial. Anesthesiology. (2021) 135(3):419–32. doi: 10.1097/aln.0000000000003873
78. Rosboch GL, Ceraolo E, De Domenici I, Guerrera F, Balzani E, Lyberis P, et al. Impact of analgesia on cancer recurrence and mortality within 5 years after stage I non-small cell lung cancer resection. Tumori J. (2021):030089162110200. doi: 10.1177/03008916211020093
79. Lee EK, Ahn HJ, Zo JI, Kim K, Jung DM, Park JH. Paravertebral block does not reduce cancer recurrence, but is related to higher overall survival in lung cancer surgery: a retrospective cohort study. Anesth Analg. (2017) 125(4):1322–8. doi: 10.1213/ane.0000000000002342
80. Tai YH, Wu HL, Mandell MS, Lin SP, Tsou MY, Chang KY. The association of non-small cell lung cancer recurrence with allogenic blood transfusion after surgical resection: a propensity score analysis of 1,803 patients. Eur J Cancer. (2020) 140:45–54. doi: 10.1016/j.ejca.2020.09.004
81. Wang T, Luo L, Huang H, Yu J, Pan C, Cai X, et al. Perioperative blood transfusion is associated with worse clinical outcomes in resected lung cancer. Ann Thorac Surg. (2014) 97(5):1827–37. doi: 10.1016/j.athoracsur.2013.12.044
82. Luan H, Ye F, Wu L, Zhou Y, Jiang J. Perioperative blood transfusion adversely affects prognosis after resection of lung cancer: a systematic review and a meta-analysis. BMC Surg. (2014) 14:34. doi: 10.1186/1471-2482-14-34
83. Latif MJ, Tan KS, Molena D, Huang J, Bott MJ, Park BJ, et al. Perioperative blood transfusion has a dose-dependent relationship with disease recurrence and survival in patients with non–small cell lung cancer. J Thorac Cardiovasc Surg. (2019) 157(6):2469–77. doi: e10. doi: 10.1016/j.jtcvs.2018.12.109
84. Neff TA, Braun J, Rana D, Puhan M, Filipovic M, Seeberger M, et al. Interleukin-6 is an early plasma marker of severe postoperative complications in thoracic surgery: exploratory results from a substudy of a randomized controlled multicenter trial. Anesth Analg. (2022) 134(1):123–32. doi: 10.1213/ane.0000000000005639
85. Zhang LB, Wang B, Wang XY, Zhang L. Influence of video-assisted thoracoscopic lobectomy on immunological functions in non-small cell lung cancer patients. Med Oncol. (2015) 32(7):201. doi: 10.1007/s12032-015-0639-2
86. Walker WS, Leaver HA. Immunologic and stress responses following video-assisted thoracic surgery and open pulmonary lobectomy in early stage lung cancer. Thorac Surg Clin. (2007) 17(2):241–9, ix. doi: 10.1016/j.thorsurg.2007.04.001
87. Sugi K, Kaneda Y, Esato K. Video-assisted thoracoscopic lobectomy reduces cytokine production more than conventional open lobectomy. Jpn J Thorac Cardiovasc Surg. (2000) 48(3):161–5. doi: 10.1007/bf03218114
88. Jones RO, Anderson NH, Murchison JT, Brittan M, Simon EJ, Casali G, et al. Innate immune responses after resection for lung cancer via video-assisted thoracoscopic surgery and thoracotomy. Innovations (Phila). (2014) 9(2):93–103; discussion. doi: 10.1097/imi.0000000000000061
89. Whitson BA, D’Cunha J, Andrade RS, Kelly RF, Groth SS, Wu B, et al. Thoracoscopic versus thoracotomy approaches to lobectomy: differential impairment of cellular immunity. Ann Thorac Surg. (2008) 86(6):1735–44. doi: 10.1016/j.athoracsur.2008.07.001
90. Craig SR, Leaver HA, Yap PL, Pugh GC, Walker WS. Acute phase responses following minimal access and conventional thoracic surgery. Eur J Cardiothorac Surg. (2001) 20(3):455–63. doi: 10.1016/s1010-7940(01)00841-7
91. Leaver HA, Craig SR, Yap PL, Walker WS. Lymphocyte responses following open and minimally invasive thoracic surgery. Eur J Clin Invest. (2000) 30(3):230–8. doi: 10.1046/j.1365-2362.2000.00622.x
92. Tavare AN, Perry NJ, Benzonana LL, Takata M, Ma D. Cancer recurrence after surgery: direct and indirect effects of anesthetic agents. Int J Cancer. (2012) 130(6):1237–50. doi: 10.1002/ijc.26448
93. Benzonana LL, Perry NJ, Watts HR, Yang B, Perry IA, Coombes C, et al. Isoflurane, a commonly used volatile anesthetic, enhances renal cancer growth and malignant potential via the hypoxia-inducible factor cellular signaling pathway in vitro. Anesthesiology. (2013) 119(3):593–605. doi: 10.1097/ALN.0b013e31829e47fd
94. Loop T, Scheiermann P, Doviakue D, Musshoff F, Humar M, Roesslein M, et al. Sevoflurane inhibits phorbol-myristate-acetate-induced activator protein-1 activation in human T lymphocytes in vitro: potential role of the P38-stress kinase pathway. Anesthesiology. (2004) 101(3):710–21. doi: 10.1097/00000542-200409000-00020
95. Matsuoka H, Kurosawa S, Horinouchi T, Kato M, Hashimoto Y. Inhalation anesthetics induce apoptosis in normal peripheral lymphocytes in vitro. Anesthesiology. (2001) 95(6):1467–72. doi: 10.1097/00000542-200112000-00028
96. Jeon S, Kim HK, Kwon JY, Baek SH, Ri HS, Choi HJ, et al. Role of sevoflurane on natural killer group 2, member D-mediated immune response in non-small-cell lung cancer: an in vitro study. Med Sci Monit. (2020) 26:e926395. doi: 10.12659/msm.926395
97. Chen RM, Chen TG, Chen TL, Lin LL, Chang CC, Chang HC, et al. Anti-inflammatory and antioxidative effects of propofol on lipopolysaccharide-activated macrophages. Ann N Y Acad Sci. (2005) 1042:262–71. doi: 10.1196/annals.1338.030
98. Ma L, Wu XY, Zhang LH, Chen WM, Uchiyama A, Mashimo T, et al. Propofol exerts anti-inflammatory effects in rats with lipopolysaccharide-induced acute lung injury by inhibition of Cd14 and Tlr4 expression. Braz J Med Biol Res. (2013) 46(3):299–305. doi: 10.1590/1414-431x20122379
99. Ma L, Wu X, Chen W, Fujino Y. Propofol has anti-inflammatory effects on alveolar type II epithelial cells. Acta Anaesthesiol Scand. (2010) 54(3):362–9. doi: 10.1111/j.1399-6576.2009.02127.x
100. Melamed R, Bar-Yosef S, Shakhar G, Shakhar K, Ben-Eliyahu S. Suppression of natural killer cell activity and promotion of tumor metastasis by ketamine, thiopental, and halothane, but not by propofol: mediating mechanisms and prophylactic measures. Anesth Analg. (2003) 97(5):1331–9. doi: 10.1213/01.Ane.0000082995.44040.07
101. Ackerman RS, Luddy KA, Icard BE, Piñeiro Fernández J, Gatenby RA, Muncey AR. The effects of anesthetics and perioperative medications on immune function: a narrative review. Anesth Analg. (2021) 133(3):676–89. doi: 10.1213/ane.0000000000005607
102. Yuan JL, Kang K, Li B, Lu J, Miao MR, Kang X, et al. The effects of sevoflurane vs. propofol for inflammatory responses in patients undergoing lung resection: a meta-analysis of randomized controlled trials. Front Surg. (2021) 8:692734. doi: 10.3389/fsurg.2021.692734
103. Xu Q, Shi N-J, Zhang H, Zhu Y-M. Effects of combined general-epidural anesthesia and total intravenous anesthesia on cellular immunity and prognosis in patients with non-small cell lung cancer: a comparative study. Mol Med Rep. (2017) 16(4):4445–54. doi: 10.3892/mmr.2017.7144
104. Sen Y, Xiyang H, Yu H. Effect of thoracic paraspinal block-propofol intravenous general anesthesia on vegf and Tgf-β in patients receiving radical resection of lung cancer. Medicine (Baltimore). (2019) 98(47):e18088. doi: 10.1097/md.0000000000018088
105. Azuma Y, Ohura K. Immunological modulation by lidocaine-epinephrine and prilocaine-felypressin on the functions related to natural immunity in neutrophils and macrophages. Curr Drug Targets Immune Endocr Metabol Disord. (2004) 4(1):29–36. doi: 10.2174/1568008043339974
106. Chamaraux-Tran TN, Piegeler T. The amide local anesthetic lidocaine in cancer surgery-potential antimetastatic effects and preservation of immune cell function? A Narrative Review. Front Med (Lausanne). (2017) 4:235. doi: 10.3389/fmed.2017.00235
107. Piegeler T, Schläpfer M, Dull RO, Schwartz DE, Borgeat A, Minshall RD, et al. Clinically relevant concentrations of lidocaine and ropivacaine inhibit tnfα-induced invasion of lung adenocarcinoma cells in vitro by blocking the activation of Akt and focal adhesion kinase. Br J Anaesth. (2015) 115(5):784–91. doi: 10.1093/bja/aev341
108. Piegeler T, Votta-Velis EG, Liu G, Place AT, Schwartz DE, Beck-Schimmer B, et al. Antimetastatic potential of amide-linked local anesthetics: inhibition of lung adenocarcinoma cell migration and inflammatory Src signaling independent of sodium channel blockade. Anesthesiology. (2012) 117(3):548–59. doi: 10.1097/ALN.0b013e3182661977
109. Garutti I, Rancan L, Simón C, Cusati G, Sanchez-Pedrosa G, Moraga F, et al. Intravenous lidocaine decreases tumor necrosis factor alpha expression both locally and systemically in pigs undergoing lung resection surgery. Anesth Analg. (2014) 119(4):815–28. doi: 10.1213/ane.0000000000000360
110. Hou Y-H, Shi W-C, Cai S, Liu H, Zheng Z, Qi F-W, et al. Effect of intravenous lidocaine on serum interleukin-17 after video-assisted thoracic surgery for non-small-cell lung cancer: a randomized, double-blind, placebo-controlled trial. Drug Des Devel Ther. (2021) Volume 15:3379–90. doi: 10.2147/dddt.s316804
111. Batchelor TJP, Rasburn NJ, Abdelnour-Berchtold E, Brunelli A, Cerfolio RJ, Gonzalez M, et al. Guidelines for enhanced recovery after lung surgery: recommendations of the enhanced recovery after surgery (Eras®) society and the European society of thoracic surgeons (ests). Eur J Cardiothorac Surg. (2019) 55(1):91–115. doi: 10.1093/ejcts/ezy301
112. Cata JP, Guerra C, Soto G, Ramirez MF. Anesthesia options and the recurrence of cancer: what we know so far? Local Reg Anesth. (2020) 13:57–72. doi: 10.2147/lra.S240567
113. Hahnenkamp K, Herroeder S, Hollmann MW. Regional anaesthesia, local anaesthetics and the surgical stress response. Best Pract Res Clin Anaesthesiol. (2004) 18(3):509–27. doi: 10.1016/j.bpa.2004.01.004
114. Okuda J, Suzuki T, Wakaizumi K, Kato J, Yamada T, Morisaki H. Effects of thoracic epidural anesthesia on systemic and local inflammatory responses in patients undergoing lung cancer surgery: a randomized controlled trial. J Cardiothorac Vasc Anesth. (2022) 36(5):1380–6. doi: 10.1053/j.jvca.2021.08.026
115. Fu S, Qu P-S, Cai S-N. Effect of anesthetic methods on postoperative Cd3+, Cd4+ and Cd4 + Cd25 + in patients with lung cancer undergoing radical operation. Oncol Lett. (2018) 16(5):6547–51. doi: 10.3892/ol.2018.9416
116. Lohser J, Slinger P. Lung injury after one-lung ventilation: a review of the pathophysiologic mechanisms affecting the ventilated and the collapsed lung. Anesth Analg. (2015) 121(2):302–18. doi: 10.1213/ane.0000000000000808
117. Jeon J, Sung S, Moon Y, Koo J, Hyun K, Han K, et al. Comparison of early postoperative cytokine changes in patients undergoing intubated and non-intubated thoracic surgery: a randomized controlled trial. Interact Cardiovasc Thorac Surg. (2021) 32(3):343–50. doi: 10.1093/icvts/ivaa265
118. Ali JM, Volpi S, Kaul P, Aresu G. Does the “non-intubated” anaesthetic technique offer any advantage for patients undergoing pulmonary lobectomy? Interact Cardiovasc Thorac Surg. (2019) 28(4):555–8. doi: 10.1093/icvts/ivy312
119. Zhang K, Chen HG, Wu WB, Li XJ, Wu YH, Xu JN, et al. Non-intubated video-assisted thoracoscopic surgery vs. intubated video-assisted thoracoscopic surgery for thoracic disease: a systematic review and meta-analysis of 1,684 cases. J Thorac Dis. (2019) 11(8):3556–68. doi: 10.21037/jtd.2019.07.48
120. AlGhamdi ZM, Lynhiavu L, Moon YK, Moon MH, Ahn S, Kim Y, et al. Comparison of non-intubated versus intubated video-assisted thoracoscopic lobectomy for lung cancer. J Thorac Dis. (2018) 10(7):4236–43. doi: 10.21037/jtd.2018.06.163
121. Tacconi F, Pompeo E, Sellitri F, Mineo TC. Surgical stress hormones response is reduced after awake videothoracoscopy⋆. Interact Cardiovasc Thorac Surg. (2010) 10(5):666–71. doi: 10.1510/icvts.2009.224139
122. Vanni G, Tacconi F, Sellitri F, Ambrogi V, Mineo TC, Pompeo E. Impact of awake videothoracoscopic surgery on postoperative lymphocyte responses. Ann Thorac Surg. (2010) 90(3):973–8. doi: 10.1016/j.athoracsur.2010.04.070
123. Mineo T, Sellitri F, Vanni G, Gallina F, Ambrogi V. Immunological and inflammatory impact of non-intubated lung metastasectomy. Int J Mol Sci. (2017) 18(7):1466. doi: 10.3390/ijms18071466
124. Eisenstein TK. The role of opioid receptors in immune system function. Front Immunol. (2019) 10:2904. doi: 10.3389/fimmu.2019.02904
125. Plein LM, Rittner HL. Opioids and the immune system - friend or foe. Br J Pharmacol. (2018) 175(14):2717–25. doi: 10.1111/bph.13750
126. Kraus J. Regulation of mu-opioid receptors by cytokines. Front Biosci (Schol Ed). (2009) 1:164–70. doi: 10.2741/s16
127. Ding M, Chen Y, Luan H, Zhang X, Zhao Z, Wu Y. Dexmedetomidine reduces inflammation in traumatic brain injury by regulating the inflammatory responses of macrophages and splenocytes. Exp Ther Med. (2019) 18(3):2323–31. doi: 10.3892/etm.2019.7790
128. Chen Y, Bian W, Xu B. Pretreatment with dexmedetomidine alleviates lung injury in a rat model of intestinal ischemia reperfusion. Mol Med Rep. (2020) 21(3):1233–41. doi: 10.3892/mmr.2020.10942
129. Xie Y, Jiang W, Zhao L, Wu Y, Xie H. Effect of dexmedetomidine on perioperative inflammation and lung protection in elderly patients undergoing radical resection of lung cancer. Int J Clin Exp Pathol. (2020) 13(10):2544–53.
130. Wu CY, Lu YF, Wang ML, Chen JS, Hsu YC, Yang FS, et al. Effects of dexmedetomidine infusion on inflammatory responses and injury of lung tidal volume changes during one-lung ventilation in thoracoscopic surgery: a randomized controlled trial. Mediators Inflamm. (2018) 2018:2575910. doi: 10.1155/2018/2575910
131. Su X, Fan Y, Yang L, Huang J, Qiao F, Fang Y, et al. Dexmedetomidine expands monocytic myeloid-derived suppressor cells and promotes tumour metastasis after lung cancer surgery. J Transl Med. (2018) 16(1):347. doi: 10.1186/s12967-018-1727-9
132. Lavon H, Matzner P, Benbenishty A, Sorski L, Rossene E, Haldar R, et al. Dexmedetomidine promotes metastasis in rodent models of breast, lung, and colon cancers. Br J Anaesth. (2018) 120(1):188–96. doi: 10.1016/j.bja.2017.11.004
133. Khan S, Andrews KL, Chin-Dusting JPF. Cyclo-oxygenase (cox) inhibitors and cardiovascular risk: are non-steroidal anti-inflammatory drugs really anti-inflammatory? Int J Mol Sci. (2019) 20(17):4262. doi: 10.3390/ijms20174262
134. Sandler AB, Dubinett SM. Cox-2 inhibition and lung cancer. Semin Oncol. (2004) 31(2 Suppl 7):45–52. doi: 10.1053/j.seminoncol.2004.03.045
135. Bacchi S, Palumbo P, Sponta A, Coppolino MF. Clinical pharmacology of non-steroidal anti-inflammatory drugs: a review. Antiinflamm Antiallergy Agents Med Chem. (2012) 11(1):52–64. doi: 10.2174/187152312803476255
136. Baumgartner JM, Nydam TL, Clarke JH, Banerjee A, Silliman CC, McCarter MD. Red blood cell supernatant potentiates lps-induced proinflammatory cytokine response from peripheral blood mononuclear cells. J Interferon Cytokine Res. (2009) 29(6):333–8. doi: 10.1089/jir.2008.0072
137. Olofsson KE, Andersson L, Nilsson J, Björkbacka H. Nanomolar concentrations of lysophosphatidylcholine recruit monocytes and induce pro-inflammatory cytokine production in macrophages. Biochem Biophys Res Commun. (2008) 370(2):348–52. doi: 10.1016/j.bbrc.2008.03.087
138. Jacobi KE, Wanke C, Jacobi A, Weisbach V, Hemmerling TM. Determination of eicosanoid and cytokine production in salvaged blood, stored red blood cell concentrates, and whole blood. J Clin Anesth. (2000) 12(2):94–9. doi: 10.1016/s0952-8180(00)00122-7
139. Du Y-T, Li Y-W, Zhao B-J, Guo X-Y, Feng Y, Zuo M-Z, et al. Long-term survival after combined epidural–general anesthesia or general anesthesia alone: follow-up of a randomized trial. Anesthesiology. (2021) 135(2):233–45. doi: 10.1097/aln.0000000000003835
140. Dubowitz JA, Cata JP, De Silva AP, Braat S, Shan D, Yee K, et al. Volatile anaesthesia and peri-operative outcomes related to cancer: a feasibility and pilot study for a large randomised control trial. Anaesthesia. (2021) 76(9):1198–206. doi: 10.1111/anae.15354
141. Schilling T, Kozian A, Huth C, Bühling F, Kretzschmar M, Welte T, et al. The pulmonary immune effects of mechanical ventilation in patients undergoing thoracic surgery. Anesth Analg. (2005) 101(4):957–65. doi: 10.1213/01.ane.0000172112.02902.77
142. Coutu BG, Johnson KC, Bhirud A, Baine MJ, Zhen W, Zhang C, et al. Systemic immune-inflammatory Index association with survival in patients undergoing trimodality therapy for lung cancer. Oncology. (2022) 100(5):247–56. doi: 10.1159/000520989
143. Endo S, Sato Y, Hasegawa T, Tetsuka K, Otani S, Saito N, et al. Preoperative chemotherapy increases cytokine production after lung cancer surgery. Eur J Cardiothorac Surg. (2004) 26(4):787–91. doi: 10.1016/j.ejcts.2004.07.015
Keywords: inflammation, lung cancer, neoplasm metastasis, neoplasm recurrence, perioperative management
Citation: Choi H and Hwang W (2022) Perioperative Inflammatory Response and Cancer Recurrence in Lung Cancer Surgery: A Narrative Review. Front. Surg. 9:888630. doi: 10.3389/fsurg.2022.888630
Received: 3 March 2022; Accepted: 24 June 2022;
Published: 11 July 2022.
Edited by:
Federico Tacconi, University of Rome Tor Vergata, ItalyReviewed by:
Francesco Zaraca, Ospedale di Bolzano, ItalyYuwei Qiu, Shanghai Jiao Tong University, China
Copyright © 2022 Choi and Hwang. This is an open-access article distributed under the terms of the Creative Commons Attribution License (CC BY). The use, distribution or reproduction in other forums is permitted, provided the original author(s) and the copyright owner(s) are credited and that the original publication in this journal is cited, in accordance with accepted academic practice. No use, distribution or reproduction is permitted which does not comply with these terms.
*Correspondence: Wonjung Hwang YW1vZWJhNzlAY2F0aG9saWMuYWMua3I=
Specialty section: This article was submitted to Thoracic Surgery, a section of the journal Frontiers in Surgery